- 1Feed and Nutrition, Norwegian Institute of Marine Research, Bergen, Norway
- 2Department of Biological Sciences, University of Bergen, Bergen, Norway
- 3Faculty of Veterinary Medicine, Norwegian University of Life Sciences, Oslo, Norway
- 4Department of Aquaculture Production Technology, Nofima AS, Sunndalsøra, Norway
- 5Department of Nutrition and Feed Technology, Nofima AS, Bergen, Norway
Little is known about the digestion of nutrients in stomachless (agastric) fishes with short intestines, such as wrasse. This study describes the digestion, absorption and evacuation rates in ballan wrasse (Labrus bergylta) fed either dry or pre-soaked diets. Ballan wrasse juveniles received either dry or pre-soaked diets containing inert markers to determine intestinal evacuation rates over a 4–14 h period after ingestion. The digestive tract evacuation was completed in 12–14 h, and was not affected by dietary moisture level. Within the different segments of the intestine, 90% of the digesta moved from the foregut to the midgut after 4–8 h, and over 7% of the digesta had arrived in the hindgut 4 h after feeding. The foregut was a major site of digestion, where 86% of carbohydrates, 74% of proteins, and 50% of lipids, were absorbed from the diet. The absorption of carbohydrates and lipids increased until the hindgut (98 and 80%, respectively). Protein absorption continued along the length of the intestine reaching 90% absorption at the hindgut. Protein and carbohydrate absorption was slightly higher in the foregut for fish fed dry versus moist diets, but this difference disappeared in the midgut. The moisture levels of diet had no effect on the digesta moisture levels, expression of metabolic genes, the levels of nutrients in plasma, or intestinal health. Nine aquaporins were found to be expressed in the wrasse intestinal tissue. Vertebrate aquaporins are involved in water transport from the intestinal lumen into the body and may play a role in maintaining stable moisture level of the digesta in the intestine of ballan wrasse regardless of the initial dietary moisture levels.
Introduction
The stomach is a specialized organ located in the anterior gut in many vertebrates, characterized by the occurrence of acid (HCl) and pepsin-producing gastric glands (Koelz, 1992). The stomach has multiple functions which include storage of food, initiation of protein digestion, inactivation of pathogens and controlling chyme release to the intestine (Wilson and Castro, 2010). The storage function of the stomach allows an animal to ingest larger meals, which is particularly important for animals that swallow whole large prey, as it occurs in many fish species. However, the stomach has been lost at least 15 times in the evolution of fishes (Castro et al., 2014), including in the ancestor of extant wrasses.
During digestion, the ingested feed is degraded by a combination of mechanical and enzymatic processes. Retention and transferring of digesta to different compartments within the gastrointestinal tract are tightly controlled processes that have evolved to optimize the digestion and absorption of nutrients from food. In gastric fishes, food is temporarily stored in the stomach, allowing time for lowering pH as well as secretion and activation of pepsinogen. The active pepsin hydrolyses specific peptide bonds and initiate protein hydrolysis. For instance, in juvenile Korean rockfish (Sebastes schlegeli), the stomach has been shown to remain full at 4 h post feeding and was gradually evacuated until empty by 20 h post feeding (Lee et al., 2000). Many gastric fish also have pyloric caeca, i.e., finger-like blind end tubes projecting off the anterior intestine. The pyloric caeca increase the overall intestinal absorptive surface area, and thus the absorption efficiency of nutrients of the gastrointestinal tract. While lacking a stomach, agastric fish may possess an intestinal bulb or swelling in the anterior intestine instead, which has been reported to function as a food storage area (Barrington, 1942; Kapoor et al., 1976; Guillaume and Choubert, 2001). Furthermore while also lacking pyloric caeca, agastric fish may have a relatively long intestine measuring several times the animal body length (e.g., bighead carp Aristichthys nobilis) (Opuszynski and Shireman, 1991), which increases the overall surface area of the intestine. However, it remains unknown how efficiently nutrients are digested and absorbed in agastric fish with short digestive tracts such as the ballan wrasse (Figure 1) (Deady and Fives, 1995; Figueiredo et al., 2005; Skiftesvik et al., 2014; Lie et al., 2018; Artüz, 2019). It is also unknown if the wider anterior segment of ballan wrasse functions as a food storage area and thus if the intestinal bulb should be considered a pseudogaster.
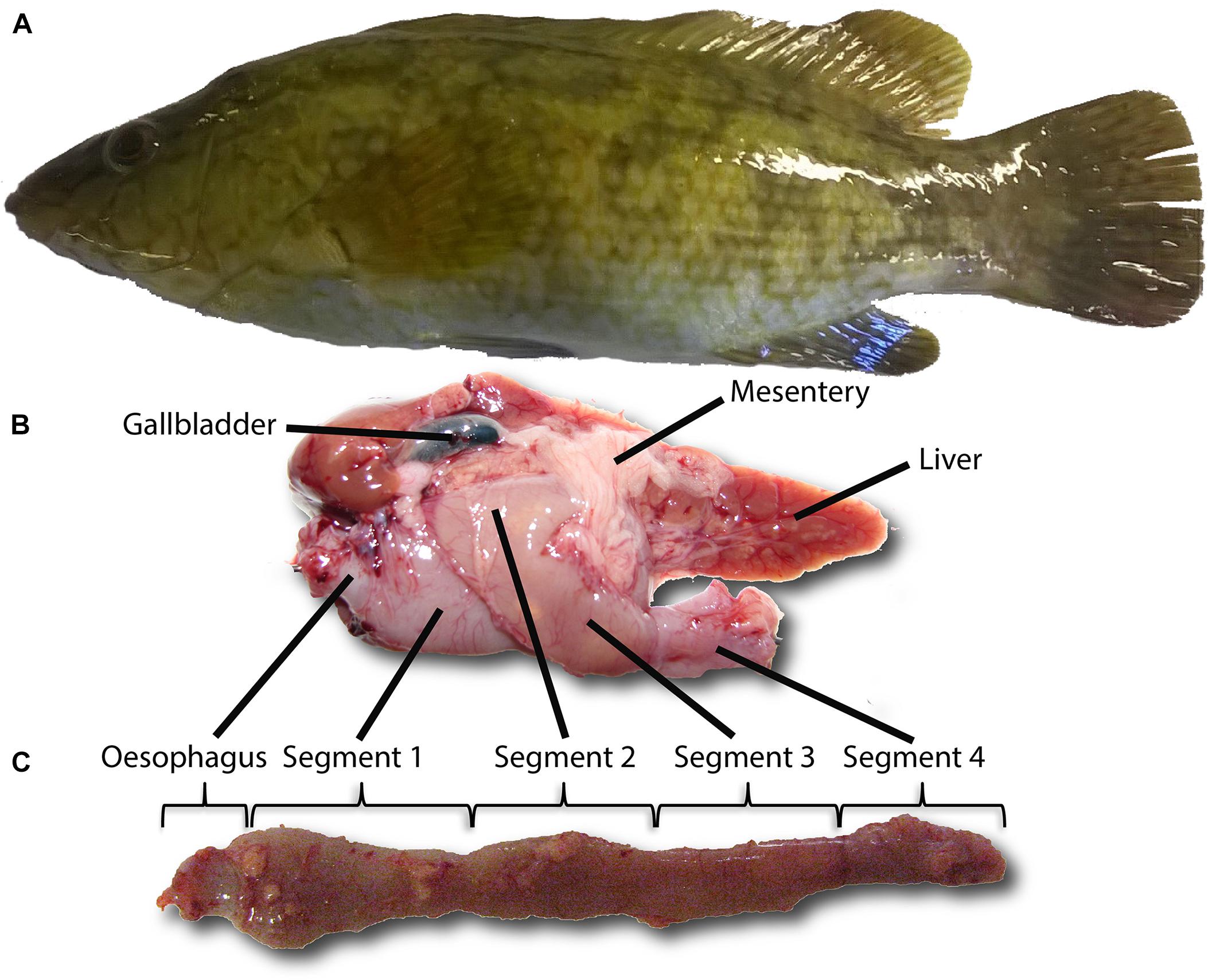
Figure 1. Digestive system of ballan wrasse. Ballan wrasse (Labrus bergylta) (A); digestive system as found in vivo (B); anatomy of the intestine and the division of the intestinal tract into 4 segments (C), based on Lie et al. (2018).
Physical characteristics and chemical composition of the diet fed to an animal affect gastrointestinal evacuation rates, which in turn is an important factor in determining the utilization of ingested dietary nutrients. Prolonging the passage time of feed has the potential to increase enzymatic digestion and mucosal absorption of nutrients and may also allow beneficial microorganisms to assist in digestion (Olsen and Ringø, 1997; Venero et al., 2015). As such, a reduced gut transit time has been found to diminish the efficiency of digestion and absorption of ingested feed in several fish species (Grove et al., 1978; Flowerdew and Grove, 1979; Jobling, 1981, 1987; Garber, 1983; Lee et al., 2000). In gastric species moist diets can have shorter passage rates than dry diets, as found for turbot Scophtalamus maximus (Grove et al., 2001). In several studies, faster gastrointestinal transit has been shown to enhance feed intake rates, e.g., in greater amberjack Seriola dumerili (Papadakis et al., 2008) and Atlantic salmon Salmo salar (Aas et al., 2013), but reduce nutrient digestibility, e.g., in European seabass Dicentrarchus labrax (Adamidou et al., 2009) and gilthead sea bream Sparus aurata (Venou et al., 2009). However, other studies found that the dietary moisture level did not affect the rate of gastrointestinal evacuation in Korean rockfish (Lee et al., 2000) and olive flounder Paralichthys olivaceus (Kim et al., 2011); or the digestibility of nutrients in rainbow trout Oncorhynchus mykiss (Aas et al., 2011) and Atlantic salmon (Oehme et al., 2014).
In this study, we investigated the effect of dietary moisture level on gut evacuation rates in ballan wrasse. Ballan wrasse were fed either dry or pre-soaked (moist) diets, and then the rate of evacuation and absorption of macronutrients from the diets in individual segments, as well as overall, were measured. Furthermore, gene expression and histology of the intestine was evaluated to identify any transcriptional or histomorphological changes due to dietary moisture level. We found that the food is evacuated from the ballan wrasse gastrointestinal tract within 12–14 h, and that the first segment of the intestine plays a major role in nutrient digestion and absorption. Dietary moisture level had no effect on the overall gastrointestinal evacuation rate or the moisture level of digesta, histomorphology and had a limited effect on gene expression. The results demonstrate that in spite of possessing a rather basic gastrointestinal tract, ballan wrasse can efficiently digest and absorb nutrients in a similar manner to species with stomach, pyloric caeca and/or long intestinal tract.
Materials and Methods
Diets
The experimental diets were produced at Nofima’s Feed Technology Centre (Bergen, Norway) in a co-rotating twin screw extruder (TX52, Wenger Manufacturing Inc., Sabetha, KS, United States) and dried in a dual layer carousel dryer (Model 200.2; Paul Klockner GmbH, Nistertal, Germany). The pellets were 2 mm in diameter and sank slowly in seawater (density >1.024 g/cm3). The diets were formulated based on information developed through a series of feeding studies (e.g., Kousoulaki et al., 2014, 2015; Krogdahl et al., 2014; Bogevik et al., 2016), and were based on high quality marine raw materials (see Table 1). Two batches were made, one with yttrium the other with ytterbium as inert markers. Moist diets were prepared by soaking dry pellets in seawater for 4–5 min prior to feeding and the proximate composition of nutrients in the dry and pre-soaked (moist) diets are showed in Table 2.
Experimental Design and Sampling
The fish used for these experiments had been reared for 1 year in a commercial farm (Marine Harvest, Bergen, Norway) before transfer to the Nofima AS land based research facility in Sunndalsøra, Norway. Before arrival, the fish had been fed the yttrium labeled dry diet for 6 weeks. The fish were transported on September the 2nd, 2015, by truck for 4 h and distributed to 12 cylindrical flat-bottomed black tanks with a volume of 350 l, equipped with separate light sources. The tank water temperature during the experiments was 14.5–17°C and the photoperiod was of 24-h daylight. Four days after arrival all fish were individually tagged [passive integrated transponder (PIT), weighed (average 89 ± 2 g, mean ± SEM) and length measured (average 17.1 ± 1.0 cm, mean ± SEM)]. Fish densities were adjusted to 20 kg/m3. Feed was distributed automatically every 10th min. using automatic belt feeders for the duration of the experiment. Each belt feeder (one per tank) was loaded with feed equivalent to 12 g feed/kg fish per day. The moist diet was prepared by weighing the daily feed amount for each tank before adding enough water to just cover the dry diet, all of the water was absorbed by the pellets. Four minutes later the moist diet was distributed to each automatic belt feeder and leveled out using a rubber spatula. Dry diet was also added and levelled out on automatic belt feeders daily.
