- 1Institute for Coastal and Marine Research, Nelson Mandela University, Port Elizabeth, South Africa
- 2Department of Biochemistry and Microbiology, Rhodes University, Grahamstown, South Africa
- 3Department of Oceanography, Institute for Coastal and Marine Research, Nelson Mandela University, Port Elizabeth, South Africa
- 4Department of Ichthyology and Fisheries Science, Rhodes University, Grahamstown, South Africa
- 5Department of Zoology, Institute for Coastal and Marine Research, Nelson Mandela University, Port Elizabeth, South Africa
- 6Department of Science and Technology/National Research Foundation Centre of Excellence at the Percy FitzPatrick Institute of African Ornithology, University of Cape Town, Cape Town, South Africa
- 7South African National Biodiversity Institute, Port Elizabeth, South Africa
- 8Department of Zoology, DST/NRF Centre of Excellence at the Percy FitzPatrick Institute, Nelson Mandela University, Port Elizabeth, South Africa
- 9Department of Zoology, Nelson Mandela University, Port Elizabeth, South Africa
In 2017, South Africa became the first African country to draft Marine Spatial Planning (MSP) legislation. The underlying legal framework supports the achievement of ecological, social and economic objectives, but a national policy to fast track the oceans economy provides a challenge for ecosystem-based approaches to MSP. During the 2018 International Marine Conservation Congress, we convened a session to present particular challenges that will likely apply to any developing country seeking to increase profits from existing, or proposed, marine activities. Here we present six multi-disciplinary research projects that support ecosystem-based approaches to MSP in South Africa, by addressing the following knowledge gaps and specific key challenges: (1) the lack of data-derived measurements of ecosystem condition (and the need to validate commonly-used proxy measures); (2) the need to develop models to better understand the potential impacts of climate change on food webs and fisheries; (3) the slow implementation of an ecosystem approach to fisheries management, and the need to implement existing legal instruments that can support such an approach; (4) the paucity of evidence supporting dynamic ocean management strategies; (5) the requirement to manage conflicting objectives in growing marine tourism industries; and (6) the need to adopt systems thinking approaches to support integrated ocean management. We provide examples of specific research projects designed to address these challenges. The ultimate goal of this research is to advance a more integrated approach to ocean management in South Africa, using tools that can be applied in countries with similar socio-political and environmental contexts.
Introduction
South Africa’s large exclusive economic zone includes the Indian, Atlantic, and Southern Oceans. Management of this ocean space has traditionally been undertaken within sectors, leading to conflict amongst sectors, and between commercial and environmental interests. As the demand for ocean space and marine resources increases, in response to an economic growth imperative defined by the National Planning Commission [NPC] (2012), a more integrated approach to management is required to ensure that both ecological and socio-economic objectives are met. Marine spatial planning (MSP) has emerged in many countries as the preferred process to achieve this integration, and in 2017, South Africa became the first African country to draft MSP legislation (Marine Spatial Planning Bill [B9-2017], 2017). In December 2018, the Bill was passed by both the South Africa National Assembly and National Council of Provinces and sent to the President to be signed into law. The underlying legal framework of the Bill supports the achievement of ecological, social and economic objectives, but a national policy to grow the oceans economy (Operation Phakisa)1 provides a challenge for mainstreaming ecosystem-based approaches to MSP into policy and decision making. The adoption of ecosystem-based approaches to MSP is globally endorsed (Ehler, 2008; Gilliland and Laffoley, 2008; Halpern et al., 2008; Foley et al., 2010; Westholm, 2018; Kirkman et al., 2019), and embodied in the United Nations Sustainable Development Goals, particularly Goal 14 (United Nations, 2015), as well as the Nairobi Convention (United Nations, 2010) of which South Africa is a contracting party. An ecosystem-based approach to MSP is founded on ecosystem health, whereas an integrated-use approach to MSP is underpinned by economic growth (Qiu and Jones, 2013).
Global economic powers are currently interested in promoting East Africa’s oceans economy via programmes such as China’s One Belt One Road Initiative and Japan and India’s Asia Africa Growth Corridor (Buys, 2018). South Africa’s current plans to grow its oceans economy have created high expectations amongst many stakeholders, and in response to this socio-political context, we have developed a research agenda to address key challenges in the country’s emerging MSP process (Table 1). From our perspective, as marine scientists, the most urgent research challenges to address fall within three broad themes. First is a critical need for an improved understanding of the cumulative impacts of human activities (including climate change) on marine ecosystem structure and function. Existing activities are intensifying, and new activities are emerging (e.g., seabed mining) and we do not know how these will impact ecosystem health or the delivery of ecosystem services. Second is the need for interdisciplinary research to inform and support integrated ocean management, including management strategies that are dynamic in space and time and can measure trade-offs between competing human activities (see, for example, Harris and Lombard, 2018). Third is the requirement for the development of robust scenario-planning tools to aid multi-sector decision making and manage conflict. We believe these challenges are applicable in all socio-economic contexts, particularly in least-developed countries with strong and urgent economic growth imperatives. We recognize that many additional challenges exist and our aim is not to be comprehensive, but merely to share our specific approaches to these challenges. Our research agenda aims to support sustainable MSP practices that do not transcend environmental tipping points or safe operating spaces for human well-being (Rockström et al., 2009; Barfuss et al., 2018). Here we describe seven research projects that specifically address components of these three broad themes, and discuss the potential of our preliminary results to advance an ecosystem-based approach to MSP.
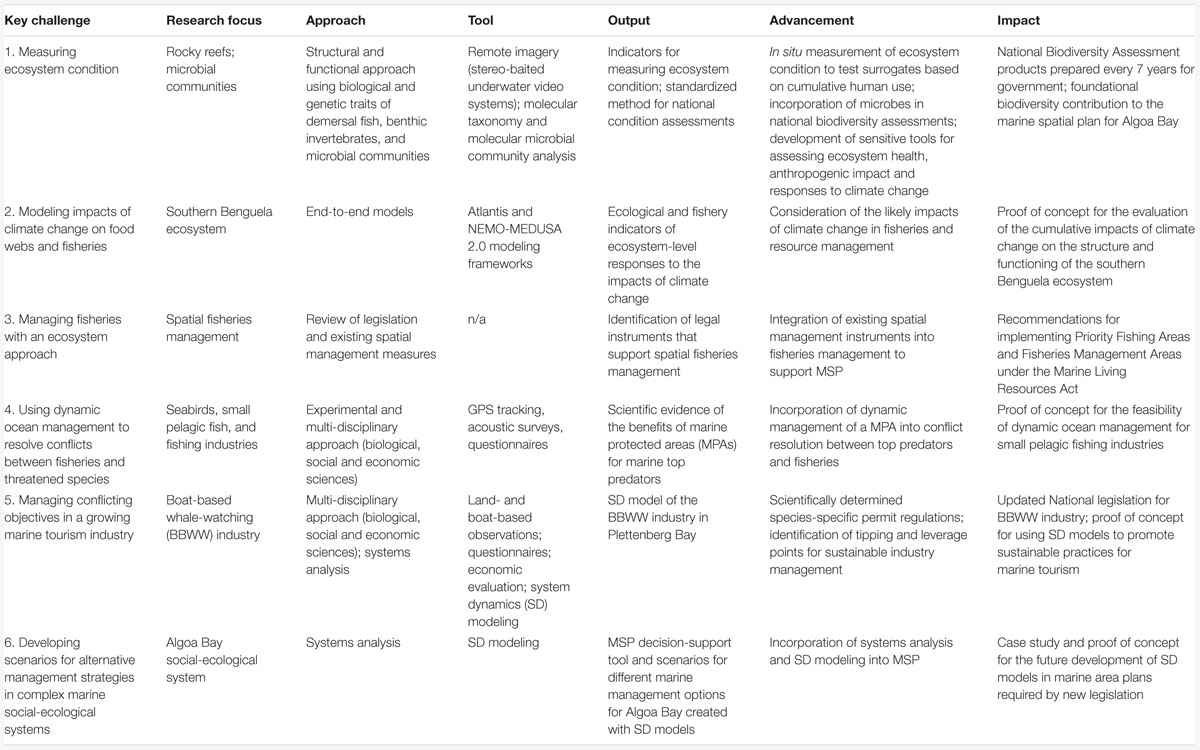
Table 1. A summary of six research projects designed to address key challenges in advancing an ecosystem-based approach to marine spatial planning (MSP) under economic growth imperatives in South Africa.
