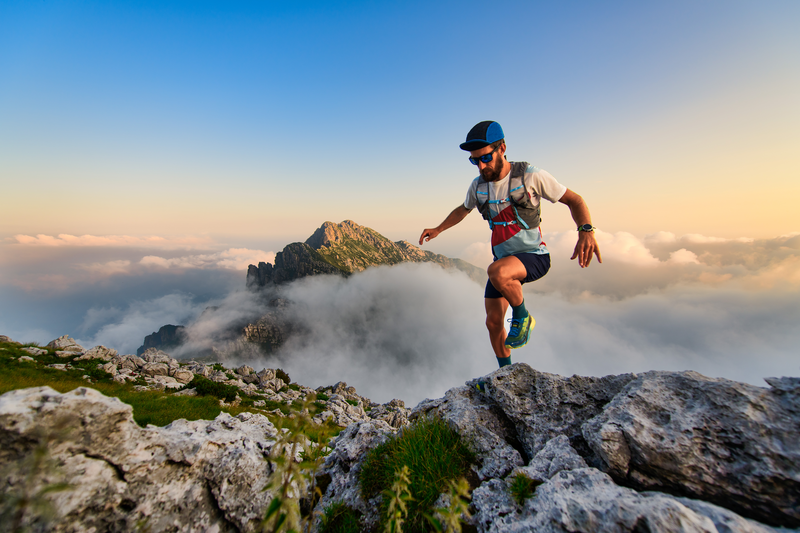
95% of researchers rate our articles as excellent or good
Learn more about the work of our research integrity team to safeguard the quality of each article we publish.
Find out more
ORIGINAL RESEARCH article
Front. Mar. Sci. , 21 May 2019
Sec. Coral Reef Research
Volume 6 - 2019 | https://doi.org/10.3389/fmars.2019.00258
Invasive lionfish (Pterois volitans and P. miles) are now ubiquitous throughout the Caribbean and Western Atlantic on shallow and deep reefs. Recent surveys in Bermuda have revealed dense aggregations of lionfish on mesophotic reefs (60 m depth), yet these densities are not pervasive across reefs at this depth. Using diver-led visual surveys of mesophotic reef sites, this study examines how variations in potential ecological drivers may affect lionfish distribution. Significant correlations of lionfish densities were found with prey fish density and prey fish biomass, where sites with higher abundances of prey fishes have greater densities of lionfish. Furthermore, higher densities of lionfish also correlated significantly with higher juvenile Paranthias furcifer biomass, a preferred prey type for lionfish. Prey fish diversity, on the other hand, was not related to lionfish density, nor did prey fish community composition differ in a way that reflected lionfish distributions. The influence of seawater temperature was found to have the strongest effect on lionfish distribution, where higher lionfish densities were found at sites with lower bottom temperature. However, temperature co-varied with prey fish density, prey fish biomass, and P. furcifer biomass, implying that physical parameters of the environment (i.e., temperature) likely influence ecological parameters (i.e., prey fish abundance), contributing to the structuring of lionfish distributions. We suggest, therefore, that cold-water upwelling currents may be fueling the food chain in certain locations, resulting in high abundances of prey fishes and thus lionfish. Understanding the factors that influence lionfish distributions will ultimately increase the efficacy of management strategies, which, as the data presented here suggest, must incorporate mesophotic lionfish populations.
Pterois miles and P. volitans, collectively known as lionfish, are predatory fish belonging to the family Scorpaenidae, native to the Indian and Pacific oceans. The first non-native lionfish were sighted near the southeast coast of North America in 1985 (Semmens et al., 2004). Genetic evidence indicates that this popular aquarium fish was likely introduced via multiple aquarium releases off the southeast coast of Florida (Betancur et al., 2011). From there, the population spread northward along the east coast of the United States and eastward toward Bermuda, before progressing southward throughout the Caribbean, Gulf of Mexico, and western Atlantic, with lionfish reported from as far north as Rhode Island and as far south as Brazil (Schofield, 2009, 2010; Johnston and Purkis, 2011; Frazer et al., 2012; Fogg et al., 2013; Ferreira et al., 2015). A high reproductive rate coupled with the lack of native predators has allowed lionfish populations in the western Atlantic to flourish, reaching densities exceeding those found in their native habitats by a factor of 13 to 15 (Darling et al., 2011; Kulbicki et al., 2012). These high densities of lionfish indicate that they have become invasive and could potentially affect the biodiversity and structure of reef fish communities, and cause significant ecosystem change (Lesser and Slattery, 2011; Albins, 2013).
Lionfish are generalist predators with a broad diet, consuming large quantities of small and juvenile fishes, along with small invertebrates (Morris and Akins, 2009; Muñoz et al., 2011; Layman and Allgeir, 2012; Valdez-Moreno et al., 2012; Dahl and Patterson, 2014; Eddy et al., 2016; Peake et al., 2018). The relative increase of lionfish from 23 to ∼40% of total predator biomass, coincident with a 65% decline in prey fish biomass between 2008 and 2010 in the Bahamas, indicates that this opportunistic generalist feeding strategy has the potential to alter reef fish communities (Green et al., 2012). While lionfish tend to be non-selective in their prey choice (Morris and Akins, 2009), contributions of various species to lionfish diets vary by location, depth, and season, indicating that lionfish may be consuming certain prey types preferentially based on their availability or ease of capture (Dahl and Patterson, 2014; Green and Côté, 2014; Eddy et al., 2016; Peake et al., 2018). It is unclear, however, if the distribution and abundance of prey types may influence lionfish abundance and distribution.
The first lionfish recorded in Bermuda was collected in 2000, yet despite being one of the first locations outside the United States to report the presence of lionfish (Smith et al., 2013), surveys indicate that the abundance of lionfish in shallow reef zones has remained relatively low (Eddy, 2016). However, lionfish populations extend well beyond shallow reefs across the invaded range, with sightings reported down to 55 m in Puerto Rico (Bejarano et al., 2014), over 100 m in The Bahamas (Lesser and Slattery, 2011), 112 m in the northwestern Gulf of Mexico (Nuttall et al., 2014), and 250 m in Honduras (Gress et al., 2017). In Bermuda, lionfish were recently sighted during submersible surveys as deep as 304 m (Gress et al., 2017). Early technical dive expeditions in Bermuda stumbled upon dense aggregations of lionfish at a few isolated deep (60 m) sites beginning in 2009. These select sites were revisited on multiple occasions over several years and consistently yielded high catches of lionfish (pers. comm. G. Maddocks1) leading to the presumption that they represented “hotspots” of lionfish abundance. Recent surveys of mesophotic coral ecosystems (MCEs) confirmed this assumption, reporting dense populations of lionfish on specific sites at or below 60 m in select locations around the Bermuda platform (Eddy, 2016; Andradi-Brown et al., 2017a). The average density of lionfish at these sites is roughly 250 fish ha–1 (Eddy, 2016), which is similar to the densities reported at heavily invaded shallow locations in the Bahamas (Green et al., 2012). Such densities are not pervasive across this depth in Bermuda however, with observations ranging from 0 fish ha–1 at some sites to 1100 fish ha–1 at others (Eddy, 2016; Figure 1). Thus, variations in the physical environment, such as temperature (Whitfield et al., 2014) or habitat complexity (Garcia-Sais et al., 2012; Bejarano et al., 2015; Hunt et al., 2019), or in the biological community, such as availability of potential prey fish (Dahl and Patterson, 2014; Harter et al., 2017), may affect lionfish densities, resulting in higher densities of lionfish at certain locations.
Figure 1. Image of resident lionfish population at the XL site, showing benthic habitat as low relief hard bottom interspersed amongst rhodolith beds.
