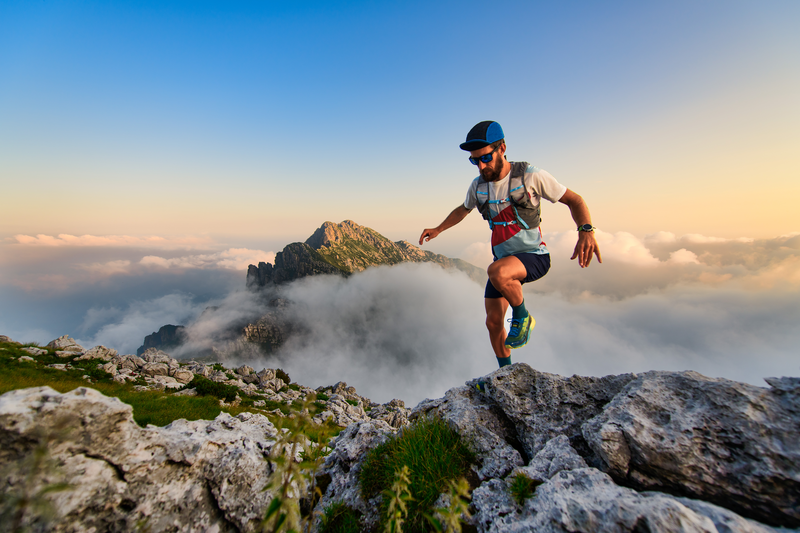
94% of researchers rate our articles as excellent or good
Learn more about the work of our research integrity team to safeguard the quality of each article we publish.
Find out more
MINI REVIEW article
Front. Mar. Sci. , 24 May 2019
Sec. Marine Megafauna
Volume 6 - 2019 | https://doi.org/10.3389/fmars.2019.00262
This article is part of the Research Topic Future Directions in Research on Marine Megafauna View all 20 articles
Traditional ecological research has focused on taxonomic units to better understand the role of organisms in marine ecosystems. This approach has significantly contributed to our understanding of how species interact with each other and with the physical environment and has led to relevant site-specific conservation strategies. However, this taxonomic-based approach can limit a mechanistic understanding of how environmental change affects marine megafauna, here defined as large fishes (e.g., shark, tuna, and billfishes), sea turtles, marine mammals, and seabirds. Alternatively, an approach based on traits, i.e., measurable behavioral, physiological, or morphological characteristics of organisms, can shed new light on the processes influencing structure and functions of biological communities. Here we review 33 traits that are measurable and comparable among marine megafauna. The variability of these traits within the organisms considered controls functions mainly related to nutrient storage and transport, trophic-dynamic regulations of populations, and community shaping. To estimate the contributions of marine megafauna to ecosystem functions and services, traits can be quantified categorically or over a continuous scale, but the latter is preferred to make comparisons across groups. We argue that the most relevant traits to comparatively study marine megafauna groups are body size, body mass, dietary preference, feeding strategy, metabolic rate, and dispersal capacity. These traits can be used in combination with information on population abundances to predict how changes in the environment can affect community structure, ecosystem functioning, and ecosystem services.
Most of the traditional research on the conservation of biological diversity focuses on species identities and on how their numbers and abundances change in space and time (Rosenzweig, 1995). Observations on biodiversity and ecosystem functioning relationships suggest, for example, that as species richness increases, the productivity and stability of communities also increase (Tilman, 2001; Tilman et al., 2014). It has been argued, however, that a focus on taxonomic units (e.g., species or genus) alone is not sufficient to predict the effects of environmental change on biological communities and their ecosystem functions (Díaz and Cabido, 2001; McGill et al., 2006; Violle et al., 2007). Species that go extinct can be replaced by species with similar traits and functions (Violle et al., 2014), and intraspecific trait variability (i.e., variability among individuals due to phenotypic plasticity or genetic differences) can be as broad as trait variations across species (Des Roches et al., 2017; Messier et al., 2010; Violle et al., 2012).
A trait-based perspective to community ecology thus resurfaced as a potential approach to enhance our mechanistic understanding of how structure and functions of communities vary along environmental gradients (McGill et al., 2006). Central to this perspective are traits, which are morphological, physiological, or behavioral features of organisms that can be quantified at different organizational levels, from individuals to ecosystems (McGill et al., 2006; Violle et al., 2007). Changes in habitat can strongly affect trait distributions and coexistence because organisms sharing traits that favor habitat occupancy are likely to persist in a given community, whereas those with poorly adapted traits are likely to disappear (Luck et al., 2013). Thus, traits can help us understand and quantify niche occupancy (Violle et al., 2007). Also, the traits that define the fitness of organisms are closely related to ecosystem functions. For example, a seabird behavioral trait such as migration ability can be related to functions like nutrient transport (Doughty et al., 2016).
Trait-based approaches are being promoted for studying community structure and functions of various groups of organisms, including terrestrial plants (Kattge et al., 2011; Kraft et al., 2015), phytoplankton (Litchman and Klausmeier, 2008; Acevedo-Trejos et al., 2018), zooplankton (Litchman et al., 2013) corals (Madin et al., 2016), mammals (Jones et al., 2009), fishes (Stuart-Smith et al., 2013; Ladds et al., 2018), and microbes (Krause et al., 2014). This approach is also fostering global collaborative efforts through the establishment of open trait databases (Jones et al., 2009; Kattge et al., 2011; Parr et al., 2014; Wilman et al., 2014; Kremer et al., 2017). However, the lack of a consistent trait-based framework for the study of marine megafauna, here comprising large fishes (e.g., billfishes, tuna, and sharks), sea turtles, marine mammals (i.e., pinnipeds, sirenians, and cetaceans) and seabirds, prevents a mechanistic understanding of the effects that changes in diversity can have on ecosystem functioning, a challenge still difficult to address for many aquatic communities (Meunier et al., 2017; Degen et al., 2018). Also, marine megafauna has been largely affected by mortality related to bycatch, various forms of pollution, overfishing, habitat degradation, and climate variability, problems that are causing population declines and loss of functional diversity at a global scale (Croxall et al., 2012; Albouy et al., 2017; Pimiento et al., 2017). Finally, the decline of populations within marine megafauna communities can reduce functional diversity, but the impacts on ecosystem functioning are far from being understood (Naeem et al., 2012; Lynam et al., 2017).
Here we list, categorize and describe relevant traits shared by communities of large fishes, sea turtles, marine mammals, and seabirds to guide further investigations and comparisons over these organisms. We then highlight the relationships between these traits and ecosystem functions and services relevant for studying the community ecology and the conservation of marine megafauna.
We listed traits of marine megafauna and the associated ecosystem functions and services based on a systematic review of scientific articles published over the last 15 years and up to March 2018. We performed the literature search in Web of Science for the terms: (1) seabird + trait; (2) marine mammal + trait; (3) dolphin + trait; (4) whale + trait; (5) seal + trait; (6) sirenian + trait; (7) manatee + trait; (8) sea turtle + trait; (9) fish + trait; (10) shark + trait; and (11) marine megafauna + trait. From the resulting list of articles, we selected those that clearly dealt with traits of marine megafauna. After an initial screening of potential traits, we defined the traits terminology based on specialized literature (Spitz et al., 2014; Costello et al., 2015; Albouy et al., 2017; Beauchard et al., 2017; Ladds et al., 2018).
Traits of marine megafauna are measurable behavioral, physiological, or morphological characteristics of sea turtles, large fishes, marine mammals, and seabirds. Functional traits are those that can affect the performance of organisms and their ecosystem functions (McGill et al., 2006). Examples include body mass, locomotion mode, feeding strategy, and life span. Functional diversity comprises the diversity of functional traits (Mason and Mouillot, 2013). Ecosystem functions encompass vital activities of organisms, including feeding, growing, moving, and excreting, and influence ecosystem functioning (DeLaplante and Picasso, 2011). Ecosystem services are defined as functions that provide goods to humans (Costanza et al., 1997; Cardinale et al., 2012; Mace et al., 2012).