The two feeding experiments followed the Norwegian animal welfare act guidelines, in accordance with the Animal Welfare Act of 20th December 1974, amended 19th of June, 2009. The facility at Sunndalsøra, Nofima (division 60) got a permission granted by the Norwegian Food Safety Authority, (FOTS ID 8060) to run these experiments. The decision was made on the basis of Regulations 18. June 2015 on the use of animals in experiments, §§6, 7, 9, 10, and 11.
Experiment 1 (Passage Rate)
To evaluate the passage rate of feed, the replacement of yttrium labeled feed by ytterbium labeled feed in the intestinal lumen was analyzed. At the start of the experiment, diets containing yttrium (Y-dry and Y-moist) were replaced by diets with ytterbium (Yb-dry and Yb-moist). Gut contents were collected at 4, 6, 8, 10, 12, and 14 h after changing to diets containing ytterbium. At each time point, 6 fish from 2 tanks per treatment were euthanized with an overdose of MS-222 before dissection. The whole intestine was dissected out and divided into 4 segments as shown in Figure 1C. Gut contents were collected from each intestinal segment and used for yttrium and ytterbium analysis.
Experiment 2 (Digestibility)
To evaluate macronutrient digestibility, gut transcriptomes and plasma indicators of metabolism, fish in two tanks were fed Y-labeled dry diet and two tanks fed Y-labeled moist diet for 5 weeks. At the termination of the feeding trial, fish were randomly taken from the tanks and euthanized with an overdose of MS-222 before tissue sampling. Firstly, blood samples were collected to analyze circulating levels of nutrients. Then the intestine was removed from the abdominal cavity, cleaned of mesenteric fat and divided into four segments as shown in Figure 1C. Each section was opened longitudinally and the gut content collected for analysis of dry matter, crude protein, fatty acid composition, and carbohydrates for calculating nutrient apparent digestibility coefficients (ADCs). For digestibility evaluation, three pooled samples of gut content from 20 randomly selected fish were collected from each of the four tanks. Intestine and liver tissues from six fish per each tank were sampled for RNA extraction (submerged in RNAlater solution, incubated at 4°C for 24 h and stored at -20°C) and histomorphological evaluation (fixed in 4% phosphate-buffered formaldehyde solution for 24 h and transferred to 70% ethanol for storage).
Chemical Analyzes
Concentrations of markers (Experiments 1 and 2), crude protein, fatty acids, and carbohydrate were measured in both feed and gut content samples (Experiment 2). Yttrium (Y) and ytterbium (Yb) were analyzed using ICP-MS with Chem Station software (Agilent 7500, Agilent Technologies Inc., Santa Clara, CA, United States). Crude protein levels were analyzed with an Elemental®Vario Macro Cube instrument (Elementar Analysensysteme GmbH, Hanau, Germany) using the standard conversion factor (× 6.25). The compositions of lipid (total fatty acids - TFA), monounsaturated fatty acids (MUFA), polyunsaturated fatty acids (PUFA), and saturated fatty acids (SFA) were determined using chromatography with the 19:0 methyl ester as an internal standard according to Lie and Lambertsen (1991). The carbohydrate contents were measured via glucose produced from hydrolysis of carbohydrate compounds, based on the Trinder reaction described in Hemre et al. (1989).
Plasma
Plasma was analyzed for cholesterol, non-esterified fatty acids (NEFA), total triacylglycerides (TAG), total protein and glucose at the Central Laboratory at the Faculty of Veterinary Medicine, Oslo according to standard, medical procedures.
Histology
Six fish from each tank in Experiment 2 were sampled and analyzed histologically for inflammation. Table 3 below shows the number of histology sections for each intestinal region per diet group that were suitable for the semi-quantitative histology evaluation.
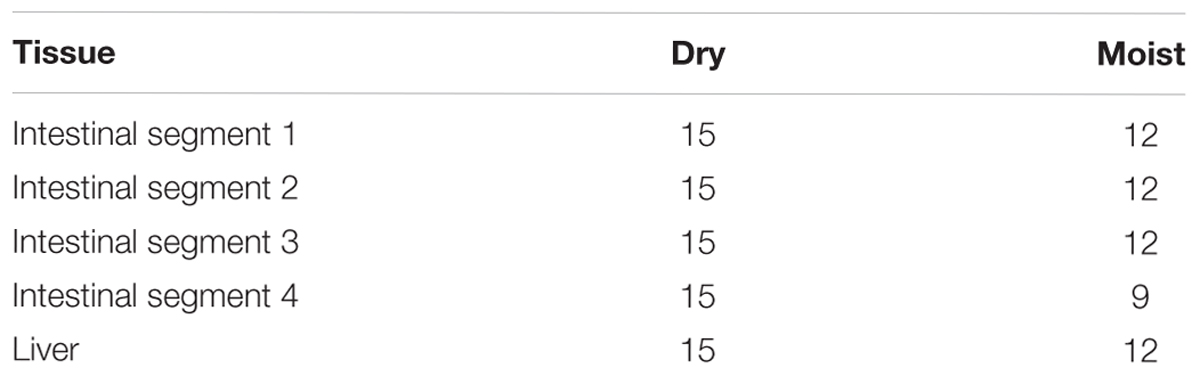
Table 3. Overview of the number of intestine and liver tissue sections for each diet group that were of suitable quality for semi-quantitative histomorphological evaluation.
Histological sections were prepared using paraffin technique and H&E (Haematoxylin and Eosin) staining system according to Baeverfjord and Krogdahl (1996). The histological sections were evaluated for morphological changes associated with inflammatory reaction in the intestinal mucosa. Other morphological features unique to the ballan wrasse were also noted and graded. The degree of change of the various morphological features was graded using a scoring system with a scale of 1–10 where 1 to <3 represented normal; 3 to <5, mild changes; 5 to <7, moderate changes; >7 to <9, marked changes, and 9–10, severe changes.
Evaluation of the liver sections focused on changes to hepatocyte morphology, and presence of specific pathological changes such as degeneration, hemorrhage, or inflammation. The degree of liver changes was graded using a scoring system with a scale of 1–5 where 1 represented normal and 2–5 represented mild, moderate, marked, and severe changes, respectively.
RNA Extraction
Total RNA was extracted and DNA removed from anterior intestinal samples (i.e., Segment 1 in Figure 1C) using a BioRobot®EZ1 and RNA Tissue Mini Kit (Qiagen, Hilden, Germany). RNA quantity and purity were assessed using a NanoDrop ND-1000 UV–vis Spectrophotometer (NanoDrop Technologies, Wilmington, DE, United States). All samples had 260/230 and 260/280 ratios above 2.0 and 2.2, respectively, indicating high RNA purity. RNA integrity was determined using the Agilent 2100 Bioanalyzer and RNA 6000 Nano LabChip kit (Agilent Technologies, Palo Alto, CA, United States). The average RNA integrity number (RIN) of all samples was 7.8 ± 0.5.
Transcriptome Sequencing
Sequencing was performed by the Norwegian Sequencing Centre1. DNA sequencing libraries were prepared with an automated NeoPrep platform (Illumina) using 90 ng total RNA input to the TruSeq Stranded mRNA Library Prep Kit (Illumina) and standard Illumina adaptors included in the kit. The libraries were sequenced using HiSeq4000 according to manufacturer instructions, generating pair end libraries with an average library size of 14.2 ± 1.4 million reads.
Calculations
Estimates of Feed Intake
The amount of feed ingested for each treatment was calculated from the sum of markers (Yb and Y2O3) in gut content from 4 intestinal segments as such: Feed intake (mg g-1) = [(Yb + Y2O3) in 4 segments (mg)]∗100∗[0.05∗BW (g)]-1, with 0.05 equalling the % of marker in feed (see Table 1).
Gut Passage Rate
Fecal concentrations for each marker were first normalized by dividing them with the dietary marker concentration. The passage rate in each segment was calculated as the percentage between Yb and the total amount of two markers in the gut content, as such: Yb (%) = 100∗Yb∗(Y2O3 + Yb)-1.
Moisture of Intestinal Content
The water content (%) of gut content was obtained as the ratio between water weight in each wet sample of gut content collected in digestibility experiment and its wet weight: water content (%) = 100 ∗ (wet weight of gut content - dry weight of gut content) ∗ (wet weight of gut content)-1.
Apparent Digestibility Coefficient of Macronutrients
The ADC of macronutrients was calculated as following: ADC (%) = [1 - (nutrient content in gut content ∗ feed marker content) ∗ (marker content in gut content ∗ feed nutrient content)-1] ∗ 100.
Data Analysis
The Y2O3:Yb ratio within intestinal segments were used to calculate the intestinal evacuation rate between intestinal segments at given time points. Yb % data, as the response variable, were treated as continuous proportions ranging from 0 to 1 as quasibinomial distribution and compared with regards to both diet and sampling time as predictor variables for each intestinal segment using a generalized linear mixed models (GLMM) (R, version 3.4.2 released 2017-09-28, within RStudio interface (version 1.1.383) for Windows. At some time-points there were large variation differences in the Yb data between the two groups (dry and moist), so the Wilcoxon Rank Sum and Signed Rank Tests (wilcox.test) was applied to test the difference.
Apparent digestibility coefficients of the nutrients as the response variable were also treated as continuous proportions ranging from 0 to 1. The nutrient ADCs for each of the four intestinal segments were clustered in sub-groups of fish from each diet group. The generalized linear mixed models (glmmPQL) test was used to analyze the correlations between diet and intestinal segment as the fixed effect factors, with individual fish as the random effect factor, and the ADC as a response variable with a quasi-binominal distribution. Changing the contrast where each gut segment (S1–S4) became the intercept of the model was applied to determine the variation in ADC between the two diets in each segment. If the full model of fixed effect factors (diet and gut section) showed the diet had no effect on the ADC, the diet factor was then removed from the model. This model was used to compare the ADC among the 4 intestinal segments by changing the contrast. The Wilcoxon Rank Sum and Signed Rank Tests (wilcox.test, paired = TRUE) was applied to test the difference in the digestibility of three lipid classes. The glmmPQL was also applied to the data of water content in gut content with the ratio of water as a response variable and the diet and intestinal section as the predictors. Data of plasma components were analyzed using one-way ANOVA. Differences were considered significant at p < 0.05 for all tests in this study. All data are presented as mean ± SEM.
Sequence Analysis
Remaining adaptors were removed using Cutadapt (Martin, 2011) and default parameters. Reads were further trimmed and filtered using Sickle2 with a 40 bp minimum remaining sequence length, Sanger quality of 20, and no 5′ end trimming. Library quality was investigated using fastQC version 0.9.2 (The FastQC project3). Each intestinal RNAseq library was mapped individually to the labrus genome assembly (European Nucleotide Archive accession number: PRJEB136874) using the Tophat2 short read aligner (Langmead et al., 2009). Transcript abundances were estimated using the FeatureCounts software of the Subread package5. The Bioconductor - DESeq2 analysis package (Love et al., 2014) was used for differential expression analysis. Genes of which read counts < 10 in all samples were excluded from further analysis prior to normalization and differential expression analysis. An adjusted p-value (p-adjust) of <0.1 was applied for further downstream analysis using the DAVID Bioinformatics Resources 6.8 (Beta) with default settings (GO and KEGG pathway analysis).
Histological Data
Differences in histological scores for the various evaluated morphological characteristics of the gut and liver tissue were analyzed for statistical significance using the Fisher’s Exact test. Post hoc analysis for significant Fisher’s Exact test results was conducted using the chi-squared post hoc test. Both statistical tests were run in the R statistical package (version 3.2.4; 2016) within the RStudio interface (version 0.99.892; 2016). Differences were considered significant at p < 0.05.
Results
Histology
Fish from Experiment 2 were evaluated for morphological changes associated with inflammatory reactions in the intestinal mucosa. The results of the histological evaluation of the four intestinal segments and the liver are shown in Figures 2, 3. A number of fish displayed mild to moderate changes in lamina propria cellularity and intraepithelial lymphocyte infiltration, predominantly in intestinal segments S2 and S3. None of the chosen indicators of gut health status were significantly different between fish fed dry or moist diets (Fisher’s Exact test, p > 0.05).