Measuring Ecosystem Condition
An ecosystem-based approach to MSP is predicated on the principle of achieving good ecological status for oceans (WFD, 2000). However, in situ measurements of ecosystem condition at broad scales remains a global knowledge gap as does a better understanding of the cumulative impact of human uses on ocean ecosystems (Borja, 2014; Ehler et al., 2019). Under Operation Phakisa, South Africa’s national interest in growing its oceans economy is outpacing the development and application of robust tools to measure marine ecosystem condition. Most important is the absence of a standardized data-derived approach. This poses challenges for national assessments, for example, the National Biodiversity Assessments (NBAs) that are conducted approximately every 7 years2 and contribute to the environmental monitoring requirements of national government. The NBA currently relies on a proxy of cumulative human impacts to infer good, fair or poor ecosystem condition at a national scale, creating the need for fine-scale ecosystem-based condition assessments using ecological data (Sink et al., 2012a).
We are presently engaged in a benthic research project designed to validate the current proxy method. Potential indicators of ecosystem condition have been identified using demersal fish and benthic invertebrate data from rocky reef ecosystems on South Africa’s east coast. This project also aims to improve the understanding of the effects of cumulative anthropogenic pressures on the structure and function of marine ecosystems. Species composition and abundance and species biological traits are being assessed to determine the functional structure of the ecosystem. Traits were chosen to represent the trophic ecology and life history of the community (for example, fish biological traits included maximum depth, maximum length, trophic level, longevity, reproductive mode and guild, gregariousness, feeding guild, habitat preference, water column position, activity, and mobility).
This benthic study tests a suite of structural (species richness, Shannon Weiner diversity, total abundance, and biomass) and functional metrics (average and proportion of biological traits and community metrics of functional richness, functional evenness, functional diversity, functional specialization, functional originality, and Rao’s quadratic entropy). Study sites are distributed along a cumulative pressure gradient, using the Pondoland no-take marine protected area (MPA) to provide a reference for good condition rocky reef ecosystems. Using fish data collected from stereo baited underwater video systems, we have been able to show that structural metrics are not sensitive to changes in cumulative pressures. In contrast, functional evenness, functional originality, mean and maximum size, mean trophic level, mean longevity and proportion of higher carnivores show a significant decline in response to increasing cumulative pressures. Multivariate analyses also identify which traits are most vulnerable to cumulative pressures, based on site differences between the no-take MPA and heavily exploited localities. These results emphasize the need to assess both the structural and functional response of biological communities to human pressures and have identified initial indicators that can be used to measure the condition of other rocky reef ecosystems nationally. The study thus provides the first validation of the proxy method used to generate national maps of marine ecosystem condition in the NBA.
In addition to broad-scale biodiversity assessments such as the NBA, there is a need for continuous monitoring of ecologically and economically important habitats that can provide early warning signs of threats to ecosystem functioning. Microorganisms account for up to 70% of the total biomass of the oceans (Bar-On et al., 2018), and play a critical role in driving global biogeochemical cycles (Azam et al., 1983). Community analysis of microbial biomass reveals extraordinary taxonomic diversity with distinct communities in different water masses (Sunagawa et al., 2015; Venkatachalam et al., 2019). Their diversity is reflected by metabolic versatility that allows marine microorganisms to respond rapidly to changes in their physical and chemical environment, including anthropogenically-driven change (Fuhrman et al., 2015). These responses, including shifts in the diversity and structure of microbial communities and their metabolic activity, can be used as a sensitive tool for assessing ecosystem health, anthropogenic impact and responses to climate change (Matcher et al., 2011).
We have employed this approach in a comparative study of the microbial communities of three estuaries, two of which are impacted by human activity, in Algoa Bay on the southeast coast of South Africa. The study showed conservation of the microbial communities of the marine-dominated compared with the freshwater-dominated estuaries and a drop in bacterial species richness in the human impacted systems. In addition, there was a significant difference in the response to the impact of agricultural versus urban activity in the river catchments and in the estuaries (Matcher et al., 2018). We are using this bottom-up approach to characterize the pelagic and benthic microbial communities of Algoa Bay, including the Sundays and Swartkops River estuaries. The data provide insight into macro- and mesoscale variability that reflects the complexity of freshwater and marine influences and anthropogenic impact with important implications for the development of a marine spatial plan for the Bay (see Dorrington et al., 2018). This local-area plan can inform the broader Marine Area Plans required by South Africa’s emerging MSP legislation.
Modeling Impacts of Climate Change on Food Webs and Fisheries
Climate change is a globally-recognized threat to marine fisheries (Cochrane et al., 2009), and of the seven broad categories of human use of the ocean, fisheries are considered to be the most vulnerable to a range of both primary and secondary drivers of climate change (Santos et al., 2016). Within South Africa, a trait-based assessment of the likely sensitivity of South African species to climate change identified that endemic and/or species with a threatened or depleted stock status ranked among the most sensitive to climate change (Ortega-Cisneros et al., 2018b). Potts et al. (2015) identified a knowledge gap regarding the predicted impacts of climate change on coastal fishes, and range shifts in economically significant species (Fairweather et al., 2006; Blamey and Branch, 2012) could be indicators of the impacts of climate change, but significant new knowledge will be required to determine if this is indeed the case. The South African National Department of Agriculture, Forestry and Fisheries (DAFF) is developing a draft Climate Change Mitigation and Adaptation Plan for marine fisheries and aquaculture. We have responded to this knowledge gap by developing models of the potential impacts of climate change on food webs.
Climate projections indicate that an increase in sea surface temperature (SST) in South African waters of approximately 3°C is expected by 2099 compared to SST observed in 2000 under the Representative Concentration Pathways (RCP) 8.5 emission scenario of the Intergovernmental Panel on Climate Change (Popova et al., 2016). Early signs of surface and shelf bottom water aragonite undersaturation are also expected by the end of the century in South Africa (Popova et al., 2016), however, this can be observed earlier in areas influenced by upwelling such as the west coast of South Africa (Gruber et al., 2012). Anthropogenic climate change (e.g., ocean warming) has been shown to have a wide range of effects on marine organisms (e.g., Edwards and Richardson, 2004; Hoegh-Guldberg et al., 2010) with consequences on catch potential (e.g., Hays et al., 2005; Cheung et al., 2011) and livelihoods (Barange et al., 2014; Pecl et al., 2017). Furthermore, sensitivity to acidification is intensified when taxa are simultaneously exposed to increased seawater temperature (Kroeker et al., 2013; Nagelkerken and Connell, 2015). The warming and acidification forecast for South Africa can be expected to have profound effects on a number of species and potentially on whole ecosystems.