We identified a total of 33 traits that can be measured and used comparably over sea turtles, large fishes, marine mammals, and seabirds (Table 1). These traits are classified into conceptual categories related to morphology, behavior, demography, physiology, biogeochemical composition, and socioeconomic importance. Characteristics such as taxonomic family, common names, IUCN threat categories can be also found in the literature (e.g., Costello et al., 2015), but were not considered here since they are derived from other traits and constitute imprecise or redundant information. We also did not consider traits related to the size of body parts because they typically scale with body size, and a number of them are not comparable (or display different functions) across different groups of marine megafauna (e.g., bill culmen, toes, size of legs, dorsal, and caudal fins). Furthermore, a trait such as diving depth can be quantified in many ways, including, for example, minimum, maximum, or average diving depth, but to avoid redundancy, here we considered average diving depth, diving duration, and diving profile (Gleiss et al., 2011; Meir et al., 2013; Ciancio et al., 2016).
Due to allometric effects, body size and body mass constitute key traits because they are correlated with many other morphological, physiological, and behavioral characteristics. Size of jaws, mobility, feeding distance, incubation time, life-span, recruiting rate, growth, metabolic rate, and excretion rate all scale with body size and body mass (Schreiber and Burger, 2002; Louzao et al., 2008; Froy and Richard, 2013; Fraija-Fernández et al., 2015; Nunes et al., 2017). This property makes body size useful for reducing the dimensionality of the broad trait space. Body size also holds the potential to describe marine life from bacteria to whales, to help overcome some of the limitations inherent to taxonomic-based studies, and to understand variability in functional diversity over very distinct classes of organisms and environmental gradients (Andersen et al., 2016; Blanchard et al., 2017). Body size and body mass are also very useful to predict organismal vital functions (e.g., metabolic rates: West et al., 2002), and their contributions to ecosystem functioning.
Metabolic theory predicts how body size scales with metabolic rate (Brown et al., 2004). However, variability can be observed across different groups of marine megafauna, due to differences in diet composition (Jobling, 1981; Lutcavage and Lutz, 1986; McNab, 1988; Williams et al., 2001). Carnivore killer whales Orcinus orca display metabolic rates of 20–30 mL O2 kg-1 min-1, substantially higher than filter-feeding sharks of similar sizes, such as the basking shark Cetorhinus maximus which the metabolic rate is estimated at around 0.01 mL O2 kg-1 min-1 (Sims, 2000; Guinet et al., 2007). For example, the carnivorous bottlenose dolphin Tursiops truncatus can exhibit a basal metabolic rate (10.12 mL O2 kg-1 min-1), which is two times higher than the one of the omnivorous leatherback sea turtle Dermochelys coriacea (4.77 mL O2 kg-1 min-1), although they both have similar body size and mass (Lutcavage and Lutz, 1986; Williams et al., 2001). Also, sea turtles feed by pursuing preys through the water column and low metabolic rates are a consequence of the long dives and of the fact that increased metabolic rates in ectoderms impose energetic imbalances (Butler et al., 1984; Lutz and Musick, 1996; Fossette et al., 2012). To better predict the effects of organisms on ecosystem functions and services, body size should, therefore, be used in combination with traits that shape the fundamental ecology of marine megafauna, including thermal tolerance (Pimiento et al., 2017), metabolic and food intakes rates (Woodward et al., 2005), and dietary preference (Houle et al., 2016).
Phenotypic trait variation within species (intraspecific variation) can be as broad as trait variation across species of fishes, sea turtles, marine mammals, and birds (Albert et al., 2010; McClain et al., 2015; Samarra et al., 2017). Total length in basking sharks, for example, ranges between 1.5 and 10 m (McClain et al., 2015). However, food web models usually rely on the biomass of organisms, which is inferred using values of body mass averaged within species level (Costa et al., 2017). This approach may overlook a substantial variation in body mass across individuals of the same species. Finding new approaches for addressing intraspecific variations in food web models can lead to improved predictions of nutrient and energy fluxes via trophic interactions and ecosystem stability. Efforts are being made to build databases that includes trait values within species of marine megafauna (Petchey et al., 2008; Wilman et al., 2014; McClain et al., 2015; Des Roches et al., 2017), but information on intraspecific trait variation is fragmented and species-specific.
A large body of research on the trait ecology of marine megafauna is based on categorical traits because these properties are straightforward to measure (Costello et al., 2015; Albouy et al., 2017). However, the qualitative nature of categorical traits can limit comparisons between different groups of organisms (McGill et al., 2006). Regarding the level of sociability, for example, an organism can be classified as gregarious, colonial, solitary, or even in intermediary categories (Costello et al., 2015). For sharks, dolphins, and whales, the term gregarious can refer to small groups of 5 to 200 individuals (Mann et al., 2000; Baird, 2009; Crowe et al., 2018), but for colonial seabirds, the term refers to thousands of individuals (Schreiber and Burger, 2002; Jovani et al., 2008). Alternatively, traits estimated quantitatively can be standardized and compared across different groups of organisms, and potential trade-offs can be explored (Genovart et al., 2013; Shoji et al., 2015; Gravel et al., 2016; Yamamoto et al., 2016). Some traits, such as body size, can be easily quantified, but others cannot. Preferred diet, for example, is largely treated as a categorical trait and categories usually include zooplankton, invertebrate, and fish (Albouy et al., 2017). Categories of food items can be standardized into semiquantitative information by considering their relative importance (Wilman et al., 2014). Furthermore, an increasing amount of numerical data for dozens of megafauna species can be freely downloaded from the COMADRE Animal Matrix Database1. Compiled information on morphological, behavioral, physiological, and demographical traits of marine megafauna can also be easily found in specialized books providing ground knowledge on the natural history of these organisms (Schreiber and Burger, 2002; Spotila, 2004; Jefferson et al., 2015).
The traits shared by marine megafauna are mainly related to ecosystem functions including nutrient storage, trophic-dynamic regulations of populations, community shaping, and habitat provision (Table 1). Also, almost all traits can be linked to nutrient storage and cycling (Table 1). Although we made a number of suggestions on how to link traits with ecosystem functions and services, establishing relationships between these properties broadly depends on the perspective of a given study. For example, food intake rate and dietary preference are intrinsically related to trophic-dynamic regulations of populations of specific taxa (Levin et al., 2001; Myers et al., 2007; Costa et al., 2017), but these traits can be also related to nutrient storage, because nutrients are transferred from the consumed to the consumer (Roman et al., 2014). Organismal dispersal performance and sociability are associated with soil fertilization via excretion (Zwolicki et al., 2013; Doughty et al., 2016), and can be related to nutrient storage by changes in primary production (Table 1). Especially body size and body mass correlate with a large number of traits and can serve as a master trait to investigate the drivers of various ecosystem functions and services (Figures 1A,B). Food intake rate, for example, varies according to body size and is related to trophic-dynamic regulations of populations and community structure since it regulates the abundance of specific prey taxa (Thomsen and Green, 2016; Figure 1B). Food intake rate can be linked to nutrient storage and cycling because it reflects nutrient flows via trophic interactions. Also, the almost ubiquitous association of nutrient storage with different traits and functions results from fundamental interactions among and between organisms and their environment, which in turn influences food chain length, trophic biomass, and nutrient cycling at a planetary scale (Loreau, 1995; Leroux et al., 2012).
Figure 1. Key traits shared by marine megafauna and their relationships with ecosystem functions and services. Body length and body mass are correlated with each other and vary over several groups (A). Body size can be considered as a master trait as it influences other physiological, behavioral, and demographic characteristics such as dietary preference, dispersal performance, metabolic rate, and mortality rate. These traits are associated to specific ecosystem functions and services (B). Trophic regulation refers to trophic-dynamic regulation of populations. Community shaping refers to shifts in community composition driven by ecosystem engineering and/or species interactions (e.g., predation). The nomenclature of ecosystem functions and services follows Costanza et al. (1997).