A suite of variables is known to affect reef fish community structure across spatiotemporal scales, including fishing pressure, larval dispersal, habitat quality, connectivity, reef structural complexity, depth, and ecological interactions, among others (see Sale, 2002), yet the factors controlling lionfish distributions remain unclear (Benkwitt et al., 2017). The presence of biotic agents such as competitors, predators, or parasites, is hypothesized to regulate the establishment of invasive populations, such as lionfish (Keane and Crawley, 2002; Hackerott et al., 2013; Tuttle et al., 2017). However, multiple studies have found no relationship between lionfish density and native predator abundance (Anton et al., 2014; Hackerott et al., 2013; Valdivia et al., 2014), and parasites were found to have only a limited direct effect on lionfish populations (Sellers et al., 2015), suggesting instead that reduced susceptibility to parasites in the invaded range may further contribute to lionfish success (Tuttle et al., 2017). Likewise, habitat complexity has been shown to positively correlate with lionfish densities in a few studies (Garcia-Sais et al., 2012; Bejarano et al., 2015; Hunt et al., 2019), but not in others (Anton et al., 2014; Hackerott et al., 2017), and thus is not a predictable indicator of lionfish distributions over large spatial scales.
The aim of this study is to identify possible ecological drivers of lionfish distribution across MCEs in Bermuda, where lionfish have been present for nearly two decades. The long-term presence of lionfish in Bermuda may, in fact, facilitate the emergence of detectable patterns of distribution through adaption to local conditions and prey communities. Using a series of visual censuses at mesophotic sites (60 m depth) across the Bermuda reef platform, we assess how variations in teleost (reef fish) communities and physical aspects of the environment may be influencing lionfish distribution. Understanding why high densities occur in specific locations will aid in our ability to identify and target areas for lionfish control efforts, thereby increasing the efficacy of future management.
Located at 32°N, 64°W, 1049 km (652 nm) southeast of Cape Hatteras, United States central east coast, Bermuda’s sub-tropical coral reefs represent the northernmost coral reef system in the Atlantic (Coates et al., 2013). Reefs persist in Bermuda partly due to the flow of the Gulf Stream, bringing warm water from the Gulf of Mexico (Coates et al., 2013; Locke et al., 2013b). Annual sea surface temperatures range from 15 to 30°C, which allows a variety of tropical marine organisms to live in this region, including 38 scleractinian coral species (Locke et al., 2013a).
The top of the seamount upon which Bermuda lies is a circular pseudo-atoll (∼900 km2) with a total reef area of 177 km2 (Coates et al., 2013). It consists of a large lagoon with thousands of patch reefs, bounded along the northern edge by a shallow annular rim reef tract that drops gradually to extensive mesophotic coral reef ecosystems (MCEs). On the southern edge, adjacent to the islands, the reefs zones are compressed and it drops quickly to the MCEs, creating a nearly continuous MCE surrounding the whole reef platform (Goodbody-Gringley et al., 2019). These MCEs are in close proximity to the shallow reefs and all reef zones are easily accessible for conducting scientific research. Shallow water coral cover in Bermuda ranks among the highest in the Caribbean and western Atlantic with an estimated mean overall cover of 38.6% (Jackson et al., 2014). Deep reef zones (below 50–60 m), such as the sites examined in the present study, are composed of extensive rhodolith beds and occasional outcroppings of hard-bottom reef with sparse scleractinian corals and low topographic complexity (Goodbody-Gringley et al., 2019).
Surveys were performed at 11 sites at 60 m depth around Bermuda between 8 September 2013 and 26 September 2015 (Figure 2). At each site, lionfish were counted within a single 25 m × 10 m quadrat, in which a diver swam in a zigzag formation and closely examined all possible habitat for lionfish, as suggested by Green et al. (2012). While genetic evidence indicates that both P. volitans and P. miles are present in Bermuda (Betancur et al., 2011), identification to the species level is not possible in situ, and thus the general classification of ‘lionfish’ in the present study includes both species. Potential prey fish, defined as any teleost individual <15 cm in total length (TL), were surveyed within 1 m of each side of a 30 m transect tape laid adjacent to the lionfish quadrat, for a total area of 60 m2 per survey (Lesser and Slattery, 2011; Pinheiro et al., 2013, 2016). Along each transect, the number of individual prey fish of each species was tallied and TL of each fish was estimated by eye and classified into 5 cm size classes. The lionfish quadrat and prey fish transects at each site were laid along the reef slope to ensure a constant depth, beginning at the closest non-living reef structure encountered to which the tapes could be secured. Bottom temperature was also recorded after a minimum of 15-min acclimation during each dive using Shearwater Petrel dive computers (precision ± 0.5°C), which continuously logged temperature throughout the dive. The number of prey fish survey transects performed at each site varied from two to six depending on the number of divers. The two sites with the highest lionfish densities (Tiger and XL) were surveyed twice, with parameters averaged across all surveys. Site names, GPS coordinates, survey dates, number of prey fish transects, lionfish density (±SE where applicable), and bottom temperature (±SE where applicable) are included in Table 1.
Figure 2. Map of Bermuda indicating the location of mesophotic sites (60 m) where surveys were performed. The size of the lionfish image at each site corresponds to the relative density (fish ha–1).
Table 1. Name and GPS locations of all survey sites, including the date of each survey, the number of prey fish surveys performed, number of lionfish per hectare, and the bottom temperature (°C) recorded at the time of the survey.
Lionfish and prey fish densities were calculated as the number of fishes per hectare, where prey fish densities represent the mean across transects for each site. Mean prey fish biomass was estimated based on total length–mass relationships from Froese and Pauly (2014), utilizing the mid-point of each 5-cm size class. When no relationship was available for a species, an average for the genus or family was applied (Froese and Pauly, 2014). Species diversity was determined using the Shannon-Weaver diversity index and averaged across transects for each site (Hill, 1973).
A correlation matrix with pairwise correlation analyses was used to compare all physical and biological parameters, including lionfish density, prey fish density, prey fish biomass, species diversity, and bottom seawater temperature, as well as biomass of the top three most abundant species, Paranthias furcifer, Chromis enchrysura, and Chromis bermudae. Further comparison of the influence of potential drivers on lionfish density was examined with a Generalized Linear Model (GLM) using a gamma distribution, which resulted in normal distributions for each driver based on visualization with normal quantile-quantile plots (Supplementary Figure S1). As all factors co-varied using a single model (Supplementary Table S1), separate GLMs were required for each potential driver, with the best fit model determined using an Akaike Information Criterion (AIC) model selection approach (Akaike, 1974). Model results were visualized using a principal component analysis incorporating maximum likelihood factor analysis. Prey fish community composition was analyzed for percent contribution of each species across sites, with communities compared using a k-means cluster analysis. All statistical analyses and data visualizations were done in R version 3.4.3 (R Core Team, 2017) with RStudio 1.1.423 (RStudio Team, 2016) using the packages tidyverse (Wickham, 2017), vegan (Oksanen et al., 2018), ggplot2 (Wickham, 2009), ggbiplot (Vu, 2011), ggcorrplot (Kassambara, 2016), devtools (Wickham et al., 2018), and ggfortify (Tang et al., 2016); data and code are available on Zenodo (doi: 10.5281/zenodo.260282).
Significant positive correlations were found between lionfish density and prey fish density (r = 0.7; p = 0.03) and between lionfish density and prey fish biomass (r = 0.7; p = 0.01), where higher lionfish density was correlated with higher prey fish density and higher prey fish biomass (Figure 3). No significant correlations were found between prey fish diversity and any other parameter (Figure 3; α = 0.05). Species-specific correlations of prey fish biomass with lionfish density revealed a significant positive correlation between lionfish density and P. furcifer biomass (r = 0.8; p = 0.006), while correlations were not significant between lionfish density and C. enchrysura biomass (r = 0.2; p = 0.50) or C. bermudae biomass (r = −0.2; p = 0.63; Figure 3). Lionfish density was significantly negatively correlated with bottom temperature (r = −0.7; p = 0.02), where higher lionfish density was found at sites with lower temperatures (Figure 3). Likewise, a significant negative correlation was found for bottom temperature with prey fish biomass (r = −0.6; p = 0.043; Figure 3). However, no significant correlations were found between temperature and prey fish density or diversity (Figure 3).