Climate projections from the coupled “NEMO – MEDUSA 2.0” model (Yool et al., 2013) under the RCP 8.5 emission scenario and the “Atlantis on the Benguela and Agulhas Currents” model (ABACuS v2, Ortega-Cisneros et al., 2017) were used to simulate the effects of ocean acidification and warming in the southern Benguela system. Model simulations represent the period 1990 to 2050. The individual and combined effects of ocean acidification and warming were compared to the control run (no fishing, warming or acidification pressures) to determine the effect of these stressors on groups’ biomass in the southern Benguela. In the simulations, because of the limited number of studies evaluating the effects of acidification on invertebrate and fish species in South Africa, acidification is assumed to affect the mortality of phyto- and zooplankton groups only. Other invertebrate groups such as macrobenthos, meiobenthos and squid as well as fish groups were assumed not to be affected by acidification.
The combined effects of warming and acidification resulted in biomass reductions for most plankton and fish groups. Several target species such as anchovy Engraulis encrasicolus, deep-water hake Merluccius paradoxus and shallow-water hake M. capensis, snoek Thyrsites atun and sardine Sardinops sagax were also negatively affected by the combined effect of warming and acidification. The observed impacts of acidification on most model groups were explained by indirect effects since acidification affected only plankton groups in our simulations. However, the impacts of warming were mostly attributed to direct effects on the consumption, growth, mortality and reproduction of model groups (Ortega-Cisneros et al., 2018a).
This study has provided insight into the potential responses of marine species to climate change in South Africa. Our results suggest that several important target species are likely to be affected by climate change, which will potentially have repercussions on the country’s economy. Our study also identified information gaps and research priorities, which is important in countries where resources and available information are limited. The consideration of the likely future impacts of ocean acidification and warming, and its combined effects with other anthropogenic stressors, is essential to better inform resource management and planning in South Africa, especially in the currently-emerging MSP processes that will need to remain adaptive to the spatial uncertainties resulting from climate change.
Managing Fisheries With an Ecosystem Approach
In 1998, an ecosystem-based fisheries management (EBFM) approach was adopted in South Africa with the enactment of the Marine Living Resources Act 18 of 1998 (MLRA). Subsequently, some ecosystem-based measures were incorporated into the permit conditions of most commercial fisheries sectors, but progress with implementation has been slow. While an EBFM approach is implicitly considered in scientific and management working groups, the implementation of explicit measures remains to be demonstrated (Hutchings et al., 2009; Petersen et al., 2010). A further deficiency in the implementation of EBFM in South Africa is the incorporation of spatial management instruments. Some spatial and temporal measures are in place, however, the rationale for the existence of many of these measures has not been explicitly stipulated and there have been calls to review and improve such measures (Shannon et al., 2006; Sink et al., 2012b). There are also concerns that expanding and diversifying mining interests may compromise the food and job security provided by fisheries (Norman et al., 2018).
The sustainable management of natural resources requires an understanding of both the spatial and temporal scales over which the social-ecological system operates (Ostrom, 2009). Although many temporal measures exist to manage fisheries globally, the spatial nature of ecosystems, natural resources and human activities intuitively requires that management measures should incorporate spatial strategies (Crowder et al., 2008). Spatial aspects of fisheries that may benefit from area-based management measures include stock structure (Reiss et al., 2009) and catch distributions (Rassweiler et al., 2012), key areas for life history stages (Fisher and Frank, 2002) (e.g., areas for spawning, nursery, migration, life history cues), bycatch hotspots (Witherell and Pautzke, 1997), and user conflict (Kaiser et al., 2000). The EBFM approach is fundamentally a spatially-explicit approach, and ocean zoning and MSP form a crucial part of this concept (Pikitch et al., 2004).
In order for South African fisheries management to fully adopt an ecosystem-based approach, the full range of potential spatial management instruments needs to be identified and considered for implementation. We have investigated spatial management options by: (i) reviewing the existing legal environment that supports spatial ocean management in South Africa; (ii) identifying potential legal instruments that are or may be implemented to support spatial fisheries management; (iii) exploring the manner in which these instruments may be implemented; and (iv) providing research recommendations to improve integration of the spatial management instruments into fisheries management. Eight Acts and Bills were examined, including the National Environmental Management Act 107 of 1998 (NEMA), National Environmental Management: Integrated Coastal Management Act 24 of 2008 (NEM:ICMA), National Environmental Management: Protected Areas Act 57 of 2003 (NEM:PAA), National Environmental Management: Biodiversity Act 10 of 2004 (NEM:BA), the Mineral and Petroleum Resources Development Act 28 of 2002 (MPRDA), the Marine Living Resources Act 18 of 1998 (MLRA), the Aquaculture Development Bill B 22-2018 and the Marine Spatial Planning Bill B 9D-2017.
From the Acts and Bills examined, 11 spatial legal instruments have been identified that can be used to improve area-based management in the ocean, including measures that could be used to implement MSP. Results show that nine instruments support area-based management of South Africa’s fisheries, however, only two have been implemented. Collectively, these instruments represent opportunities to improve spatial fisheries management, for example, spatial measures currently implemented through permit conditions may be formalized through the implementation of legal instruments. Some existing spatial closures could be considered or strengthened as Other Effective Area Based Conservation Measures (OECMs) recognized by the Convention on Biological Diversity. With the elevation of the MSP Bill to the Presidency for enactment, there is an incentive to formalize spatial management measures to facilitate the MSP process. In particular, it is recommended that the identification and implementation of Fisheries Management Areas and Priority Fishing Areas under the MLRA be prioritized to support an EBFM approach to fisheries, and the development of an ecosystem-based approach to MSP.
Using Dynamic Ocean Management to Resolve Conflicts Between Fisheries and Endangered Penguins
No-take zones can be important tools within an ecosystem-based approach to achieve sustainable fishing and re-establish ecosystem integrity (Roberts et al., 2005). However, their potential benefits for vagile species such as small pelagic fish and top predators remain questionable. In addition, they are generally designed with definite sizes and shapes, although these can be dynamically managed in space or time. For example, high-risk areas for bycatch of yellowtail flounders Limanda ferruginea by a New England scallop fishery are identified and updated daily, which allows the lifting of the closed seasons previously in place, thereby adding $10 million to the fishery each year (O’Keefe and DeCelles, 2013). Maxwell et al. (2015) provide additional examples of successful dynamic ocean management measures.
In South Africa, the population of the endemic African penguin Spheniscus demersus has halved since 2004 (Crawford et al., 2011). They predominantly feed on sardines and anchovies which are also the target species of the purse-seine commercial fishery. Since 2008, a 20 km radius experimental purse-seine fishing exclusion has been initiated around two pairs of penguin colonies (Dassen and Robben islands on the West Coast, and St Croix and Bird islands in Algoa Bay on the East Coast, the latter supporting half of the global population) with alternating closure regimes in 3 years cycles (e.g., Pichegru et al., 2012; Sherley et al., 2018). Between 2012 and 2017, in addition to collecting information on the foraging performance and reproductive success of penguins in Algoa Bay, we conducted small-scale acoustic surveys to determine the relative abundance of pelagic fish around their colonies (see McInnes et al., 2015). We related these to the fishing exclusion patterns, while controlling for monthly environmental conditions. We also compared fishing patterns (costs in terms of travel time and benefits in terms of size of landings) during and outside closures to estimate the potential socio-economic impact of no-take zones to the industry.