Nutrient storage, which is closely related to the contribution of marine megafauna to nutrient cycling, is also fundamentally related to food production, the latter being an essential ecosystem service for human well-being (Pauly and Christensen, 1995; Costanza et al., 1997). Nutrient storage, converted to food source for humans by means of fishing or aquaculture production, had intensified over past half-century leading to overexploitation of various populations of marine megafauna (Pauly et al., 2002; Springer et al., 2003). Body size, in combination with population abundances is highly associated to food production because fishing methods typically select for larger piscivorous organisms (e.g., haddocks, bonitos, rays, billfishes, tunas, sharks, and marine mammals), which have higher nutritional and economic value (Pauly and Christensen, 1995; Clements et al., 2017). However, as larger fishes get scarcer, fishing targets shift to smaller planktivorous fishes and invertebrates (Pauly, 1998; Pauly et al., 2002). This shift has been altering the structure of marine food webs, and posing a risk not only to global biodiversity, but also to food production (Springer et al., 2003; Worm et al., 2006; Butchart et al., 2010).
Large fishes, sea turtles, marine mammals, and seabirds are particularly important for the biogeochemical cycle of major elements because they are widespread, display high mobility, and are abundant worldwide (Speakman, 2005; Wing et al., 2014). Body size, is one of the most crucial traits related to nutrient transport because bigger animals hold more nutrients and feature impressive dispersal capacities (Andersen et al., 2016; Doughty et al., 2016; See Figure 1). For example, a 15 m long humpback whale Megaptera novaeangliae weights ca. 35,000 kg (Lockyer, 1976), which is thousands times heavier than seabirds such as the Manx shearwater Puffinus puffinus, which has an average size of 0.34 m and a weight 0.46 kg (Schreiber and Burger, 2002). However, the global population of shearwaters (790,000 individuals) is 13 times larger than that of humpback whales (about 60,000 individuals), thus making the contribution of seabirds to nutrient cycling also quite important. Combining body size and population abundances is thus crucial for understanding the contribution of different marine megafauna communities to nutrient storage and cycling. This is particularly relevant because the capacity of marine megafauna to transfer nutrients across land and ocean decreased by 6% of its global natural capacity due to population declines (Doughty et al., 2016). Global seabird populations, for example, have declined by almost 70% over the last 50 years (Paleczny et al., 2015), with unknown impacts on nutrient storage and transport worldwide.
Information on population abundances is often valuable to trait-based approaches since the number of organisms can affect the variability and dominance of traits within biological communities. For example, whaling activities during the first half of the nineteen century have reduced the population of blue whales Balaenoptera musculus in the southern hemisphere to about 1% (Christensen, 2006), with only a slow recovery being forecasted in the next decades (Tulloch et al., 2017). The dramatic population decline of these large whales provoked an excess in krill availability, which not only reshaped the krill community but also increased the populations of other smaller vertebrates, such as mink whales B. acutorostrata, penguins, and various pinnipeds (Estes, 2009; Roman et al., 2014). The current population of Caribbean green sea turtles is 3–7% the size of the population prior to the exploitation period, i.e., by about 1800, when turtles and their eggs were intensively and persistently harvested (Jackson, 1997, 2001). The decrease in the number of sea turtles triggered an expansion of their main food source, the seagrass Thalassia testudinum, which, in turn, caused an increase in the density of epibiont. These changes facilitated the deposition of the substrate and the colonization for diverse species of invertebrates and fishes (Bjorndal and Jackson, 2003). Compared to sharks, dolphins and whales, seabirds are relatively small, but with larger population sizes they can shape biological communities by transferring matter between distant areas via nutrient uptake, excretion, and carcass deposition (Polis and Hurd, 1996). Soils hosting large colonies of seabirds, such as blue-footed boobies Sula nebouxii show very high concentrations of ammonium and phosphate, which favors the establishment of creeping vegetation and the associated invertebrates (Havik et al., 2014; Lynam et al., 2017; Graham et al., 2018). However, the impact of seabird population declines on biological communities associated to breeding colonies is still limited. To investigate the contribution of megafauna to community structure, population abundance can be used in combination with other traits related to nutrient storage and nutrient cycling, such as excretion rate, reproductive location, and level of sociability.
Marine megafauna includes large, widespread and easily observable organisms displaying a set of traits appreciated by humans. These organisms are thus among the most charismatic in the world (Albert et al., 2018). Charismatic potential can be considered as trait contingent on human (or societal) perception with a strong association to recreational and cultural ecosystem services (Costanza et al., 1997). Such trait leads to services that provide economical (tourism) or cultural (aesthetic) benefits. The economical revenues derived by such services can, in turn, help to mitigate the negative impacts of biodiversity loss (Costanza et al., 1997; Whelan et al., 2015; Table 1). For example, whale watching generates an income of 10 billion US $ per year worldwide, and national parks in the United States and Germany earn, respectively 10 billion US $ and 500 million € per year (Mayer et al., 2010; Daniel et al., 2012). These activities provide a wealth and an environmental awareness that can indirectly influence other ecosystem functions and services.
Trait-based approaches can help us to understand how the structure of marine megafauna communities re-organize under environmental change and adverse conditions, including habitat loss, increasing pollution, and disease spreading (Keesing et al., 2010; Cardinale et al., 2012; Pimiento et al., 2017). A relatively unexplored avenue of research in the ecology of marine megafauna is the use of trait-based approaches to elucidate the importance of functional diversity, an essential component of overall biodiversity (Tilman, 2001). This field of research can provide valuable insights into the mechanisms shaping the patterns of megafaunal trait diversity and ecosystem function relationships.
One of the most significant quests in ecology today is to understand the impacts of plastic pollution on populations of marine megafauna. Evidence is accumulating about the ingestion of plastic by fishes, sea turtles, seabirds, and marine mammals (Schuyler et al., 2016; Lynam et al., 2017; Tavares et al., 2017). Plastics are also abundant in nests of some seabirds, such as the brown boobies S. leucogaster, with unknown consequences to chickens and the quality of breeding habitats (Grant et al., 2018; Battisti et al., 2019). Future research should concentrate efforts on investigating which type of traits are most closely related to plastic accumulation among marine vertebrates. Feeding strategy and depth, for example, may play an important role in relation to plastic ingestion (Tavares et al., 2017).
Information on the frequency and number of individuals stranded among sea turtles, pinnipeds, cetaceans, and seabirds has been used recently to quantify mortality patterns (Moura et al., 2016; Tavares et al., 2016; Flint et al., 2017). Carcasses found washed onshore can be collected easily and with low costs, and constitute a convenient source of information for assessing the distribution and variability of morphological, physiological, and demographical traits in relation to local environmental stressors (Spitz et al., 2006; Thompson et al., 2013; Amano et al., 2014; Fruet et al., 2015). This approach is particularly useful for species that are rare and difficult to observe, such as melon-headed whales Peponocephala electra (Amano et al., 2014). Examining stranded carcasses is also a non-invasive way of measuring traits in species with critical conservation status and in declining populations, such as the white-chinned petrel Procellaria aequinoctialis (Cipro et al., 2013).
Measuring traits of marine megafauna can be a challenging task. In contrast to plants, coral reefs, plankton, and invertebrates, marine megafauna is highly mobile and hard to capture. For example, some rare and cryptic marine mammals, such as the dwarf sperm whale Kogia sima and the franciscana dolphin Pontoporia blainvillei are difficult to detect in the field (Moura et al., 2009; Hodge et al., 2018). For these types of organisms, it is challenging, if not impossible, to measure traits such as food intake rate and metabolic rate. Hence, body size and body mass are particularly valuable as they can be precisely quantified and correlated with other traits at different levels of organization, from individuals to communities.
DT, EA-T, JdM, and AM conceived the study. DT, JdM, and EA-T revised the literature. DT built the table of traits. DT wrote the manuscript. AM, EA-T, and JdM assisted with writing and discussed the contents.