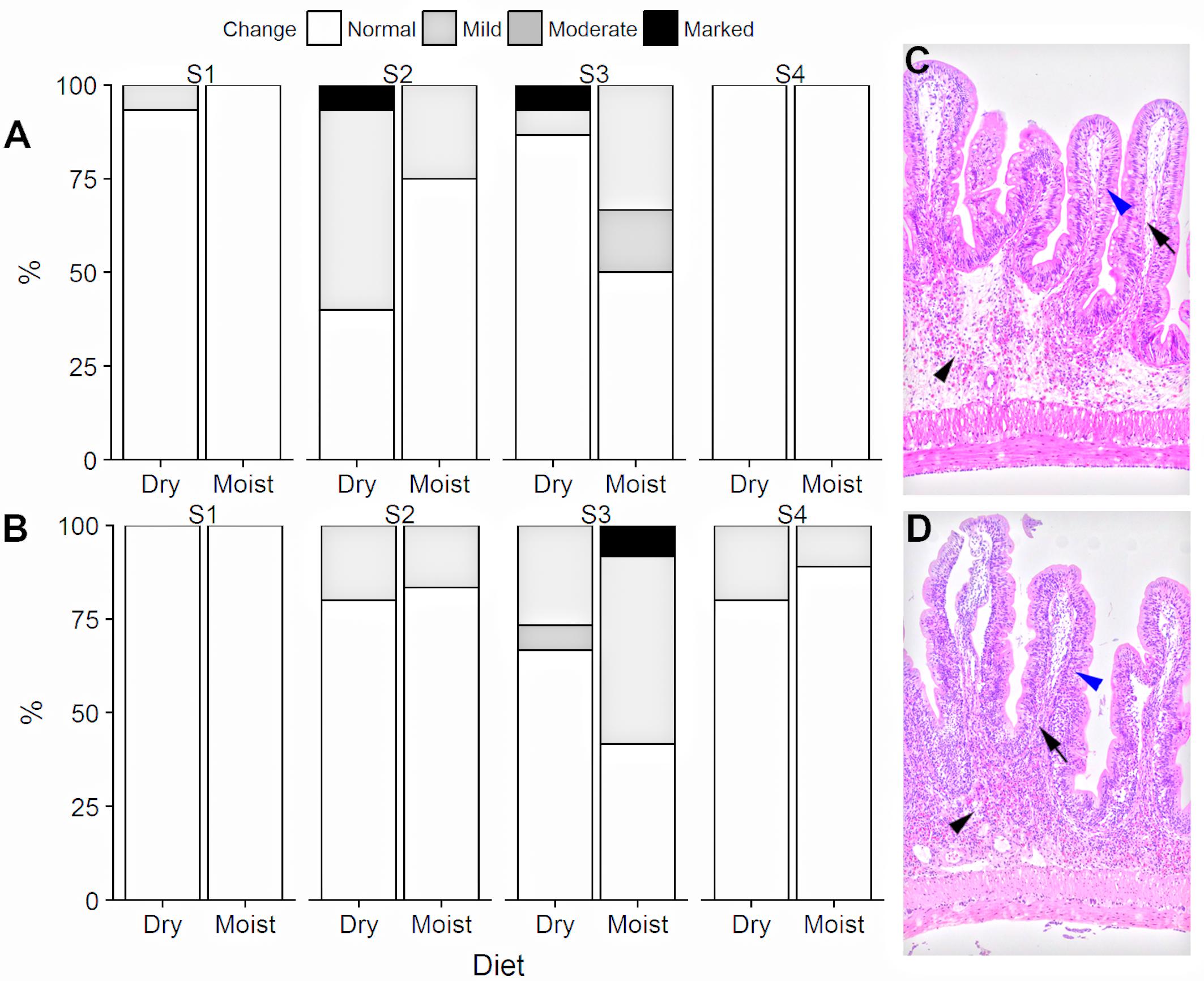
Figure 2. Histological evaluation of intestinal segments in ballan wrasse fed either dry or moist diets. The percentage of each intestinal segment that had normal, mild, moderate, or marked infiltration of mixed populations of inflammatory cells in the intestinal lamina propria (A) and intraepithelial lymphocyte infiltration (B). S1, S2, S3, S4 stand for Segments 1, 2, 3, 4. Data were obtained from 15 fish each for all segments for the dry diet, 12 each (S1–S3) and 9 each (S4) fish for the moist diet. (C) Normal. (D) Moderate submucosa (black arrowhead) and lamina propria (black arrow) increase in cellularity due to infiltration by a mixed population of inflammatory cells and infiltration of the intraepithelial space (blue arrowheads). There was no statistical difference between moist and dry diets (Fisher’s Exact test, p > 0.05).
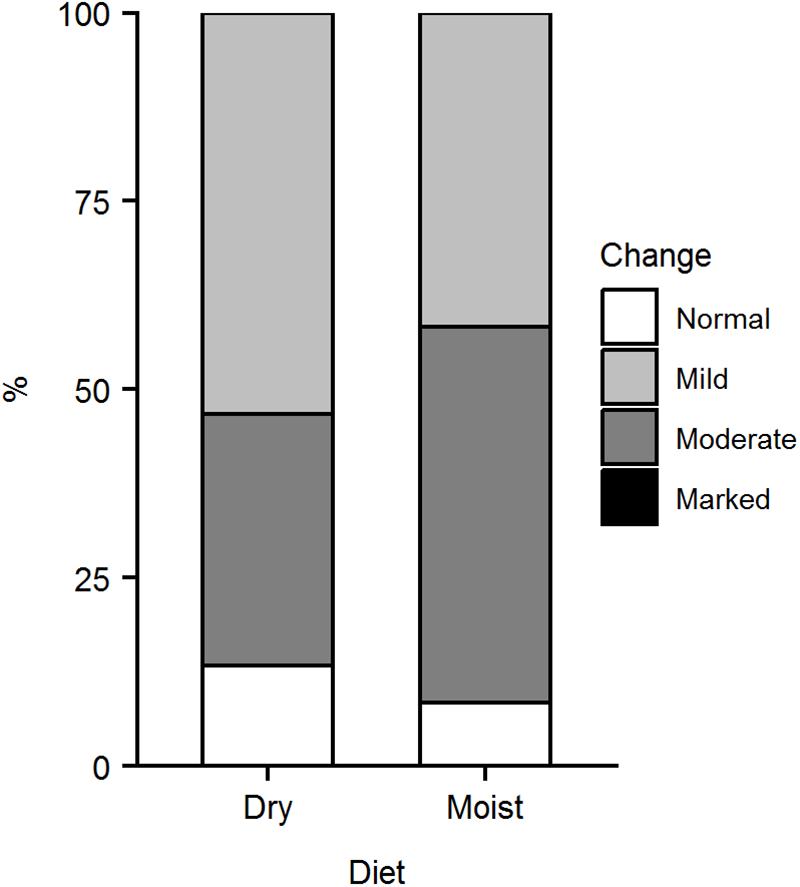
Figure 3. Histological evaluation of liver in ballan wrasse fed either dry or moist diets. The graph shows the percentage of hepatocytes with either marked, moderate, mild or normal levels of vacuolization in the liver. Data were from 15 (dry diet) or 12 (moist diet) fish. There was no statistical difference between moist and dry diets (Fisher’s Exact test, p > 0.05).
Evaluation of the liver sections focusing on the degree of vacuolization of the hepatocytes showed that most fish had mild to moderate vacuolization of the hepatocytes (Figure 3). There was no significant difference in hepatocyte vacuolization between the two diet groups (Fisher’s Exact test, p > 0.05).
Feed Intake and Gut Evacuation Rate
The fish weighed 100.0 ± 1.8 g at the time of sampling in Experiment 1. The estimated feed intake (feed dry weight/body wet weight) based on the yttrium marker was 1.3 ± 0.1 mg/g in fish fed the dry diet and 1.1 ± 0.1 mg/g in fish fed the moist diet. There was no significant difference in estimated feed intake between the two groups. Since the fish were fed continuously and feed could be found in most segments in most of the fish analyzed, no evidence for meal-based feeding behavior could be identified.
Segment 1
Four hours post dietary label change (hpc), 90.0 ± 3.0% (n = 4) and 97.9 ± 0.3% (n = 3) of the initial Y-labeled feed had been replaced by the Yb-labeled feed in the fish fed dry diet or moist diet, respectively. The difference between the two treatments was not significant (GLMM, p > 0.05). The amount of Yb in Segment 1 increased to the maximum at 8 hpc and it then plateaued within six following hours for both diet treatments (from 97.0 ± 0.6 to 98.5 ± 0.1% for dry, from 96.2 ± 1.4 to 98.3 ± 0.1% for moist) (Segment 1, Figure 4).
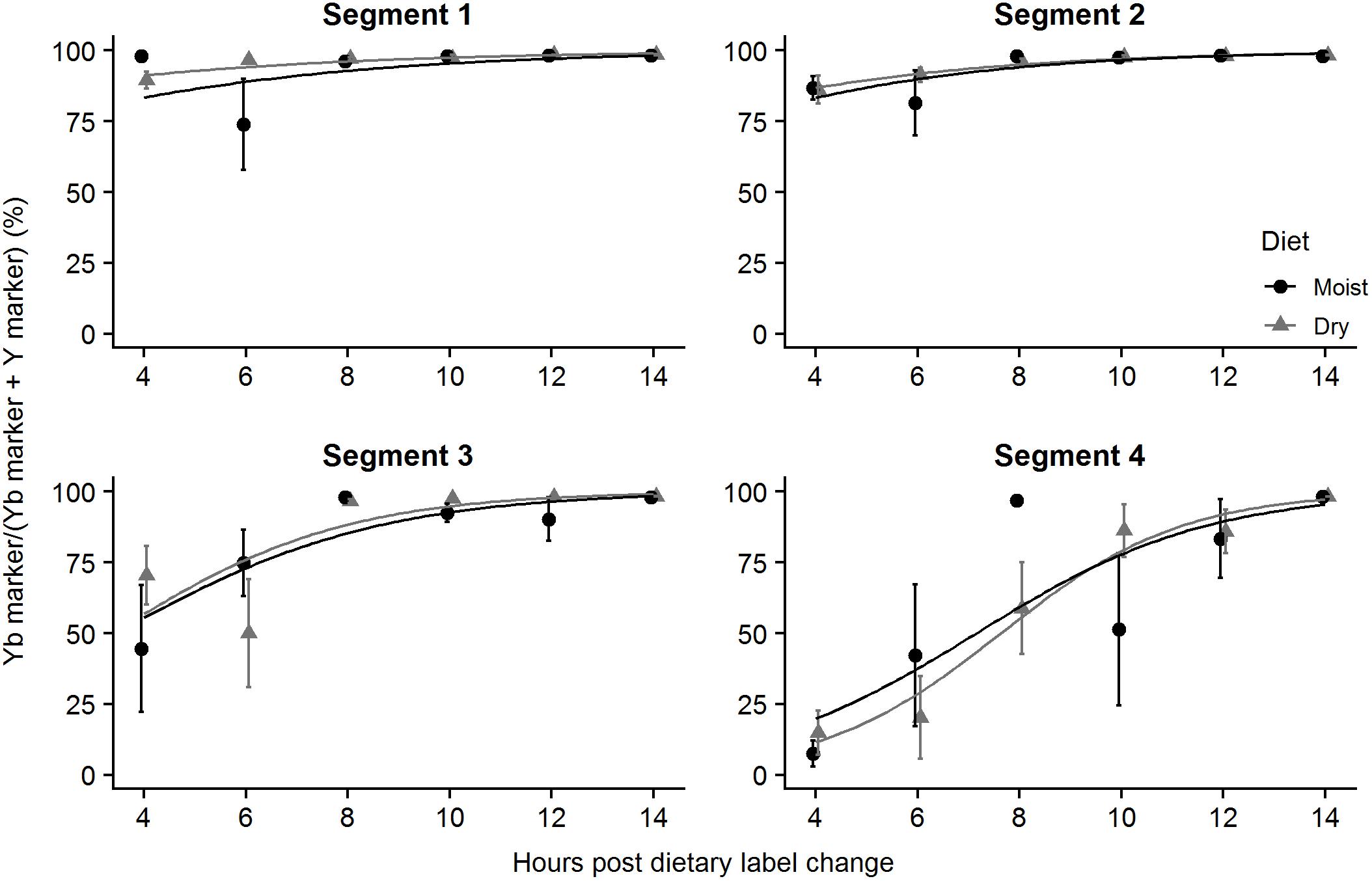
Figure 4. Passage rate (% evacuation) of dry and moist diets through ballan wrasse intestine in vivo. The figures show the ratio (mean ± SEM) between Yb marker and the sum of markers [Yb × (Y2O3 + Yb)-1 × 100] represented in the gut content collected from the 4 intestinal segments (n = 12 for each segment) at 4, 6, 8, 10, 12, and 14 h after fish fed Y-labeled feed were switched to those containing Yb. Some error bars are not visible as they are shorter than symbol heights. For each intestinal segment, no significant difference in the percentages of Yb between moist and dry diet treatments (GLMM, p > 0.05). The curves were predicted by general linear mixed models in R.
Segment 2
Four hpc 86.2 ± 4.9% for dry diet and 86.7 ± 4.1% for moist diet of the Y-labeled diet had been replaced by the Yb-labeled diet. Ten hpc 97.9 ± 0.5% of Y-dry diet and 98.1 ± 0.2% of Y-moist diet were evacuated out of the Segment 2. In both treatment groups, a tiny portion of Y marker still remained (∼2% of the total sum of the markers) at 14 hpc (Segment 2, Figure 4). The diet type had no effect on the evacuation rate in the Segment 2.