The results show that the costs of the closure remained low to the fishing industry and catches remained stable through time, despite variability in fish abundance. By contrast, fishing exclusions largely benefitted penguins in terms of breeding success and foraging performance, particularly during periods of poor environmental conditions. Consequently, interviews were conducted with stakeholders in the fishing industry, initiating a discussion platform between fishers and scientists, to explore if a dynamically-managed, adjustable no-take zone in Algoa Bay can better protect penguin food sources, while still considering fisher economies (Ginsburg et al., 2018). Monthly acoustic surveys assessing fish abundance could be used to suggest size and timing of fishing exclusions, thereby allowing fishers additional operational areas when fish stocks are high. Our research provides a proof of concept for spatially and temporally dynamic management practices, as well as the co-development of these practices with resource-dependent stakeholders. Given that the current MSP process in South Africa lacks a platform for meaningful engagement with civil society (Reed and Lombard, 2017), our research outputs provide an encouraging example of the potential for win-win outcomes that can be achieved with purposeful stakeholder consultation.
Managing Conflicting Objectives in a Growing Marine Tourism Industry
Coastal and marine tourism have the potential to make an important contribution to the blue economy. Boat-based whale-watching (BBWW) has become one of the fastest growing marine tourism industries worldwide, benefitting many communities and countries, and affecting many nearshore cetacean populations (O’Connor et al., 2009). BBWW was initially viewed as a sustainable and non-consumptive use of cetaceans (Allen, 2014), and a profitable replacement for commercial whaling (Hoyt and Hvenegaard, 2002). More recent research has shown the potential for BBWW to have extensive negative impacts, including local extinctions (Lusseau et al., 2006; Parsons, 2012; Senigaglia et al., 2016). In the case of tourism practices that rely on human-wildlife encounters, long-term sustainability requires the continued presence of wildlife, and ethical codes of conduct require empirical evidence to set specific standards.
The Indian Ocean Rim Association (IORA) recognizes the vital contribution of marine tourism to the blue economy (see Rogerson et al., 2018). South Africa, currently serving as the IORA chair, is at the forefront of promoting the inclusion of marginalized groups in marine tourism, with a focus on BBWW. The South African BBWW industry was formalized in 1998, requiring permits to operate under a framework of regulations and a voluntary code of conduct. The Department of Environmental Affairs (DEA) is responsible for administering the permits and enforcing the regulations. Globally, the South African BBWW industry is perceived as one of the most sustainable of its kind, owing to the strong scientific basis from which the regulations and code of conduct were designed. However, an assessment of the efficacy of these regulations to protect all targeted species has not been done and species-specific regulations may be needed for the more vulnerable and less conspicuous populations e.g., the Endangered humpback dolphin (Sousa plumbea) and Vulnerable inshore Bryde’s whale (Balaenoptera edeni) (Penry et al., 2016; Plön et al., 2016). Additionally, increasing pressure to expand the industry for economic growth and social upliftment under Operation Phakisa, requires a thorough understanding of the resource value, it’s socio-economic potential, and potential impacts on target species.
In 2005, an economic assessment by Turpie et al. (2005) established that the BBWW industry contributed approximately R37 million to South Africa’s Gross Domestic Product annually. Their report supported the growth of the industry based on the potential economic benefits, suggesting that the number of operators could be increased by up to 40% “in the near future.” Since then studies on the growth and subsequent re-evaluation of the economic importance of this industry to South Africa have been lacking. Although the socio-economic benefits of whale and dolphin tourism and growth of the industry are apparent, with an increase from 20 to 40 available permits between 1999 and 2017 (Department of Environmental Affairs [DEA], 2017a,b), the benefits must be considered in light of the dependence of the industry on the availability of a resource in the form of regular and reliable sightings of whales and dolphins. This increase was based on the recognition of the potential economic and social benefits of the industry, but with little or no consideration of the ecological factors on which the industry is dependent. Sustainable BBWW depends on continuous monitoring of the impacts on cetaceans, compliance with enforceable regulations, commercial profitability, and tourist satisfaction (Hoyt, 2003; Corkeron, 2006).
A multi-disciplinary team is conducting research in Plettenberg Bay on the South coast of South Africa, to assess the current status of, and develop sustainable practices for, boat-based marine tourism. The strategic intent of the program is to support Operation Phakisa’s initiative to promote the development of a sustainable oceans economy. The objectives are to: measure the behavioral responses of whales and dolphins to vessel approaches; assess the effectiveness of existing guidelines to mitigate potential negative impacts; determine rates of permit regulation transgression; quantify the direct and indirect economic value of the industry; and determine levels of customer satisfaction in relation to their perceptions and expectations based on marketing of the industry. The overall goal is to develop a sustainable marine tourism sector with minimal impact on the resource that it depends upon. We are using a systems analysis approach with system dynamics (SD) modeling tools to identify tipping points at which the industry becomes economically or ecologically unsustainable, and leverage points where management or policy interventions could move the system onto a sustainable path. SD models allow diverse stakeholders to contribute both qualitative and quantitative information, and to see the impact of different decisions on their variables of interest (e.g., annual earnings or whale population numbers). The Plettenberg Bay project is providing a platform for future group model-building exercises in a multi-stakeholder environment, drawing on biophysical and socio-economic research methods. From our perspective, multi-disciplinary research projects of this nature have the highest likelihood of success in the management of conflicting objectives in a growing oceans economy.
Developing Scenarios for Alternative Management Strategies in Complex Marine Social-Ecological Systems
Marine environments and marine governance structures are both complex systems, and management strategies often fail to encompass this complexity (Hazen et al., 2018; Lewison et al., 2018; Lombard et al., in press). Integrated management approaches should therefore supplement sector-specific approaches and should adopt methods of analysis and decision-support tools that recognize multiple forms of complexity (Douvere, 2008; Lombard et al., 2019). There is a growing interest in complexity research globally, given the increasing impacts of humans on the biosphere (Steffen et al., 2015), and MSP processes can benefit from a paradigm shift from linear management approaches, to more adaptive approaches that consider uncertainties, feedbacks, and plausible future scenarios. Systems analysis, an approach that addresses complexity, can provide a framework to understand key dynamic interactions, feedbacks and unintended consequences across multiple sectors (Pongsiri et al., 2017) and is therefore well suited to MSP (Wang et al., 2014; Boumans et al., 2015). SD modeling, a structured approach to systems analysis, is a rigorous method for modeling complex systems and building computer simulations which can assist with understanding the behavior of systems under different conditions and future scenarios (Sterman, 2000; Ford, 2009; Deenapanray and Bassi, 2015). SD models can integrate environmental, social and economic components within the marine system and therefore provide holistic decision-support to understand the varying levels of complexity (Deenapanray and Bassi, 2015).
Weller et al. (2014, 2016) used SD models to better understand the impacts of multiple drivers on endangered African penguin populations on two islands in South Africa. Their model scenarios identified which management strategies were likely to provide the most benefit for penguin populations. We are using a broadly similar modeling approach to develop scenarios for multiple sectors in Algoa Bay, South Africa, and how these sectors will (1) be impacted by external drivers (e.g., harmful algal blooms, range shifts in commercial species) and (2) impact one another as they respond to external drivers (e.g., an increase in fishing effort for small pelagic species may impact top predators reliant on the same species). SD models will be developed with stakeholders to allow them to identify which mitigation and adaptation strategies will most likely reduce their sector’s vulnerability to the negative impacts of external drivers, while also minimizing any negative interactions among sectors.
Our particular study forms part of a broader programme to develop a marine spatial plan for Algoa Bay (Dorrington et al., 2018). Given that the focus of MSP is inherently spatial, and that SD models provide temporal outputs (in the form of behavior-over-time graphs), we are developing methods to “soft-couple” spatial and temporal outputs in a single decision-making framework. Soft coupling provides a trade-off between complexity and tractability, and our intention is to provide a proof of concept for SD modeling as a tool for MSP in least developed countries (that do not have the resources for fully integrated model coupling).
