- 1Molecular Ecology of the Marine Benthos Group, Centre d’Estudis Avançats de Blanes, Consejo Superior de Investigaciones Científicas, Blanes, Spain
- 2Integrative Freshwater Ecology Group, Centre d’Estudis Avançats de Blanes, Consejo Superior de Investigaciones Científicas, Blanes, Spain
- 3Marine Invertebrate Phylogenetics Laboratory, Scripps Institution of Oceanography, La Jolla, CA, United States
Understanding community assembly and processes driving diversity in deep-sea environments is a major challenge in marine microbial ecology. The deep sea represents the largest ecosystem on Earth, but its remoteness makes the microbial community composition and functionality largely unknown. Moreover, microbial-focused studies comparing different deep-sea habitats like dynamic submarine canyons and slope ecosystems altogether are rare. The present work aims to study the deep-sea seafloor microbial communities (Bacteria and Archaea) of Blanes Canyon and its adjacent western open slope (NW Mediterranean) at ca. 1500 m deep, in autumn and spring, and along the vertical sediment profile. Microbial assemblages were studied in terms of abundance, diversity (α and β), community structure and functional potential through 16S rRNA tag-sequencing to assess their adaptations to the canyon’s idiosyncrasy. Furthermore, the relationships of microbes with environmental variables and a potential predator (nematodes) were also assessed. We observed the microbial assemblages and their predicted functional profiles to be more heterogeneous and with higher temporal variability in the canyon than in the open slope. Although their phyla composition was similar, both the dominant and richest phyla showed significant differences in proportion between canyon and slope. Bacterial diversities were higher in the canyon than in the open slope, together with nematode abundances. Along the vertical sediment profile, microbial abundances consistently decreased with depth in the open slope, while we found more variability within the canyon, linked to an enhancement of aerobic metabolisms in the most superficial sediment layer. Grain size was correlated with microbial abundances and explained part of the variability in the community structure. Nematode and microbial abundances were correlated in slope environments, while in the canyon phytodetritics inputs (Chl a and Chl a: phaeo) and organic carbon seemed to play a role in controlling microbial diversity and abundance. These results suggest that the deep-sea seafloor is strongly connected to coastal and pelagic productive areas through the canyon system in a stronger manner than to the open slope, thus modulating resource availability while driving changes in the microbial biosphere and the higher trophic levels of the deep-sea food web.
Introduction
The deep seafloor comprises a variety of dynamic habitats that highly contribute to the heterogeneity and diversity of biota and ecosystems on Earth (Stuart et al., 2003; Ramirez-Llodra et al., 2010). These habitats are generally characterized by low organic matter (OM) supply, with the food web depending ultimately on the photosynthetic production supply from the photic zone (Gage, 2003). Bacteria dominate these deep-sea environments in terms of abundance and biomass, playing a key role in the food web (Deming and Baross, 1993; Pfannkuche, 1993) and mobilizing buried nutrients through biogeochemical cycles (Berner, 1982). Indeed, deep-sea benthic microbial communities are generally more diverse than pelagic or open ocean surface waters (Zinger et al., 2011; Lindh et al., 2017). Still, studies in the marine realm mainly focused on pelagic ecosystems (e.g., Galand et al., 2010; Sunagawa et al., 2015; Sebastián et al., 2018). Large-scale patterns of deep-sea microbes seem to indicate that communities are vulnerable to temperature shifts, and very susceptible to changes in trophic characteristics and ‘food’ inputs (Danovaro et al., 2016). At the regional scale, submarine canyons are among the most pervasive and dynamic deep-sea habitats (Ramirez-Llodra et al., 2010) that play the role of preferential particle-transport conduits and therefore act as drivers of trophic characteristics. However, because of the difficulties in accessing the deep seafloor, there is a fragmented available knowledge regarding the processes ruling the microbial community composition in these systems (Barone et al., 2018; Rzeznik-Orignac et al., 2018).
Canyons drive shelf-slope exchange as they accelerate the particle transit from both productive coastal zones and inner shelf environments toward the deep seafloor (Allen and Durrieu de Madron, 2009; Puig et al., 2014). They can also enhance seasonal processes such as the upwelling of cold, nutrient-rich waters (Allen et al., 2001) or increase primary productions during spring and summer (Granata et al., 2004; Harris and Whiteway, 2011; Chauvet et al., 2018) and are also subject to seasonally variable disturbances caused by bottom currents, benthic storms, dense shelf water cascading events (Canals et al., 2006) and mass wasting events (De Stigter et al., 2007). Furthermore, particle fluxes, often enriched in OM, are funneled and sediment accumulation rates may even be of one order of magnitude higher than those in adjacent non-dissected margins at comparable depths in the north-western Mediterranean Sea, thus altering sediment vertical composition (Zúñiga et al., 2009; Pasqual et al., 2010; López-Fernández et al., 2013). Furthermore, anthropogenic activities like intensive bottom trawling along canyon flanks proved to impact canyon sedimentary regimes (Puig et al., 2014; Pusceddu et al., 2014). In the NW Mediterranean and, more specifically in Blanes Canyon, sediment rates substantially increased since the 1970s in parallel with the expansion of the trawling fleet (Paradis et al., 2018). Submarine canyons thus offer heterogeneous habitats that are proved to host highly diverse fauna at different spatial scales, which are subjected to complex natural and anthropogenic processes (Sardà et al., 2009; De Leo et al., 2014; Ismail et al., 2018). Despite the increasing research focusing over the last decades on canyon ecosystems and the associated environmental functioning, the microbial assemblages thriving under these circumstances are still being unveiled (Barone et al., 2018; Celussi et al., 2018; Rzeznik-Orignac et al., 2018), and their response to both canyons’ natural and anthropogenic processes remains poorly known. Remarkably, very few studies have aimed at evaluating how different sedimentation conditions (e.g., OM accumulation) may alter the microbial communities, the first compartment of benthic food web, along submarine canyons (Danovaro et al., 2000; Goffredi and Orphan, 2010). Nevertheless, higher bacterial (Danovaro et al., 1999) and fungal (Barone et al., 2018) biomasses have been reported along canyon axes in response to OM increases in sediments, while parallel information on associated changes in community composition remains scarce (Goffredi and Orphan, 2010; Barone et al., 2018).
Microbial-focused studies taking into account canyon and adjacent open slope ecosystems altogether are very rare (but see Polymenakou et al., 2009). Since microbial community idiosyncrasies in canyons and in their adjacent slopes may associate to differential seafloor dynamics, comparative studies may reveal effects along the whole food web. Specifically, patterns of association between individual canyon bacteria and nematodes have been reported (Rzeznik-Orignac et al., 2018) through predation (Moens and Vincx, 1997) or microbial commensalism (Moens et al., 2005). Indeed, organically enriched sediments in canyons support higher nematode densities, biomasses (Ingels et al., 2009; Leduc et al., 2014) and diversities (Bianchelli et al., 2013; Román, 2017; Bianchelli and Danovaro, 2019) than at the adjacent open slope. Moreover, seafloor surface and subsurface sediments also have distinct microbial (Bienhold et al., 2016; Lindh et al., 2017) and nematode distributions (Román et al., 2016), likely performing different processes with particular nutrient or oxygenation requirements. Therefore, since the functional and structural attributes of the benthic communities may result from the interaction of the multiple parts of the trophic food web (Leduc et al., 2014; Romano et al., 2016), a deeper understanding of the higher trophic levels should be contextualized within the particularities of the microbial communities at different scales (Zinger et al., 2011; Sevastou et al., 2013).
Accordingly, the main objective of the present study was to investigate the microbial community (Bacteria and Archaea) of Blanes Canyon (NW Mediterranean Sea) and its adjacent open slope in terms of abundance, diversity, composition, and potential functionality. In particular, we compared two locations at similar depths (1500 m) along the canyon axis and on its western open slope. We studied the microbial responses at three different levels: ecosystem (canyon vs. slope), vertical sediment profile (three progressively deeper layers) and season (autumn vs. spring). We also tested whether the environmental/trophic variables (mainly sediment characteristics) and biotic conditions (meiofaunal nematode abundances as potential predators) inside the canyon do affect the microbial communities differently to those on the adjacent open slope.
Materials and Methods
Study Area
The head of Blanes Canyon (North Catalan Margin, Iberian Peninsula, NW Mediterranean, Figures 1A,B) deeply cuts the continental shelf slope at 60 m depth, 4 km off the Tordera Delta (Figures 1B,C). The canyon (184 km long, 20 km of maximum width at about 2000 m depth) has a complex topography strongly influencing the near bottom currents resulting in highly variable (eastern wall) and prevailing offshore-directed (western wall) flows (Zúñiga et al., 2009; Lastras et al., 2011). Major particle transport responds to flooding from Tordera River and the numerous coastal creeks of its catchment and to major coastal storms (autumn–winter), as well as to phytoplankton blooms (spring–summer) (Flexas et al., 2008; Zúñiga et al., 2009; López-Fernández et al., 2013).

Figure 1. (A) Location of the study area in the Mediterranean Sea. (B) Color bathymetric map of the study area in the North-Catalan margin. (C) Color bathymetric map and the sampling stations (white and black dots) in the Blanes Canyon region (marked with an arrow in B). ToR, Tordera River; TeR, Ter River; FR, Fluvià River; BC, Blanes canyon; LFC, La Fonera Canyon; CCC, Cap de Creus Canyon; OS, Open slope. Color maps are redrawn from the bathymetric map of GRC of Marine Geosciences of University of Barcelona.
Sampling Strategy and Data Collection
Sediment samples were obtained on board of the R/V García del Cid in autumn 2012 and spring 2013 in two stations 35 km apart (Blanes Canyon axis, western open slope) at ca. 1500 m depth (Figure 1C and Supplementary Table S1, Román et al., 2016). Sediment was collected by means of 6-tube multiple-corer (KC Denmark A/S, inner diameter 9.4 cm; length 60 cm), yielding intact sediment profiles. Multicore deployments (three replicates per sampling station) yielded large sediment corers that were sub-sampled on board for microbial and sediment analyses with a sub-corer (3.6 cm diameter, 5 cm thickness), and sliced down to 5 cm into three sediment layers (0–1 cm: surface; 1–2 cm: sub-surface; 2–5 cm: deep) thus giving rise to 36 individual sediment samples. Samples for microbial and environmental sediment analyses were preserved in absolute ethanol and stored frozen at -20°C, respectively. Among the full set of sediment variables described by Román et al. (2016), we are here using grain size (clay, silt, and sand fractions), organic carbon (OC, %), total nitrogen (TN, %), chlorophyll a (Chl a, μg/g) and the chloroplastic pigments equivalents (CPEs: sum of Chl a and its degradation products as phaeopigments, mg/g), as a proxy of surface-produced OM (Thiel, 1978), and the Chl a: phaeopigments ratio (Chl a: phaeo), as a proxy of the freshness of photosynthetically derived OM (Plante-Cuny and Bodoy, 1987). High phaeopigments vs. Chl a ratios are generally associated with detrital and degraded autotrophic matter inputs.
Total microbial community DNA was extracted from 10 g of sediment per sample and processed with the PowerMax®Soil DNA Isolation Kit from MO BIO Laboratories, Inc. (Guardiola et al., 2016). Meiofaunal nematode samples, methods and data (i.e., abundance) come from Román et al. (2016, 2018).
Sequencing and Data Filtering
High-speed multiplexed 16S rRNA gene microbial sequencing with the Illumina MiSeq System (2 × 250 bp) was carried out in the V4 region using 515f and 806r primers (Caporaso et al., 2011), the earth microbiome project protocols (EMP), and the genomics core facilities of the Research Technology Support Facility, Michigan State University (RTSF-MSU, United States). The selected primers are widely used to amplify both Bacteria and Archaea with few known biases (Apprill et al., 2015; Parada et al., 2016). A total of 5398793 raw sequences were filtered and further clustered with UPARSE (Edgar, 2013) by read length (above or equal to 250 pb) with an expected error of 0.25. After singleton and chimera removal, sequences were further clustered into 22059 97%-identity OTUs. The 3530733 filtered sequences were mapped back into these OTUs and classified with the SILVA 119 database (Quast et al., 2013) through the SILVA-ngs pipeline1. Raw files are available in the National Center for Biotechnology (NCBI) repository under the project code PRJNA489177.