Segment 3
In Segment 3, 70.4 ± 10.3% for dry diet and 44.6 ± 22.4% for moist diet of Yb-labeled diet had been taken over the Y-labeled diet at 4 hpc. The proportion of Yb marker then rose significantly and reached to the peak at 98.3 ± 0.1% (in dry group) and 98.1 ± 0.2% (in moist group) at 14 hpc (Segment 3, Figure 4). No effect of the moist levels of feed on the passage rate was found in the Segment 3.
Segment 4
14.8 ± 7.8% Y marker from dry diet and 7.5 ± 4.5% Y marker from moist diet were replaced by Yb marker in the last segment at 4 hpc. Over the following 10 h, the amount of Y marker replaced by Yb marker increase to a maximum of 98.3 ± 0.0% for the dry diet group and 98.3 ± 0.1% for the moist diet group at 14 hpc. Only tiny amounts of Y marker still remained in the fourth segment at 14 hpc (Segment 4, Figure 4). The proportions of Yb marker were not statistically different between the dry and moist group.
Overall, the feed resided 4–8 h in the foregut and the total passage rate was ∼12–14 h. GLMM for fixed effects (diet and sampling time point) showed that the proportion of Yb in the digesta in each segment increased with time, but diet type had no effect (Figure 4).
Moisture of Gut Content
The water content of the digesta was ∼80% by weight and was not statistically different between the four intestinal segments or between fish fed either dry or moist diets (Figure 5) (glmmPQL, p > 0.05).
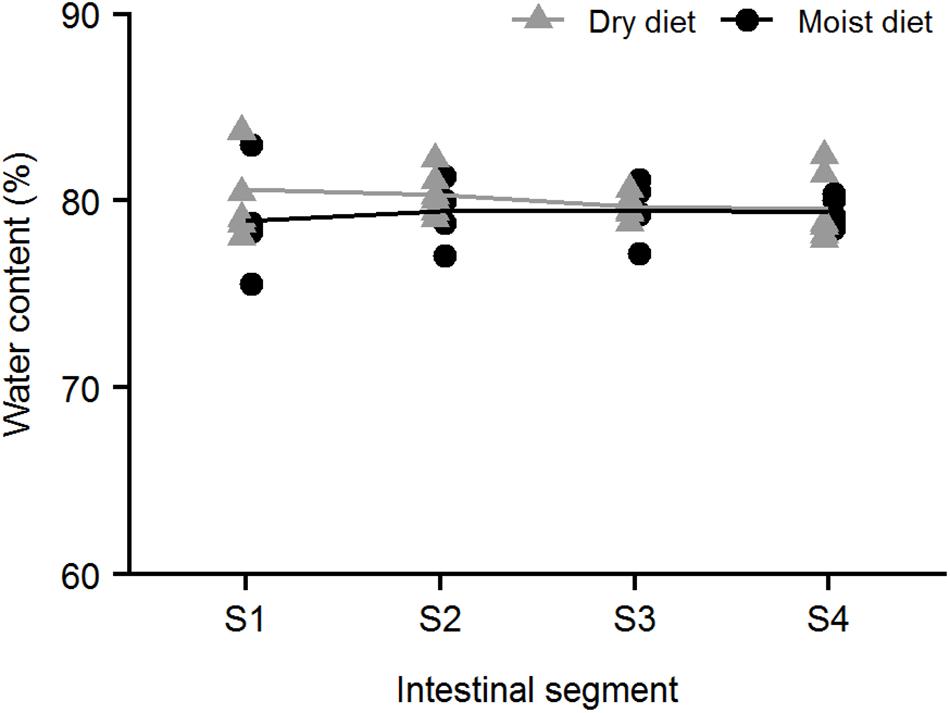
Figure 5. Water content of digesta in ballan wrasse intestine. The water content of digesta from the four intestinal segments (S1–S4) of fish that were fed either moist or dry diets (n = 6 for each segment each diet).
Macronutrient Digestibility
Apparent digestibility coefficients for protein and carbohydrates in the foregut (Segment 1, Figures 6, 7) were higher (p < 0.05, glmPQL) in the fish fed the dry compared to moist diets. However, in the midgut (Segments 2 and 3) and hindgut (Segment 4), the ADC for proteins and carbohydrates were not significantly different between fish fed dry or moist diets. The digestibility of lipid was not affected by dietary moisture level (glmPQL, p > 0.05, Figure 8). However, the ADC for fatty acids were different between three fatty acid classes. Polyunsaturated fatty acids (PUFA) had higher ADCs in all the four segments than monounsaturated fatty acids (MUFA) and saturated fatty acids (SFA) (wilcox.test, p < 0.01). The ADC for SFA was lower than that for MUFA in Segments 1 and 3 (wilcox.test, p < 0.05); but they were similar in Segments 2 and 4. ADCs showed that digestion and absorption of protein, carbohydrate and lipid had similar patterns in the intestine of ballan wrasse. Up to 85.87, 73.57, and 48.97% of carbohydrates, proteins, and lipid, respectively, were digested and absorbed in Segment 1. In addition, the ADC of all macronutrients increased significantly along the intestine, with the ADCs increasing to a maximum for carbohydrate and lipid in Segment 3, and for protein in Segment 4 (glmPQL, p < 0.05, Figures 6–8).
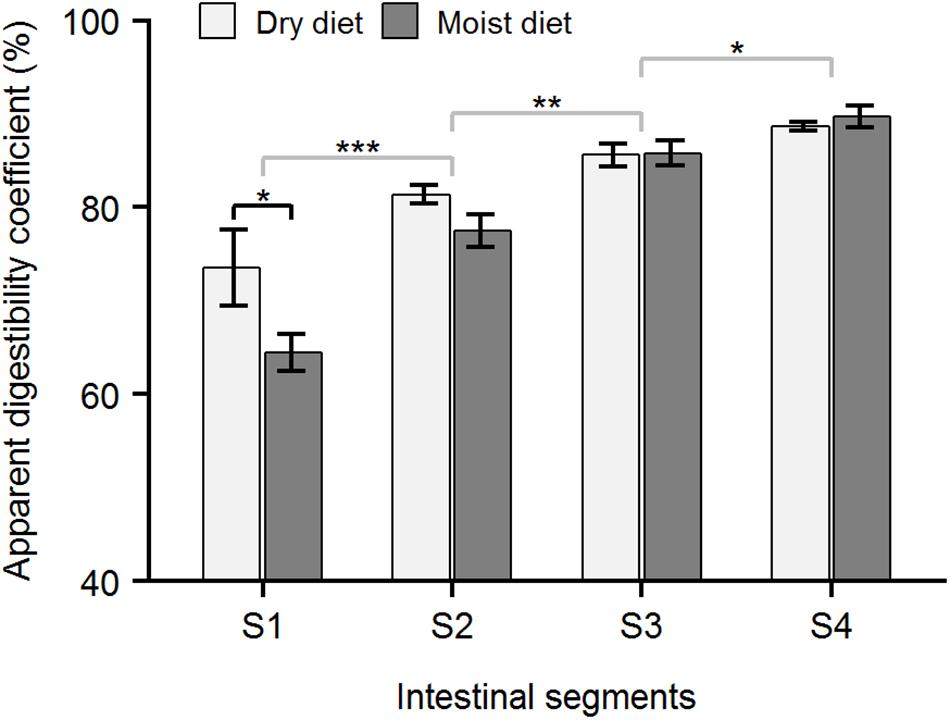
Figure 6. Apparent digestibility coefficient (ADC) of protein in ballan wrasse intestine. The figure shows the ADC (%) of protein in the 4 intestinal segments (S1–S4) of fish fed with dry or moist diets. Asterisk’s (∗) above horizontal square brackets indicate significant differences (glmmPQL test in R) between treatments (black line) or between adjacent intestinal segments (gray lines). ∗∗∗p < 0.001, ∗∗p < 0.01, ∗p < 0.05. Data are mean ± SEM, n = 6.
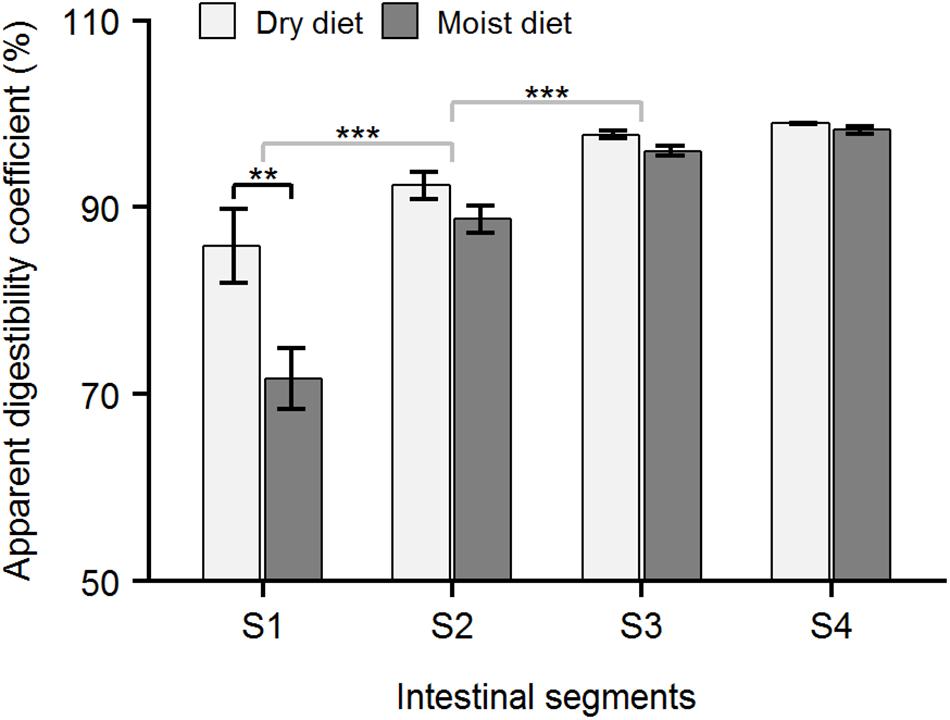
Figure 7. Apparent digestibility coefficient (ADC) of carbohydrate in ballan wrasse intestine. The figure shows the ADC (%) of carbohydrate in the 4 intestinal segments (S1–S4) of ballan wrasse fed with dry or moist diets. Asterisk’s (∗) above horizontal square brackets indicate significant differences (glmmPQL test in R) between treatments (black line) or between adjacent intestinal segments (gray lines). ∗∗∗p < 0.001, ∗∗p < 0.01. Data are mean ± SEM, n = 6.
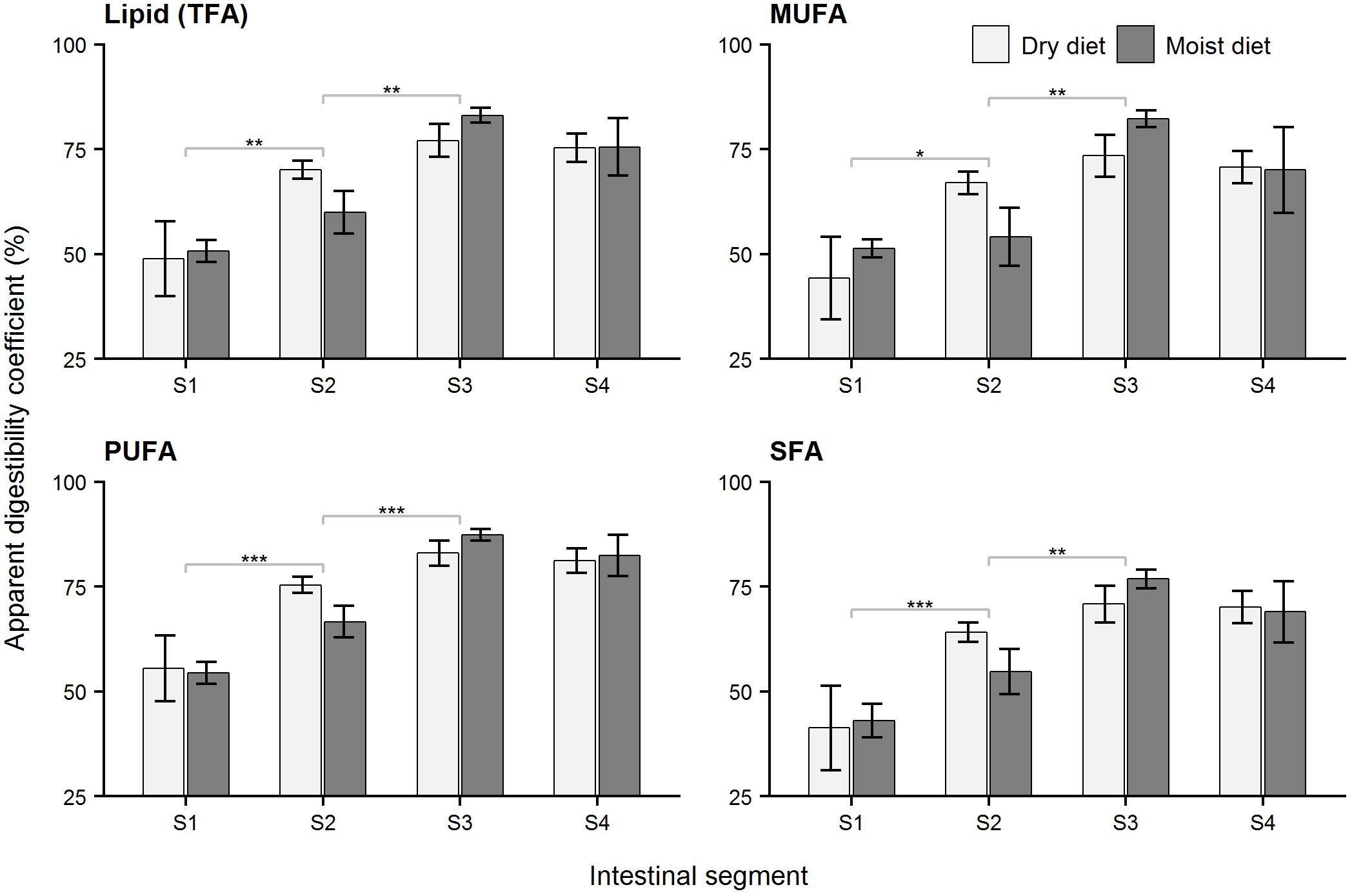
Figure 8. Apparent digestibility coefficient (ADC) of lipid in ballan wrasse intestine. The figures show ADC (%) of lipid (total fatty acids), TFA; monounsaturated fatty acids, MUFA; polyunsaturated fatty acids, PUFA; and saturated fatty acids, SFA in the 4 intestinal segments (S1–S4) of ballan wrasse fed dry or moist diets. Asterisk’s (∗) above gray horizontal square bracket indicate significant differences between adjacent segments (glmmPQL test in R). ∗∗∗p < 0.001, ∗∗p < 0.01, ∗p < 0.05. No significant differences occurred in fatty acid ADCs within intestinal segments between fish fed dry and moist diets. Data are mean ± SEM, n = 6.