From our perspective, systems-based approaches can support more efficient planning and implementation processes and help to optimize policy interventions by identifying trade-offs and consequences related to different management strategies (White et al., 2012; Lester et al., 2013; Pongsiri et al., 2017). Adopting a complex systems analysis approach to MSP in South Africa could therefore provide a realistic research lens and could assist in informed and coordinated decision-making about the future management of the country’s marine environment, aiming to ensure the rational exploitation of its marine resources and facilitate sustainable development of the marine economy.
Conclusion
Through its National Development Plan and Operation Phakisa, South Africa has a strong commitment to grow its oceans economy. At the same time, the country’s Marine Spatial Planning Bill is based on the principles of ecosystem-based MSP, as opposed to integrated-use MSP. Careful navigation will be required to ensure that short-term gains in one sector will not outweigh concerns about long-term sustainability in other sectors, and that all sectors will have equal power at the negotiating table. As marine scientists, we need to identify what sort of scientific information will be most useful, and have most traction, at the policy level. To this end, we have adopted a multi-disciplinary approach to address what we believe are the key challenges in advancing an ecosystem-based approach to MSP in a political climate of economic growth imperatives.
The outputs of projects described in this manuscript are designed to assist at the ecosystem assessment and monitoring level (with data-derived methods to measure ecosystem condition), the scenario planning and stakeholder engagement level (with climate, ecosystem, and SD models), the management level (particularly of resources that require dynamic management), and the policy level (by developing evidence-based codes of conduct for tourism, and informing and applying existing legal instruments for spatial fisheries management). Ultimately, the hope is to advance a more integrated and adaptive approach to ocean management in South Africa, using tools that can be applied in countries with similar socio-political and environmental contexts. Recognizing that our research projects address only some of the many challenges in ecosystem-based approaches to MSP, we are continuing to develop new programmes to address additional challenges (e.g., the impact of seabed mining on fisheries, and the impact of ocean noise on ecosystem and species). Nonetheless, we hope that we can add to the global research knowledge base, and other MSP processes, with the lessons we have learned thus far.
Data Availability
The datasets for this study will not be made publicly available because the data are part of students theses. The theses will be published online in due course.
Author Contributions
AL conceptualized idea for research, wrote manuscript with case study inputs from co-authors, post-graduate supervisor of five of the co-authors, post-doctoral host of two co-authors. RD, KO-C, GP, LP, JR, KPS, EV, MW, and KJS wrote a subsection of the manuscript. TG and AM conducted analyses for a subsection of the manuscript. All authors provided final editorial input.
Conflict of Interest Statement
The authors declare that the research was conducted in the absence of any commercial or financial relationships that could be construed as a potential conflict of interest.
Acknowledgments
We thank the Marine section of the Society for Conservation Biology for convening the Fifth International Marine Conservation Congress in Kuching, Borneo, Malaysia, at which we presented the case studies described here. We acknowledge the Department of Science and Technology (DST) and the National Research Foundation (NRF) of South Africa for financial support to attend the Congress. This research was funded by a NRF SARChI Communities of Practice Grant to RD (Principal Investigator) and AL (UID: 110612). GP and MW acknowledge the Worldwide Fund for Nature (South Africa) Nedbank Green Trust for project funding, the DEA for permit approval and endorsement of the project (Permit numbers 2018/63, 2019/57), and the Nature’s Valley Trust for administrative and logistic support for the Marine Tourism Sustainability team. GP acknowledges the NRF and Nelson Mandela University for a postdoctoral bursary, MW acknowledges the NRF for a postgraduate bursary, and KO-C acknowledges support from the Claude Leon Foundation through a postdoctoral fellowship as well as support and resources from the Cluster for High Performance Computing at the Council for Scientific and Industrial Research. KPS acknowledges the South African Institute for Aquatic Biodiversity and the African Coelacanth Ecosystem Programme for equipment and co-funding. KJS acknowledges the Pew Charitable Trusts and the Foundational Biodiversity Information Program of the NRF. Opinions expressed and conclusions arrived at are those of the authors and are not attributed to any of the above-mentioned donors.
Footnotes
References
Allen, S. J. (2014). “From exploitation to adoration: the historical and contemporary contexts of human-cetacean interactions,” in Whale-Watching: Sustainable Tourism and Ecological Management, eds J. E. S. Higham, L. Bejder, and R. Williams (Cambridge: Cambridge University Press), 31–47.
Azam, F., Fenchel, T., Field, J. G., Gray, J. S., Meyer-Reil, L. A., and Thingstad, F. (1983). The ecological role of water-column microbes in the sea. Mar. Ecol. Prog. Ser. 10, 257–263. doi: 10.3354/meps010257
Barange, M., Merino, G., Blanchard, J. L., Scholtens, J., Harle, J., Allison, E. H., et al. (2014). Impacts of climate change on marine ecosystem production in societies dependent on fisheries. Nat. Clim. Chang. 4, 211–216. doi: 10.1111/gcb.14512
Barfuss, W., Donges, J. F., Lade, S. J., and Kurths, J. (2018). When optimization for governing human-environment tipping elements is neither sustainable nor safe. Nat. Commun. 9:2354. doi: 10.1038/s41467-018-04738-z
Bar-On, Y. M., Phillips, R., and Milo, R. (2018). The biomass distribution on Earth. Proc. Natl. Acad. Sci. U.S.A. 115, 6506–6511. doi: 10.1073/pnas.1711842115
Blamey, L. K., and Branch, G. M. (2012). Regime shift of a kelp-forest benthic community induced by an ‘invasion’of the rock lobster Jasus lalandii. J. Exp. Mar. Biol. Ecol. 420, 33–47. doi: 10.1016/j.jembe.2012.03.022
Borja, A. (2014). Grand challenges in marine ecosystems ecology. Front. Mar. Sci. 12:1. doi: 10.3389/fmars.2014.00001
Boumans, R., Roman, J., Altman, I., and Kaufman, L. (2015). The Multiscale Integrated Model of Ecosystem Services (MIMES): simulating the interactions of coupled human and natural systems. Ecosyst. Serv. 12, 30–41. doi: 10.1016/j.ecoser.2015.01.004
Buys, A. (2018). China, Japan, India and the East Africa Blue Economy. Policy Insights No 61. Johannesburg: South African Institute of International Affairs.
Cheung, W. W. L., Dunne, J., Sarmiento, J. L., and Pauly, D. (2011). Integrating ecophysiology and plankton dynamics into projected maximum fisheries catch potential under climate change in the Northeast Atlantic. ICES J. Mar. Sci. 68, 1008–1018. doi: 10.1093/icesjms/fsr012
Cochrane, K., De Young, C., Soto, D., and Bahri, T. (2009). Climate change implications for fisheries and aquaculture. FAO Fish. Aquac. Tech. Pap. 530:212.
Corkeron, P. J. (2006). How Shall We Watch Whales. In D. M. Lavigne (ed.) Gaining Ground: In Pursuit of ecological Sustainability. Proceedings of an International Forum. Guelph, ON: International Fund for Animal Welfare, 161–170.