This study was funded by the Deutscher Akademischer Austauschdienst – DAAD grants (57384894/08) and Alexander von Humboldt Foundation/CAPES (Project number 88881.162169/2017-01). EA-T was funded by the German Research Foundation (DFG) through the grant AC 331/1-1, as part of the Priority Program 1704 (Dynatrait).
The authors declare that the research was conducted in the absence of any commercial or financial relationships that could be construed as a potential conflict of interest.
We are grateful to the two reviewers for providing us with relevant comments on the manuscript.
Abadi, F., Barbraud, C., and Gimene, O. (2017). Integrated population modeling reveals the impact of climate on the survival of juvenile emperor penguins. Glob. Chang. Biol. 23, 1353–1359. doi: 10.1111/gcb.13538
Acevedo-Trejos, E., Marañón, E., and Merico, A. (2018). Phytoplankton size diversity and ecosystem function relationships across oceanic regions. Proc. R. Soc. B 285:20180621. doi: 10.1098/rspb.2018.0621
Albert, C., Luque, G. M., and Courchamp, F. (2018). The twenty most charismatic species. PLoS One 13:e0199149. doi: 10.1371/journal.pone.0199149
Albert, C. H., Thuiller, W., Yoccoz, N. G., Douzet, R., Aubert, S., and Lavorel, S. (2010). A multi-trait approach reveals the structure and the relative importance of intra- vs. interspecific variability in plant traits. Funct. Ecol. 24, 1192–1201. doi: 10.1111/j.1365-2435.2010.01727.x
Albouy, C., Delattre, V. L., Mérigot, B., Meynard, C. N., and Leprieur, F. (2017). Multifaceted biodiversity hotspots of marine mammals for conservation priorities. Divers. Distrib. 23, 615–626. doi: 10.1111/ddi.12556
Amano, M., Yamada, T. K., Kuramochi, T., Hayano, A., Kazumi, A., and Sakai, T. (2014). Life history and group composition of melon-headed whales based on mass strandings in Japan. Mar. Mammal Sci. 30, 480–493. doi: 10.1111/mms.12050
Andersen, K. H., Berge, T., Gonçalves, R. J., Hartvig, M., Heuschele, J., Hylander, S., et al. (2016). Characteristic sizes of life in the oceans, from bacteria to whales. Ann. Rev. Mar. Sci. 8, 217–241. doi: 10.1146/annurev-marine-122414-034144
Baird, R. W. (2009). “Risso’s Dolphin: Grampus griseus,” in Encyclopedia of Marine Mammals, 2nd Edn, eds W. F. Perrin, B. Würsig, and J. G. M. Thewissen (London: Academic Press), 975–976.
Battisti, C., Staffieri, E., Poeta, G., Sorace, A., Luiselli, L., and Amori, G. (2019). Interactions between anthropogenic litter and birds: a global review with a ‘black-list’ of species. Mar. Pollut. Bull. 138, 93–114. doi: 10.1016/j.marpolbul.2018.11.017
Beauchard, O., Veríssimo, H., Queirós, A. M., and Herman, P. M. J. (2017). The use of multiple biological traits in marine community ecology and its potential in ecological indicator development. Ecol. Indic. 76, 81–96. doi: 10.1016/j.ecolind.2017.01.011
Bjorndal, K. A., and Jackson, J. B. C. (2003). “Roles of sea turtles in marine ecosystems: reconstructing the past,” in The Biology of Sea Turtles, eds P. L. Lutz and J. A. Musick (Florida: CRC Press), 259–273.
Blanchard, J. L., Heneghan, R. F., Everett, J. D., Trebilco, R., and Richardson, A. J. (2017). From bacteria to whales: using functional size spectra to model marine ecosystems. Trends Ecol. Evol. 32, 174–186. doi: 10.1016/j.tree.2016.12.003
Brown, J. H., Gillooly, J. F., Allen, A. P., Savage, V. M., and West, G. B. (2004). Toward a metabolic theory of ecology. Ecology 85, 1771–1789.
Butchart, S. H. M., Walpole, M., Collen, B., van Strien, A., Scharlemann, J. P. W., Almond, R. E. A., et al. (2010). Global biodiversity: indicators of recent declines. Science 1164:1187512. doi: 10.1126/science.1187512
Butler, P. J., Milsom, W. K., and Woakes, A. J. (1984). Respiratory, cardiovascular and metabolic adjustments during steady state swimming in the green turtle. Chelonia mydas. J. Comp. Physiol. B 154, 167–174. doi: 10.1007/BF00684141
Carbone, C., Codron, D., Scofield, C., Clauss, M., and Bielby, J. (2014). Geometric factors influencing the diet of vertebrate predators in marine and terrestrial environments. Ecol. Lett. 17, 1553–1559. doi: 10.1111/ele.12375
Cardinale, B. J., Duffy, J. E., Gonzalez, A., Hooper, D. U., Perrings, C., Venail, P., et al. (2012). Biodiversity loss and its impact on humanity. Nature 486, 59–67. doi: 10.1038/nature11148
Cavallo, C., Dempster, T., Kearney, M. R., Kelly, E., Booth, D., Hadden, K. M., et al. (2015). Predicting climate warming effects on green turtle hatchling viability and dispersal performance. Funct. Ecol. 29, 768–778. doi: 10.1111/1365-2435.12389
Ceia, F. R., and Ramos, J. A. (2015). Individual specialization in the foraging and feeding strategies of seabirds: a review. Mar. Biol. 162, 1923–1938. doi: 10.1007/s00227-015-2735-4
Christensen, L. B. (2006). Marine Mammal Populations: Reconstructing Historical Abundances at the Global Scale. Vancouver: University of British Columbia.
Ciancio, J. E., Quintana, F., Sala, J. E., and Wilson, R. P. (2016). Cold birds under pressure: can thermal substitution ease heat loss in diving penguins? Mar. Biol. 163:43. doi: 10.1007/s00227-016-2813-2
Cipro, C. V. Z., Colabuono, F. I., Taniguchi, S., and Montone, R. C. (2013). Polybrominated diphenyl ethers in fat samples from white-chinned petrels (Procellaria aequinoctialis) and spectacled petrels (P. conspicillata) off southern Brazil. Mar. Pollut. Bull. 77, 396–399. doi: 10.1016/j.marpolbul.2013.09.042
Clements, C. F., Blanchard, J. L., Nash, K. L., Hindell, M. A., and Ozgul, A. (2017). Body size shifts and early warning signals precede the historic collapse of whale stocks. Nat. Ecol. Amp. Evol. 1:188. doi: 10.1038/s41559-017-0188
Copello, S., Blanco, G. S., Pon, J. P. S., Quintana, F., and Favero, M. (2016). Exporting the problem: issues with fishing closures in seabird conservation. Mar. Policy 74, 120–127.
Costa, L. L., Tavares, D. C., Suciu, M. C., Rangel, D. F., and Zalmon, I. R. (2017). Human-induced changes in the trophic functioning of sandy beaches. Ecol. Indic. 82, 304–315. doi: 10.1016/j.ecolind.2017.07.016
Costanza, R., d’Arge, R., de Groot, R., Farber, S., Grasso, M., Hannon, B., et al. (1997). The value of the world’s ecosystem services and natural capital. Nature 387, 253–260. doi: 10.1038/387253a0
Costello, M. J., Claus, S., Dekeyzer, S., Vandepitte, L., Tuama,ÉÓ, Lear, D., et al. (2015). Biological and ecological traits of marine species. PeerJ 3:e1201. doi: 10.7717/peerj.1201
Crowe, L. M., O’Brien, O., Curtis, T. H., Leiter, S. M., Kenney, R. D., Duley, P., et al. (2018). Characterization of large basking shark Cetorhinus maximus aggregations in the western North Atlantic Ocean. J. Fish Biol. 92, 1371–1384. doi: 10.1111/jfb.13592
Croxall, J. P., Butchart, S. H. M., Laslecelles, B., Stattersfield, A. J., Sullivan, B., Symes, A., et al. (2012). Seabird conservation status, threats and priority actions: a global assessment. Bird Conserv. Int. 22, 1–34. doi: 10.1017/S0959270912000020
Daniel, T. C., Muhar, A., Arnberger, A., Aznar, O., Boyd, J. W., Chan, K. M. A., et al. (2012). Contributions of cultural services to the ecosystem services agenda. Proc. Natl. Acad. Sci. 109, 8812–8819. doi: 10.1073/pnas.1114773109
de Groot, R. S., Wilson, M. A., and Boumans, R. M. J. (2002). A typology for the classification, description and valuation of ecosystem functions, goods and services. Ecol. Econ. 41, 393–408.