Figure 3. Correlation matrix for comparisons between lionfish density (fish ha–1), prey fish density (fish ha–1), prey fish biomass (kg ha–1), prey fish diversity (Shannon-Weaver Index), P. furcifer biomass (kg ha–1), C. enchrysura biomass (kg ha–1), C. bermudae biomass (kg ha–1), and bottom seawater temperature (°C). Positive correlations are indicated with warm hues (red); negative correlations are indicated with cool hues (violet). Strength of the correlation (r) is indicated by the intensity of the color, where darker colors have stronger correlations, with non-significant correlations crossed out (α = 0.05).
A GLM with gamma distribution including all factors revealed covariance among factors, thereby requiring separate models for each potential driver with best fit found based on AIC model selection. Results of the GLMs show a significant effect of temperature on lionfish density, while no other comparisons were significant (Figure 4 and Table 2). Furthermore, the GLM for lionfish density by temperature resulted in the lowest AIC value compared to the other models, with the closest second being prey fish biomass (+1.54 AIC), indicating that the model for temperature as a potential driver of lionfish density was the best fit (Table 2).
Figure 4. Factanal PCA plot of relative effect of prey fish density (fish ha–1), prey fish biomass (kg ha–1), prey fish diversity (Shannon-weaver Index), P. furcifer biomass (kg ha–1), and bottom temperature (°C) on lionfish density (no. fish ha–1 indicated by relative size and color of points). Arrows indicate eigenvalues. Variables with a significant effect are indicated with an asterisk (*; α = 0.05) as determined by GLM with gamma distribution.
Table 2. Results of generalized linear models (GLM) with gamma distribution for comparisons of lionfish density with prey fish biomass (kg/ha), prey fish density (fish ha–1), prey fish diversity (Shannon-Weaver index), biomass of P. furcifer (kg/ha), and bottom temperature (°C).
Prey fish species composition differed by site (Figure 5), however, the most abundant species across sites were consistently C. bermudae (972 fish ha–1 ± 315), C. enchrysura (974 fish ha–1 ± 465), and P. furcifer (845 fish ha–1 ± 499), with the next most abundant species (Chaetodon sedentarius) present at considerably lower densities (193 fish ha–1 ± 45). Cluster analysis using k-means revealed two distinct communities (Figure 6), which group based on geographic location (Figure 2). The first group consists of sites that are all located along the Northeast edge of the Bermuda platform, where the dominant prey fish species was C. bermudae (Figures 5, 6). The latter group consists of all other sites, spanning the Southern and Western edges, where the dominant prey fish species was either C. enchrysura or P. furcifer (Figures 5, 6). Cluster analysis however, did not reveal any relationship of species assemblages and lionfish densities, as sites did not cluster relative to lionfish densities.
Figure 5. Heat map of prey fish community composition at each mesophotic (60 m) site surveyed. Hue intensity indicates relative percent contribution per species to the total population at each site based on density (mean no. fish ha–1).
Figure 6. K-means cluster analysis of prey fish community composition at each mesophotic (60 m) site; groupings based on 2 clusters, axes represent principal components.
The present study documents significant correlations between lionfish densities and ecological as well as environmental parameters related to fish community structure, which suggest that seawater temperatures and prey availability may affect lionfish distribution on mesophotic reefs in Bermuda. Our data show significant positive correlations between lionfish density and prey fish density as well as prey fish biomass, where an increase in lionfish density is associated with an increase in prey fish abundance (Figures 3, 4). Moreover, lionfish density and prey fish biomass were negatively correlated with bottom seawater temperature, where higher lionfish density and prey fish biomass were found in colder waters (Figures 3, 4).
Previous examinations of lionfish distribution patterns have failed to find an ecological driver for the presence of lionfish. For example, Bejarano et al. (2015) found lionfish densities in Little Cayman varied irrespective of prey fish density. Likewise, Anton et al. (2014) found no correlations between lionfish densities and prey density or predator abundance in the Bahamas. Yet, a positive relationship with food availability has been clearly defined for terrestrial predator populations, where the density of predators is known to be dependent on prey biomass (Carbone and Gittleman, 2002). This discrepancy may be due to long-term removal of fish species simultaneously from all trophic levels, which is typical of subsistence fishing practices on Caribbean coral reefs, masking our ability to track patterns of predator-prey relationships (Paddack et al., 2009; Alvarez-Filip et al., 2015). For example, Newman et al. (2006) found that while increased predator biomass was correlated with increased fish biomass across several reef sites spanning the Caribbean, predators were only abundant within large marine reserves, suggesting that populations outside of the reserves may be more strongly driven by fishing pressure than by natural fluctuations in predator-prey abundances.
MCEs are often buffered from anthropogenic impacts, including overfishing (Tyler et al., 2009; Lindfield et al., 2016; Pinheiro et al., 2016), likely because of their distance from shore in most regions, as well as the additional costs of fishing at these depths. Fish traps, traditionally used across a broad range of depths by Bermuda’s commercial fishery, were banned in 1990 (Butler et al., 1993), and spear fishing, with the exception of permitted lionfish culling, is restricted to recreational users without SCUBA (Bermuda Fisheries Act, 1972; Fisheries Regulations, 2010). Thus, fishing impacts are confined to relatively shallow areas today. Indeed, fish diversity and biomass are documented to increase with increasing depth within the mesophotic range in Bermuda (Pinheiro et al., 2016). However, Stefanoudis et al. (in press) found a gradual decrease in fish biomass with depth over a broader depth range (to 90 m) on Bermuda MCEs but noted significant variability in lionfish density at 60 m within their 4 study sites, with three sites in common to this study. Thus, despite continued hook and line fisheries and occasional bycatch in lobster traps, fish communities in Bermuda in general (Paddack et al., 2009; Jackson et al., 2014), and at mesophotic depths in particular, may be less impacted by fishing pressure than those in other locations where lionfish have been studied. Hence, the positive correlation found between lionfish density and prey fish biomass in this study may be facilitated by reduced fishing pressure at the surveyed sites exposing predator-prey relationships that might be masked by the influence of overfishing in other regions. Moreover, the presence of lionfish in Bermuda for nearly two decades has likely allowed sufficient time for this invasive species to adapt to local conditions and distributions of prey, in turn facilitating the emergence of detectable patterns of distribution based on ecological characteristics.
Although variations in overall prey fish community assemblages between sites were not related to lionfish density (Figure 6), a significant correlation was found between lionfish density and P. furcifer biomass (Figure 3). Paranthias furcifer is the second most common teleost prey species by occurrence consumed by lionfish in Bermuda, following the top teleost prey species, Thalassoma bifasciatum (Eddy et al., 2016), which was not present in significant abundance at the sites surveyed for this study (<0.5% contribution to biomass; Figure 5). On the other hand, despite being the top two contributors to fish communities across the MCEs examined (Figure 5), C. enchrysura and C. bermudae biomass did not correlate significantly with lionfish density (Figure 3). In contrast, Harter et al. (2017) found C. enchrysura to be a significant predictor of lionfish densities on MCEs in the Pulley Ridge reef system. In Bermuda, C. enchrysura and C. bermudae are not typically consumed by lionfish (Eddy et al., 2016), and hence the lack of correlation with these species may point to the influence of preferential feeding habits on the relationship between lionfish density and availability of preferred prey types, in this case P. furcifer. It is important to note, however, that a large portion of the lionfish diet in Bermuda is composed of invertebrates, such as crustaceans and mollusks, which were not included in the present study because of their diurnal crypticity, and thus the influence of these prey types on lionfish distribution remains unknown (Eddy et al., 2016). Therefore, further comparisons of feeding patterns in relation to prey availability as predictors of lionfish distribution should include a broader variety of prey types at additional locations, such as Pulley Ridge, Cayman Islands etc., in order to advance our understanding of this relationship.