Crawford, R. J. M., Altwegg, R., Barham, B. J., Barham, P. J., Durant, J. M., Dyer, B. M., et al. (2011). Collapse of South Africa’s penguins in the early 21st century: a consideration of food availability. Afr. J. Mar. Sci. 33, 139–156. doi: 10.2989/1814232X.2011.572377
Crowder, L. B., Hazen, E. L., Avissar, N., Bjorkland, R., Latanich, C., and Ogburn, M. B. (2008). The Impacts of fisheries on marine ecosystems and the transition to ecosystem-based management. Annu. Rev. Ecol. Evol. Syst. 39, 259–278. doi: 10.1146/annurev.ecolsys.39.110707.173406
Deenapanray, P. N. K., and Bassi, A. M. (2015). System dynamics modelling of the power sector in mauritius. Environ. Clim. Technol. 16, 20–35. doi: 10.1515/rtuect-2015-0010
Department of Environmental Affairs [DEA] (2017a). Invitation to Apply for Permits. Available at: https://www.environment.gov.za/sites/default/files/docs/bbww_wscd_invitationnotice.pdf
Department of Environmental Affairs [DEA] (2017b). Policy on Boat-Based. (Whale) and Dolphin Watching. Available at: http://extwprlegs1.fao.org/docs/pdf/saf167497.pdf
Dorrington, R. A., Lombard, A. T., Bornman, T. G., Adams, J. B., Cawthra, H. C., Deyzel, S., et al. (2018). Working together for our oceans: a marine spatial plan for Algoa Bay, South Africa. South Afr. J. Sci. 114:6. doi: 10.17159/sajs.2018/a0247
Douvere, F. (2008). The importance of marine spatial planning in advancing ecosystem-based sea use management. Mar. Policy 32, 762–771. doi: 10.1016/j.marpol.2008.03.021
Edwards, M., and Richardson, A. J. (2004). Impact of climate change on marine pelagic phenology and trophic mismatch. Nature 430, 881–884. doi: 10.1038/nature02808
Ehler, C. (2008). Conclusions: benefits, lessons learned, and future challenges of marine spatial planning. Mar. Policy 32, 840–843. doi: 10.1016/j.marpol.2008.03.014
Ehler, C., Zaucha, J., and Gee, K. (2019). “Maritime/marine spatial planning at the interface of research and practice,” in Maritime Spatial Planning, eds J. Zaucha and K. Gee (Cham: Palgrave Macmillan), 1–22.
Fairweather, T. P., Van Der Lingen, C. D., Booth, A. J., Drapeau, L., and Van Der Westhuizen, J. J. (2006). Indicators of sustainable fishing for South African sardine Sardinops sagax and anchovy Engraulis encrasicolus. Afr. J. Mar. Sci. 28, 661–680. doi: 10.2989/18142320609504215
Fisher, J. A. D., and Frank, K. T. (2002). Changes in finfish community structure associated with an offshore fishery closed area on the Scotian Shelf. Mar. Ecol. Prog. Ser. 240, 249–265. doi: 10.3354/meps240249
Foley, M. M., Halpern, B. S., Micheli, F., Armsby, M. H., Caldwell, M. R., Crain, C. M., et al. (2010). Guiding ecological principles for marine spatial planning. Mar. Policy 34, 955–966. doi: 10.1002/eap.1469
Fuhrman, J. A., Cram, J. A., and Needham, D. M. (2015). Marine microbial community dynamics and their ecological interpretation. Nat. Rev. Microbiol. 13, 133–146. doi: 10.1038/nrmicro3417
Gilliland, P. M., and Laffoley, D. (2008). Key elements and steps in the process of developing ecosystem-based marine spatial planning. Mar. Policy 32, 787–796. doi: 10.1016/j.marpol.2008.03.022
Ginsburg, T., Pichegru, L., and Lombard, A. T. (2018). Plunging into new waters: investigating a novel marine protected area management plan in South Africa. SANCOR. Newsletter 220, 1–3.
Gruber, N., Hauri, C., Lachkar, Z., Loher, D., Frölicher, T. L., and Plattner, G.-K. (2012). Rapid progression of ocean acidification in the california current system. Science 337, 220–223. doi: 10.1126/science.1216773
Halpern, B. S., McLeod, K. L., Rosenberg, A. A., and Crowder, L. B. (2008). Managing for cumulative impacts in ecosystem-based management through ocean zoning. Ocean Coast. Manage. 51, 203–211. doi: 10.1111/j.1749-6632.2009.04496.x
Harris, J., and Lombard, A. T. (2018). Socio-Ecological Resilience in South Africa’s Blue Economy: The Role of Marine Protected Areas. Policy Briefing No 176. Johannesburg: South African Institute of International Affairs.
Hays, G. C., Richardson, A. J., and Robinson, C. (2005). Climate change and marine plankton. Trends Ecol. Evol. 20, 337–344. doi: 10.1016/j.tree.2005.03.004
Hazen, E. L., Scales, K. L., Maxwell, S. M., Briscoe, D. K., Welch, H., Bograd, S. J., et al. (2018). A dynamic ocean management tool to reduce bycatch and support sustainable fisheries. Sci. Adv. 4:eaar3001. doi: 10.1126/sciadv.aar3001
Hoegh-Guldberg, O., Bruno, J. F., Bounama, C., Bloh, W., von Franck, S., Rosenzweig, C., et al. (2010). The impact of climate change on the world’s marine ecosystems. Science 328, 1523–1528. doi: 10.1126/science.1189930
Hoyt, E. (2003). “Toward a new ethic for watching dolphins and whales,” in Between species: Celebrating the Dolphin-Human Bond, eds T. Frohoff and B. Peterson (San Fransisco, CA: Sierra Club Books), 168–177.
Hoyt, E., and Hvenegaard, G. T. (2002). A review of whale-watching and whaling with applications for the Caribbean. Coast. Manage. 30, 381–399. doi: 10.1080/089207502900273
Hutchings, L., Augustyn, C., Cockcroft, A., Van der Lingen, C., Coetzee, J., Leslie, R., et al. (2009). Marine fisheries monitoring programmes in South Africa. South Afr. J. Mar. Sci. 105, 182–192.
Kaiser, M. J., Spence, F. E., and Hart, P. J. B. (2000). Fishing-gear restrictions and conservation of benthic habitat complexity. Conserv. Biol. 14, 1512–1525. doi: 10.1046/j.1523-1739.2000.99264.x
Kirkman, S. P., Holness, S., Harris, L. R., Sink, K. J., Lombard, A. T., Kainge, P., et al. (2019). Using systematic conservation planning to support marine spatial planning and achieve marine protection targets in the transboundary benguela ecosystem. Ocean Coast. Manage. 168, 117–129. doi: 10.1016/j.ocecoaman.2018.10.038
Kroeker, K. J., Kordas, R. L., Crim, R., Hendriks, I. E., Ramajo, L., Singh, G. S., et al. (2013). Impacts of ocean acidification on marine organisms: quantifying sensitivities and interaction with warming. Glob. Chang. Biol. 19, 1884–1896. doi: 10.1111/gcb.12179
Lester, S. E., Costello, C., Halpern, B. S., Gaines, S. D., White, C., and Barth, J. A. (2013). Evaluating tradeoffs among ecosystem services to inform marine spatial planning. Mar. Policy 38, 80–89. doi: 10.1016/j.marpol.2012.05.022
Lewison, R. L., Johnson, A. F., and Verutes, G. M. (2018). Embracing complexity and complexity-awareness in marine megafauna conservation and research. Front. Mar. Sci. 5:207. doi: 10.3389/fmars.2018.00207
Lombard, A. T., Clifford-Holmes, J. K., Vermeulen, E. A., Witteveen, M., and Snow, B. (2019). “The advantages of system dynamics models in decision-support for integrated ocean management,” Proceedings of the Sixth Annual System Dynamics Conference in South Africa, ed. N. S. Pillay (Johannesburg: Eskom SOC & University of Witwatersrand).
Lombard, A. T., Ban, N. C., Smith, J. L., Wood, S. A., Lester, S. E., Sink, K. J., et al. (in press). Practical approaches and advances in spatial tools to achieve multi-objective marine spatial planning. Front. Mar. Sci.