Functional Prediction
Functional predictions based on representative genomes are useful for the estimation of metabolic potential when metagenomes are not available (Langille et al., 2013; Nemergut et al., 2015). Although some strains within the same taxonomic rank may have distinct functional signatures or environmental distributions, functional predictions provide an approximation to functional patterns at the community level (Ortiz-Álvarez et al., 2018). We applied an adaptation of the Tax4Fun2 routine (Wemheuer et al., 2018) using gene presence/absence weighted by the relative abundance of each taxa in each sample. The numeric results represent a fraction of the community that matched with the functional database, and indicate the community proportions containing each specific function. We filtered a total of 106 KEGGs (functional orthologs) within 30 metabolic pathways (Llorens-Marès et al., 2015), including five additional phosphorous cycle pathways (G-3-P transport, phosphate transport -high and low efficiency-, phosphonate transport, and phosphonate metabolism) (Vila-Costa et al., 2013), and four complex OM decomposition pathways (chitin, cellulose, hemicellulose, and lignin) (Lüneberg et al., 2018).
Quantification of 16S rRNA Gene (qPCR Analyses) and Microbial Abundance Estimation
The determination of 16S rRNA gene copies was carried out per sample through quantitative PCR amplification using the bacterial universal primers 341F-534R (Muyzer et al., 1996; Watanabe et al., 2001). Q-PCR assays were run on 96-well white qPCR plates (Bio-Rad, Hercules, CA, United States) in a DNA engine thermal cycler (Bio-Rad) equipped with a Chromo 4 real-time detector (Bio-Rad) (López-Gutiérrez et al., 2004). The reaction mixture (20 μl) contained 10 μl of SsoFast EvaGreen supermix (Bio-Rad), 5 μl of template DNA (1 ng), 10 μM primers and molecular biology-grade water (Sigma, St. Louis, MO, United States). Standard curves were obtained from clones containing E. coli 16S rRNA using 10-fold serial dilutions. All reactions were run in triplicate with standard curves spanning from 102 to 107 DNA copies. Since each bacterial taxon has different 16S gene copies in their genomes, qPCR results required a 16S copy number correction by bacterial OTU. We used the curated database rrnDB version 5.4 (Stoddard et al., 2014) to calculate an estimated 16S rRNA copy number for each OTU based on the lower taxonomic rank available with a 0.85 cut-off. The conversion factor was the average number of copies per OTU weighted by their relative abundances per sample. The final abundance estimate was corrected by the % of archaea per sample, since the qPCR primers were bacteria-specific.
Statistical Analyses
Alpha diversity, as OTU Richness and Shannon index (Shannon, 1948), were estimated after rarefaction to the minimum sampling size of 35000 sequences per sample, and separately for archaea (2000 sequences) and bacteria (35000 sequences). One canyon sample was removed due to a low number of sequences after rarefaction. Shannon index was calculated with the function diversity in package ‘vegan’ (Oksanen et al., 2017). The community structure based on OTUs was standardized through Hellinger transformation, fitted under a non-metric multidimensional scaling (nMDS) based on Bray–Curtis dissimilarities calculated with function vegdist in package ‘vegan,’ and plotted with ggplot2 function in package ‘ggplot2’ (Wickham, 2009). We quantified beta diversity (i.e., variability in species composition between sampling units for a given area, per habitat and per sediment layer) using the function betadisper in package ‘vegan.’ Indicative OTUs were detected with function IndVal in package ‘labdsv’ (Roberts, 2013). Microbial community descriptors (estimated abundance, alpha diversity, community structure, and functional prediction structure) were analyzed by means of non-parametric permutational analyses of variance (PERMANOVA) using PRIMER v6 (Anderson, 2005; Anderson et al., 2008). Similarity matrices for univariate descriptors (i.e., standing stocks and structural diversity) were built based on Euclidean similarity. Differences in microbial communities were analyzed using a three-way fixed model, with a fully crossed design by habitat (Ha: canyon and slope), season (Se: autumn and spring) and sediment layer (SL: surface, sub-surface and deep) in PERMANOVA + for Primer (Anderson et al., 2008). The same three-way design was used to analyze differences in sediment environmental variables by univariate PERMANOVAs, except for the grain size, where clay, silt, and sand percentages were considered as multivariate variables. Sediment variable matrices were based on Euclidean similarities. Additionally, principal component analysis (PCA) was performed on the environmental data matrix to show the ordination pattern of the samples. Significant differences were indicated as p < 0.05, p < 0.01, or p < 0.001.
The strength of the relationships between the selected environmental and biological variables (clay, silt, sand, OC, TN, Chl a, CPE, and Chl a: phaeo, nematode densities) and univariate community descriptors (i.e., abundance and structural diversity), for both canyon and slope habitats separately, were assessed by Spearman Rank correlations by means of the XLSTAT (Addinsoft) software, with a fdr p-adjustment. RELATE and DISTLM (distance-based linear model) PRIMER routines based on the normalized environmental data (Anderson et al., 2008) allowed to analyze and model the relationship between microbial community structure and environmental variables, following the same design as for univariate metrics. Metabolism differences by factors were explored through Kruskal–Wallis test (Ha and SL) and Spearman correlations with univariate descriptors (% Archaea, microbial abundances, and nematode densities). The same PERMANOVA design described for microbial assemblages was used to analyze metabolism differences, based on a Bray–Curtis dissimilarity matrix.
Results
Sediment Physical and Chemical Variability
Both canyon and slope sediments were predominantly muddy (2–63 μm in grain diameter) with high silt content (64 to 77%, respectively) (Figure 2). However, canyon and slope samples were differentiated according to the environmental variables in the PCA plot (Figure 3). The first two PCA axes explained a total of 66.5% of the variation (Figure 3). The main contributors were clay (-0.392), sand (0.449) and Chl a (0.398) for PC1, and OC (-0.517), TN (-0.444), silt (-0.444), and CPE (-0.423) for PC2 (numbers in parenthesis represent eigenvectors). Sediment grain size showed significant differences for the interactions Ha × Se and Ha × SL (Table 1: PERMANOVA, p < 0.01). Differences between seasons indicated that sediments were coarser in spring than in autumn (pair-wise test, Ha × Se, p < 0.05, see Figure 2), and particularly, canyon sediments had up to 20% more sand in spring. Slope sediments became progressively finer along the vertical sediment profile (pair-wise test, Ha × SL, p < 0.05, see Supplementary Figure S1).
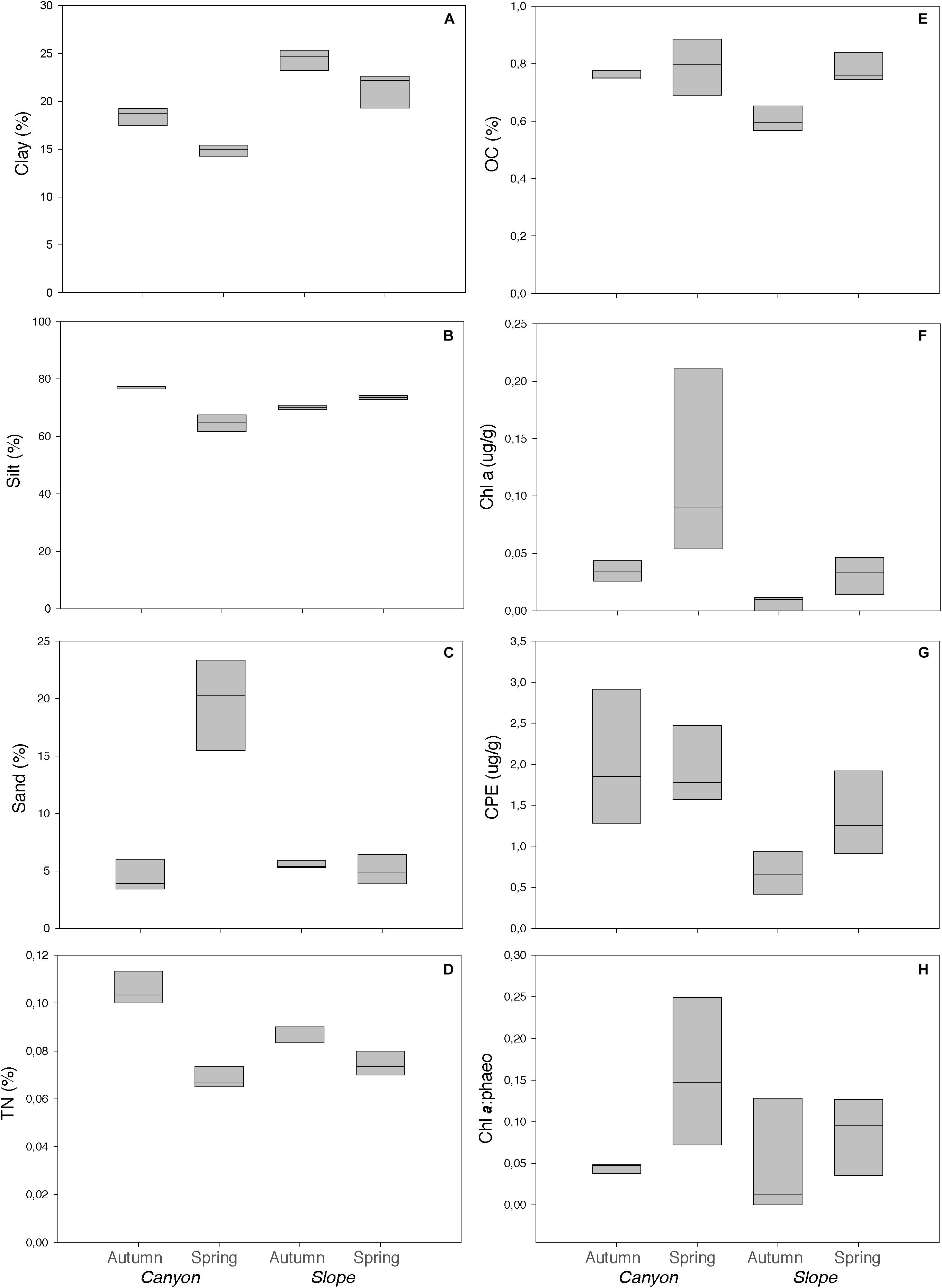
Figure 2. Environmental variables used in this study for canyon (BC) and slope (OS) stations: (A–C) Granulometry (%Clay, %Silt, and %Sand), (D) TN (total nitrogen), (E) OC (organic carbon), (F) Chl a (Chlorophyll a), (G) CPEs (chloroplastic pigment equivalents) and (H) Chl a: phaeo (Chlorophyll a: phaeopigments). Black lines represent the median, and lower box indicates the first quartile and upper box the third quartile. Upper line on the boxes shows the maximum value and lower line the minimum value.
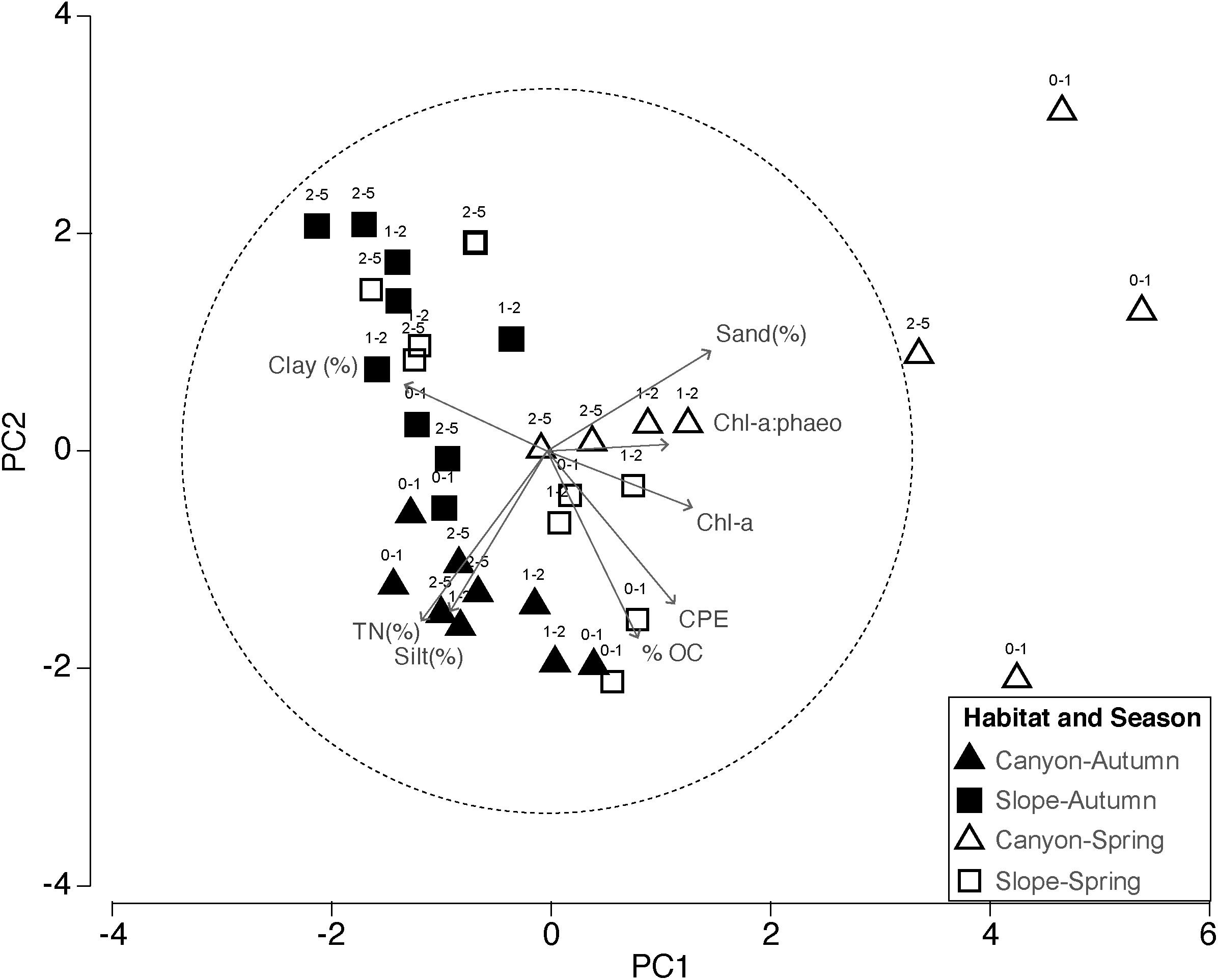
Figure 3. Principal component analysis (PCA) ordination based on selected environmental variables at the studied stations. Parameters included in the analyses were: clay (%), silt (%); sand (%), organic carbon (OC), total nitrogen (TN), chlorophyll a (chl a), chloroplast pigments equivalents (CPE), chlorophyll a: phaeopigment ratio (chl a: phaeo).