Nutrient Markers in Plasma
No statistically significant differences were observed for plasma concentrations of cholesterol, free fatty acid, glucose, triacylglycerides or protein between fish fed dry or moist diets (Figure 9).
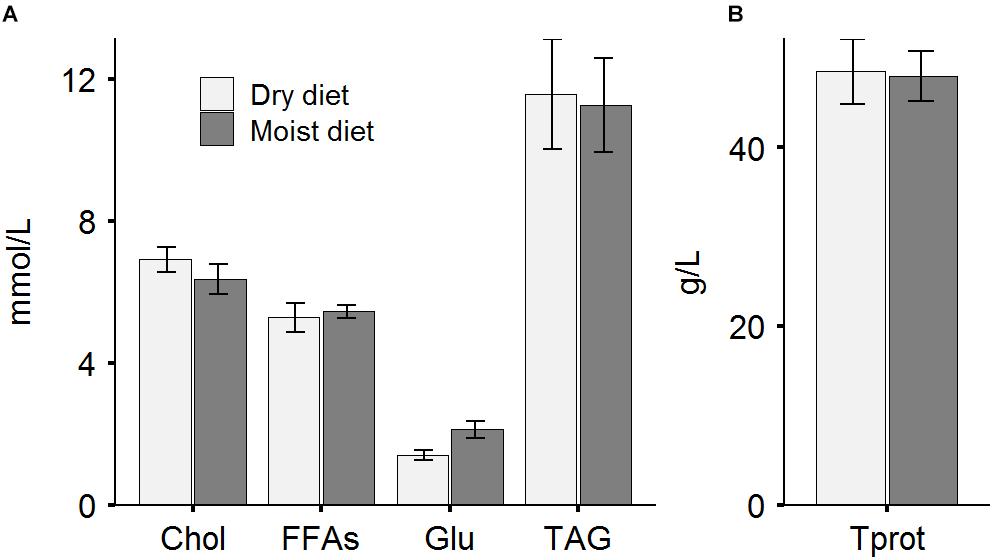
Figure 9. Plasma concentrations of metabolites. (A) The levels of cholesterol, Chol; free fatty acids, FFAs; glucose, Glu; total triacylglycerides, TAG; and (B) total protein, Tprot in plasma collected from ballan wrasse fed dry or moist diets. Data are mean ± SEM (n = 9 or 12 for dry or moist diets, respectively).
Transcriptomic Effects of Dry and Moist Diets
The transcriptomic expressions of Segments 1 and 4 were analyzed in the two feeding groups. According to the DESeq2 analysis, 59 genes were differentially expressed (DE) (q < 0.1) between the two treatments in Segment 1 (Supplementary Table S1) 26 genes in Segment 4 (Supplementary Table S2). However, none of the DE genes in Segment 1 were overlapping with the DE genes of Segment 4 (Figure 10).
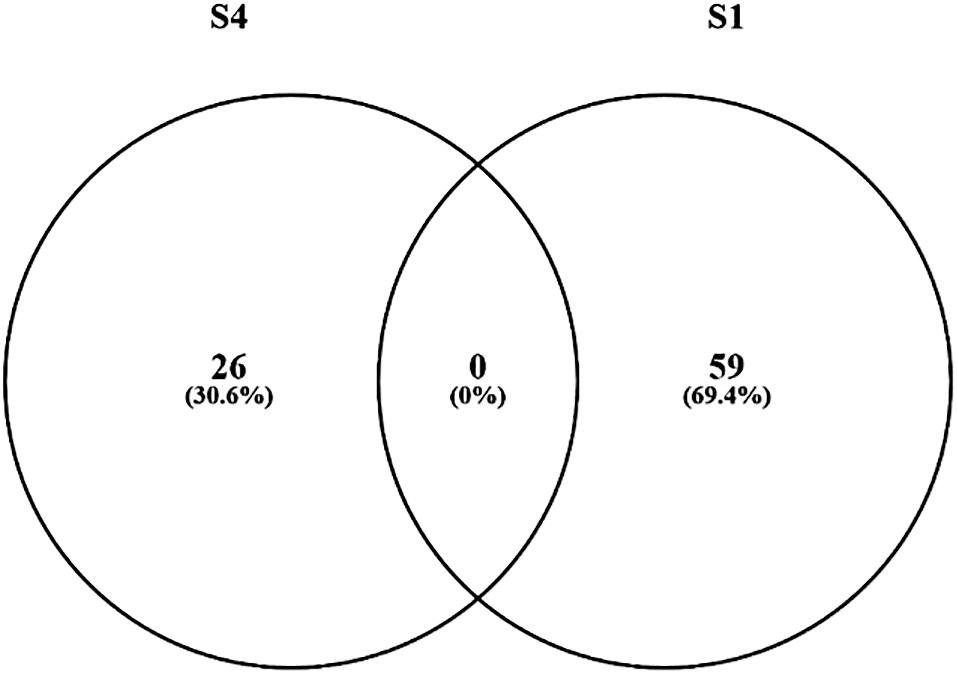
Figure 10. Diets induce changes in gene expression in ballan wrasse intestine. The figure shows the Venn diagram of the DE genes in Segment 1 (S1) and Segment 4 (S4) in the two treatments (moist and dry diets).
Of DE genes in Segment 1, 24 were increased and 25 were reduced in fish in the moist diet treatment compared to fish in the dry diet treatment (Supplementary Table S1). Downstream analysis of Segment 1 DE genes revealed several enriched pathways of which genes related to cholesterol biosynthetic process were enriched by the moist diet (Figure 11). No enriched pathways (FDR > 10%) were observed following pathway analysis using the DAVID Functional Annotation Tool (version 6.8).
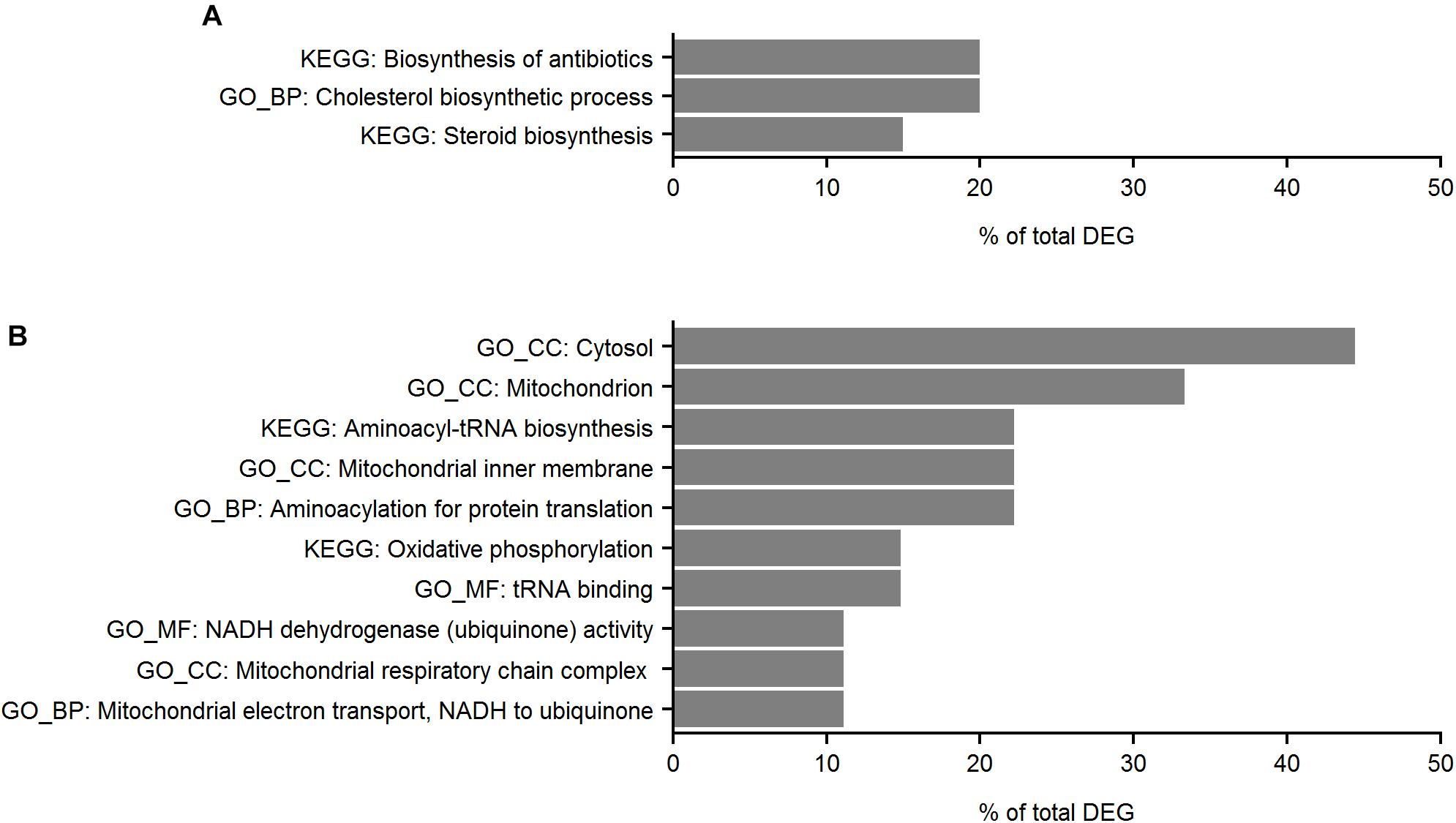
Figure 11. Functional analysis of differentially expressed genes (DEG) enriched in the Segment 1 of wrasse intestine. (A) Moist diet; (B) dry diet. The graph shows the percentages of total DEG present in each of the KEGG and Gene Ontology (GO) categories. For the GOs, biological process (BP), cellular compartment (CC) and molecular function (MF) of the genes were considered in the analysis. For the enrichment analysis FDR < 5% was used as the significant threshold.
Nine aquaporin genes (Aqp1, 3, 4, 7, 8, 9, 10, 11, and 12) were identified in intestinal tissue of ballan wrasse. Aqp1, 8, and 10b had an opposite expression pattern, with relatively low expression in Segment 1 followed by higher levels of expression in more distally located segment/s (Figure 12). Aqp11 and Aqp10a were highly expressed in Segment 1 with declining expression levels toward the distal part of the intestine. The expression of the remaining Aqp genes did not differ throughout the intestine. Sodium–dependent glucose cotransporter 1 (Sglt1) gene was highly expressed in three proximal segments with lower expression in the last segment (Figure 12). The expression of nine Aqp genes and Sglt1 had no difference between moist and dry diet treatments.