Lusseau, D., Slooten, L., and Currey, R. J. (2006). Unsustainable dolphin-watching tourism in Fiordland, New Zealand. Tour. Mar. Environ. 3, 173–178. doi: 10.3727/154427306779435184
Marine Spatial Planning Bill [B9-2017] (2017). Department of Environmental Affairs. Government Gazette No. 40726 of 28 March 2017. Available at: https://cer.org.za/wp-content/uploads/2016/03/MSP-Bill-2017-Explanatory-Memo.pdf.
Matcher, G. F., Dorrington, R. A., Henninger, T. O., and Froneman, P. W. (2011). Insights into the bacterial diversity in a freshwater-deprived permanently open Eastern Cape estuary, using 16S rRNA pyrosequencing analysis. Water 37, 381–390.
Matcher, G. F., Froneman, P. W., Meiklejohn, I., and Dorrington, R. A. (2018). Distinct responses of bacterial communities to agricultural and urban impacts in temperate southern African estuaries. Estuar. Coast. Shelf Sci. 200, 224–233. doi: 10.1016/j.ecss.2017.11.015
Maxwell, S. M., Hazen, E. L., Lewison, R. L., Dunn, D. C., Bailey, H., Bograd, S. J., et al. (2015). Dynamic ocean management: defining and conceptualizing real-time management of the ocean. Mar. Policy 58, 42–50. doi: 10.1016/j.marpol.2015.03.014
McInnes, A. M., Khoosal, A., Murrell, B., Merkle, D., Lacerda, M., Nyengera, R., et al. (2015). Recreational fish-finders - an inexpensive alternative to scientific echo-sounders for unravelling the links between marine top predators and their prey. PLoS One 10:e0140936. doi: 10.1371/journal.pone.0140936
Nagelkerken, I., and Connell, S. D. (2015). Global alteration of ocean ecosystem functioning due to increasing human CO2 emissions. Proc. Natl. Acad. Sci. U.S.A. 112, 13272–13277. doi: 10.1073/pnas.1510856112
National Planning Commission [NPC] (2012). National Development Plan 2030: Our Future – Make It Work. Pretoria: National Planning Commission.
Norman, S., Wilkinson, S., Japp, D. W., Reed, J., and Sink, K. J. (2018). A Review and Strengthening of the Spatial Management in South Africa’s Offshore Fisheries. Pretoria: South African National Biodiversity Institute.
O’Connor, S., Campbell, R., Cortez, H., and Knowles, T. (2009). Whale Watching Worldwide: Tourism Numbers, Expenditures and Expanding Economic Benefits. A Special Report from the International Fund for Animal Welfare. Yarmouth: IFAW.
O’Keefe, C., and DeCelles, G. R. (2013). Forming a partnership to avoid bycatch. Fish. Mag. 38, 433–434. doi: 10.1080/03632415.2013.838122
Ortega-Cisneros, K., Cochrane, K., and Fulton, E. A. (2017). An Atlantis model of the southern Benguela upwelling system: validation, sensitivity analysis and insights into ecosystem functioning. Ecol. Modell. 355, 49–63. doi: 10.1016/j.ecolmodel.2017.04.009
Ortega-Cisneros, K., Cochrane, K. L., Fulton, E. A., Gorton, R., and Popova, E. (2018a). Evaluating the effects of climate change in the southern Benguela upwelling system using the Atlantis modelling framework. Fish. Oceanogr. 27, 489–503. doi: 10.1111/fog.12268
Ortega-Cisneros, K., Yokwana, S., Sauer, W., Cochrane, K., Cockcroft, A., James, N. C., et al. (2018b). Assessment of the likely sensitivity to climate change for the key marine species in the southern Benguela system. Afr. J. Mar. Sci. 40, 279–292. doi: 10.2989/1814232X.2018.1512526
Ostrom, E. (2009). A general framework for analyzing sustainability of social-ecological systems. Science 325, 419–422. doi: 10.1126/science.1172133
Parsons, E. C. M. (2012). The negative impacts of whale-watching. J. Mar. Biol. 2012:e807294. doi: 10.1155/2012/807294
Pecl, G. T., Araújo, M. B., Bell, J. D., Blanchard, J., Bonebrake, T. C., Chen, I.-C., et al. (2017). Biodiversity redistribution under climate change: impacts on ecosystems and human well-being. Science 355:eaai9214. doi: 10.1126/science.aai9214
Penry, G., Findlay, K., and Best, P. (2016). “A conservation assessment of Balaenoptera edeni,” in The Red List of Mammals of South Africa, Swaziland and Lesotho, eds M. F. Child, L. Roxburgh, E. Do Linh San, D. Raimondo, and H. T. Davies-Mostert (Modderfontein: South African National Biodiversity Institute and Endangered Wildlife Trust).
Petersen, S., Paterson, B., Basson, J., Moroff, N., Roux, J.-P., Augustyn, C. J., et al. (2010). Tracking the Implementation of an Ecosystem Approach to Fisheries in Southern Africa. WWF South Africa Report Series 2010/Marine/001. Available at: http://assets.wwfza.panda.org/downloads/ecosystem_approach_to_fisheries_april2010_volume_1.pdf
Pichegru, L., Ryan, P. G., van Eeden, R., Reid, T., Grémillet, D., and Wanless, R. (2012). Industrial fishing, no-take zones and endangered penguins. Biol. Conserv. 156, 117–125. doi: 10.1016/j.biocon.2011.12.013
Pikitch, E. K., Santora, C., Babcock, E. A., Bakun, A., Bonfil, R., Conover, D. O., et al. (2004). Ecosystem-based fishery management. Science 305, 346–347. doi: 10.1126/science.1098222
Plön, S., Atkins, S., Conry, D., Pistorius, P., Cockcroft, V. G., and Child, M. F. (2016). “A conservation assessment of Sousa plumbea,” in The Red List of Mammals of South Africa, Swaziland and Lesotho, eds M. F. Child, L. Roxburgh, E. Do Linh San, D. Raimondo, and H. T. Davies-Mostert (Modderfontein: South African National Biodiversity Institute and Endangered Wildlife Trust).
Pongsiri, M. J., Gatzweiler, F. W., Bassi, A. M., Haines, A., and Demassieux, F. (2017). The need for a systems approach to planetary health. Lancet Planet. Heal. 1, 257–259. doi: 10.1016/S2542-5196(17)30116-X
Popova, E., Yool, A., Byfield, V., Cochrane, K., Coward, A. C., Salim, S. S., et al. (2016). From global to regional and back again: common climate stressors of marine ecosystems relevant for adaptation across five ocean warming hotspots. Glob. Chang. Biol. 22, 2038–2053. doi: 10.1111/gcb.13247
Potts, W. M., Götz, A., and James, N. (2015). Review of the projected impacts of climate change on coastal fishes in southern Africa. Rev. Fish. Biol. Fish. 25, 603–630. doi: 10.1007/s11160-015-9399-5
Qiu, W., and Jones, P. J. (2013). The emerging policy landscape for marine spatial planning in Europe. Mar. Policy 39, 182–190. doi: 10.1016/j.marpol.2012.10.010
Rassweiler, A., Costello, C., and Siegel, D. A. (2012). Marine protected areas and the value of spatially optimized fishery management. Proc. Natl. Acad. Sci. U.S.A. 109, 11884–11889. doi: 10.1073/pnas.1116193109
Reed, J., and Lombard, A. T. (2017). The Role of Civil Society in Supporting Marine Spatial Planning. Maritime Review Africa. Available at: http://www.maritimesa.co.za/BackIssues/2017/MayJune2017/tabid/172/Default.aspx
Reiss, H., Hoarau, G., Dickey-Collas, M., and Wolff, W. J. (2009). Genetic population structure of marine fish: mismatch between biological and fisheries management units. Fish Fish. 10, 361–395. doi: 10.1111/j.1467-2979.2008.00324.x
Roberts, C. M., Hawkins, J. P., and Gell, F. R. (2005). The role of marine reserves in achieving sustainable fisheries. Philos. Trans. R. Soc. B 360, 123–132. doi: 10.1098/rstb.2004.1578
Rockström, J., Steffen, W., Noone, K., Persson,Å, Chapin, F. S. III, Lambin, E. F., et al. (2009). A safe operating space for humanity. Nature 461, 472–475. doi: 10.1038/461472a
Rogerson, C., Benkenstein, A., and Mwongera, N. (2018). Coastal Tourism and Economic Inclusion in Indian Ocean Rim Association States. Policy Briefing October 2018. Johannesburg: South African Institute of International Affairs.