The strongest driver of lionfish density, however, was found to be seawater temperature, with significantly more lionfish found at colder locations (Figures 3, 4). Previous examinations of the impact of temperature on lionfish densities have focused on the minimum temperatures at which lionfish are found, where the minimum thermal limit for lionfish survival is 10°C and feeding ceases at 15°C, thereby limiting their latitudinal and bathymetric distribution (Kimball et al., 2004). Within the range of temperature tolerance, however, only a few studies address variations in lionfish densities in relation to seawater temperature. In their native habitat, which ranges from South Korea at 35°N to New Zealand at 34°S, higher densities of P. volitans are found at high latitude regions compared to low latitude locations. However, there is no correlation between temperature and lionfish density within locations across this range (Kulbicki et al., 2012). Within the invaded range, along the coast of North Carolina, Whitfield et al. (2014) found the highest densities of lionfish at the deepest sites examined (38–46 m depths), which they suggest may be related to warmer mean winter temperatures at depth, although no variation in temperature was found among sites at the time of surveys.
Temperature is documented to affect the bioenergetics of lionfish, with variations in growth and consumption rates occurring in habitats with differing temperature regimes. For example, Edwards et al. (2014) found lionfish growth rates in tropical waters near the Cayman Islands to be higher than those found by Potts et al. (2010) and Barbour et al. (2011) in temperate waters off North Carolina. Likewise, Cerino et al. (2013) found consumption rates by lionfish increase with increasing water temperature from 14 to 29.7°C. Metabolism, on the other hand, is slowed in colder temperatures for other fish species and thus assimilation may be higher, thereby reducing overall energetic costs when residing in colder waters (Bevelhimer and Adams, 1993). Previous studies have shown that fish of a particular species will spend the majority of time (75%) within a thermal range that promotes optimal physiological activities and results in maximum growth (Magnuson et al., 1979; Magnuson and Destasio, 1997). The increased density of lionfish at colder sites in Bermuda, therefore, may reflect an energy conservation strategy involving trade-offs between rates of consumption and metabolism (Sims et al., 2006; Eddy, 2016). However, additional studies of lionfish bioenergetics are required that include seasonal variations, as anecdotal evidence in Bermuda indicates seasonal shifts in lionfish distribution to shallower reefs in winter months (pers. obs. all authors).
The presence of colder water at specific mesophotic sites might also suggest mixing of deep water at or below the thermocline, which may be promoted by site specific bathymetry, as the tidal exchange around the Bermuda Islands and over the shallow reef platform appears to promote upwelling at the eastern and western ends of the reef system (Goodbody-Gringley et al., 2019). Moore (1949) studied zooplankton horizontal and vertical distribution patterns around Bermuda and speculated on the presence of episodic upwelling to account for some patterns. Vertical mixing is typically associated with increased transport of nutrients that fuel the population growth of zooplankton species in the waters surrounding Bermuda (Bodungen et al., 1982; Sigman and Hain, 2012). For example, Jiang et al. (2007) found that a decrease in temperature by 0.4–0.8°C in the open ocean near Bermuda was correlated with an increase in zooplankton biomass at 70-m depth, which corresponds to the deep chlorophyll maximum zone (DCM) around the thermocline. Planktivores, such as P. furcifer, generally concentrate along the reef edge and feed mainly in midwater on zooplankton such as copepods, pelagic tunicates, and various crustacean larvae (Hobson, 1991; Rocha et al., 2008). This feeding strategy makes water currents important for supplying food to planktivores, resulting in stationary aggregations that feed around currents and then dissipate as currents slacken (Stevenson et al., 1972; Thresher, 1983; Hobson, 1991). In fact, previous studies document planktivores dominating fish community assemblages on MCEs (Colin et al., 1986; Dennis and Bright, 1988; Garcia-Sais, 2010; Bryan et al., 2013; Bejarano et al., 2014; Pinheiro et al., 2016; Rosa et al., 2016), and often cite increased abundances of zooplankton from upwelling currents as a likely cause. Thus, increased lionfish densities associated with colder seawater temperatures may be due to the effects of vertical mixing, via upwelling, increasing local productivity and fueling the food chain at specific sites, resulting in high abundances of planktivorous prey fishes and thus in turn lionfish (Figure 5), particularly given that prey fish abundance and temperature were found to co-vary with lionfish density (Figure 4).
Importantly, temperature is not the only physical characteristic that may impact lionfish distribution. For example, despite being one of the coldest sites, the surveyed area at North Northeast did not support the highest density of lionfish (Table 1), which is likely attributable to the composition of the benthos at that site being dominated by beds of rhodolith balls and macroalgae, and lacking solid reef substrate or topographic complexity. Previous studies have found habitat complexity to be a strong predictor of lionfish abundances on shallow reefs, where increased lionfish density is associated with higher topographic complexity (Bejarano et al., 2015) and/or benthic community diversity (Hunt et al., 2019). However, in Bermuda, topographic complexity at the depths surveyed in this study is low overall, with sites generally dominated by rhodolith beds and occasional outcroppings of low relief reef structures (Figure 1; Goodbody-Gringley et al., 2019). In the northern Gulf of Mexico, lionfish are found at increased densities on artificial reef structures compared to natural reef habitat (Dahl and Patterson, 2014). Surrounded by large expanses of flat sandy bottom, these artificial reefs are thought to serve to concentrate settling juvenile lionfish that cue to high vertical relief (Dahl and Patterson, 2014). Thus, slight differences in benthic composition, such as the occasional outcropping of solid substrate within a field of rhodoliths, may also be influencing lionfish distributions in MCEs. Full characterization of the physical environment, including plankton abundance and composition, as well as benthic habitat characterization in MCEs around Bermuda would aid in such interpretations.
The extent of the lionfish invasion and the formation of reproductive populations throughout the invaded range indicates that the lionfish are established permanently in the Caribbean and Western Atlantic. While complete eradication of lionfish is unlikely, various management strategies have been proposed to reduce and/or control local populations (Albins and Hixon, 2008; Côté and Maljkovic, 2010; Arias-Gonzalez et al., 2011; Green et al., 2011; Muñoz et al., 2011; Pitt and Trott, 2014). The majority of control measures involve managed manual removal through targeted spear fishing by SCUBA and free divers and/or establishment of a commercial fishery aimed at increasing public consumption of lionfish (Muñoz et al., 2011; Frazer et al., 2012; Albins and Hixon, 2013; Gleason and Gullick, 2014). Recent work indicates that targeted removal by organized volunteers can be successful in controlling local populations, finding that continued removal of individuals from shallow populations results in an overall reduction of lionfish biomass at fished locations compared to unfished locations (de León et al., 2013; Green and Côté, 2014). However, the inability to remove individuals from deep reefs will enable continued recruitment to fished locations, as mesophotic lionfish are highly fecund (Eddy, 2016; Andradi-Brown et al., 2017b), potentially offsetting the efficacy of local shallow water culling (Johnston and Purkis, 2015; Andradi-Brown et al., 2017a). Given the documented importance of MCEs as critical nursery habitats for several reef fish species (Lobel, 1981; Randall and Chen, 1985; Brokovich et al., 2007; Garcia-Sais, 2010; Sazima et al., 2010; Lindfield et al., 2016), it is important that lionfish populations at mesophotic depths are incorporated into management strategies. The results presented here suggest that lionfish distribution on mesophotic reefs is influenced in part by physical parameters of the environment, such as temperature, as well as ecological parameters, such as the availability of potential prey. Understanding the factors that drive lionfish distributions will ultimately aid in our ability to successfully identify and target areas with high lionfish densities, thereby increasing the efficacy of management and control efforts throughout the invaded range in order to minimize negative impacts to these threatened ecosystems.