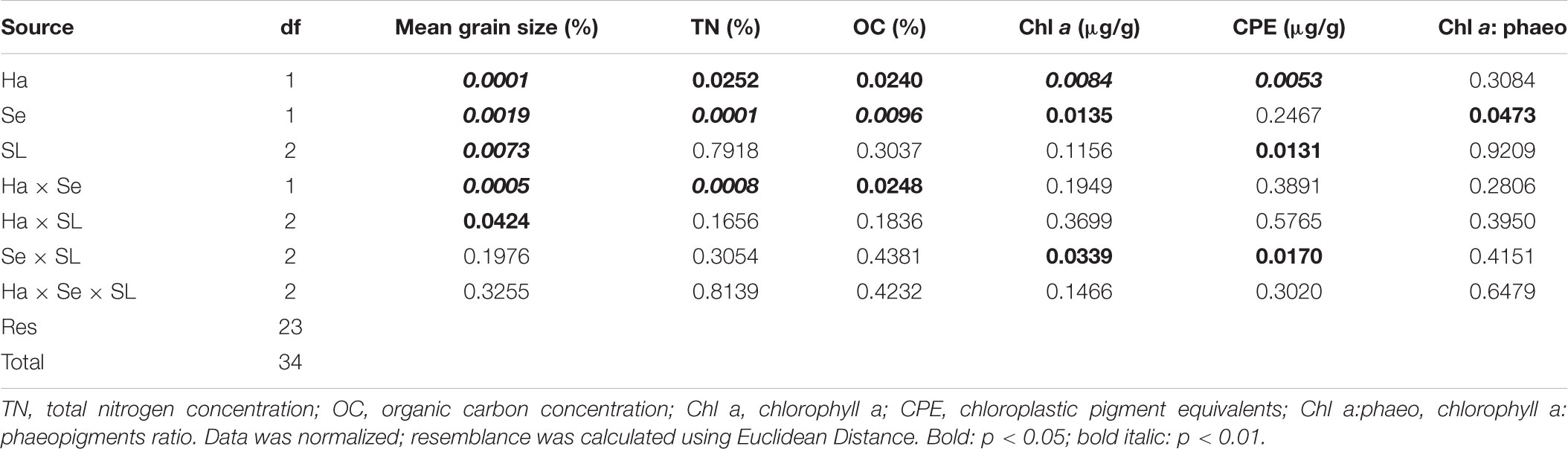
Table 1. Results from univariate and multivariate PERMANOVA three-way analyses for differences in environmental sediment variables, using Habitat (Ha), Season (Se), and Sediment Layer (SL) as factors.
OC and TN differed significantly between habitats and seasons, as well as in the interaction Ha × Se (PERMANOVA, p < 0.05, Table 1). In autumn, they were significantly higher in the canyon (0.76 and 0.11%, respectively) than on the slope (0.60 and 0.09%, respectively) (p < 0.05, see Figure 2). On the slope, they differed in autumn and spring, while in the canyon only TN differed (pair-wise, p < 0.05, see Figure 2).
Chl a and CPE also differed significantly between habitats, being higher in the canyon (0.03 and 0.7 μg/g on average, respectively) than on the slope (0.01 and 0.3 μg/g on average, respectively) (Figure 2 and Table 1), but also the interaction Se × SL differed significantly (Table 1). Chl a at the surface sediment layer was higher in spring (pair-wise test, Se × SL, p < 0.05, Supplementary Figure S1), while CPE was progressively lower along the vertical sediment profile (pair-wise test, Se × SL, p < 0.05, Supplementary Figure S1). The Chl a: phaeo ratio was significantly higher in spring (PERMANOVA, p < 0.05, Table 1).
Taxonomic Groups of Bacteria and Archaea
The dataset comprised 16694 OTUs in the canyon and 13318 in the slope, sharing 37% of the total (8161). Sequences were classified into 10 archaeal and 45 bacterial phyla, out of which 10 were candidate divisions. Classified sequences (98.7%) were assigned to members of bacteria (87% canyon, 85% slope), with Proteobacteria (including Alpha-, Beta-, Gamma-, Delta- and Epsilon- proteobacteria) being dominant (45% canyon, 36% slope) (Figure 4A). Gammaproteobacteria (1679 OTUs) were dominant among them, showing a higher proportion in the canyon than in the slope (25.2% canyon vs. 18.4% slope). The Deltaproteobacteria (3343 OTUs) were also enhanced in the canyon (12.7% canyon vs. 9.8% slope), being surpassed by Planctomycetes (4055 OTUs) on the open slope (10.6% canyon, 12.9% slope). Within this phylum, we found genera such as Scalindua and Kuenenia, involved in the annamox pathway. Also relevant in proportion and richness were Acidobacteria (1379 OTUs; 9% canyon, 13% slope) and Alphaproteobacteria (1049 OTUs; both 5.7%). Interestingly, the ratio richness:abundance consistently differed by bacterial phyla in the canyon and on the slope (Figure 4). Particularly Gammaproteobacteria (mostly Oceanospirillales and Xanthomonadales) and Alphaproteobacteria were more abundant than rich. Conversely, Planctomycetes or Deltaproteobacteria showed a higher OTU richness:abundance ratio (Figure 4). Regarding the sediment layers, there were not evident differences in taxonomic profile nor at the phylum nor at the class level and only Verrucomicrobia (236 OTUs) significantly (p < 0.001) decreased in abundance along the vertical sediment profile. At lower taxonomic ranks, there were many OTUs that exhibited differences between the three sediment layers according to their indicative values (IndVal > 0.5). These indicative OTUs showed differences at the phyla level: Planctomycetes and Nitrospirae had more indicators in the deeper layer, while Gammaproteobacteria, Alphaproteobacteria, and Bacteroidetes were more characteristic of the surface and subsurface layers (Supplementary Figure S2).
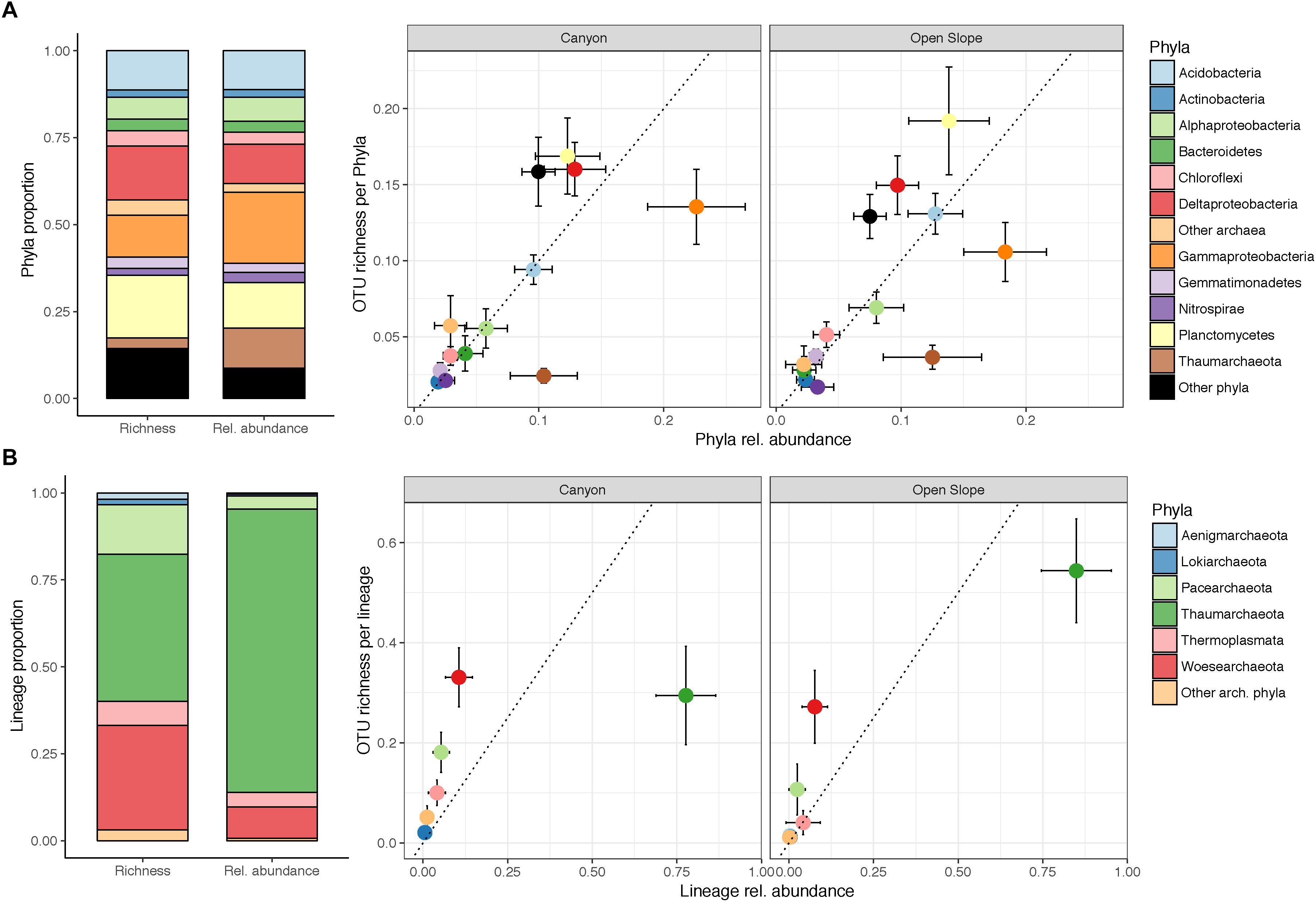
Figure 4. Average microbial community profile per sample (richness and relative abundance), and phyla ratios between the proportion of richness and relative abundances per habitat (canyon and slope) based on the average number of reads per sample. Error bars indicate the standard deviation (SD). Panels indicate: (A) overall taxonomic profile and ratios and (B) archaea specific profile and ratios.
Archaea were highly abundant in most samples, accounting for a 14% on average (ranging from 4.2 to 23.4%). Archaeal sequences were classified within the lineages Euryarchaeota, Aenigmarchaeota, Woesearchaeota, Pacearchaeota, Diapherotrites, Lokiarchaeota, Crenarchaeota, and Thaumarchaeota. However, most OTUs belonged to the Woesearchaeota (1250), followed by Pacearchaeota (651), Euryarchaeota (187), and Thaumarchaeota (181). Thaumarchaeota showed the highest abundance, which was not linked to a high proportion of OTUs (Figure 4B). Moreover, OTU proportions of Woesearchaeota and Thaumarchaeota greatly differed between canyon and slope, inverting their ranks. Some of the most abundant (14) and widespread (100%) OTUs were assigned to Candidatus Nitrosopumilus. The thaumarchaeotal groups AK31 and Marine Benthic group ‘A’ were significantly more abundant on the slope.