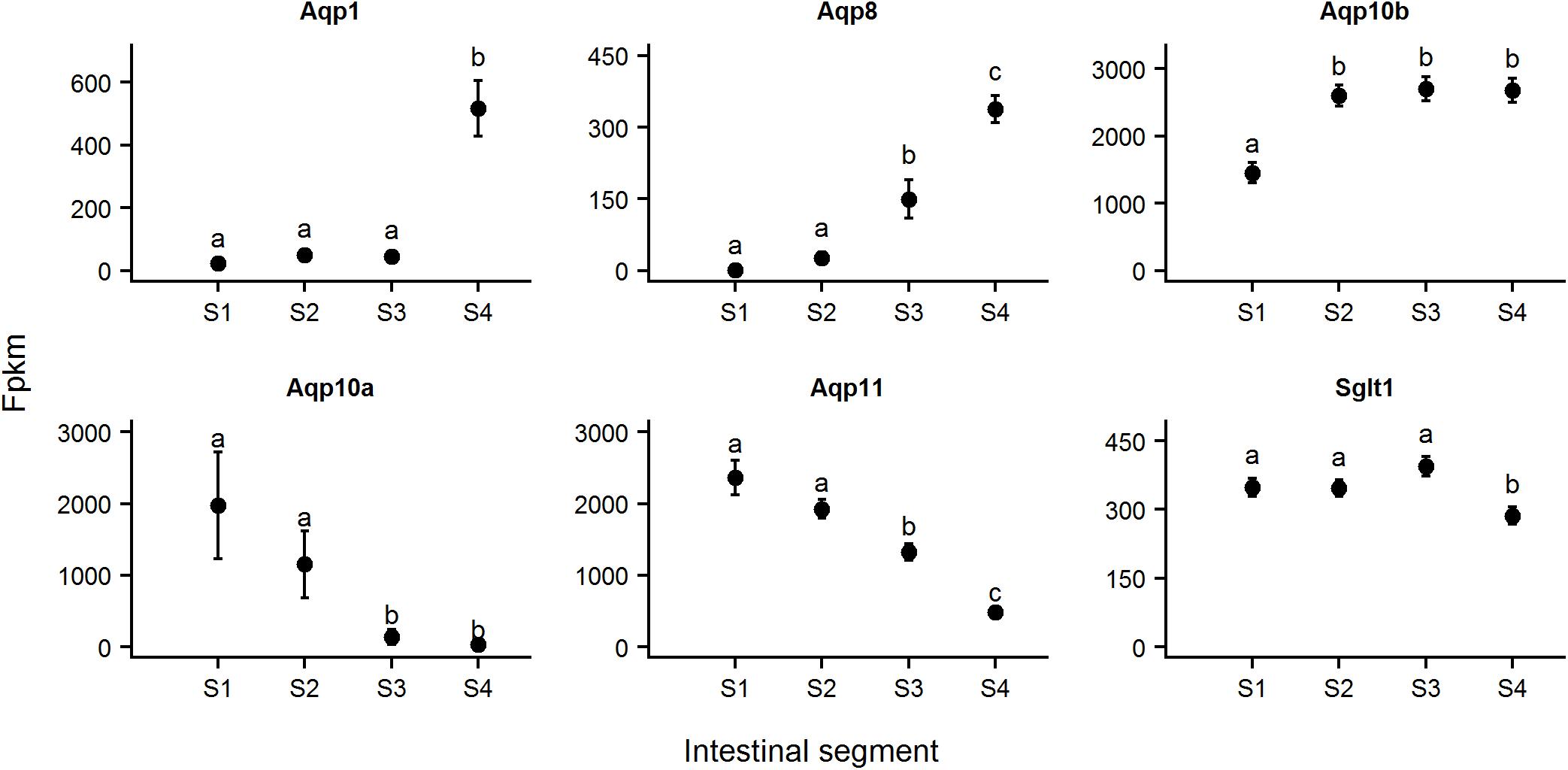
Figure 12. Expression of Aquaporins (Aqp) and sodium–dependent glucose cotransporter 1 (Sglt1) genes. The graphs show the expression of these genes in tissues from four intestinal segments (S1–S4) of ballan wrasse. Fpkm, fragments per kilobase million. Different letters denote significant differences between intestinal segments (p < 0.05).
Discussion
Intestinal Health
Wild fish have evolved consuming prey with high moisture contents, whereas cultured fish are generally fed on commercial pellets with low water contents (Buddington et al., 1997). Thus, it is possible that dry hard pellets may physically interact with and damage the epithelial layers of the gut wall (Hedrera et al., 2013; Couto et al., 2015). Earlier reports on gut health in ballan wrasse described severe inflammations in the epithelial tissues [see Krogdahl et al. (2014) in Leppeprod report]. It was hypothesized that the dry and hard feed pellets used in intensive farming triggered irritations and inflammations. However, in the current study severe intestinal inflammation was not found, nor did diet moisture level has an effect on overall intestinal inflammatory status. Most fish ate the pellets immediately after they were introduced into the tank, but a “docile” species like the wrasse will also feed from the bottom of the tank. Pellets on the tank bottom will be soaked like the moist diet, eliminating the difference in dietary treatment. However, these feeding conditions were the same as for the fish previously observed with intestinal inflammations. It is therefore most likely that previous observed intestinal inflammations were caused by other factors than the hardness of the feed pellets.
Gut Evacuation in the Agastric Fish, Ballan Wrasse
Short feeding intervals and large meals increase the rate of gut evacuation (Grove et al., 1978; Flowerdew and Grove, 1979; Jobling, 1981; Garber, 1983; Lee et al., 2000). The “gastric” evacuation time (time for >90% digesta propelled out of the anterior bulbous – Segment1 to next intestinal sections) in ballan wrasse in the current study was of ∼4 h, which was relatively faster than other species, despite relatively low feed intakes [∼0.1% body weight in ballan wrasse, compared to ∼1% body weight in salmon (Aas et al., 2017)]. For instance, the gastric emptying time (GET) for clownfish (Amphiprion ocellaris) fed pellets to satiation is 36 h (Ling and Ghaffar, 2014). Atlantic salmon force fed a single meal of 10 mg/g body weight needed 24 h to evacuate 92–99% of the feed from the stomach to the intestine (Aas et al., 2017). The fast evacuation time in ballan wrasse may be due to the short feeding intervals (10 min) and/or the continuous light regime, which might force wrasse to eat continuously. Increasing feeding frequency can result in faster gut evacuation rates, as a previous meal may physically push the digesta along the gastrointestinal tract. For example, Nile tilapia (Oreochromis niloticus) fed at 2–3 h intervals had a faster evacuation rate compared to those fed at 4 h intervals (Riche et al., 2004). A shorter gut transit time due to higher feeding frequencies has also been reported in other gastric fish species, such as; rainbow trout (Grove et al., 1978), Korean rockfish (Lee et al., 2000), Atlantic cod (Gadus morhua) (Tyler, 1970), common dab (Limanda limanda) (Gwyther and Grove, 1981); and several agastric; shanny (Lipophrys pholis) (Grove and Crawford, 1980), rare minnow (Gobiocypris rarus) (Wu et al., 2015). To summon up; no effect of dietary moisture level on the passage rate of digesta through different segments, or over the entire length, of the gastrointestinal tract were found the current study. Segment 1 only took 4–8 h to transfer >90% of the digesta (as indicated by the replacement of Y-labeled feed to Yb-labeled) to Segment 2. This indicates that Segment 1, also referred to as the bulbous, plays only a minor, if any, role as a temporary food storage area in ballan wrasse.
We hypothesized, based on pilot studies, that moistening of the pellets prior to feeding would increase digesta moisture levels in the first intestinal segment, and that the dry matter of digesta would decrease by Segment 3 or 4 as seen in European sea bass (Nikolopoulou et al., 2011). However, moisture levels of digesta were not different, nor did water content change in the last intestinal segments, in ballan wrasse fed either dry or moist diet. The missing difference in digesta moisture in the Segment 1 is probably due to a large variation when the different fish ate the pellets. Only fish that ate relatively soon after the dry pellets were introduced to the tank actually ate dry diet. However, a lot of fish were observed to eat pellets from the bottom of the tanks, pellets that would be moistened by the time they were eaten.
In addition to the functions of digestion and absorption, the intestine is also pivotal in water and electrolyte balance (Buddington et al., 1997). For osmoregulation, marine fish drink seawater, active uptake of NaCl in the esophagus and intestine passively co-transports water, excess NaCl is excreted via the gills (Whittamore, 2012). Like this teleost effectively absorbs water in the intestine to compensate for water losses to the hyperosmotic marine environment. In gastric fish, drinking is reduced when the stomach is distended by eating (Takei et al., 1998). However, it is not known how this is regulated in agastric fish. As feed were observed in small quantities in all intestinal segments in most of the fish analyzed in the current work, we hypothesize that this fish eats more or less continuously and have a similar drinking pattern. Thus, a high drinking rate together with frequent eating may play the dominating role for digesta moisture levels diminishing the effects of feed moisture levels. At the cellular level, the stability of digesta water content throughout the wrasse intestine might be regulated by the absorption of water involving aquaporins.
Aquaporins (Aqp), of which 17 isoforms have been identified in fish (Whittamore, 2012; Finn et al., 2014), are cell membrane proteins that function as channels for passive transport of H2O and/or small organic molecules via transmembrane osmotic gradients (Matsuzaki et al., 2004; Finn and Cerdà, 2011; Madsen et al., 2015). The dominant expression of Aqp10a and Aqp11 in Segment 1 may be associated with the major digestion and absorption of macronutrients of this section. In some fish species, including the wrasses, triglycerides (TG) are completely hydrolyzed during digestion to free fatty acids and a free glycerol molecule in the intestinal lumen (Lie et al., 2018; Sæle et al., 2018). We hypothesize that Aqp10a, which is permeable to glycerol (Madsen et al., 2015), may be involved in glycerol absorption to the enterocytes. With the low permeability for water (Yakata et al., 2011), Aqp11 may support the transportation of small organic molecules rather than water uptake in the intestine. Water might be mainly absorbed in the posterior intestinal segments since the increasing expressions of Aqp1 and Aqp10b; those Aqps, in mammals, have been known as “true aquaporins” and have high permeability to water (Madsen et al., 2015). The high expression of Aqp8 in the hindgut suggests this Aqp is involved in the excretion of ammonia in the hindgut, as Aqp8 allows NH3 to permeate through it channel on the intestinal wall (Saparov et al., 2007). The presence of the “true aquaporins” Apq1, -4 and -10b, and sodium-glucose cotransporters 1 (Sglt1) in the intestinal tracts of wrasse may have contributed to maintaining the stable digesta moisture levels we observed in the wrasse irrespective of whether dry or moist diet was fed. The Sglt1 may also play a role in active water absorption along the teleost gut (Whittamore, 2012) and its gene was constituently expressed along the length of the wrasse intestine. Furthermore, dry diet that is not eaten immediately will moisten in the water, decreasing any difference between moisture levels between dry and pre-soaked feeds prior to ingestion.
Digestion and Absorption of Macronutrients
Apparent digestibility coefficients demonstrated the availability of energy and nutrients in a diet for a given species, and thus facilitate formulation of diets that satisfy the nutritional requirements of a species (Bureau et al., 1999). Increased feed moisture levels can increase (Grove et al., 2001; Baeverfjord et al., 2006), decrease (Adamidou et al., 2009; Venou et al., 2009), or have no affect (Aas et al., 2011; Oehme et al., 2014) on nutrient digestibility. Feed moisture level-induced changes in gastrointestinal transit of digesta can affect the digestibility of proteins and lipids (Adamidou et al., 2009; Venou et al., 2009). In the present study, fish fed moist diet had lower ADCs for protein and carbohydrates than those fed dry diet in Segment 1, however, this difference was evened out in subsequent segments.
Our data demonstrated that ballan wrasse, despite the lack of stomach and pyloric caeca, is able to digest and absorb efficiently protein from high protein diets. In gastric fishes, the ADC of protein in Senegalese sole (Solea senegalensis) is ∼94% for ∼55% protein diet (Dias et al., 2010), in Atlantic halibut (Hippoglossus hippoglossus) is ∼83% for ∼41% protein diet (Mundheim et al., 2004; Hatlen et al., 2005), in Atlantic cod (Gadus morhua) is >92% for ∼44–60% protein diet (Tibbetts et al., 2006), and in haddock (Melanogrammus aeglefinus L.) is ∼73–95% for ∼44–51% protein diet (Tibbetts et al., 2004). Compared to gastric fishes, the ADC of protein at 90% for ∼50–61% protein feed in ballan wrasse is quite high. It illustrates that the stomach conditions of pepsin and low pH are not definitive requirement for the efficient digestion of protein.
Our data showed that, lipid digestibility increased with increasing degree of desaturation; higher unsaturated the fatty acids, more the ADC. This is in line with previous findings for differences in intestinal absorption of saturated and unsaturated fatty acids (Ockner et al., 1972; British Nutrition Foundation, 1992; Ramírez et al., 2001). However, the ADC of SFA and MUFA were similar in intestinal Segments 2 and 4, possibly due to the SFA being more efficiently absorbed in Segments 2 and 4 than Segments 1 and 3. Thus, the lower ADC of SFA than MUFA in Segments 1 and 3 was compensated with higher amount of SFA than MUFA that were absorbed in Segments 2 and 4.