All the authors developed the concept for this study, executed the field work, collected the data, and contributed to writing of the manuscript. GG-G undertook statistical analyses and composed data visualizations.
This work was primarily funded by the Darwin Plus Overseas Territories Environment and Climate Fund through the United Kingdom Department of Environment Food and Rural Affairs (DPLUS001) to support costs associated with field work, salary support, and travel. Funds were provided by the Bermuda Zoological Society Research and Conservation Committee to extend the field work. Support for the associated Ph.D. student, CE provided through a National Science Foundation Graduate Research Fellowship (DGE-1144241), and by the University of Massachusetts at Dartmouth. Funds were provided by the BIOS Cawthorn Innovation Fund and the Bermuda Zoological Society to cover the costs of open-access publication.
The authors declare that the research was conducted in the absence of any commercial or financial relationships that could be construed as a potential conflict of interest.
The reviewer DA-B declared a past co-authorship with the authors to the handling Editor.
The authors thank G. Maddocks, S. Bennett, F. Williams Jr., M. Cooper, M. Gascoigne, and J. Adams for support and assistance with aspects of technical diving for this research. Special thanks are also given to N. Silbiger for assistance with statistics and coding.
The Supplementary Material for this article can be found online at: https://www.frontiersin.org/articles/10.3389/fmars.2019.00258/full#supplementary-material
Akaike, H. (1974). A new look at the statistical model identification. IEEE Trans. Automat. Control 19, 716–723. doi: 10.1109/TAC.1974.1100705
Albins, M. (2013). Effects of invasive Pacific red lionfish Pterois volitans versus a native predator on Bahamian coral-reef fish communities. Biol. Invasions 15, 29–43. doi: 10.1007/s10530-012-0266-1
Albins, M., and Hixon, M. (2008). Invasive Indo-Pacific lionfish Pterois volitans reduce recruitment of Atlantic coral-reef fishes. Mar. Ecol. Prog. Ser. 367, 233–238. doi: 10.3354/meps07620
Albins, M., and Hixon, M. (2013). Worst case scenario: potential long-term effects of invasive predatory lionfish (Pterois volitans) on Atlantic and Caribbean coral-reef communities. Environ. Biol. Fishes 96, 1151–1157. doi: 10.1007/s10641-011-9795-1
Alvarez-Filip, L., Paddack, M. J., Collen, B., Robertson, D. R., and Côté, I. M. (2015). Simplification of Caribbean reef-fish assemblages over decades of coral reef degradation. PLoS One 10:e0126004. doi: 10.1371/journal.pone.0126004
Andradi-Brown, D. A., Grey, R., Hendrix, A., Hitchner, D., Hunt, C. L., Gress, E., et al. (2017a). Depth-dependent effects of culling – do mesophotic lionfish populations undermine current management? R. Soc. Open Sci. 4:170027. doi: 10.1098/rsos.170027
Andradi-Brown, D. A., Vermeij, M. J. A., Slattery, M., Lesser, M., Bejarano, I., Appeldoorn, R., et al. (2017b). Large-scale invasion of western Atlantic mesophotic reefs by lionfish potentially undermines culling-based management. Biol. Invasions 19, 939–954. doi: 10.1007/s10530-016-1358-0
Anton, A., Simpson, M. S., and Vu, I. (2014). Environmental and biotic correlates to lionfish invasion success in Bahamian coral reefs. PLoS One 9:e106229. doi: 10.1371/journal.pone.0106229
Arias-Gonzalez, J. E., Gonzalez-Gandara, D., Cabrera, J. L., and Christensen, V. (2011). Predicted impact of the invasive lionfish Pterois volitans on the food web of a Caribbean coral reef. Environ. Res. 111, 917–925. doi: 10.1016/j.envres.2011.07.008
Barbour, A. B., Allen, M. S., Frazer, T. K., and Sherman, K. D. (2011). Evaluating the potential efficacy of invasive lionfish (Pterois volitans) removals. PLoS One 6:e19666. doi: 10.1371/journal.pone.0019666
Bejarano, I., Appeldoorn, R., and Nemeth, M. (2014). Fishes associated with mesophotic coral ecosystems in La Parguera, Puerto Rico. Coral Reefs 33, 313–328. doi: 10.1007/s00338-014-1125-6
Bejarano, S., Lohr, K., Hamilton, S., and Manfrino, C. (2015). Relationships of invasive lionfish with topographic complexity, groupers, and native prey fishes in Little Cayman. Mar. Biol. 162, 253–266. doi: 10.1007/s00227-014-2595-3
Benkwitt, C. E., Albins, M. A., Buch, K. L., Ingeman, K. E., Kindinger, T. L., Pusack, T. J., et al. (2017). Is the lionfish invasion waning? Evidence from The Bahamas. Coral Reefs 36, 1255–1261. doi: 10.1007/s00338-017-1620-7
Bermuda Fisheries Act (1972). Available at: www.bermudalaws.bm/laws/Consolidated%20Laws/Fisheries%20Act%201972.pdf (accessed 2016).
Betancur, R., Hines, A., Acero, P. A., Orti, G., Wilbur, A. E., and Freshwater, D. W. (2011). Reconstructing the lionfish invasion: insights into Greater Caribbean biogeography. J. Biogeogr. 38, 1281–1293. doi: 10.1111/j.1365-2699.2011.02496.x
Bevelhimer, M., and Adams, S. (1993). A bioenergetics analysis of diel vertical migration by Kokanee salmon, Oncorhynchus nerka. Can. J. Fish. Aquat. Sci. 50, 2336–2349. doi: 10.1139/f93-258
Bodungen, B. V., Jickells, T. D., Smith, S. R., Ward, J. A. D., and Hillier, G. B. (1982). The Bermuda Marine Environment - Volume III. The final report of the Bermuda Inshore Waters Investigations 1975-1980. BBSR Spec. Publ. 18:123.
Brokovich, E., Eindbinder, S., Kark, S., Shashar, N., and Kiflawi, M. (2007). A deep nursery for juveniles of the zebra angelfish Genicanthus caudovittatus. Environ. Biol. Fishes 80, 1–6. doi: 10.1007/s10641-006-9160-y
Bryan, D. R., Kilfoyle, K., Gilmore, R. G., and Spieler, R. E. (2013). Characterization of the mesophotic reef fish community in south Florida, USA. J. Appl. Ichthyol. 29, 108–117. doi: 10.1111/j.1439-0426.2012.02055.x
Butler, J. N., Burnett-Herkes, J., Barnes, J. A., and Ward, J. (1993). The Bermuda fisheries a tragedy of the commons averted? Environ. Sci. Policy Sustain. Dev. 35, 6–33. doi: 10.1080/00139157.1993.9929067
Carbone, C., and Gittleman, J. L. (2002). A common rule for the scaling of carnivore density. Science 295, 2273–2276. doi: 10.1126/science.1067994
Cerino, D., Overton, A. S., Rice, J. A., and Morris, J. A. J. (2013). Bioenergetics and trophic impacts of the invasive Indo-Pacific lionfish. Trans. Am. Fish. Soc. 142, 1522–1534. doi: 10.1080/00028487.2013.811098
Coates, K., Fourqurean, J., Kenworthy, W., Logan, A., Manuel, S., and Smith, S. R. (2013). “Introduction of Bermuda: geology, oceanography and climate,” in Coral Reefs of the World. Coral Reefs of the UK Overseas Territories, ed. C. Sheppard (Berlin: Springer), 115–133. doi: 10.1007/978-94-007-5965-7_10
Colin, P. L., Devaney, D. M., Hollis-Colinvaux, L., Suchanek, T. H., and Harrison, J. T. III (1986). Geology and biological zonation of the reef slope, 50-360 m depth at Enewetak Atoll, Marshall Islands. Bull. Mar. Sci. 38, 111–128.