Patterns of Microbial Abundances and Diversity
The estimated cell abundances in the canyon and on the slope ranged from 1.56 × 106 to 3.57 × 1010 and 3.81 × 109 to 4.39 × 1010 cells per sediment gram, respectively (Figure 5). Both habitats showed significant higher abundances in autumn than in spring in the surface and subsurface layers (Se × SL PERMANOVA, Table 2 and Supplementary Table S2). The abundances decreased along the vertical sediment profile on the slope, particularly in autumn, while were evenly distributed in the canyon (Figure 5). Furthermore, abundances were significantly higher in the canyon than on the slope only for the deep sediment layer (Ha × SL PERMANOVA, Table 2 and Supplementary Table S2). Alpha diversity differed significantly between habitats (ρ < 0.05, Table 2). The richness of Archaea and Bacteria was higher in the canyon than on the slope, being strongly correlated with microbial abundances (ρ = 0.42, p < 0.01). Shannon index was higher in the canyon than on the slope for Bacteria, contrary to Archaea (Figure 6). Beta diversity was higher in the canyon along the whole vertical sediment profile (Figure 7).
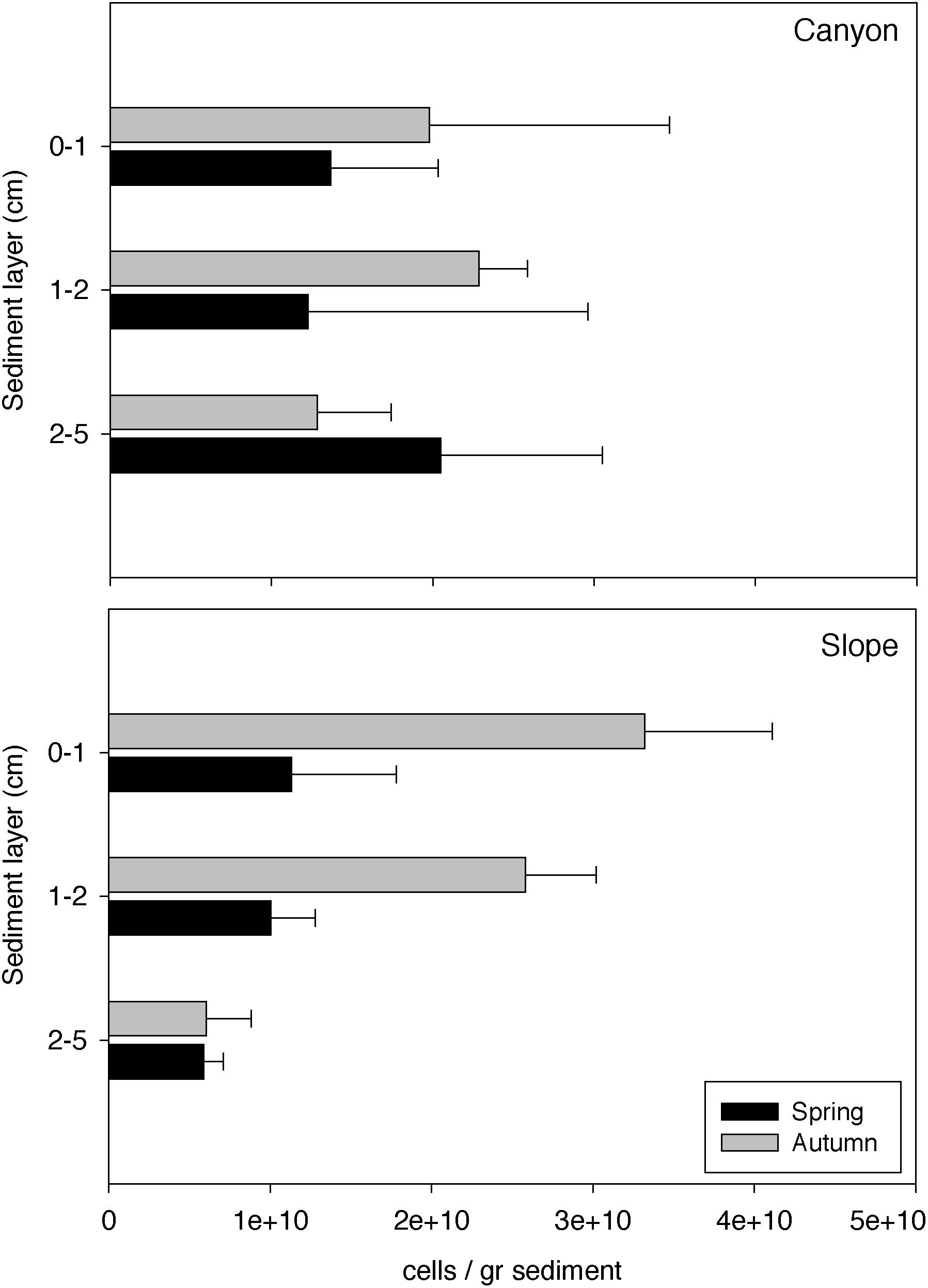
Figure 5. Estimated microbial abundances (number of cells/gram of sediment) along the vertical sediment profile in the canyon and on the slope during autumn and spring. Errors bars are standard deviations.
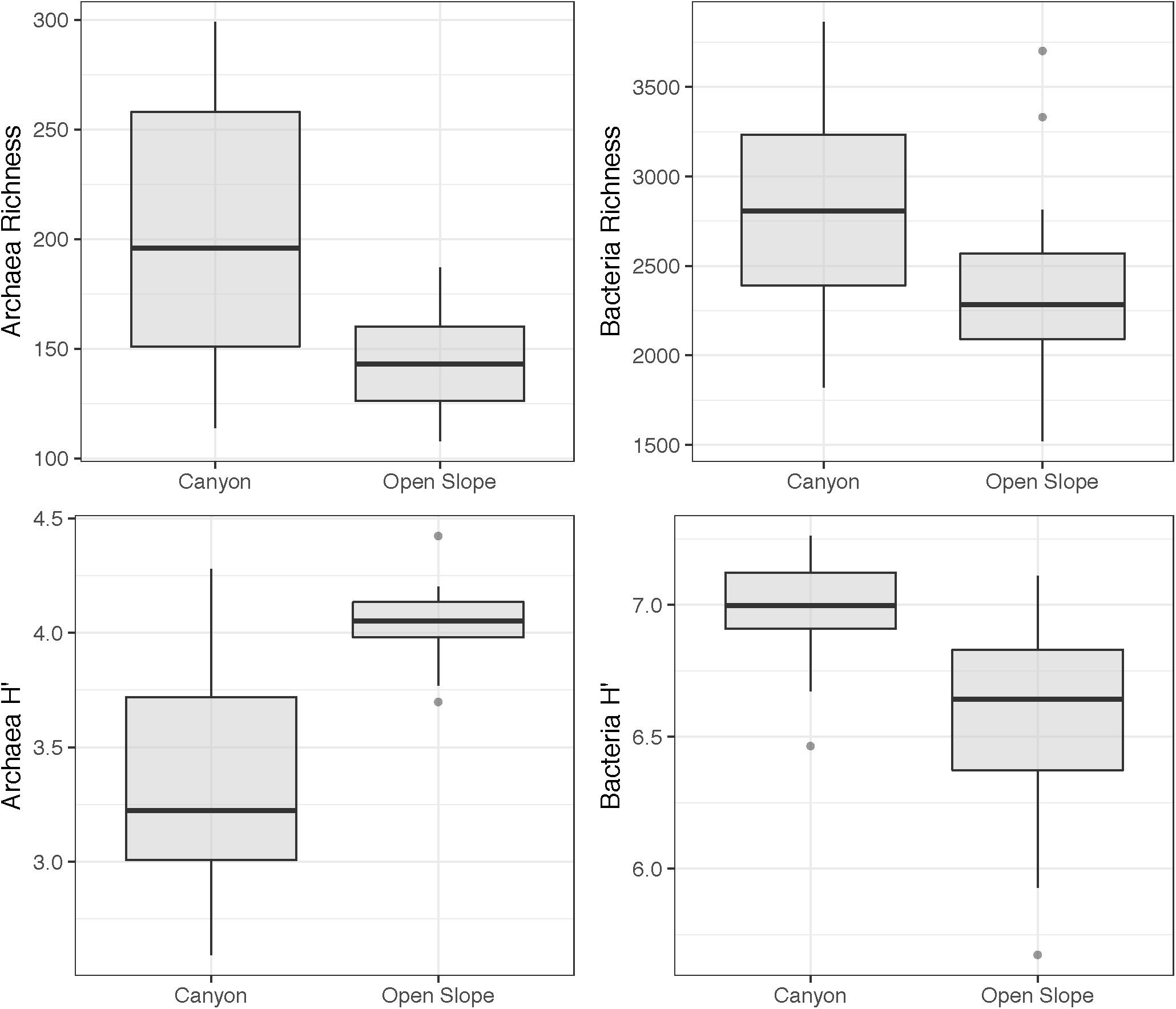
Figure 6. Alpha diversity of Archaea and Bacteria measured as Richness (OTU number) and Shannon index (H′).
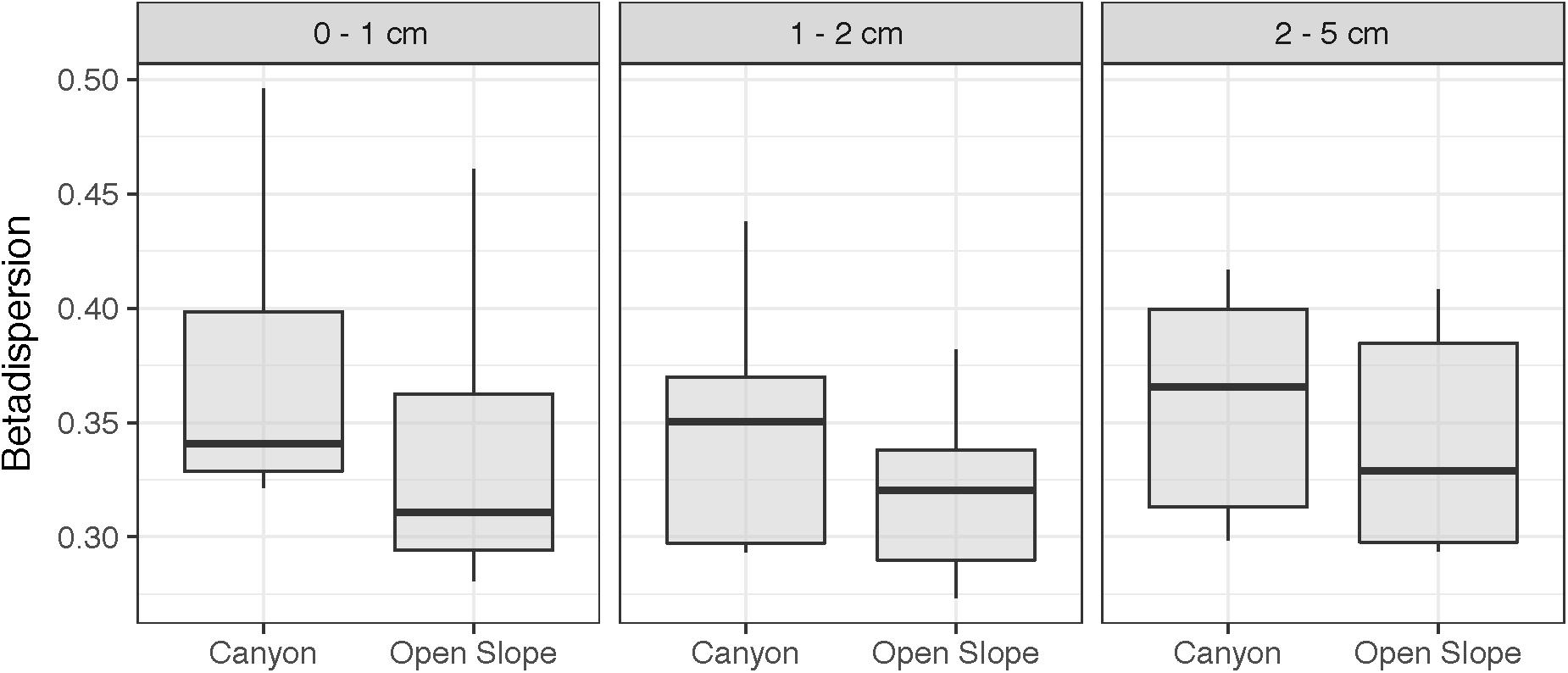
Figure 7. Beta-diversity (as Betadispersion) of samples (with both Archaea and Bacteria) grouped by habitat and sediment layer.