Dietary lipid and amino acids, but not carbohydrate, are the principle source of energy for most fish, especially in carnivorous and omnivorous species (Dabrowski and Guderley, 2003). Modeling of data from a comprehensive feeding trial determined that maximum lengthwise growth of ballan wrasse would occur with diets containing, in the dry matter, 65% protein, 12% lipid, and 16% carbohydrate (Hamre et al., 2013). In the present study, the fish were fed diets with 6% carbohydrate which was lower than the optimal level found by Hamre et al. (2013). Carbohydrate ADCs in carnivorous fish tends to be high (>90%) at low dietary carbohydrate (<10% dry matter) inclusions (Krogdahl et al., 2005). Thus, the low dietary carbohydrate level in the current study may explain why the ADCs for carbohydrate (∼98% in Segment 4) were higher than for protein (∼89% in Segment 4) or lipid (∼76% in Segment 4) in ballan wrasse.
Effect of Dry and Moist Diets on the Transcriptome
Very few (59) differentially expressed genes were observed in the intestine of moist versus dry diet fed wrasse. It has been known that expression of cholesterol biosynthetic genes is affected by dietary feed composition [e.g., replacement of fish meal or fish oil by plant-based ingredients resulted in the increase in expression of genes involved in cholesterol synthesis (Leaver et al., 2008; Kortner et al., 2013, 2014)]. In this study, we found that the moisture levels of feed also had an effect on genes related to cholesterol biosynthetic processes which were enriched in fish fed moist diet compared to those fed dry diet. However, no significant differences were observed in blood cholesterol values between the two groups. This suggests that despite small differences in expression of genes related to cholesterol biosynthesis, these diets composed of the same ingredients had no further effects on cholesterol homeostasis. Dry diet resulted in increased expression of genes related to mitochondrial activity and protein translation, but these changes cannot easily be linked to other measurements taken in the study. As discussed, digesta moisture contents were similar between treatments, so it is not surprising that few differences in gene expression were observed. In accordance with the histological evaluation, we observed no apparent diet effect on immune- and inflammatory related gene expression.
Conclusion
The anterior segment of the ballan wrasse intestine does not appear to function as a food storage region, but it does play a key role in macronutrient digestion. Even though the experimental design in the present study did allow for some moist pellet ingestion in the dry pellet group, moisture levels did affect the speed of protein and carbohydrate digestion and absorption (ADC) in the first intestinal segment where nutrient absorption were highest. However, the higher digestibility of carbohydrate and protein in the first intestinal segment was later compensated for by the following intestinal segments. Lipid digestibility was not affected by feed moisture levels. The macronutrients were digested and absorbed along the whole intestinal tract but mainly in the first segment. The dry diet did not damage the gut tissue or negatively affect fish health.
Data Availability
The dataset for this study can be found in European Nucleotide Archive and the accession number is PRJEB13687.
Author Contributions
ØS, ÅK, TK, IL, and KK: planning and preparing experiments. IL, KK, and ØS: participated in carrying out experiments. HL, XS, ÅK, TK, KL, and ØS: analysis. HL, XS, ÅK, TK, IL, KK, KL, and ØS: writing and editing.
Funding
This work was funded by The Research Council of Norway (Project; Intestinal function and health ballan wrasse. Grant No. 244170). The funders had no role in study design, data collection and analysis, decision to publish, or preparation of the manuscript. The commercial company Nofima AS did not have role in funding for this study. The roles of authors (IL and KK) working in Nofima AS have been described in the author contributions section.
Conflict of Interest Statement
The authors declare that the research was conducted in the absence of any commercial or financial relationships that could be construed as a potential conflict of interest.
Acknowledgments
We thank Bashir Abdulkader, Edel Erdal, Berit Solli, and Jacob Wessels for support in chemical analysis and Knut Helge Jensen for his help in data analysis.
Supplementary Material
The Supplementary Material for this article can be found online at: https://www.frontiersin.org/articles/10.3389/fmars.2019.00140/full#supplementary-material
Footnotes
- ^www.sequencing.uio.no
- ^https://github.com/najoshi/sickle
- ^http://www.bioinformatics.babraham.ac.uk/projects/fastqc/
- ^http://www.ebi.ac.uk/ena/data/view/PRJEB13687
- ^http://subread.sourceforge.net/
References
Aas, T. S., Sixten, H. J., Hillestad, M., Sveier, H., Ytrestøyl, T., Hatlen, B., et al. (2017). Measurement of gastrointestinal passage rate in Atlantic salmon (Salmo salar) fed dry or soaked feed. Aquac. Rep. 8(Suppl. C), 49–57. doi: 10.1016/j.aqrep.2017.10.001
Aas, T. S., Terjesen, B. F., Sigholt, T., Hillestad, M., Holm, J., Refstie, S., et al. (2011). Nutritional responses in rainbow trout (Oncorhynchus mykiss) fed diets with different physical qualities at stable or variable environmental conditions. Aquac. Nutr. 17, 657–670. doi: 10.1111/j.1365-2095.2011.00868.x
Aas, T. S., Ytrestøyl, T., Hatlen, B., Sixten, H. J., Hillestad, M., and Åsgård, T. (2013). Gastrointestinal passage rate in Atlantic salmon (Salmo salar) Fed Dry or Soaked Feed. A CREATE Project. Romsø: Nofima.
Adamidou, S., Nengas, I., Alexis, M., Foundoulaki, E., Nikolopoulou, D., Campbell, P., et al. (2009). Apparent nutrient digestibility and gastrointestinal evacuation time in European seabass (Dicentrarchus labrax) fed diets containing different levels of legumes. Aquaculture 289, 106–112. doi: 10.1016/j.aquaculture.2009.01.015
Artüz, M. L. (2019). Feeding accustomation of the ballan wrasse in the Sea of Marmara. Hidrobiologica 2004/10, 17–19.
Baeverfjord, G., and Krogdahl, A. (1996). Development and regression of soybean meal induced enteritis in Atlantic salmon, Salmo salar L., distal intestine: a comparison with the intestines of fasted fish. J. Fish Dis. 19, 375–387. doi: 10.1046/j.1365-2761.1996.d01-92.x
Baeverfjord, G., Refstie, S., Krogedal, P., and Åsgård, T. (2006). Low feed pellet water stability and fluctuating water salinity cause separation and accumulation of dietary oil in the stomach of rainbow trout (Oncorhynchus mykiss). Aquaculture 261, 1335–1345. doi: 10.1016/j.aquaculture.2006.08.033
Barrington, E. J. W. (1942). Gastric digestion in the lower vertebrates. Biol. Rev. 17, 1–27. doi: 10.1111/j.1469-185X.1942.tb00429.x
British Nutrition Foundation (1992). Unsaturated Fatty Acids: Nutritional and Physiological Significance: The Report of the British Nutrition Foundation’s Task Force, (Boston, MA: Springer), 24–27.
Bogevik, A. S., Kousoulaki, K., Skiftesvik, A. B., and Opstad, I. (2016). Fishmeal quality and ethoxyquin effects on the weaning performance of ballan wrasse (Labrus bergylta) larvae. Aquac. Nutr. 22, 46–50. doi: 10.1111/anu.12225
Buddington, R. K., Krogdahl, A., and Bakke-Mckellep, A. M. (1997). The intestines of carnivorous fish: structure and functions and the relations with diet. Acta Physiol. Scand. Suppl. 638, 67–80.
Bureau, D. P., Harris, A. M., and Cho, C. Y. (1999). Apparent digestibility of rendered animal protein ingredients for rainbow trout (Oncorhynchus mykiss). Aquaculture 180, 345–358. doi: 10.1016/S0044-8486(99)00210-0
Castro, L. F. C., Gonçalves, O., Mazan, S., Tay, B.-H., Venkatesh, B., and Wilson, J. M. (2014). Recurrent gene loss correlates with the evolution of stomach phenotypes in gnathostome history. Proc. Biol. Sci. 281:20132669. doi: 10.1098/rspb.2013.2669
Couto, A., Kortner, T. M., Penn, M., Bakke, A. M., Krogdahl,Å, and Oliva-Teles, A. (2015). Dietary saponins and phytosterols do not affect growth, intestinal morphology and immune response of on-growing European sea bass (Dicentrarchus labrax). Aquac. Nutr. 21, 970–982. doi: 10.1111/anu.12220
Dabrowski, K., and Guderley, H. (2003). “6 - Intermediary metabolism A2,” in Fish Nutrition, 3rd Edn, eds J. E. Halver, and R. W. Hardy (San Diego, CA: Academic Press), 309–365.
Deady, S., and Fives, J. M. (1995). Diet of ballan wrasse, Labrus bergylta, and some comparisons with the diet of corkwing wrasse, Crenilabrus melops. J. Mar. Biol. Assoc. U.K. 75, 651–665. doi: 10.1017/S0025315400039072
Dias, J., Yúfera, M., Valente, L. M. P., and Rema, P. (2010). Feed transit and apparent protein, phosphorus and energy digestibility of practical feed ingredients by Senegalese sole (Solea senegalensis). Aquaculture 302, 94–99. doi: 10.1016/j.aquaculture.2010.02.013
Figueiredo, M., Morato, T., Barreiros, J. P., Afonso, P., and Santos, R. S. (2005). Feeding ecology of the white seabream, Diplodus sargus, and the ballan wrasse, Labrus bergylta, in the Azores. Fish. Res. 75, 107–119. doi: 10.1016/j.fishres.2005.04.013
Finn, R. N., and Cerdà, J. (2011). Aquaporin evolution in fishes. Front. Physiol. 2:44. doi: 10.3389/fphys.2011.00044
Finn, R. N., Chauvigné, F., Hlidberg, J. B., Cutler, C. P., and Cerdà, J. (2014). The lineage-specific evolution of aquaporin gene clusters facilitated tetrapod terrestrial adaptation. PLoS One 9:e113686. doi: 10.1371/journal.pone.0113686
Flowerdew, M. W., and Grove, D. J. (1979). Some observations of the effects of body weight, temperature, meal size and quality on gastric emptying time in the turbot, Scophthalmus maximus (L.) using radiography. J. Fish Biol. 14, 229–238. doi: 10.1111/j.1095-8649.1979.tb03514.x
Garber, K. J. (1983). Effect of fish size, meal size and dietary moisture on gastric evacuation of pelleted diets by yellow perch, Perca flavescens. Aquaculture 34, 41–49. doi: 10.1016/0044-8486(83)90290-9
Grove, D., Genna, R., Paralika, V., Boraston, J., Hornyold, M. G., and Siemens, R. (2001). Effects of dietary water content on meal size, daily food intake, digestion and growth in turbot, Scophthalmus maximus (L.). Aquac. Res. 32, 433–442. doi: 10.1046/j.1365-2109.2001.00585.x
Grove, D. J., and Crawford, C. (1980). Correlation between digestion rate and feeding frequency in the stomachless teleost, Blennius pholis L. J. Fish Biol. 16, 235–247. doi: 10.1111/j.1095-8649.1980.tb03701.x
Grove, D. J., Loizides, L. G., and Nott, J. (1978). Satiation amount, frequency of feeding and gastric emptying rate in Salmo gairdneri. J. Fish Biol. 12, 507–516. doi: 10.1111/j.1095-8649.1978.tb04195.x
Guillaume, J., and Choubert, G. (2001). “4 Digestive physiology and nutrient digestibility in fishes,” in Nutrition and Feeding of Fish and Crustaceans, eds J. Guillaume and P. Publishing (Berlin: Springer).
Gwyther, D., and Grove, D. J. (1981). Gastric emptying in Limanda limanda (L.) and the return of appetite. J. Fish Biol. 18, 245–259. doi: 10.1111/j.1095-8649.1981.tb03767.x
Hamre, K., Nordgreen, A., Grøtan, E., and Breck, O. (2013). A holistic approach to development of diets for Ballan wrasse (Labrus berggylta) – a new species in aquaculture. PeerJ 1:e99. doi: 10.7717/peerj.99
Hatlen, B., Grisdale-Helland, B., and Helland, S. J. (2005). Growth, feed utilization and body composition in two size groups of Atlantic halibut (Hippoglossus hippoglossus) fed diets differing in protein and carbohydrate content. Aquaculture 249, 401–408. doi: 10.1016/j.aquaculture.2005.03.040
Hedrera, M. I., Galdames, J. A., Jimenez-Reyes, M. F., Reyes, A. E., Avendaño-Herrera, R., Romero, J., et al. (2013). Soybean meal induces intestinal inflammation in zebrafish larvae. PLoS One 8:e69983. doi: 10.1371/journal.pone.0069983
Hemre, G.-I., Lie,Ø., Lied, E., and Lambertsen, G. (1989). Starch as an energy source in feed for cod (Gadus morhua): Digestibility and retention. Aquaculture 80, 261–270. doi: 10.1016/0044-8486(89)90174-9
Jobling, M. (1981). Dietary digestibility and the influence of food components on gastric evacuation in plaice, Pleuronectes platessa L. J. Fish Biol. 19, 29–36. doi: 10.1111/j.1095-8649.1981.tb05808.x
Jobling, M. (1987). Influences of food particle size and dietary energy content on patterns of gastric evacuation in fish: test of a physiological model of gastric emptying. J. Fish Biol. 30, 299–314. doi: 10.1111/j.1095-8649.1987.tb05754.x
Kapoor, B. G., Smit, H., and Verighina, I. A. (1976). “The alimentary canal and digestion in teleosts,” in Advances in Marine Biology, eds S. R. Frederick and Y. Maurice (Cambridge, MA: Academic Press), 109–239.