Côté, I., and Maljkovic, A. (2010). Predation rates of Indo-Pacific lionfish on Bahamian coral reefs. Mar. Ecol. Prog. Ser. 404, 219–225. doi: 10.3354/meps08458
Dahl, K. A., and Patterson, W. F. III (2014). Habitat-specific density and diet of rapidly expanding invasive red lionfish, Pterois volitans, populations in the Northern Gulf of Mexico. PLoS One 9:e105852. doi: 10.1371/journal.pone.0105852
Darling, E., Green, S., O’Leary, J., and Côté, I. (2011). Indo-Pacific lionfish are larger and more abundant on invaded reefs: a comparison of Kenyan and Bahamian lionfish populations. Biol. Invasions 13, 2045–2051. doi: 10.1007/s10530-011-0020-0
de León, R., Vane, K., Bertuol, P., Chamberland, V. C., Simal, F., Imms, E., et al. (2013). Effectiveness of lionfish removal efforts in the southern Caribbean. Endanger. Species Res. 22, 175–182. doi: 10.3354/esr00542
Dennis, G. D., and Bright, T. J. (1988). Reef fish assemblages on hard banks in the northwest Gulf of Mexico. Bull. Mar. Sci. 43, 280–307.
Eddy, C. (2016). An Investigation of the Biology and Ecology of the Invasive Lionfish (Pterois volitans and P. miles) to Explore their Ecological Impact and inform Management in Bermuda’s Marine Ecosystem. Ph.D. dissertation, University of Massachusetts Dartmouth, Dartmouth, MA.
Eddy, C., Pitt, J., Morris, J. A. J., Smith, D. B., Goodbody-Gringley, G., and Bernal, D. (2016). Diet and prey selectivity of invasive lionfish (Pterois volitans and P. miles) in Bermuda. Mar. Ecol. Prog. Ser. 558, 193–206. doi: 10.3354/meps11838
Edwards, M. A., Frazer, T. K., and Jacoby, C. A. (2014). Age and growth of invasive lionfish (Pterois spp.) in the Caribbean Sea, with implications for management. Bull. Mar. Sci. 90, 953–966. doi: 10.5343/bms.2014.1022
Ferreira, C. E. L., Luiz, O. J., Floeter, S. R., Lucena, M. B., Barbosa, M. C., Rocha, C. R., et al. (2015). First record of invasive lionfish (Pterois volitans) for the Brazilian coast. PLoS One 10:e0123002. doi: 10.1371/journal.pone.0123002
Fisheries Regulations (2010). Available at: www.bermudalaws.bm/laws/Consoli dated%20Laws/Fisheries%20Regulations%202010.pdf (accessed 2016).
Fogg, A. Q., Hoffmayer, E. R., Driggers, W. B. III, Cambell, M. D., Pellegrin, G. J., and Stein, W. (2013). Distribution and length frequency of invasive lionfish (Pterois sp.) in the Northern Gulf of Mexico. Gulf Caribb. Res. 25, 111–115.
Frazer, T. K., Jacoby, C. A., Edwards, M. A., Barry, S. C., and Manfrino, C. M. (2012). Coping with the lionfish invasion: can targeted removals yield beneficial effects? Rev. Fish. Sci. 20, 185–191. doi: 10.1080/10641262.2012.700655
Garcia-Sais, J., Sabater-Clavell, J., Esteves, R., and Carlo, M. (2012). Fishery Independent Survey of Commercially Exploited Fish and Shellfish Populations from Mesophotic Reefs within the Puertorrican EEZ. Final Report. Caribbean Fishery Management Council. San Juan: CFMC, 1–86.
Garcia-Sais, J. R. (2010). Reef habitats and associated sessile-benthic and fish assemblages across a euphotic–mesophotic depth gradient in Isla Desecheo, Puerto Rico. Coral Reefs 29, 277–288. doi: 10.1007/s00338-009-0582-9
Gleason, J., and Gullick, H. (2014). Bermuda Lionfish Control Plan. Bermuda: Bermuda Lionfish Taskforce, 1–60.
Goodbody-Gringley, G., Noyes, T. J., and Smith, S. R. (2019). “Bermuda,” in In Coral Reefs of the World. Mesophotic Coral Ecosystems (MCEs), eds Y. Loya, K. A. Puglise, and T. C. L. Bridge (Berlin: Springer).
Green, S. J., Akins, J. L., and Côté, I. M. (2011). Foraging behaviour and prey consumption in the Indo-Pacific lionfish on Bahamian coral reefs. Mar. Ecol. Prog. Ser. 433, 159–167. doi: 10.3354/meps09208
Green, S. J., Akins, J. L., Maljkovic, A., and Côté, I. M. (2012). Invasive lionfish drive Atlantic coral reef fish declines. PLoS One 7:e32596. doi: 10.1371/journal.pone.0032596
Green, S. J., and Côté, I. (2014). Trait-based diet selection: prey behavior and morphology predict vulnerability to predation in reef fish communities. Ecology 83, 1451–1460. doi: 10.1111/1365-2656.12250
Gress, E., Andradi-Brown, D., Woodall, L., Schofield, P. J., Stanley, K., and Rogers, A. D. (2017). Lionfish (Pterois spp.) invade the upper-bathyal zone in the western Atlantic. PeerJ 5:e3683. doi: 10.7717/peerj.3683
Hackerott, S., Valdivia, A., Cox, C. E., Silbiger, N. J., and Bruno, J. F. (2017). Invasive lionfish had no measurable effect on prey fish community structure across the Belizean Barrier Reef. PeerJ 5:e3270. doi: 10.7717/peerj.3270
Hackerott, S., Valdivia, A., Green, S. J., Côté, I. M., Cox, C. E., Akins, L., et al. (2013). Native predators do not influence invasion success of Pacific lionfish on Caribbean reefs. PLoS One 8:e68259. doi: 10.1371/journal.pone.0068259
Harter, S. L., Moe, H., Reed, J. K., and David, A. W. (2017). Fish assemblages associated with red grouper pits at Pulley Ridge, a mesophotic reef in the Gulf of Mexico. Fish. Bull. 115, 419–432. doi: 10.7755/fb.115.3.11
Hill, M. O. (1973). Diversity and evenness: a unifying notaion and its consequences. Ecology 54, 427–432. doi: 10.2307/1934352
Hobson, E. (1991). “Trophic relationships of fishes specialized to feed on zooplankters above coral reefs,” in The Ecology of Fishes on Coral Reefs, ed. P. Sale (San Diego, CA: Academic Press), 69–95. doi: 10.1016/b978-0-08-092551-6.50009-x
Hunt, C. L., Kelly, G. R., Windmill, H., Curtis-Quick, J., Conlon, H., Bodmer, M. D. V., et al. (2019). Aggregating behaviour in invasive Caribbean lionfish is driven by habitat complexity. Sci. Rep. 9:783. doi: 10.1038/s41598-018-37459-w
Jackson, J. B. C., Donovan, M., Cramer, K., and Lam, V. (2014). Status and Trends of Caribbean Coral Reefs: 1970-2012. Gland: Global Coral Reef Monitoring Network.