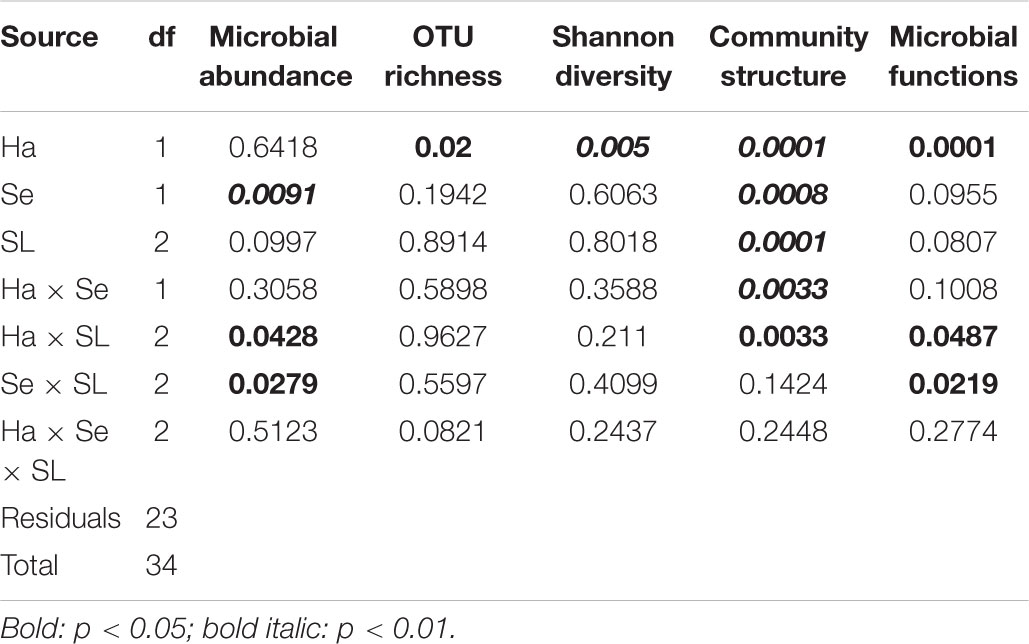
Table 2. Results of three-way PERMANOVA analyses for differences in microbial descriptors using Habitat (Ha), Season (Se), and Sediment Layer (SL) as factors.
On the slope, abundance and Shannon Index were negatively correlated with clay and positively with silt, and positively with nematode densities (Table 3). In the canyon, there was a negative correlation between abundance and OC, while alpha diversity was positively correlated with Chl a only in autumn (Table 3). Additionally, only archaeal richness was negatively correlated with nematode abundance (ρ = -0.43, p < 0.01) on the slope.
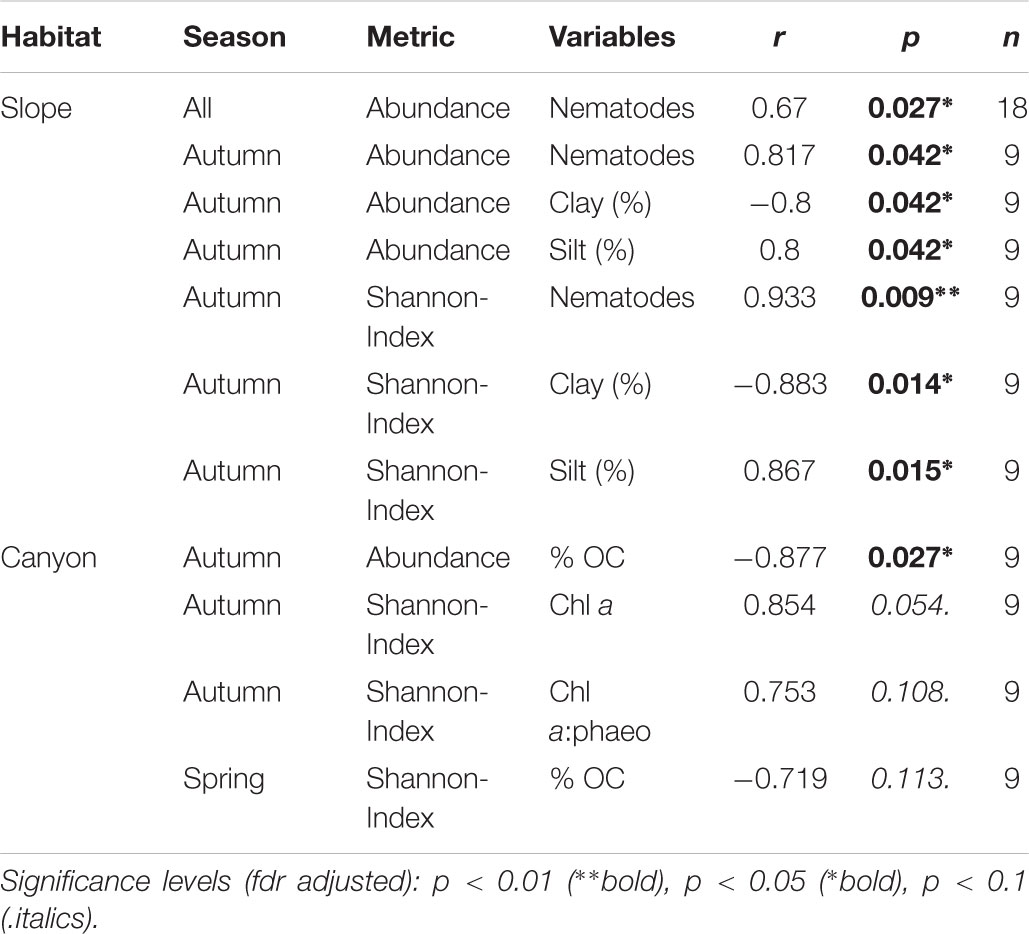
Table 3. Spearman correlations between independent variables (environmental factors and nematode abundance) with microbial metrics (abundance and Shannon index) for both habitats.
Patterns of Community Structure
Community structure based on OTU-derived Bray–Curtis dissimilarities, clearly differed between canyon and slope (Figure 8 and Table 2), showing significant differences in terms of Habitat, Season and Sediment Layer, but also for the interactions Ha × Se and Ha × SL (PERMANOVA, Table 2). Canyon and slope significantly differed in both seasons, but seasonal variations were only significant in the canyon (pair-wise tests, Figure 8A and Supplementary Table S3). Additionally, all sediment layers showed strongly differentiated OTU communities both in the canyon and on the slope (Ha × SL, Figure 8B and Supplementary Table S3). Within habitats, significant differences among all sediment layers were found on the slope, while in the canyon these were mainly observed between surface and deep layers (Figure 8B and Supplementary Table S3).
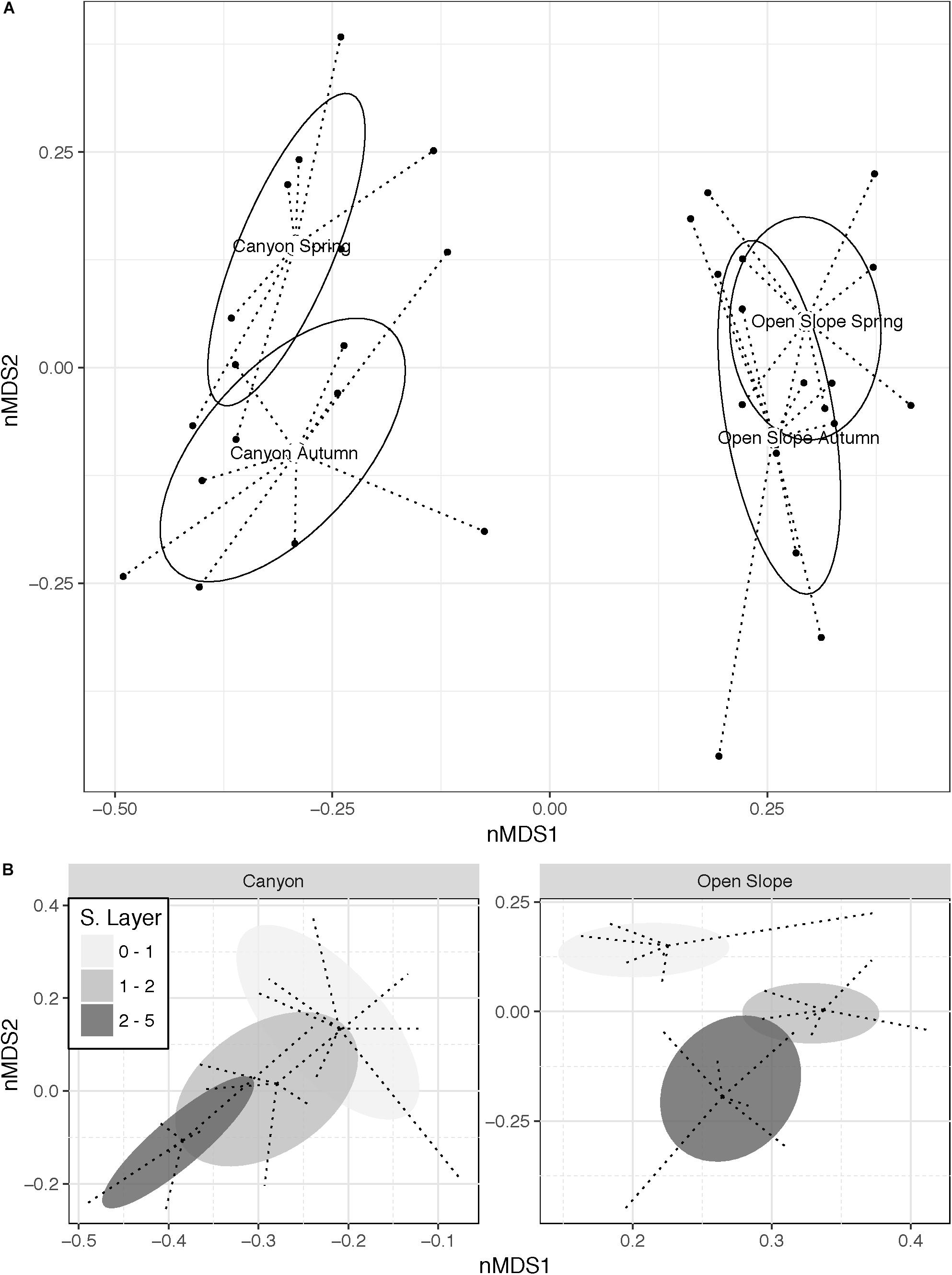
Figure 8. Non-metric MDS plots (Bray–Curtis similarity) based on Hellinger transformed microbial community data (OTUs) illustrating the significant interactions: (A) Habitat × Season and (B) Habitat × Sediment Layer.
We only found significant explanatory environmental variables in the canyon, explaining 24.4% of the community variability (according to RELATE analysis-PRIMER). The responses were TN, clay, sand, and silt (marginal -individual variables- test, DISTLM, p < 0.05; 12.9, 11.7, 11.1, and 10.7% of variation explained, respectively, Supplementary Table S5). The combination TN and silt constituted the best explanatory model for the community patterns (sequential test, ca. 58% of total variability explained), which imply that grain size explains a large part of the observed variability (Supplementary Table S5). Samples showed separation between seasons, with the most influencing environmental variables being Chl a and Chl a: phaeo in spring (Supplementary Figure S3). The first two dbRDA axes explained 42.2% of the community variation (Supplementary Figure S3).
Relationships of Functional Predictions With Spatial Factors and Densities
The functional predictions correspond, on average, to 30% of reads/sample (minimum 24.4%, maximum 40.5%), according to the matches with the Tax4Fun2 reference functional dataset. Without considering the spatial factors, the community proportion of pathways of the different biogeochemical cycles (Figure 9) had significant correlations with independently measured variables such as nematode density, which was positively correlated with aerobic respiration, and negatively with the NO3 reduction/NO2 oxidation two-way pathway. Also, microbial abundance was positively correlated to the carbon cycle, specifically with arnon C fixation and fermentative processes. Furthermore, the relative abundance of Archaea was positively correlated with N mineralization and nitrification.