Degen, R., Aune, M., Bluhm, B. A., Cassidy, C., Kêdra, M., Kraan, C., et al. (2018). Trait-based approaches in rapidly changing ecosystems: a roadmap to the future polar oceans. Ecol. Indic. 91, 722–736. doi: 10.1016/j.ecolind.2018.04.050
DeLaplante, K., and Picasso, V. (2011). “The biodiversity - ecoystem function debate in ecology,” in Handbook of the Phylosophy of Science. Phylosophy of Ecology, eds K. DeLaplante, B. Brown, and K. A. Peacock (Amsterdam: Elsevier), 169–200.
Des Roches, S., Post, D. M., Turley, N. E., Bailey, J. K., Hendry, A. P., Kinnison, M. T., et al. (2017). The ecological importance of intraspecific variation. Nat. Ecol. Evol. 2, 57–64. doi: 10.1038/s41559-017-0402-5
Díaz, S., and Cabido, M. (2001). Vive la différence: plant functional diversity matters to ecosystem processes. Trends Ecol. Evol. 16, 646–655. doi: 10.1016/S0169-5347(01)02283-2
Doughty, C. E., Roman, J., Faurby, S., Wolf, A., Haque, A., Bakker, E. S., et al. (2016). Global nutrient transport in a world of giants. Proc. Natl. Acad. Sci. U.S.A. 113, 868–873. doi: 10.1073/pnas.1502549112
Douglas, H. D., Co, J. E., Jones, T. H., and Conner, W. E. (2004). Interspecific differences in Aethia Spp. auklet odorants and evidence for chemical defense against ectoparasites. J. Chem. Ecol. 30, 1921–1935. doi: 10.1023/B:JOEC.0000045586.59468.de
Durrett, M. S., Wardle, D. A., Mulder, C. P. H., and Barry, R. P. (2014). Seabirds as agents of spatial heterogeneity on New Zealand’s offshore islands. Plant Soil 383, 139–153. doi: 10.1007/s11104-014-2172-z
Estes, J. A. (2009). “Ecological effects of marine mammals,” in Encyclopedia of Marine Mammals, eds W. F. Perrin and B. Wursig (California: Academic Press).
Fay, R., Barbraud, C., Delord, K., and Weimerskir, H. (2018). From early life to senescence: individual heterogeneity in a long-lived seabird. Ecol. Monogr. 88, 60–73. doi: 10.1002/ecm.1275/full
Fay, R., Barbraud, C., Delord, K., and Weimerskirch, H. (2017). Contrasting effects of climate and population density over time and life stages in a long-lived seabird. Funct. Ecol. 31, 1275–1284. doi: 10.1111/1365-2435.12831
Flint, J., Flint, M., Limpus, C. J., and Mills, P. C. (2017). The impact of environmental factors on marine turtle stranding rates. PLoS One 12:e0182548. doi: 10.1371/journal.pone.0182548
Foote, A. D. (2008). Mortality rate acceleration and post-reproductive lifespan in matrilineal whale species. Biol. Lett. 4, 189–191. doi: 10.1098/rsbl.2008.0006
Fossette, S., Schofield, G., Lielley, M. K. S., Gleiss, A. C., and Hays, G. C. (2012). Acceleration data reveal the enery management strategy of a marine ectotherm during reproduction. Funct. Ecol. 26, 324–333. doi: 10.1111/j.1365-2435.2011.01960.x
Fraija-Fernández, N., Fernández, M., Raga, J. A., and Aznar, F. J. (2015). Life-history trade-offs in a generalist digenean from cetaceans: the role of host specificity and environmental factors. Parasit. Vectors 8, 1–13. doi: 10.1186/s13071-015-1273-8
Friesen, M. R., Beggs, J. R., and Gaskett, A. C. (2017). Sensory-based conservation of seabirds: a review of management strategies and animal behaviours that facilitate success. Biol. Rev. 1777, 1769–1784. doi: 10.1111/brv.12308
Froy, H., and Richard, A. (2013). Age-related variation in reproductive traits in the wandering albatross: evidence for terminal improvement following senescence. Ecol. Lett. 16, 642–649. doi: 10.1111/ele.12092
Fruet, P. F., Genoves, R. C., Möller, L. M., Botta, S., and Secchi, E. R. (2015). Using mark-recapture and stranding data to estimate reproductive traits in female bottlenose dolphins (Tursiops truncatus) of the Southwestern Atlantic Ocean. Mar. Biol. 162, 661–673. doi: 10.1007/s00227-015-2613-0
Genovart, M., Sanz-Aguilar, A., Fernández-Chacón, A., Igual, J. M., Pradel, R., Forero, M. G., et al. (2013). Contrasting effects of climatic variability on the demography of a trans-equatorial migratory seabird. J. Anim. Ecol. 82, 121–130. doi: 10.1111/j.1365-2656.2012.02015.x
Gleiss, A. C., Norman, B., and Wilson, R. P. (2011). Moved by that sinking feeling: variable diving geometry underlies movement strategies in whale sharks. Funct. Ecol. 25, 595–607. doi: 10.1111/j.1365-2435.2010.01801.x
Graham, N. A. J., Wilson, S. K., Carr, P., Hoey, S., Jennings, S., and MacNeil, M. A. (2018). Seabirds enhance coral reef productivity and functioning in the absence of invasive rats. Nature 559, 250–253. doi: 10.1038/s41586-018-0202-3
Grant, M. L., Lavers, J. L., Stuckenbrock, S., Sharp, P. B., and Bond, A. L. (2018). The use of anthropogenic marine debris as a nesting material by brown boobies (Sula leucogaster). Mar. Pollut. Bull. 137, 96–103. doi: 10.1016/j.marpolbul.2018.10.016
Gravel, D., Albouy, C., and Thuiller, W. (2016). The meaning of functional trait composition of food webs for ecosystem functioning. Philos. Trans. R. Soc. B Biol. Sci. 371:20150268. doi: 10.1098/rstb.2015.0268
Grémillet, D., Meslin, L., and Lescroël, A. (2012). Heat dissipation limit theory and the evolution of avian functional traits in a warming world. Funct. Ecol. 26, 1001–1006. doi: 10.1111/j.1365-2435.2012.02048.x
Guinet, C., Domenici, P., De Stephanis, R., Barrett-Lennard, L., Ford, J. K. B., and Verborgh, P. (2007). Killer whale predation on bluefin tuna: exploring the hypothesis of the endurance-exhaustion technique. Mar. Ecol. Prog. Ser. 347, 111–119. doi: 10.3354/meps07035
Hassrick, J. L., Crocker, D. E., and Costa, D. P. (2013). Effects of maternal age and mass on foraging behaviour and foraging success in the northern elephant seal. Funct. Ecol. 27, 1055–1063. doi: 10.1111/1365-2435.12108
Hattam, C., Atkins, J. P., Beaumont, N., Börger, T., Böhnke-Henrichs, A., Burdon, D., et al. (2015). Marine ecosystem services: linking indicators to their classification. Ecol. Indic. 49, 61–75. doi: 10.1016/j.ecolind.2014.09.026
Havik, G., Catenazzi, A., and Holmgren, M. (2014). Seabird nutrient subsidies benefit non-nitrogen fixing trees and alter species composition in South American coastal dry forests. PLoS One 9:e86381. doi: 10.1371/journal.pone.0086381
Hilton, G. M., Furness, R. W., and Houston, D. C. (2000). A comparative study of digestion in North Atlantic seabirds. J. Avian Biol. 31, 36–46. doi: 10.1034/j.1600-048X.2000.310106.x
Hilton, G. M., Houston, D. C., and Furness, R. W. (2008). Which components of diet quality affect retention time of digesta in seabirds? Funct. Ecol. 12, 929–939. doi: 10.1046/j.1365-2435.1998.00267.x
Hodge, L. E. W., Baumann-Pickering, S., Hildebrand, J. A., Bell, J. T., Cummings, E. W., Foley, H. J., et al. (2018). Heard but not seen: occurrence of Kogia spp. along the western North Atlantic shelf break. Mar. Mammal Sci. 34, 1141–1153. doi: 10.1111/mms.12498
Houle, J. E., Castro, F., Cronin, M. A., Farnsworth, K. D., Gosch, M., and Reid, D. G. (2016). Effects of seal predation on a modelled marine fish community and consequences for a commercial fishery. J. Appl. Ecol. 53, 54–63. doi: 10.1111/1365-2664.12548
Jackson, J. B. C. (2001). What was natural in the coastal oceans? Proc. Natl. Acad. Sci. U.S.A. 98, 5411–5418. doi: 10.1073/pnas.091092898
Jefferson, T., Webber, M., and Pitman, R. (2015). Marine Mammals of the World. London: Academic Press.