Jiang, S., Dickey, T. D., Steinberg, D. K., and Madin, L. P. (2007). Temporal variability of zooplankton biomass from ADCP backscatter time series data at the Bermuda Testbed Mooring site. Deep Sea Res. Part I Oceanogr. Res. Pap. 54, 608–636. doi: 10.1016/j.dsr.2006.12.011
Johnston, M. W., and Purkis, S. J. (2011). Spatial analysis of the invasion of lionfish in the western Atlantic and Caribbean. Mar. Pollut. Bull. 62, 1218–1226. doi: 10.1016/j.marpolbul.2011.03.028
Johnston, M. W., and Purkis, S. J. (2015). A coordinated and sustained international strategy is required to turn the tide on the Atlantic lionfish invasion. Mar. Ecol. Prog. Ser. 533, 219–235. doi: 10.3354/meos11399
Kassambara, A. (2016). ggcorrplot: Visualization of a Correlation Matrix using ’ggplot2’. R package version 0.1.1. Available at: https://CRAN.R-project.org/package=ggcorrplot (accessed September 11, 2018).
Keane, R. M., and Crawley, M. J. (2002). Exotic plant invasions and the enemy release hypothesis. Trends Ecol. Evol. 17, 164–170. doi: 10.1016/s0169-5347(02)02499-0
Kimball, M. E., Miller, J., Whitfield, P. E., and Hare, J. A. (2004). Thermal tolerance and potential distribution of invasive lionfish (Pterois volitans/miles complex) on the east coast of the United States. Mar. Ecol. Prog. Ser. 283, 269–278. doi: 10.3354/meps283269
Kulbicki, M., Beets, J., Chabanet, P., Cure, K., Darling, E., Floeter, S. R., et al. (2012). Distributions of Indo-Pacific lionfishes Pterois spp. in their native ranges: implications for the Atlantic invasion. Mar. Ecol. Prog. Ser. 446, 189–205. doi: 10.3354/meps09442
Layman, C., and Allgeir, J. (2012). Characterizing trophic ecology of generalist consumers: a case study of the invasive lionfish in The Bahamas. Mar. Ecol. Prog. Ser. 448, 131–141. doi: 10.3354/meps09511
Lesser, M. P., and Slattery, M. (2011). Phase shift to algal dominated communities at mesophotic depths associated with lionfish (Pterois volitans) invasion on a Bahamian coral reef. Biol. Invasions 13, 1855–1868. doi: 10.1007/s10530-011-0005-z
Lindfield, S. J., Harvey, E. S., Halford, A. R., and McIlwain, J. L. (2016). Mesophotic depth as refuge areas for fishery-targeted species on coral reefs. Coral Reefs 35, 125–137. doi: 10.1007/s00338-015-1386-8
Lobel, P. S. (1981). Bodianus prognathus (Labridae, Pisces), a new longnose hogfish from the Central Pacific. Pac. Sci. 35, 45–50.
Locke, J., Bilewitch, J., and Coates, K. (2013a). “Scleractinia, Octocorallia and Antipatharia of Bermuda’s reefs and deep-water coral communities: a taxonomic perspective including new records,” in Coral Reefs of the World. Coral Reefs of the UK Overseas Territories, ed. C. Sheppard (Berlin: Springer), 189–200. doi: 10.1007/978-94-007-5965-7_14
Locke, J., Coates, K., Bilewitch, J., Holland, L., Pitt, J., Smith, S. R., et al. (2013b). “Biogeography, biodiversity and connectivity of Bermuda’s coral reefs,” in Coral Reefs of the World. Coral Reefs of the UK Overseas Territories, ed. C. Sheppard (Berlin: Springer), 153–172. doi: 10.1007/978-94-007-5965-7_12
Magnuson, J. J., Crowder, L., and Medvick, P. (1979). Temperature as an ecological resource. Am. Zool. 19, 331–343. doi: 10.1093/icb/19.1.331
Magnuson, J. J., and Destasio, B. T. (1997). “Thermal niche of fishes and global warming,” in Global Warming: Implications for Freshwater and Marine Fish, eds D. Wood and D. McDonald (Cambridge: Cambridge University Press), 377–408. doi: 10.1017/cbo9780511983375.016
Moore, H. B. (1949). The zooplankton of the upper waters of the Bermuda area of the North Atlantic. Bull. Bingham Oceanogr. Collect. 12, 1–97.
Morris, J., and Akins, J. L. (2009). Feeding ecology of invasive lionfish (Pterois volitans) in the Bahamian archipelago. Environ. Biol. Fishes 86, 389–398. doi: 10.1007/s10641-009-9538-8
Muñoz, R. C., Currin, C. A., and Whitfield, P. E. (2011). Diet of invasive lionfish on hard bottom reefs of the Southeast USA: insights from stomach. Mar. Ecol. Prog. Ser. 432, 181–193. doi: 10.3354/meps09154
Newman, M. J. H., Paredes, G. A., Sala, E., and Jackson, J. B. C. (2006). Structure of Caribbean coral reef communities across a large gradient of fish biomass. Ecol. Lett. 9, 1216–1227. doi: 10.1111/j.1461-0248.2006.00976.x
Nuttall, M. F., Johnston, M. A., Eckert, R. J., Embesi, J. A., Hickerson, E. L., and Schmahl, G. P. (2014). Lionfish (Pterois volitans [Linnaeus, 1758] and P. miles [Bennett, 1828]) records within mesophotic depth ranges on natural banks in the Northwestern Gulf of Mexico. Bioinvasions Rec. 3, 111–115. doi: 10.3391/bir.2014.3.2.09
Oksanen, J., Blanchet, F., Friendly, M., Kindt, R., Legendre, P., McGlinn, D., et al. (2018). Vegan: Community Ecology Package. R package version 2.5-1. Available at: https://CRAN.R-project.org/package=vegan (accessed February 4, 2019).
Paddack, M. J., Reynolds, J. D., Aguilar, C., Appeldoorn, R. S., Beets, J., Burkett, E. W., et al. (2009). Recent region-wide declines in Caribbean reef fish abundance. Curr. Biol. 19, 590–595. doi: 10.1016/j.cub.2009.02.041
Peake, J., Bogdanoff, A. K., Layman, C. A., Castillo, B., Reale-Munroe, K., Chapman, J., et al. (2018). Feeding ecology of invasive lionfish (Pterois volitans and Pterois miles) in the temperate and tropical western Atlantic. Biol. Invasions 20, 2567–2597. doi: 10.1007/s10530-018-1720-5
Pinheiro, H. T., Goodbody-Gringley, G., Jessup, M. E., Shepherd, B., Chequer, A., and Rocha, L. A. (2016). Upper and lower mesophotic coral reef fish communities evaluated by underwater visual censuses in two Caribbean locations. Coral Reefs 35, 139–151. doi: 10.1007/s00338-015-1381-0
Pinheiro, H. T., Martins, A. S., and Joyeux, J. C. (2013). The importance of small-scale environment factors to community structure patterns of tropical rocky reef fish. J. Mar. Biol. Assoc. U.K. 93, 1175–1185. doi: 10.1017/s0025315412001749
Pitt, J. M., and Trott, T. M. (2014). Efforts to Develop a Lionfish Specific Trap for use in Bermuda Waters. Marathon, FL: Gulf and Caribbean Fisheries Institute, 188–190.
Potts, J. C., Berrane, D., and Morris, J. A. J. (2010). “Age and growth of lionfish from the western north Atlantic,” in Proceedings of the Annual Meeting Gulf and Caribbean Fisheries Institute, San Andres, 314.
R Core Team (2017). R: A Language and Environment for Statistical Computing. Vienna: R Foundation for Statistical Computing.
Randall, R. E., and Chen, C. (1985). First record of the labrid fish Bodianus cylindriatus (Tanaka) from the Hawaiian Islands. Pac. Sci. 39, 291–293.