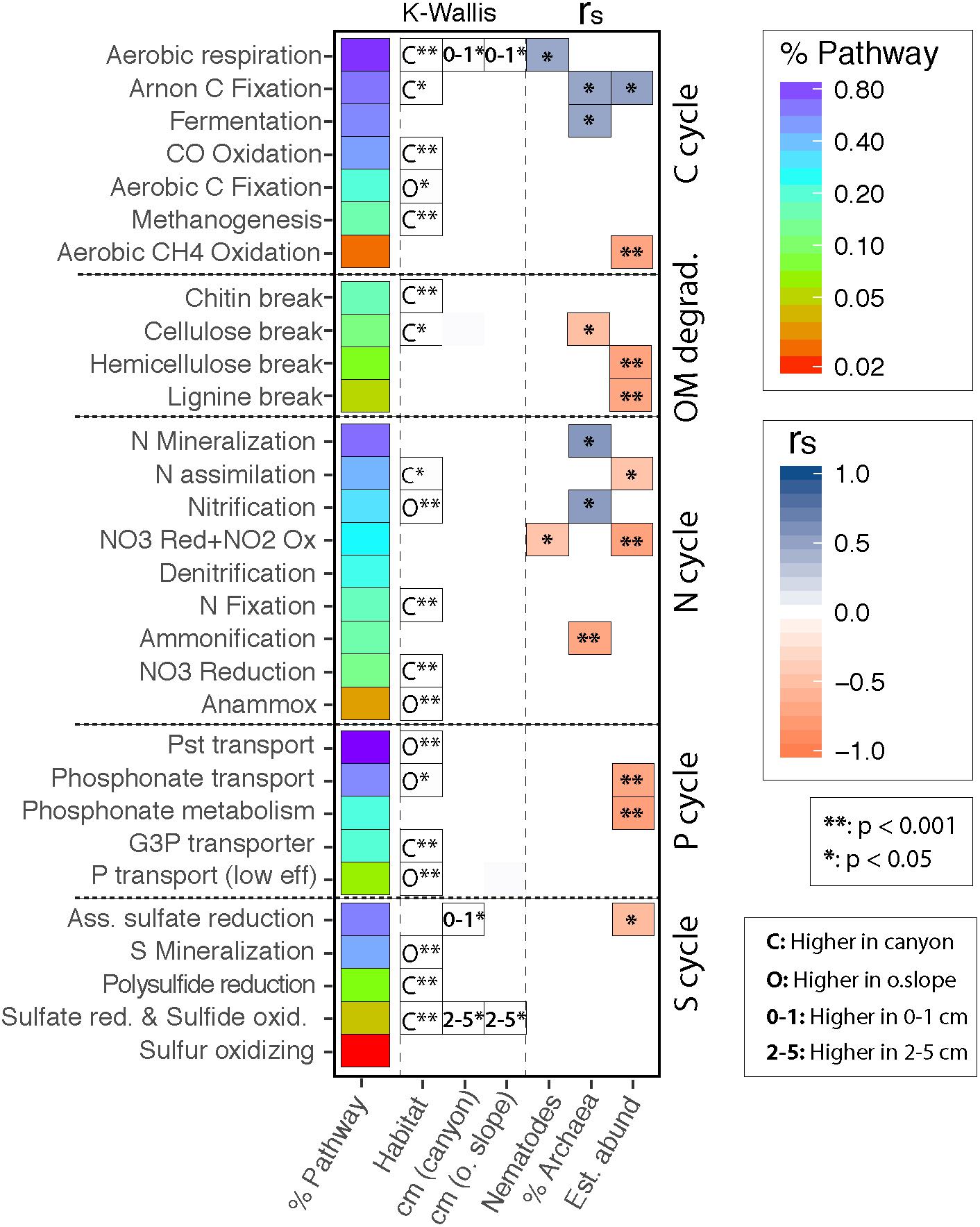
Figure 9. Predicted functional pathways sorted by mean dataset abundance and element. Significant differences for each pathway are shown for spatial factors (Habitat and Sediment Layer in the Canyon and on the Slope separately), nematodes and microbial abundances, and the % of archaea/sample. The heatmap displays statistically significant relationships after fdr adjustment (∗∗p < 0.001, ∗p < 0.05), according to Kruskal–Wallis test (spatial factors) and spearman correlations (univariate metrics). C/O 0-1/2-5 indicate the factor level harboring a significantly increased value, and the color scale the Spearman’s r magnitude.
In addition, spatial factors and seasons significantly separated the functional profiles according to PERMANOVA (Figure 9, Table 2 and Supplementary Table S4). Habitat alone statistically differentiated the profiles, but there were post hoc differences between habitats in the three sediment layers (Ha × SL). Specifically, aerobic respiration, Arnon C fixation, chitin and cellulose break, or N assimilation/fixation were significantly higher in the canyon; while nitrification, annamox or P transport were significantly higher on the open slope. Furthermore, there were differences between the three sediment layers but only during autumn (Se × SL), and regardless of the season solely on the open slope (Ha × SL). The upper layer had significantly higher aerobic respiration (both habitats), and assimilatory sulfate reduction (canyon only). The deeper layer showed higher potential for the sulfate reduction and sulfide oxidation pathway (both habitats) (Figure 9).
Discussion
Differences Between Canyon and Open Slope
We expected that the differential environmental dynamics affecting canyon and slope habitats would lead the respective microbial communities to assemble differently, thus giving place to particular patterns. Indeed, Blanes Canyon’s higher dynamism promotes diversity (both for bacterial and archaeal taxa pools) and community heterogeneity. The complex topography and strong, variable currents characterising submarine canyons enhance marine landscape diversity at regional scales, providing singular habitats to a highly diverse fauna (Huvenne and Davies, 2014; Fernandez-Arcaya et al., 2017; Ismail et al., 2018). In Blanes Canyon, high-rank taxa showed similar profiles to the open slope, but only 37% of the OTUs were shared. Accordingly, the two habitats at the same depth (ca. 1,500 m) showed clearly different sediment communities. Deep-sea microbial communities often have shown a high degree of specificity in terms of taxa and ecological specializations as a response to characteristic nutrient and particle fluxes (Dang et al., 2009; Li et al., 2009; Jacob et al., 2013; Sevastou et al., 2013; Ristova et al., 2015). Blanes Canyon shows a high TN level through its whole vertical sediment profile, suggesting sedimentation rates higher than those on the slope leading to major environmental differences. OM indicators (OC, Chl a, CPE) were also higher in the canyon (Román et al., 2016), which may contribute to the environmental idiosyncrasy influencing microbial community assembly. Downward particle fluxes are higher in the canyon axis than those in the eastern and western adjacent open slopes due to external forcing such as storm and/or dense shelf water cascading events (Canals et al., 2006; Zúñiga et al., 2009; López-Fernández et al., 2013). In particular, sedimentation rates at 1500 m depth inside the canyon were twice as high than in the slope (Paradis et al., 2018). These environmental differences among habitats may certainly contribute to explain the canyon’s singularity as a habitat for microbial communities. Differences also occur in meiofaunal communities between Blanes Canyon and their adjacent slopes (Romano et al., 2013a; Román et al., 2016). Consequently, ecological interactions may also contribute to the observed differences in microbial communities. For instance, specific associations between nematodes and bacteria have been detected in a nearby canyon system (Rzeznik-Orignac et al., 2018). As a more general trend, we have observed that nematode densities lacked seasonal changes and showed positive relationships with bacterial diversity and abundance only on the slope, whereas nematode densities increased in the canyon during spring (Román et al., 2016). This suspected association between meiofaunal and microbial communities might reflect different trophic interactions in the benthic sediments at the two contrasting habitats. Predatory/scavenger nematodes (such as Sphaerolaimus and Pomponema) dominate the Blanes Canyon communities, while non-selective deposit feeders were less numerous compared to the adjacent slope (Román et al., 2018), which appear to be related to their different roles in the trophic web (Ingels et al., 2009). These differences in nematode community composition may thus enhance the differential environmental trends discussed above, joining them in explaining the observed between-habitat differences concerning the microbial assemblages’ establishment and growth.
Interestingly, when comparing bacterial and archaeal phyla by habitat, we observed that Planctomycetes and Thaumarchaeota, both containing taxa with roles in the N cycle (Sintes et al., 2013; Restrepo-Ortiz et al., 2014; Kuypers et al., 2018), had higher richness in the open slope than in the canyon. Part of the microbial richness observed may also act as seed microbes or opportunists, actively changing their abundances as a response to environmental situations matching their functional requirements (Sebastián et al., 2018). These functional requirements displayed differences along habitats and sediment layers. The pathway with the highest proportion, aerobic respiration, is more abundant in the canyon, particularly in surface sediments. In parallel, nematode abundance is also higher in the canyon (Supplementary Figure S4), contributing to sediment oxygenation through bioturbation (Sturdivant et al., 2012). Also, vegetal debris occurred more often in Blanes Canyon than on the open slope (C. Romano, unpublished data), together with the associated wood-degrading macrofauna (Romano et al., 2013b, 2014). Therefore, we also expected to encounter enhanced predicted chitin and cellulose pathways in the canyon sediments.
Differences Between Seasons
The marked between-season differences in microbial communities observed in Blanes Canyon was associated to increases in available phytodetritus in spring. These may probably be explained by the winter 2012 unusual atmospheric conditions, which led to the formation of dense shelf water cascading in the whole NW Mediterranean (Durrieu de Madron et al., 2013). These increases in available phytodetritus in spring (Román et al., 2016) may have led to increased nematode densities (Supplementary Figure S4), and surprisingly lower microbial densities. Despite microbial community structure lacked relationships with environmental variables on the open slope, we found positive relationships between microbial abundance and diversity with nematode densities and silt content solely during autumn. This may be explained by multiple heterogeneous processes, including anthropogenic activities, affecting the Blanes submarine canyon and its sedimentation characteristics (López-Fernández et al., 2013; Román et al., 2016; Paradis et al., 2018) with distinct intensities over time. Food availability may certainly be a key driver (Goffredi and Orphan, 2010; Sevastou et al., 2013; Bienhold et al., 2016), but also others may play a role in shaping these communities that may explain the often non-consistent relationships with food sources. Therefore, the consistency of these observations require further checking over time before allowing us to derive accurate seasonal predictions in microbial assemblage trends.
Adaptations Along the Vertical Sediment Profile
Substrate heterogeneity has often been considered a possible cause of spatial variability in deep-sea environments and their biodiversity (Rex and Etter, 2010). In our study, the differentiation of microbial community structure and abundance along the vertical sediment profile has been the second most important pattern observed. Differences between microbial communities from upper to deeper layers have been reported for both habitats, being in agreement with the down-core stratification reported in previous studies (e.g., Fry et al., 2006; Jorgensen et al., 2012; Bienhold et al., 2016). Surface and subsurface communities in the canyon seemed to harbor homogeneous communities, which may be supported by mixing and depositional processes (Harrison et al., 2018), consistent with its sedimentation characteristics (Lastras et al., 2009; Zúñiga et al., 2009; Pusceddu et al., 2010). Microbial abundances decreased particularly on the open slope, suggesting a more stable sediment stratification responding to a higher environmental homogeneity and stability (e.g., in terms of particle fluxes or ocean currents) compared to Blanes Canyon (Zúñiga et al., 2009). In the canyon, clay content gradually increased with depth, as reported in other regions (Nunoura et al., 2018), while productivity variables were linked to seasonal events showing higher variability. Consequently, the high microbial abundances in canyon’s subsurface and deeper layers may be explained by the higher seasonal production variability together with its higher sediment heterogeneity (Ingels et al., 2009; Zúñiga et al., 2009). Overall, the differences in sediment and OM deposition and accumulation rates appear to be mirrored by the microbial communities (likely due to their size), thus contributing to explain the more homogeneous distribution patterns observed on the slope. The relationship between sediment environmental variables and microbial abundances also differed between habitats, suggesting the existence of different drivers. Sediment OM content emerged as a key factor associated with changes in bacterial community structure in other locations (Sevastou et al., 2013; Bienhold et al., 2016). However, while we have found weak ‘food-source’ relationships with the microbial descriptors (abundance and community), these only occurred in the canyon, where the sediments were more organically enriched than on the slope (Román et al., 2016; Román, 2017). In turn, sediment granulometry arose as a fundamental driver for microbial assemblages both in canyon and slope environments.