Jenouvrier, S., Péron, C., and Weimerskirch, H. (2015). Extreme climate events and individual heterogeneity shape life-history traits and population dynamics. Ecol. Monogr. 85, 605–624. doi: 10.1890/14-1834.1.sm
Jobling, M. (1981). The influences of feeding on the metabolic rate of fishes: a short review. J. Fish Biol. 18, 385–400.
Jones, K. E., Bielby, J., Cardillo, M., Fritz, S. A., O’Dell, J., Orme, C. D. L., et al. (2009). PanTHERIA: a species-level database of life history, ecology, and geography of extant and recently extinct mammals. Ecology 90, 2648–2648. doi: 10.1890/08-1494.1
Jovani, R., Mavor, R., and Oro, D. (2008). Hidden patterns of colony size variation in seabirds: a logarithmic point of view. Oikos 117, 1774–1781.
Kattge, J., Díaz, S., Lavorel, S., Prentice, I. C., Leadley, P., Bönisch, G., et al. (2011). TRY - a global database of plant traits. Glob. Chang. Biol. 17, 2905–2935. doi: 10.1111/j.1365-2486.2011.02451.x
Keesing, F., Belden, L. K., Daszak, P., Dobson, A., Harvell, C. D., Holt, R. D., et al. (2010). Impacts of biodiversity on the emergence and transmission of infectious diseases. Nature 468, 647–652. doi: 10.1038/nature09575
Kraft, N. J. B., Godoy, O., and Levine, J. M. (2015). Plant functional traits and the multidimensional nature of species coexistence. Proc. Natl. Acad. Sci. U.S.A. 112, 797–802. doi: 10.1073/pnas.1413650112
Krause, S., Le Roux, X., Niklaus, P. A., van Bodegom, P. M., Lennon, J. T., Bertilsson, S., et al. (2014). Trait-based approaches for understanding microbial biodiversity and ecosystem functioning. Front. Microbiol. 5:251. doi: 10.3389/fmicb.2014.00251
Kremer, C. T., Williams, A. K., Finiguerra, M., Fong, A. A., Kellerman, A., Paver, S. F., et al. (2017). Realizing the potential of trait-based aquatic ecology: new tools and collaborative approaches. Liminol. Oceanogr. 62, 253–271.
Ladds, M. A., Sibanda, N., Arnold, R., and Dunn, M. R. (2018). Creating functional groups of marine fish from categorical traits. PeerJ 6:e5795. doi: 10.7717/peerj.5795
Leroux, S. J., Hawlena, D., and Schmitz, O. J. (2012). Predation risk, stoichiometric plasticity and ecosystem elemental cycling. Proc. R. Soc. B Biol. Sci. 279, 4183–4191. doi: 10.1098/rspb.2012.1315
Levin, L. A., Boesch, D. F., Covich, A., Dahm, C., Erséus, C., Ewel, K. C., et al. (2001). The function of marine critical transition zones and the importance of sediment biodiversity. Ecosystems 4, 430–451.
Litchman, E., and Klausmeier, C. A. (2008). Trait-based community ecology of phytoplankton. Annu. Rev. Ecol. Evol. Syst. 39, 615–639. doi: 10.1146/annurev.ecolsys.39.110707.173549
Litchman, E., Ohman, M. D., and Kiørboe, T. (2013). Trait-based approaches to zooplankton communities. J. Plankton Res. 35, 473–484. doi: 10.1093/plankt/fbt019
Lockyer, C. (1976). Body weights of some species of large whales. ICES J. Mar. Sci. 36, 259–273. doi: 10.1093/icesjms/36.3.259
Loreau, M. (1995). Consumers as maximizers of matter and energy flows in ecosystems. Am. Nat. 145, 22–42.
Louzao, M., Igual, J. M., Genovart, M., Forero, M. G., Hobson, K. A., and Oro, D. (2008). Spatial variation in egg size of a top predator: interplay of body size and environmental factors? Acta Oecol. 34, 186–193. doi: 10.1016/j.actao.2008.05.007
Lowther, A. D., and Goldsworthy, S. D. (2011). Maternal strategies of the Australian sea lion (Neophoca cinerea) at Dangerous Reef. South Australia. Aust. J. Zool. 59, 54–62. doi: 10.1071/ZO11025
Luck, G. W., Carter, A., and Smallbone, L. (2013). Changes in bird functional diversity across multiple land uses: interpretations of functional redundancy depend on functional group identity. PLoS One 8:e63671. doi: 10.1371/journal.pone.0063671
Lutcavage, M., and Lutz, P. L. (1986). Metabolic rate and food energy requirements of the leatherback sea turtle. Dermochelys coriacea. Copeia 1986, 796–798. doi: 10.2307/1444962
Lynam, C. P., Llope, M., Möllmann, C., Helaouët, P., Bayliss-Brown, G. A., and Stenseth, N. C. (2017). Interaction between top-down and bottom-up control in marine food webs. Proc. Natl. Acad. Sci. U.S.A. 114, 1952–1957. doi: 10.1073/pnas.1621037114
Mace, G. M., Norris, K., and Fitter, A. H. (2012). Biodiversity and ecosystem services: a multilayered relationship. Trends Ecol. Evol. 27, 19–25. doi: 10.1016/j.tree.2011.08.006
Madin, J. S., Hoogenboom, M. O., Connolly, S. R., Darling, E. S., Falster, D. S., Huang, D., et al. (2016). A trait-based approach to advance coral reef science. Trends Ecol. Evol. 31, 419–428. doi: 10.1016/j.tree.2016.02.012
Mann, J., Connor, R. C., Barre, L. M., and Heithaus, M. R. (2000). Female reproductive success in bottlenose dolphins (Tursiops sp.): life history, habitat, provisioning, and group-size effects. Behav. Ecol. 11, 210–219.