Santos, C. F., Agardy, T., Andrade, F., Barange, M., Crowder, L. B., Ehler, C. N., et al. (2016). Ocean planning in a changing climate. Nat. Geosci. 9:730. doi: 10.1038/ngeo2821
Senigaglia, V., Christiansen, F., Bejder, L., Gendron, D., Lundquist, D., Noren, D., et al. (2016). Meta-analyses of whale-watching impact studies: comparisons of cetacean responses to disturbance. Mar. Ecol. Prog. Ser. 542, 251–263. doi: 10.3354/meps11497
Shannon, L. J., Cury, P. M., Nel, D., Van Der Lingen, C. D., Leslie, R. W., Brouwer, S. L., et al. (2006). How can science contribute to an ecosystem approach to pelagic, demersal and rock lobster fisheries in South Africa? Afr. J. Mar. Sci. 28, 115–157. doi: 10.2989/18142320609504139
Sherley, R. B., Barham, B. J., Barham, P. J., Campbell, K. C., Grigg, J., Horswill, C., et al. (2018). Bayesian inference reveals positive but subtle effects of experimental fishery closures on marine predator demographics. Proc. R. Soc. Lond. B 285:20172443. doi: 10.1098/rspb.2017.2443
Sink, K. J., Holness, S., Harris, L., Majiedt, P., Atkinson, L., Robinson, T., et al. (2012a). National Biodiversity Assessment 2011, Technical Report. Volume 4: Marine and Coastal Component. Pretoria: South African National Biodiversity Institute.
Sink, K. J., Wilkinson, S., Atkinson, L. J., Sims, P. F., Leslie, R. W., and Attwood, C. G. (2012b). The Potential Impacts of South Africa’s Demersal Hake Trawl Fishery on Benthic Habitats: Historical Perspectives, Spatial Analyses, Current Review and Potential Management Actions. Pretoria: South African National Biodiversity Institute.
Steffen, W., Richardson, K., Rockström, J., Cornell, S. E., Fetzer, I., Bennett, E. M., et al. (2015). Planetary boundaries: guiding human development on a changing planet. Science 347:1259855. doi: 10.1126/science.1259855
Sterman, J. (2000). Business Dynamics: Systems Thinking and Modeling for a Complex World. New York, NY: McGraw-Hill Education.
Sunagawa, S., Coelho, L. P., Chaffron, S., Kultima, J. R., Labadie, K., Salazar, G., et al. (2015). Structure and function of the global ocean microbiome. Science 348:1261359. doi: 10.1126/science.1261359
Turpie, J. K., Savy, C., Clark, B. M., and Atkinson, L. J. (2005). Boat-based whale watching in south africa: an economic perspective. Report to Department of Environmental Affairs and Tourism: Marine and Coastal Management. Cape Town: Anchor Environmental Consultants.
United Nations (2010). Amended Nairobi Convention for the Protection, Management and Development of the Marine and Coastal Environment of the Western Indian Ocean. Available at: https://www.unenvironment.org/nairobiconvention/
United Nations (2015). Transforming Our World: The 2030 Agenda for Sustainable Development. New York, NY: UN Publishing.
Venkatachalam, S., Matcher, G. F., Lamont, T., van den Berg, M., Ansorge, I. J., and Dorrington, R. A. (2019). Influence of oceanographic variability on nearshore microbial communities of the sub-Antarctic Prince Edward Islands. Limnol. Oceanogr. 64, 258–271. doi: 10.1002/lno.11035
Wang, H., Li, M., Hu, N., and Gao, Y. (2014). Utilization effectiveness of marine functional zones using system dynamics for China: modeling and assessment: a case study of Qingdao Mariculture Functional Zones. J. Coast. Conserv. 18, 609–616. doi: 10.1007/s11852-014-0328-x
Weller, F., Cecchini, L.-A., Shannon, L., Sherley, R. B., Crawford, R. J. M., Altwegg, R., et al. (2014). A system dynamics approach to modelling multiple drivers of the African penguin population on Robben Island, South Africa. Ecol. Modell. 277, 38–56. doi: 10.1016/j.ecolmodel.2014.01.013
Weller, F., Sherley, R. B., Waller, L. J., Ludynia, K., Geldenhuys, D., Shannon, L. J., et al. (2016). System dynamics modelling of the Endangered African penguin populations on Dyer and Robben islands, South Africa. Ecol. Modell. 327, 44–56. doi: 10.1016/j.ecolmodel.2016.01.011
Westholm, A. (2018). Appropriate scale and level in marine spatial planning–management perspectives in the Baltic Sea. Mar. Policy 98, 264–270. doi: 10.1016/j.marpol.2018.09.021
WFD (2000). Directive 2000/60/EC of the European Parliament and of the Council Establishing a Framework for the Community Action in the Field of Water Policy. Available at: http://ec.europa.eu/environment/water/water-framework/index_en.html
White, C., Halpern, B. S., and Kappel, C. V. (2012). Ecosystem service tradeoff analysis reveals the value of marine spatial planning for multiple ocean uses. Proc. Natl. Acad. Sci. U.S.A. 109, 4696–4701. doi: 10.1073/pnas.1114215109
Witherell, D., and Pautzke, C. (1997). A brief history of bycatch management measures for eastern Bering Sea groundfish fisheries. Mar. Fish. Rev. 59, 15–22.
Keywords: ecosystem condition, oceans economy, climate change, dynamic ocean management, scenario planning, system dynamics models, complex systems, trade-offs
Citation: Lombard AT, Dorrington RA, Reed JR, Ortega-Cisneros K, Penry GS, Pichegru L, Smit KP, Vermeulen EA, Witteveen M, Sink KJ, McInnes AM and Ginsburg T (2019) Key Challenges in Advancing an Ecosystem-Based Approach to Marine Spatial Planning Under Economic Growth Imperatives. Front. Mar. Sci. 6:146. doi: 10.3389/fmars.2019.00146
Received: 08 November 2018; Accepted: 07 March 2019;
Published: 27 March 2019.
Edited by:
Edward Jeremy Hind-Ozan, Department for Environment, Food and Rural Affairs, United KingdomReviewed by:
Wesley Flannery, Queen’s University Belfast, United KingdomDi Jin, Woods Hole Oceanographic Institution, United States
Copyright © 2019 Lombard, Dorrington, Reed, Ortega-Cisneros, Penry, Pichegru, Smit, Vermeulen, Witteveen, Sink, McInnes and Ginsburg. This is an open-access article distributed under the terms of the Creative Commons Attribution License (CC BY). The use, distribution or reproduction in other forums is permitted, provided the original author(s) and the copyright owner(s) are credited and that the original publication in this journal is cited, in accordance with accepted academic practice. No use, distribution or reproduction is permitted which does not comply with these terms.
*Correspondence: Amanda T. Lombard, bWFuZHkubG9tYmFyZEBtYW5kZWxhLmFjLnph