Ecological Roles and Potential Functionality of Deep-Sea Sediments
We found a noteworthy proportion of sequences assigned to archaeal lineages. In general, contrasting to their scarcity in the water column (Sunagawa et al., 2015), archaeal contribution to the total benthic microbial biomass in particular from Thaumarchaeota, has been pointed out to be greater than previously hypothesized (Danovaro et al., 2016). Some of these archaea are critical players in the N cycle in marine sediments (Kuypers et al., 2018), and the observed high relative abundances in our study probably benefit from the ammonia excretion coming from the nematodes (Barnes, 1980). However, despite the high proportion of Thaumarchaeota per sample, the richest groups were Pacearchaeota and Woesearchaeota, considering the whole sampling area but particularly in the canyon. These lineages have an unknown ecological role in the Blanes Canyon system but display an enormous environmental versatility and biome occurrence (Ortiz-Álvarez and Casamayor, 2016). Studies in inland environments support their anaerobic heterotrophic lifestyle and metabolic complementarity with other microbes (Liu et al., 2018). Given that archaeal richness was negatively correlated with nematode abundance, we suggest a niche differentiation of these lineages along the vertical sediment profile. Regarding N, both microbial abundances and nematode densities were negatively correlated to the predicted NO3 reduction/NO2 oxidation two-way pathway. Accordingly, nematodes likely play a strong role in the N cycle by supplying ammonia to the system (Ferris et al., 1998). And both nitrification and anammox processes, which require ammonia, are particularly enhanced on the open slope. Annamox is a process exclusive to a few genera (Kuenenia and Scalindua) from the phylum Planctomycetes (Kuenen, 2008; Sonthiphand et al., 2014), genera that were indeed detected in our dataset. Planctomycetes is also dominant in the deep-sea sediments from the Eastern Mediterranean Sea (Quaiser et al., 2011; Bienhold et al., 2016), and the South Atlantic Ocean (Schauer et al., 2010). In addition, in South China sediments, the deep-sea harbors a higher abundance of microbes involved in nitrification, anammox, and ammonification than the shallower sediments (Yu et al., 2018).
The deep ocean floor copes with all the debris deposited from the water column. In this environment, biogeochemical cycling is key in avoiding the particles to remain buried by transforming them into available nutrients that nurture the complex trophic chain. Microbes degrading sunken wood produce significant amounts of hydrogen sulfide through S mineralization, which is the base of the wood chemosynthetic ecosystem (Kalenitchenko et al., 2017), that also exist in Blanes Canyon (Fagervold et al., 2013, 2014; Romano et al., 2013b, 2014; Bessette et al., 2014). The high burial rates in the canyon (López-Fernández et al., 2013) lead oxygen levels to quickly drop a few centimeters below the sediment surface, while causing higher aerobic activity in the surface sediment layers (indicated by the increased aerobic respiration and aerobic sulfate reduction pathway). The occurrence of the chemosynthetic nematode Astomonema only in the canyon (Román, 2017), especially at deeper layers (Román et al., 2018), supports the presence of hydrogen sulfide by the lack of oxygen and sulfate respiration in the sediments (Ingels et al., 2011). The reduced conditions at the deep layers found both in the canyon and on the slope were supported by the dominance of Sabatieria (Román et al., 2018), indicating reduced oxygen availability (Soetaert et al., 1995; Vanreusel et al., 1997).
Conclusion
We report idiosyncratic microbial characteristics (in terms of abundance, diversity, composition, and predicted functions) in two closely related geographical regions, a canyon and its adjacent open slope. Our results suggest that the deep-sea seafloor is strongly connected to coastal and pelagic productive areas through the canyon system in a stronger manner than to the open slope, thus modulating resource availability while driving changes in the microbial biosphere and the deep-sea food web. Overall, the microbial communities were dominated by bacteria, in which Gammaproteobacteria were the most abundant, followed by Deltaproteobacteria (the richest group), Planctomycetes, Acidobacteria, and Alphaproteobacteria. Although not dominant, Archaeal contribution to diversity and abundance was significant. Most OTUs belonged to Woesearchaeota followed by Pacearchaeota, Euryarchaeota and Thaumarchaeota, which showed the higher proportion and occurrence. The gradually decreasing abundance along the vertical sediment profile on the slope was explained by environmental stability and correspondingly low food availability. Conversely, canyon sediments showed much more variable geomorphological and environmental conditions which, together with the greater amount of available food (as Chl a, CPE, and OC), lead this habitat to harbor distinctive microbial assemblages in terms of composition and structure. In addition, the observed differences in community structure between autumn and spring were only evident in the canyon, again tracing a higher dynamism than on the open slope. The high downward particle fluxes driven by bottom trawling activities along canyon flanks appear to be directly related with the high reported sedimentation rates inside the canyon. This supports the existence of a considerable anthropogenic influence on microbial assemblages at the studied depth, but long-term studies are required to confirm this hypothesis. Furthermore, the arrangement of communities along the 0–5 cm vertical sediment profile, and the differences in predicted metabolisms, indicates niche specialization with potential consequences in higher trophic levels. Correlations between sediment microbiota and nematodes were only observed on the slope, in the absence of seasonal variability, and corresponding with differences along the vertical sediment profile. However, only ecological studies targeting both fauna and microbiological composition, for example under a co-occurrence network approach, will reveal the different ecological specificities of these two food web compartments. In light of our results, we conclude that Blanes Canyon notably contributes to the deep-sea microbial biodiversity, enlarging the idea of the ‘canyon effect’ shaping deep-sea microbial benthic communities.
Author Contributions
SR and RO-A contributed equally to the manuscript, leading writing, and data analysis. SR, CR, and DM collected and processes samples. RO-A and EC contributed with sequence analysis, statistical analyses, and microbiological interpretations. SR, RO-A, and CR contextualized microbial data in the deep-sea realm. All authors contributed to manuscript revision, read and approved the submitted version.
Funding
This research has been supported by DOS MARES (Reference No. CTM2010-21810-C03-03) research project, funded by the Spanish “Agencia Estatal de Investigación” (AEI) and the European Funds for Regional Development (FEDER). CR received an International Outgoing Fellowship from the People Programme (Marie S. Curie Actions) of the European Union’s Seventh Framework Programme (FP7/2007-2013) under REA Grant Agreement No. PIOF-GA-2013-628146. RO-A and SR were supported by the FPI fellowship programme from the Spanish Government. DM was supported by the Consolidated Research Group on Marine Benthic Ecology of the Generalitat de Catalunya (2017SGR378) and the Research Project PopCOmics (CTM2017-88080), funded by AEI and FEDER. RO-A and EC were supported by project DARKNESS CGL2012-32747.
Conflict of Interest Statement
The authors declare that the research was conducted in the absence of any commercial or financial relationships that could be construed as a potential conflict of interest.
Acknowledgments
The authors would like to thank the crew of the R.V. García del Cid and colleagues from CSIC, UB, and other institutions for helping on board. Thanks to Dr. Galderic Lastras (UB) for GIS expertise. In addition, they thank M. Guardiola, G. Carreras, and X. Turon for laboratory assistance.
Supplementary Material
The Supplementary Material for this article can be found online at: https://www.frontiersin.org/articles/10.3389/fmars.2019.00108/full#supplementary-material
Footnotes
References
Allen, S., and Durrieu de Madron, X. (2009). A review of the role of submarine canyons in deep-ocean exchange with the shelf. Ocean Sci. 5, 607–620. doi: 10.5194/os-5-607-2009
Allen, S., Vindeirinho, C., Thomson, R., Foreman, M. G., and Mackas, D. (2001). Physical and biological processes over a submarine canyon during an upwelling event. Can. J. Fish. Aquat. Sci. 58, 671–684. doi: 10.1139/f01-008
Anderson, M., Gorley, R. N., and Clarke, K. R. (2008). PERMANOVA for PRIMER: Guide to Software and Statistical Methods. Plymouth: PRIMER-E.
Anderson, M. J. (2005). Permutational multivariate analysis of variance. Dep. Stat. Univ. Auckland 26, 32–46.
Apprill, A., Mcnally, S., Parsons, R., and Weber, L. (2015). Minor revision to V4 region SSU rRNA 806R gene primer greatly increases detection of SAR11 bacterioplankton. Aquat. Microb. Ecol. 75, 129–137. doi: 10.3354/ame01753
Barone, G., Rastelli, E., Corinaldesi, C., Tangherlini, M., Danovaro, R., and Dell’Anno, A. (2018). Benthic deep-sea fungi in submarine canyons of the Mediterranean Sea. Prog. Oceanogr. 168, 57–64. doi: 10.1016/j.pocean.2018.09.011
Berner, R. A. (1982). Burial of organic carbon and pyrite sulfur in the modern ocean: its geochemical and environmental significance. Am. J. Sci. 282, 451–473. doi: 10.2475/ajs.282.4.451
Bessette, S., Fagervold, S., Romano, C., Martin, D., Le Bris, N., and Galand, P. E. (2014). Diversity of bacterial communities on sunken woods in the Mediterranean Sea. J. Mar. Sci. Technol. 22, 60–66.
Bianchelli, S., and Danovaro, R. (2019). Meiofaunal biodiversity in submarine canyons of the Mediterranean Sea: a meta-analysis. Prog. Oceanogr. 170, 69–80. doi: 10.1016/j.pocean.2018.10.018
Bianchelli, S., Gambi, C., Mea, M., Pusceddu, A., and Danovaro, R. (2013). Nematode diversity patterns at different spatial scales in bathyal sediments of the Mediterranean Sea. Biogeosciences 10, 5465–5479. doi: 10.5194/bg-10-5465-2013
Bienhold, C., Zinger, L., Boetius, A., Ramette, A., and Kellogg, C. (2016). Diversity and biogeography of bathyal and abyssal seafloor bacteria. PLoS One 11:e0148016. doi: 10.1371/journal.pone.0148016
Canals, M., Puig, P., de Madrn, X., Heussner, S., Palanques, A., and Fabre, J. (2006). Flushing submarine canyons. Nature 444, 354–357. doi: 10.1038/nature05271
Caporaso, J. G., Lauber, C. L., Walters, W. A., Berg-Lyons, D., Lozupone, C. A., Turnbaugh, P. J., et al. (2011). Global patterns of 16S rRNA diversity at a depth of millions of sequences per sample. Proc. Natl. Acad. Sci. U.S.A. 108, 4516–4522. doi: 10.1073/pnas.1000080107
Celussi, M., Quero, G. M., Zoccarato, L., Franzo, A., Corinaldesi, C., Rastelli, E., et al. (2018). Planktonic prokaryote and protist communities in a submarine canyon system in the Ligurian Sea (NW Mediterranean). Prog. Oceanogr. 168, 210–221. doi: 10.1016/j.pocean.2018.10.002
Chauvet, P., Metaxas, A., Hey, A., and Matabos, M. (2018). Annual and seasonal variations in the deep-sea megafaunal epibenthic communities in Barkley Canyon (British Columbia, Canada): a response to surface and deep-sea environment. Prog. Oceanogr. 169, 89–105. doi: 10.1016/j.pocean.2018.04.002
Dang, H., Li, J., Chen, M., Li, T., Zeng, Z., and Yin, X. (2009). Fine-scale vertical distribution of bacteria in the East Pacific deep-sea sediments determined via 16S rRNA gene T-RFLP and clone library analyses. World J. Microbiol. Biotechnol. 25, 179–188.
Danovaro, R., Dinet, A., Duineveld, G., and Tselepides, A. (1999). Benthic response to particulate fluxes in different trophic environments: a comparison between the Gulf of Lions–Catalan Sea (western-Mediterranean) and the Cretan Sea (eastern-Mediterranean). Prog. Oceanogr. 44, 287–312. doi: 10.1016/S0079-6611(99)00030-0
Danovaro, R., Marrale, D., Dell’Anno, A., Della Croce, N., Tselepides, A., and Fabiano, M. (2000). Bacterial response to seasonal changes in labile organic matter composition on the continental shelf and bathyal sediments of the Cretan Sea. Prog. Oceanogr. 46, 345–366. doi: 10.1016/S0079-6611(99)00030-0
Danovaro, R., Molari, M., Corinaldesi, C., and Dell’Anno, A. (2016). Macroecological drivers of archaea and bacteria in benthic deep-sea ecosystems. Sci. Adv. 2:e1500961. doi: 10.1126/sciadv.1500961
De Leo, F. C., Vetter, E. W., Smith, C. R., Rowden, A. A., and Mcgranaghan, M. (2014). Spatial scale-dependent habitat heterogeneity influences submarine canyon macrofaunal abundance and diversity off the main and Northwest Hawaiian Islands. Deep Sea Res. Part II 104, 267–290. doi: 10.1016/j.dsr2.2013.06.015
De Stigter, H., Boer, W., Thomsen, L., and Jesus, C. (2007). Recent sediment transport and deposition in the Nazaré Canyon, Portuguese continental margin. Mar. Geol. 246, 144–164. doi: 10.1016/j.dsr2.2013.06.015
Deming, J. W., and Baross, J. A. (1993). The Early Diagenesis of Organic Matter: Bacterial Activity. Organic Geochemistry. Berlin: Springer. doi: 10.1016/j.margeo.2007.04.011
Durrieu de Madron, X., Houpert, L., Puig, P., Sanchez-Vidal, A., Testor, P., Bosse, A., et al. (2013). Interaction of dense shelf water cascading and open-sea convection in the northwestern Mediterranean during winter 2012. Geophys. Res. Lett. 40, 1379–1385. doi: 10.1002/grl.50331
Edgar, R. C. (2013). UPARSE: highly accurate OTU sequences from microbial amplicon reads. Nat. Methods 10:996. doi: 10.1038/nmeth.2604
Fagervold, S., Bessette, S., Romano, C., Martin, D., Plyuscheva, M., Le Bris, N., et al. (2013). Microbial communities associated with the degradation of oak wood in the Blanes submarine canyon and its adjacent open slope (NW Mediterranean). Prog. Oceanogr. 118, 137–143. doi: 10.1007/s00248-016-0923-5
Fagervold, S., Romano, C., Kalenitchenko, D., Martin, D., and Galand, P. E. (2014). Microbial communities in sunken wood are structured by wood-boring bivalves and location in a submarine canyon. PLoS One 9:e96248. doi: 10.1371/journal.pone.0096248
Fernandez-Arcaya, U., Ramirez-Llodra, E., AguzzI, J., Allcock, A. L., Davies, J. S., Dissanayake, A., et al. (2017). Ecological role of submarine canyons and need for canyon conservation: a review. Front. Mar. Sci. 4:5. doi: 10.3389/fmars.2017.00005
Ferris, H., Venette, R. C., Van Der Meulen, H. R., and Lau, S. S. (1998). Nitrogen mineralization by bacterial-feeding nematodes: verification and measurement. Plant Soil 203, 159–171. doi: 10.1023/A:1004318318307
Flexas, M. M., Boyer, D. L., Espino, M., Puig De Fabregas, J., and Rubio, A. (2008). Circulation over a submarine canyon in the NW Mediterranean. J. Geophys. Res. 113, 1–18. doi: 10.1029/2006JC003998
Fry, J. C., Webster, G., Cragg, B. A., Weightman, A. J., and Parkes, R. J. (2006). Analysis of DGGE profiles to explore the relationship between prokaryotic community composition and biogeochemical processes in deep subseafloor sediments from the Peru Margin. FEMS Microbiol. Ecol. 58, 86–98. doi: 10.1111/j.1574-6941.2006.00144.x
Gage, J. (2003). in Food Inputs, Utilization, Carbon Flow and Energetics, ed. P. A. Tyler (Amsterdam: Elsevier Science B.V).