Mason, N. W. W., and Mouillot, D. (2013). Functional diversity measures. Encycl. Biodivers. 3, 597–608. doi: 10.1016/B978-0-12-384719-5.00356-7
Mayer, M., Müller, M., Woltering, M., Arnegger, J., and Job, H. (2010). The economic impact of tourism in six German national parks. Landsc. Urban Plan. 97, 73–82. doi: 10.1016/j.landurbplan.2010.04.013
McClain, C. R., Balk, M. A., Benfield, M. C., Branch, T. A., Chen, C., Crosgrove, J., et al. (2015). Sizing ocean giants: patterns of intraspecific size variation in marine megafauna. PeerJ 3:e715. doi: 10.7717/peerj.715
McDonald, B. I., Goebel, M. E., Crocker, D. E., and Costa, D. P. (2012). Dynamic influence of maternal and pup traits on maternal care during lactation in an income breeder, the Antarctic fur seal. Physiol. Biochem. Zool. 85, 243–254. doi: 10.1086/665407
McGill, B. J., Enquist, B. J., Weiher, E., and Westoby, M. (2006). Rebuilding community ecology from functional traits. Trends Ecol. Evol. 21, 178–185. doi: 10.1016/j.tree.2006.02.002
McNab, B. K. (1988). Complications inherent in scaling the basal rate of metabolism in mammals. Q. Rev. Biol. 63, 25–54.
Meir, J. U., Robinson, P. W., Vilchis, L. I., Kooyman, G. L., Costa, D. P., and Ponganis, P. J. (2013). Blood oxygen depletion is independent of dive function in a deep diving vertebrate, the northern elephant eeal. PLoS One 8:e83248. doi: 10.1371/journal.pone.0083248
Messier, J., McGill, B. J., and Lechowicz, M. J. (2010). How do traits vary across scales? Ecol. Lett. 13, 838–848. doi: 10.1111/j.1461-0248.2010.01476.x
Meunier, C. L., Boersma, M., El-Sabaawi, R., Halvorson, H. M., Herstoff, E. M., Van de Waal, D. B., et al. (2017). From elements to function: toward unifying ecological stoichiometry and trait-based ecology. Front. Environ. Sci. 5:18. doi: 10.3389/fenvs.2017.00018
Moura, J. F., Acevedo-Trejos, E., Tavares, D. C., Meirelles, A. C. O., Silva, C. P. N., Oliveira, L. R., et al. (2016). Stranding events of Kogia whales along the Brazilian coast. PLoS One 11:e0146108. doi: 10.1371/journal.pone.0146108
Moura, J. F., Rodrigues, E. S., Sholl, T. G. C., and Siciliano, S. (2009). Franciscana dolphin (Pontoporia blainvillei) on the north-east coast of Rio de Janeiro State, Brazil, recorded during a long term monitoring programme. Mar. Biodivers. Rec. 2:e66. doi: 10.1017/S1755267209000803
Myers, R. A., Baum, J. K., Shepherd, T. D., Powers, S. P., and Peterson, C. H. (2007). Cascading effects of the loss of apex predatory sharks from a coastal ocean. Science 315, 1846L–1850L. doi: 10.1126/science.1138657
Naeem, S., Duffy, J. E., and Zavaleta, E. (2012). The functions of biological diversity in an age of extinction. Science 336, 1401–1406. doi: 10.1126/science.1215855
Nunes, G. T., Mancini, P. L., and Bugoni, L. (2017). When Bergmann’s rule fails: evidences of environmental selection pressures shaping phenotypic diversification in a widespread seabird. Ecography 40, 365–375. doi: 10.1111/ecog.02209
Paleczny, M., Hammill, E., Karpouzi, V., and Pauly, D. (2015). Population trend of the world’s monitored seabirds, 1950-2010. PLoS One 10:e0129342. doi: 10.1371/journal.pone.0129342
Paredes, R., Orben, R. A., Roby, D. D., Irons, D. B., Young, R., Renner, H., et al. (2015). Foraging ecology during nesting influences body size in a pursuit-diving seabird. Mar. Ecol. Prog. Ser. 533, 261–276. doi: 10.3354/meps11388
Parr, C. S., Wilson, N., Leary, P., Schulz, K. S., Lans, K., Walley, L., et al. (2014). The encyclopedia of life v2: providing global access to knowledge about life on earth. Biodivers. Data J. 2:e1079. doi: 10.3897/BDJ.2.e1079
Pauly, D. (1998). Fishing down marine food webs. Science 279, 860–863. doi: 10.1126/science.279.5352.860
Pauly, D., and Christensen, V. (1995). Primary production required to sustain global fisheries. Nature 376:279.
Pauly, D., Christensen, V., Guénette, S., Pitcher, T. J., Sumaila, U. R., Walters, C. J., et al. (2002). Towards sustainability in world fisheries. Nature 418, 689–695.
Petchey, O. L., Beckerman, A. P., Riede, J. O., and Warren, P. H. (2008). Size, foraging, and food web structure. Proc. Natl. Acad. Sci.U.S.A. 105, 4191–4196. doi: 10.1073/pnas.0710672105
Pimiento, C., Griffin, J. N., Clements, C. F., Silvestro, D., Varela, S., Uhen, M. D., et al. (2017). The Pliocene marine megafauna extinction and its impact on functional diversity. Nat. Ecol. Evol. 1, 1100–1106. doi: 10.1038/s41559-017-0223-6
Plot, V., Criscuolo, F., Zahn, S., and Georges, J.-Y. (2012). Telomeres, age and reproduction in a long-lived reptile. PLoS One 7:e40855. doi: 10.1371/journal.pone.0040855
Polis, G. A., and Hurd, S. D. (1996). Linking marine and terrestrial food webs: allochthonous input from the ocean supports high secondary productivity on small islands and coastal land communities. Am. Nat. 147, 396–423. doi: 10.1086/285858
Putman, N. F., Mansfield, K. L., He, R., Shaver, D. J., and Verley, P. (2018). Predicting the distribution of oceanic-stage Kemp’s ridley sea turtles. Biol. Lett. 9:20130345. doi: 10.1098/rsbl.2013.0345
Robeck, T. R., Willis, K., Scarpuzzi, M. R., and O’Brien, J. K. (2015). Comparisons of life-history parameters between free-ranging and captive killer whale (Orcinus orca) populations for application toward species management. J. Mammal 96, 1055–1070. doi: 10.1093/jmammal/gyv113
Roman, J., Estes, J. A., Morissette, L., Smith, C., Costa, D., McCarthy, J., et al. (2014). Whales as marine ecosystem engineers. Front. Ecol. Environ. 12, 377–385. doi: 10.1890/130220
Rosenzweig, M. L. (1995). Species Diversity in Space and Time. New York, NY: Cambridge University Press.
Samarra, F. I. P., Vighi, M., Aguilar, A., and Vikingsson, G. A. (2017). Intra-population variation in isotopic niche in herring-eating killer whales off Iceland. Mar. Ecol. Prog. Ser. 564, 199–210. doi: 10.3354/meps11998
Schuyler, Q. A., Wilcox, C., Townsend, K. A., Wedemeyer-Strombel, K. R., Balazs, G., van Sebille, E., et al. (2016). Risk analysis reveals global hotspots for marine debris ingestion by sea turtles. Glob. Chang. Biol. 22, 567–576. doi: 10.1111/gcb.13078
Shoji, A., Elliott, K. H., Aris-Brosou, S., Wilson, R. P., and Gaston, A. J. (2015). Predictors of incubation costs in seabirds: an evolutionary perspective. Ibis 157, 44–53. doi: 10.1111/ibi.12219
Simeone, A., and Wilson, R. P. (2003). In-depth studies of Magellanic penguin (Spheniscus magellanicus) foraging: can we estimate prey consumption by perturbations in the dive profile? Mar. Biol. 143, 825–831.
Sims, D. W. (2000). Can threshold foraging responses of basking sharks be used to estimate their metabolic rate? Mar. Ecol. Prog. Ser. 200, 289–296.
Spitz, J., Ridoux, V., and Brind’Amour, A. (2014). Let’s go beyond taxonomy in diet description: testing a trait-based approach to prey-predator relationships. J. Anim. Ecol. 83, 1137–1148. doi: 10.1111/1365-2656.12218
Spitz, J., Rousseau, Y., and Ridoux, V. (2006). Diet overlap between harbour porpoise and bottlenose dolphin: an argument in favour of interference competition for food? Estuar. Coast. Shelf Sci. 70, 259–270. doi: 10.1016/j.ecss.2006.04.020
Spotila, J. R. (2004). Sea Turtles: A Complete Guide to Their Biology, Behavior, And Conservation. Baltimore: Johns Hopkins University Press.