Kim, K.-D., Kim, K.-W., Kang, Y.-J., Son, M.-H., and Lee, S.-M. (2011). Effects of the dietary moisture levels and feeding rate on the growth and gastric evacuation of young olive flounder Paralichthys olivaceus. Fish Aquat. Sci. 14, 105–110. doi: 10.5657/FAS.2011.0105
Koelz, H. R. (1992). Gastric acid in vertebrates. Scand. J. Gastroenterol. 27, 2–6. doi: 10.3109/00365529209095998
Kortner, T. M., Björkhem, I., Krasnov, A., Timmerhaus, G., and Krogdahl, Å (2014). Dietary cholesterol supplementation to a plant-based diet suppresses the complete pathway of cholesterol synthesis and induces bile acid production in Atlantic salmon (Salmo salar L.). Br. J. Nutr. 111, 2089–2103. doi: 10.1017/S0007114514000373
Kortner, T. M., Gu, J., Krogdahl,Å, and Bakke, A. M. (2013). Transcriptional regulation of cholesterol and bile acid metabolism after dietary soyabean meal treatment in Atlantic salmon (Salmo salar L.). Br. J. Nutr. 109, 593–604. doi: 10.1017/S0007114512002024
Kousoulaki, K., Bogevik, A. S., Skiftesvik, A. B., Jensen, P. A., and Opstad, I. (2015). Marine raw material choice, quality and weaning performance of Ballan wrasse (Labrus bergylta) larvae. Aquac. Nutr. 21, 644–654. doi: 10.1111/anu.12186
Kousoulaki, K., Grøtan, E., Kvalheim, K., Høstmark,Ø., Klinge, M., and Bogevik, A. (2014). “Protein quality, commodity options and ballan wrasse weaning performance,” in Proceedings of the Production of Ballan Wrasse-Science and Practice, Tromsø.
Krogdahl,Å., Hemre, G. I., and Mommsen, T. P. (2005). Carbohydrates in fish nutrition: digestion and absorption in postlarval stages. Aquac. Nutr. 11, 103–122. doi: 10.1111/j.1365-2095.2004.00327.x
Krogdahl,Å., Sæle,Ø., Lie, K., Kousoulaki, K., Hamre, K., Helland, S., et al. (2014). “Characteristics of the digestive functions in ballan wrasse fed dry and moist diets-production of ballan wrasse,” in Proceedings of the Production of Ballan Wrasse-Science and Practice, Tromsø.
Langmead, B., Trapnell, C., Pop, M., and Salzberg, S. L. (2009). Ultrafast and memory-efficient alignment of short DNA sequences to the human genome. Genome Biol. 10:R25. doi: 10.1186/gb-2009-10-3-r25
Leaver, M. J., Villeneuve, L. A., Obach, A., Jensen, L., Bron, J. E., Tocher, D. R., et al. (2008). Functional genomics reveals increases in cholesterol biosynthetic genes and highly unsaturated fatty acid biosynthesis after dietary substitution of fish oil with vegetable oils in Atlantic salmon (Salmo salar). BMC Genomics 9:299. doi: 10.1186/1471-2164-9-299
Lee, S.-M., Hwang, U.-G., and Cho, S. H. (2000). Effects of feeding frequency and dietary moisture content on growth, body composition and gastric evacuation of juvenile Korean rockfish (Sebastes schlegeli). Aquaculture 187, 399–409. doi: 10.1016/S0044-8486(00)00318-5
Lie, K. K., Tørresen, O. K., Solbakken, M. H., Rønnestad, I., Tooming-Klunderud, A., Nederbragt, A. J., et al. (2018). Loss of stomach, loss of appetite? Sequencing of the ballan wrasse (Labrus bergylta) genome and intestinal transcriptomic profiling illuminate the evolution of loss of stomach function in fish. BMC Genomics 19:186. doi: 10.1186/s12864-018-4570-8
Lie, O., and Lambertsen, G. (1991). Fatty acid composition of glycerophospholipids in seven tissues of cod (Gadus morhua), determined by combined high-performance liquid chromatography and gas chromatography. J. Chromatogr. 565, 119–129. doi: 10.1016/0378-4347(91)80376-N
Ling, K. M., and Ghaffar, M. A. (2014). Estimation of gastric emptying time (GET) in clownfish (Amphiprion ocellaris) using X-radiography technique. AIP Conf. Proc. 1614, 624–628. doi: 10.1063/1.4895275
Love, M. I., Huber, W., and Anders, S. (2014). Moderated estimation of fold change and dispersion for RNA-seq data with DESeq2. Genome Biol. 15, 550–550. doi: 10.1186/s13059-014-0550-8
Madsen, S. S., Engelund, M. B., and Cutler, C. P. (2015). Water transport and functional dynamics of aquaporins in osmoregulatory organs of fishes. Biol. Bull. 229, 70–92. doi: 10.1086/BBLv229n1p70
Martin, M. (2011). Cutadapt removes adapter sequences from high-throughput sequencing reads. EMBnetJ. 17, 10–12. doi: 10.14806/ej.17.1.200
Matsuzaki, T., Tajika, Y., Ablimit, A., Aoki, T., Hagiwara, H., and Takata, K. (2004). Aquaporins in the digestive system. Med. Electron. Microsc. 37, 71–80. doi: 10.1007/s00795-004-0246-3
Mundheim, H., Aksnes, A., and Hope, B. (2004). Growth, feed efficiency and digestibility in salmon (Salmo salar L.) fed different dietary proportions of vegetable protein sources in combination with two fish meal qualities. Aquaculture 237, 315–331. doi: 10.1016/j.aquaculture.2004.03.011
Nikolopoulou, D., Moutou, K. A., Fountoulaki, E., Venou, B., Adamidou, S., and Alexis, M. N. (2011). Patterns of gastric evacuation, digesta characteristics and pH changes along the gastrointestinal tract of gilthead sea bream (Sparus aurata L.) and European sea bass (Dicentrarchus labrax L.). Comp. Biochem. Physiol. A Mol. Integr. Physiol. 158, 406–414. doi: 10.1016/j.cbpa.2010.11.021
Ockner, R. K., Pittman, J. P., and Yager, J. L. (1972). Differences in the intestinal absorption of saturated and unsaturated long chain fatty acids. Gastroenterology 62, 981–992. doi: 10.1016/S0016-5085(72)80115-X
Oehme, M., Aas, T. S., Olsen, H. J., Sørensen, M., Hillestad, M., Li, Y., et al. (2014). Effects of dietary moisture content of extruded diets on physical feed quality and nutritional response in Atlantic salmon (Salmo salar). Aquac. Nutr. 20, 451–465. doi: 10.1111/anu.12099
Olsen, R. E., and Ringø, E. (1997). Lipid digestibility in fish: a review. Recent Res. Dev. Lipid Res. 1, 199–164.
Opuszynski, K., and Shireman, J. V. (1991). Food passage time and daily ration of bighead carp, Aristichthys nobilis, kept in cages. Environ. Biol. Fishes 30, 387–393. doi: 10.1007/bf02027982
Papadakis, I. E., Chatzifotis, S., Divanach, P., and Kentouri, M. (2008). Weaning of greater amberjack (Seriola dumerilii Risso 1810) juveniles from moist to dry pellet. Aquac. Int. 16, 13–25. doi: 10.1007/s10499-007-9118-x
Ramírez, M., Amate, L., and Gil, A. (2001). Absorption and distribution of dietary fatty acids from different sources. Early Hum. Dev. 65, S95–S101. doi: 10.1016/S0378-3782(01)00211-0
Riche, M., Haley, D. I., Oetker, M., Garbrecht, S., and Garling, D. L. (2004). Effect of feeding frequency on gastric evacuation and the return of appetite in tilapia Oreochromis niloticus (L.). Aquaculture 234, 657–673. doi: 10.1016/j.aquaculture.2003.12.012
Saparov, S. M., Liu, K., Agre, P., and Pohl, P. (2007). Fast and selective ammonia transport by Aquaporin-8. J. Biol. Chem. 282, 5296–5301. doi: 10.1074/jbc.M609343200
Skiftesvik, A. B., Durif, C. M. F., Bjelland, R. M., and Browman, H. I. (2014). Distribution and habitat preferences of five species of wrasse (Family Labridae) in a Norwegian fjord. ICES J. Mar. Sci. 72, 890–899. doi: 10.1093/icesjms/fsu211
Sæle,Ø., Rød, K. E. L., Quinlivan, V. H., Li, S., and Farber, S. A. (2018). A novel system to quantify intestinal lipid digestion and transport. Biochim. Biophys. Acta 1863, 948–957. doi: 10.1016/j.bbalip.2018.05.006
Takei, Y., Tsuchida, T., and Tanakadate, A. (1998). Evaluation of water intake in seawater adaptation in eels using a synchronized drop counter and pulse injector system. Zool. Sci. 15, 677–682. doi: 10.2108/zsj.15.677
Tibbetts, S. M., Lall, S. P., and Milley, J. E. (2004). Apparent digestibility of common feed ingredients by juvenile haddock, Melanogrammus aeglefinus L. Aquac. Res. 35, 643–651. doi: 10.1111/j.1365-2109.2004.01060.x
Tibbetts, S. M., Milley, J. E., and Lall, S. P. (2006). Apparent protein and energy digestibility of common and alternative feed ingredients by Atlantic cod, Gadus morhua (Linnaeus, 1758). Aquaculture 261, 1314–1327. doi: 10.1016/j.aquaculture.2006.08.052
Tyler, A. V. (1970). Rates of gastric emptying in young cod. J. Fish. Res. Board Can. 27, 1177–1189. doi: 10.1139/f70-140
Venero, J. A., Miles, R. D., and Chapman, F. A. (2015). Food transit time and site of absorption of nutrients in gulf of Mexico sturgeon. N. Am. J. Aquac. 77, 275–280. doi: 10.1080/15222055.2015.1017129
Venou, B., Alexis, M. N., Fountoulaki, E., and Haralabous, J. (2009). Performance factors, body composition and digestion characteristics of gilthead sea bream (Sparus aurata) fed pelleted or extruded diets. Aquac. Nutr. 15, 390–401. doi: 10.1111/j.1365-2095.2008.00603.x
Whittamore, J. M. (2012). Osmoregulation and epithelial water transport: lessons from the intestine of marine teleost fish. J. Comp. Physiol. B 182, 1–39. doi: 10.1007/s00360-011-0601-3
Wilson, J. M., and Castro, L. F. C. (2010). “1 - Morphological diversity of the gastrointestinal tract in fishes,” in Fish Physiology, eds A. P. F. Martin Grosell and J. B. Colin (Cambridge, MA: Academic Press), 1–55.
Wu, B., Luo, S., and Wang, J. (2015). Effects of temperature and feeding frequency on ingestion and growth for rare minnow. Physiol. Behav. 140(Suppl. C), 197–202. doi: 10.1016/j.physbeh.2014.12.034
Keywords: intestine, digestibility, evacuation rate, stomachless fish, aquaporin, wrasse, digestive physiology, transcriptome
Citation: Le HTMD, Shao X, Krogdahl Å, Kortner TM, Lein I, Kousoulaki K, Lie KK and Sæle Ø (2019) Intestinal Function of the Stomachless Fish, Ballan Wrasse (Labrus bergylta). Front. Mar. Sci. 6:140. doi: 10.3389/fmars.2019.00140
Received: 21 November 2018; Accepted: 05 March 2019;
Published: 26 March 2019.
Edited by:
Francesco Fazio, University of Messina, ItalyReviewed by:
Tiziano Verri, University of Salento, ItalyCarlos Rosas, National Autonomous University of Mexico, Mexico
Copyright © 2019 Le, Shao, Krogdahl, Kortner, Lein, Kousoulaki, Lie and Sæle. This is an open-access article distributed under the terms of the Creative Commons Attribution License (CC BY). The use, distribution or reproduction in other forums is permitted, provided the original author(s) and the copyright owner(s) are credited and that the original publication in this journal is cited, in accordance with accepted academic practice. No use, distribution or reproduction is permitted which does not comply with these terms.
*Correspondence: Hoang T. M. D. Le, aG9hbmcubGVAaGkubm8=