Rocha, L. A., Ferreira, B. P., Choat, J. H., Craig, M. T., and Sadovy, Y. (2008). Paranthias furcifer. London: The IUCN Red List of Threatened Species. doi: 10.2305/IUCN.UK.2008.RLTS.T132727A3434288.en
Rosa, M. R., Alves, A. C., Medeiros, D. V., Coni, E. O. C. C., Ferreira, B. P., Rosa, R. D. S., et al. (2016). Mesophotic reef fish assemblages of the remote St. Peter and St. Paul’s Archipelago, Mid-Atlantic Ridges, Brazil. Coral Reefs 35, 113–123. doi: 10.1007/s00338-15-1368-x
Sale, P. F. (2002). Coral Reef Fishes: Dynamics and Diversity in a Complex Ecosystem. San Diego, CA: Academic Press.
Sazima, I., Grossman, A., and Sazima, C. (2010). Deep cleaning: a wrasse and a goby clean reef fish below 60 m depth in the tropical southwestern Atlantic. Mar. Biodivers. Rec. 3, 60–63.
Schofield, P. (2009). Geographic extent and chronology of the invasion of non-native lionfish (Pterois volitans [Linnaeus 1758] and P. miles [Bennett 1828]) in the Western North Atlantic and Caribbean Sea. Aquat. Invasions 4, 473–479. doi: 10.3391/ai.2009.4.3.5
Schofield, P. (2010). Update on geographic spread of invasive lionfishes (Pterois volitans [Linnaeus, 1758] and P. miles [Bennett, 1828]) in the Western North Atlantic Ocean, Caribbean Sea and Gulf of Mexico. Aquat. Invasions 5, S117–S122. doi: 10.3391/ai.2009.4.3.5
Sellers, A. J., Ruiz, G. M., Leung, B., and Torchin, M. E. (2015). Regional variation in parasite species eichness and abundance in the introduced range of the invasive lionfish, Pterois volitans. PLoS One 10:e0131075. doi: 10.1371/journal.pone.0131075
Semmens, B. X., Buhle, E. R., Salomon, A. K., and Pattengill-Semmens, C. V. (2004). A hotspot of non-native marine fishes: evidence for the aquarium trade as an invasion pathway. Mar. Ecol. Prog. Ser. 266, 239–244. doi: 10.3354/meps266239
Sigman, D. M., and Hain, M. P. (2012). The biological productivity of the ocean. Nat. Educ. 3, 1–16.
Sims, D. W., Wearmouth, V. J., Southall, E. J., Hill, J. M., Moore, P., Rawlinson, K., et al. (2006). Hunt warm, rest cool; bioenergetic strategy underlying diel vertical migration of a benthic shark. J. Anim. Ecol. 75, 176–190. doi: 10.1111/j.1365-2656.2005.01033.x
Smith, S. R., Sarkis, S., Murdoch, T. J., Weil, E., Croquer, A., Bates, N. R., et al. (2013). “Threats to coral reefs of Bermuda,” in Coral Reefs of the World. Coral Reefs of the UK Overseas Territories, ed. C. Sheppard (Berlin: Springer), 173–188. doi: 10.1007/978-94-007-5965-7_13
Stefanoudis, P. V., Gress, E., Pitt, J. M., Smith, S. R., Kincaid, T., Rivers, M., et al. (in press). Depth-dependent structuring of reef fish assemblanges from the shallows to the rariphotic zone. Front. Mar. Sci.Google Scholar
Stevenson, R. A. (1972). “Regulation of feeding behavior of the bicolor damselfish (Eupomacentrus particus Poey) by environmental factors,” in Behaviour of Marine Animals. 2: Vertebrates, ed. H. G.Winn (New York, NY: Plenum).
Tang, Y., Horikoshi, M., and Li, W. (2016). ggfortify: unified interface to visualize statistical results of popular R packages. R J. 8, 478–489.
Thresher, R. E. (1983). Environmental correlates of the distribution of planktivorous fishes in the One Tree Reef lagoon. Mar. Ecol. Prog. Ser. 10, 137–145. doi: 10.3354/meps010137
Tuttle, L. J., Sikkel, P. C., Cure, K., and Hixon, M. A. (2017). Parasite-mediated enemy release and low biotic resistance may facilitate invasion of Atlantic coral reefs by Pacific red lionfish (Pterois volitans). Biol. Invasions 19, 563–575. doi: 10.1007/s10530-016-1342-8
Tyler, E., Speight, M., Henderson, P., and Manica, A. (2009). Evidence for a depth refuge effect in artisanal coral reef fisheries. Biol. Conserv. 142, 652–667. doi: 10.1016/j.biocon.2008.11.017
Valdez-Moreno, M., Quintal-Lizama, C., Gomez-Lozano, R., and Garcia-Rivas, M. (2012). Monitoring an alien invasion: DNA barcoding and the identification of lionfish and their prey on coral reefs of the Mexican Caribbean. PLoS One 7:e36636. doi: 10.1371/journal.pone.0036636
Valdivia, A., Bruno, J. F., Cox, C. E., Hackerott, S., and Green, S. J. (2014). Re-examining the relationship between invasive lionfish and native grouper in the Caribbean. PeerJ 2:e348. doi: 10.7717/peerj.348
Vu, V. (2011). ggbiplot: A ggplot2 Based Biplot. R package version 0.55. Available at: http://github.com/vqv/ggbiplot (accessed September, 2018).
Whitfield, P. E., Munoz, R. C., Buckel, C. A., Degan, B. P., Freshwater, D. W., and Hare, J. A. (2014). Native fish community structure and Indo-Pacific lionfish Pterois volitans densities along a depth-temperature gradient in Onslow Bay, North Carolina, USA. Mar. Ecol. Prog. Ser. 509, 241–254. doi: 10.3354/meps10882
Wickham, H. (2017). tidyverse: Easily Install and Load the ’Tidyverse’. R package version 1.2.1. Available at: https://CRAN.R-project.org/package=tidyverse (accessed November 14, 2017).
Wickham, H., Hester, J., and Chang, W. (2018). ”devtools: Tools To Make Developing R Packages Easier”. R package version 1.13.6. Available at: https://CRAN.R-project.org/package=devtools (accessed April 8, 2019).
Keywords: invasive species, mesophotic, Bermuda, prey availability, fish biodiversity, lionfish, temperature effects
Citation: Goodbody-Gringley G, Eddy C, Pitt JM, Chequer AD and Smith SR (2019) Ecological Drivers of Invasive Lionfish (Pterois volitans and Pterois miles) Distribution Across Mesophotic Reefs in Bermuda. Front. Mar. Sci. 6:258. doi: 10.3389/fmars.2019.00258
Received: 30 January 2019; Accepted: 02 May 2019;
Published: 21 May 2019.
Edited by:
Jesús Ernesto Arias González, Centro de Investigación y de Estudios Avanzados (CINVESTAV), MexicoReviewed by:
Carlos Eduardo Leite Ferreira, Universidade Federal Fluminense, BrazilCopyright © 2019 Goodbody-Gringley, Eddy, Pitt, Chequer and Smith. This is an open-access article distributed under the terms of the Creative Commons Attribution License (CC BY). The use, distribution or reproduction in other forums is permitted, provided the original author(s) and the copyright owner(s) are credited and that the original publication in this journal is cited, in accordance with accepted academic practice. No use, distribution or reproduction is permitted which does not comply with these terms.
*Correspondence: Gretchen Goodbody-Gringley, Z3JldGNoZW4uZ2dyaW5nbGV5QGJpb3MuZWR1
Disclaimer: All claims expressed in this article are solely those of the authors and do not necessarily represent those of their affiliated organizations, or those of the publisher, the editors and the reviewers. Any product that may be evaluated in this article or claim that may be made by its manufacturer is not guaranteed or endorsed by the publisher.
Research integrity at Frontiers
Learn more about the work of our research integrity team to safeguard the quality of each article we publish.