Springer, A. M., Estes, J. A., van Vliet, G. B., Williams, T. M., Doak, D. F., Danner, E. M., et al. (2003). Sequential megafaunal collapse in the North Pacific Ocean: an ongoing legacy of industrial whaling? Proc. Natl. Acad. Sci. U.S.A. 100, 12223–12228.
Stuart-Smith, R. D., Bates, A. E., Lefcheck, J. S., Duffy, J. E., Baker, S. C., Thomson, R. J., et al. (2013). Integrating abundance and functional traits reveals new global hotspots of fish diversity. Nature 501, 539–542. doi: 10.1038/nature12529
Szostek, K. L., and Becker, P. H. (2015). Survival and local recruitment are driven by environmental carry-over effects from the wintering area in a migratory seabird. Oecologia 178, 643–657. doi: 10.1007/s00442-015-3298-2
Tavares, D. C., Moura, J. F., Merico, A., and Siciliano, S. (2017). Incidence of marine debris in seabirds feeding at different water depths. Mar. Pollut. Bull. 119, 68–73. doi: 10.1016/j.marpolbul.2017.04.012
Tavares, D. C., Moura, J. F., and Siciliano, S. (2016). Environmental predictors of seabird wrecks in a tropical coastal area. PLoS One 11:e0168717. doi: 10.1371/journal.pone.0168717
Teixeira, C. M. G. L., Sousa, T., Marques, G. M., Domingos, T., and Kooijman, S. A. L. M. (2014). A new perspective on the growth pattern of the wandering albatross (Diomedea exulans) through DEB theory. J. Sea Res. 94, 117–127. doi: 10.1016/j.seares.2014.06.006
Thompson, K. F., Millar, C. D., Scott Baker, C., Dalebout, M., Steel, D., van Helden, A. L., et al. (2013). A novel conservation approach provides insights into the management of rare cetaceans. Biol. Conserv. 157, 331–340. doi: 10.1016/J.BIOCON.2012.07.017
Thomsen, S. K., and Green, D. J. (2016). Cascading effects of predation risk determine how marine predators become terrestrial prey on an oceanic island. Ecology 97, 3530–3537. doi: 10.1002/ecy.1614
Tilman, D. (2001). “Functional diversity,” in Encyclopedia of Biodiversity, ed. S. Levin (New York, NY: Academic Press), 109–120.
Tilman, D., Isbell, F., and Cowles, J. M. (2014). Biodiversity and ecosystem functioning. Annu. Rev. Ecol. Evol. Syst. 45, 471–493. doi: 10.1146/annurev-ecolsys-120213-091917
Tulloch, V. J. D., Plagányi,É, Matear, R., Brown, C. J., and Richardson, A. J. (2017). Ecosystem modelling to quantify the impact of historical whaling on Southern Hemisphere baleen whales. Fish Fish. 19, 117–137. doi: 10.1111/faf.12241
Violle, C., Enquist, B. J., McGill, B. J., Jiang, L., Albert, C. H., Hulshof, C., et al. (2012). The return of the variance: intraspecific variability in community ecology. Trends Ecol. Evol. 27, 244–252. doi: 10.1016/j.tree.2011.11.014
Violle, C., Navas, M.-L., Vile, D., Kazakou, E., Fortunel, C., Hummel, I., et al. (2007). Let the concept of trait be functional! Oikos 116, 882–892. doi: 10.1111/j.2007.0030-1299.15559.x
Violle, C., Reich, P. B., Pacala, S. W., Enquist, B. J., and Kattge, J. (2014). The emergence and promise of functional biogeography. Proc. Natl. Acad. Sci. U.S.A. 111, 13690–13696. doi: 10.1073/pnas.1415442111
West, G. B., Woodruff, W. H., and Brown, J. H. (2002). Allometric scaling of metabolic rate from molecules and mitochondria to cells and mammals. Proc. Natl. Acad. Sci. U.S.A. 99, 2473–2478. doi: 10.1073/pnas.012579799
Whelan, C. J., Şekercioğlu, ÇH., and Wenny, D. G. (2015). Why birds matter: from economic ornithology to ecosystem services. J. Ornithol. 156, 227–238. doi: 10.1007/s10336-015-1229-y
Williams, T. M., Haun, J., Davis, R. W., Fuiman, L. A., and Kohin, S. (2001). A killer appetite: metabolic consequences of carnivory in marine mammals. Comp. Biochem. Physiol. 129, 785–796. doi: 10.1016/S1095-6433(01)00347-6
Wilman, H., Belmaker, J., Simpson, J., de la Rosa, C., Rivadeneira, M. M., and Jetz, W. (2014). EltonTraits 1.0: species-level foraging attributes of the world’s birds and mammals. Ecology 95:2027. doi: 10.1890/13-1917.1
Wing, S. R., Jack, L., Shatova, O., Leichter, J. J., Barr, D., Frew, R. D., et al. (2014). Seabirds and marine mammals redistribute bioavailable iron in the Southern Ocean. Mar. Ecol. Prog. Ser. 510, 1–13. doi: 10.3354/meps10923
Woodward, G., Ebenman, B., Emmerson, M., Montoya, J. M., Olesen, J. M., Valido, A., et al. (2005). Body size in ecological networks. Trends Ecol. Evol. 20, 402–409. doi: 10.1016/j.tree.2005.04.005
Worm, B., Barbier, E. B., Beaumont, N., Duffy, J. E., Folke, C., Halpern, B. S., et al. (2006). Impacts of biodiversity loss on Ocean Ecosystem Services. Science 314, 787–790. doi: 10.1126/science.1132294
Yamamoto, T., Kohno, H., Mizutani, A., Yoda, K., Matsumoto, S., Kawabe, R., et al. (2016). Geographical variation in body size of a pelagic seabird, the streaked shearwater Calonectris leucomelas. J. Biogeogr. 43, 801–808. doi: 10.1111/jbi.12654
Young, J. W., Lansdell, M. J., Campbell, R. A., Cooper, S. P., Juanes, F., and Guest, M. A. (2010). Feeding ecology and niche segregation in oceanic top predators off eastern Australia. Mar. Biol. 157, 2347–2368. doi: 10.1007/s00227-010-1500-y
Keywords: body size, cetacean, dietary preference, fish, seabird, sea turtle
Citation: Tavares DC, Moura JF, Acevedo-Trejos E and Merico A (2019) Traits Shared by Marine Megafauna and Their Relationships With Ecosystem Functions and Services. Front. Mar. Sci. 6:262. doi: 10.3389/fmars.2019.00262
Received: 30 June 2018; Accepted: 02 May 2019;
Published: 24 May 2019.
Edited by:
Mark Meekan, Australian Institute of Marine Science (AIMS), AustraliaReviewed by:
Jerome Spitz, Université de la Rochelle, FranceCopyright © 2019 Tavares, Moura, Acevedo-Trejos and Merico. This is an open-access article distributed under the terms of the Creative Commons Attribution License (CC BY). The use, distribution or reproduction in other forums is permitted, provided the original author(s) and the copyright owner(s) are credited and that the original publication in this journal is cited, in accordance with accepted academic practice. No use, distribution or reproduction is permitted which does not comply with these terms.
*Correspondence: Davi Castro Tavares, ZGF2aS50YXZhcmVzQGxlaWJuaXotem10LmRl; d2V0bGFuZGJpcmRzYnJhemlsQGdtYWlsLmNvbQ==
Disclaimer: All claims expressed in this article are solely those of the authors and do not necessarily represent those of their affiliated organizations, or those of the publisher, the editors and the reviewers. Any product that may be evaluated in this article or claim that may be made by its manufacturer is not guaranteed or endorsed by the publisher.
Research integrity at Frontiers
Learn more about the work of our research integrity team to safeguard the quality of each article we publish.