Galand, P. E., Gutiérrez-Provecho, C., Massana, R., Gasol, J. M., and Casamayor, E. O. (2010). Interannual recurrence of archaeal assemblages in the coastal NW Mediterranean Sea (Blanes Bay Microbial Observatory). Limnol. Oceanogr. 55, 2117–2125. doi: 10.4319/lo.2010.55.5.2117
Goffredi, S. K., and Orphan, V. J. (2010). Bacterial community shifts in taxa and diversity in response to localized organic loading in the deep sea. Environ. Microbiol. 12, 344–363. doi: 10.1111/j.1462-2920.2009.02072.x
Granata, T. C., Estrada, M., Zika, U., and Merry, C. (2004). Evidence for enhanced primary production resulting from relative vorticity induced upwelling in the Catalan Current. Cont. Shelf Res. 19, 1249–1263. doi: 10.1016/S0278-4343(98)00118-6
Guardiola, M., Wangensteen, O. S., Taberlet, P., Coissac, E., Uriz, M. J., and Turon, X. (2016). Spatio-temporal monitoring of deep-sea communities using metabarcoding of sediment DNA and RNA. PeerJ 4:e2807. doi: 10.7717/peerj.2807
Harris, P., and Whiteway, T. (2011). Global distribution of large submarine canyons: geomorphic differences between active and passive continental margins. Mar. Geol. 285, 69–86. doi: 10.1016/j.margeo.2011.05.008
Harrison, B. K., Myrbo, A., Flood, B. E., and Bailey, J. V. (2018). Abrupt burial imparts persistent changes to the bacterial diversity of turbidite-associated sediment profiles. Geobiology 16, 190–202. doi: 10.1111/gbi.12271
Huvenne, V. A., and Davies, J. S. (2014). Towards a new and integrated approach to submarine canyon research. Introduction. Deep Sea Res. Part II 104, 1–5. doi: 10.1016/j.dsr2.2013.09.012
Ingels, J., Kiriakoulakis, K., Wolff, G., and Vanreusel, A. (2009). Nematode diversity and its relation to the quantity and quality of sedimentary organic matter in the deep Nazaré Canyon, Western Iberian Margin. Deep Sea Res. Part 1 Oceanogr. Res. Papers 56, 1521–1539. doi: 10.1016/j.dsr.2009.04.010
Ingels, J., Tchesunov, A. V., and Vanreusel, A. (2011). Meiofauna in the gollum channels and the Whittard Canyon, Celtic margin – how local environmental conditions shape nematode structure and function. PLoS One 9:e20094. doi: 10.1371/journal.pone.0020094
Ismail, K., Huvenne, V., and Robert, K. (2018). Quantifying spatial heterogeneity in submarine canyons. Prog. Oceanogr. 169, 181–198. doi: 10.1016/j.pocean.2018.03.006
Jacob, M., Soltwedel, T., Boetius, A., and Ramette, A. (2013). Biogeography of deep-sea benthic bacteria at regional scale (LTER HAUSGARTEN, Fram Strait, Arctic). PLoS One 8:e72779. doi: 10.1371/journal.pone.0072779
Jorgensen, S. L., Hannisdal, B., Lanzén, A., Baumberger, T., Flesland, K., Fonseca, R., et al. (2012). Correlating microbial community profiles with geochemical data in highly stratified sediments from the Arctic Mid-Ocean Ridge. PNAS 109, E2846–E2855. doi: 10.1073/pnas.1207574109
Kalenitchenko, D., Le Bris, N., Dadaglio, L., Peru, E., Besserer, A., and Galand, P. E. (2017). Bacteria alone establish the chemical basis of the wood-fall chemosynthetic ecosystem in the deep-sea. ISME J. 12:367. doi: 10.1038/ismej.2017.163
Kuenen, J. G. (2008). Anammox bacteria: from discovery to application. Nat. Rev. Microbiol. 6:320. doi: 10.1038/nrmicro1857
Kuypers, M. M., Marchant, H. K., and Kartal, B. (2018). The microbial nitrogen-cycling network. Nat. Rev. Microbiol. 16:263. doi: 10.1038/nrmicro.2018.9
Langille, M. G., Zaneveld, J., Caporaso, J. G., Mcdonald, D., Knights, D., Reyes, J. A., et al. (2013). Predictive functional profiling of microbial communities using 16S rRNA marker gene sequences. Nat. Biotechnol. 31:814. doi: 10.1038/nbt.2676
Lastras, G., Arzola, R. G., Masson, D. G., and Wynn, R. B. (2009). Geomorphology and sedimentary features in the central portuguese submarine canyons, Western Iberian margin. Geomorphology 103, 310–329. doi: 10.1016/j.geomorph.2008.06.013
Lastras, G., Canals, M., Amblas, D., Lavoie, C., and Church, I. (2011). Understanding sediment dynamics of two large submarine valleys from seafloor data: blanes and la fonera canyons, northwestern Mediterranean Sea. Mar. Geol. 280, 20–39. doi: 10.1016/j.margeo.2010.11.005
Leduc, D., Rowden, A. A., Nodder, S. D., Berkenbusch, K., Probert, P. K., and Hadfield, M. G. (2014). Unusually high food availability in Kaikoura Canyon linked to distinct deep-sea nematode community. Deep Sea Res. Part II 104, 310–318. doi: 10.1016/j.dsr2.2013.06.003
Li, H., Yu, Y., Luo, W., Zeng, Y., and Chen, B. (2009). Bacterial diversity in surface sediments from the Pacific Arctic Ocean. Extremophiles 13, 233–246. doi: 10.1007/s00792-009-0225-7
Lindh, M., Maillot, B., Shulse, C., Gooday, A., Amon, D., Smith, C., et al. (2017). From the surface to the deep-sea: bacterial distributions across polymetallic nodule fields in the clarion-clipperton zone of the Pacific Ocean. Front. Microbiol. 8:1696. doi: 10.3389/fmicb.2017.01696
Liu, X., Li, M., Castelle, C. J., Probst, A. J., Zhou, Z., Pan, J., et al. (2018). Insights into the ecology, evolution, and metabolism of the widespread Woesearchaeotal lineages. Microbiome 6:102. doi: 10.1186/s40168-018-0488-2
Llorens-Marès, T., Yooseph, S., Goll, J., Hoffman, J., Vila-Costa, M., Borrego, C. M., et al. (2015). Connecting biodiversity and potential functional role in modern euxinic environments by microbial metagenomics. ISME J. 9:1648. doi: 10.1038/ismej.2014.254
López-Fernández, P., Calafat, A., Sanchez-Vidal, A., Canals, M., Flexas, M. M., Cateura, J., et al. (2013). Multiple drivers of particle fluxes in the Blanes submarine canyon and southern open slope: results of a year round experiment. Prog. Oceanogr. 118, 95–107. doi: 10.1016/j.pocean.2013.07.029
López-Gutiérrez, J. C., Henry, S., Hallet, S., Martin-Laurent, F., Catroux, G., and Philippot, L. (2004). Quantification of a novel group of nitrate-reducing bacteria in the environment by real-time PCR. J. Microbiol. Methods 57, 399–407. doi: 10.1016/j.mimet.2004.02.009
Lüneberg, K., Schneider, D., Siebe, C., and Daniel, R. (2018). Drylands soil bacterial community is affected by land use change and different irrigation practices in the Mezquital Valley, México. Sci. Rep. 8:1413. doi: 10.1038/s41598-018-19743-x
Moens, T., Bouillon, S., and Gallucci, F. (2005). Dual stable isotope abundances unravel trophic position of estuarine nematodes. J. Mar. Biol. Assoc. U.K. 85, 1401–1407. doi: 10.1017/S0025315405012580
Moens, T., and Vincx, M. (1997). Observations on the feeding ecology of estuarine nematodes. J. Mar. Biol. Assoc. U.K. 77, 211–227. doi: 10.1017/S0025315400033889
Muyzer, G., Hottentrager, S., Teske, A., and Waver, C. (1996). “Denaturing gradient gel electrophoresis of PCR-amplified 16S rDNA. A new molecular approach to analyze the genetic diversity of mixed microbial communities,” in Molecular Microbial Ecology Manual, eds A. D. L. Akkermans, J. D. van Elsas, and F. J. de Bruijn (Dordrecht: Kluwer Academic Publishing).
Nemergut, D. R., KnelmaN, J. E., Ferrenberg, S., Bilinski, T., Melbourne, B., Jiang, L., et al. (2015). Decreases in average bacterial community rRNA operon copy number during succession. ISME J. 10:1147. doi: 10.1038/ismej.2015.191
Nunoura, T., Nishizawa, M., Hirai, M., Shimamura, S., Harnvoravongchai, P., Koide, O., et al. (2018). Microbial diversity in sediments from the bottom of the challenger deep, the mariana trench. Microbes Environ. 33, 186–194. doi: 10.1264/jsme2.ME17194
Oksanen, J., Blanchet, F., Friendly, M., Kindt, R., Legendre, P., and Mcglinn, D. (2017). vegan: Community Ecology Package. R Packag. Version 2.4–2.
Ortiz-Álvarez, R., and Casamayor, E. O. (2016). High occurrence of Pacearchaeota and Woesearchaeota (A rchaea superphylum DPANN) in the surface waters of oligotrophic high altitude lakes. Environ. Microbiol. Rep. 8, 210–217. doi: 10.1111/1758-2229.12370
Ortiz-Álvarez, R., Fierer, N., De Los Ríos, A., Casamayor, E. O., and Barberán, A. (2018). Consistent changes in the taxonomic structure and functional attributes of bacterial communities during primary succession. ISME J. 12:1658. doi: 10.1038/s41396-018-0076-2
Parada, A. E., Needham, D. M., and Fuhrman, J. A. (2016). Every base matters: assessing small subunit rRNA primers for marine microbiomes with mock communities, time-series and global field samples. Environ. Microbiol. 18, 1403–1414. doi: 10.1111/1462-2920.13023
Paradis, S., Puig, P., Sánchez-Vidal, A., Masqué, P., García-Orellana, J., Calafat, A., et al. (2018). Spatial distribution of sedimentation-rate increases in Blanes Canyon caused by technification of bottom trawling fleet. Prog. Oceanogr. 169, 241–252. doi: 10.1016/j.pocean.2018.07.001
Pasqual, C., Sanchez Vidal, A., Zuniga, D., Calafat, A., and Canals, M. (2010). Flux and composition of settling particles across the continental margin of the gulf of lion: the role of dense shelf water cascading. Biogeosciences 7, 217–231. doi: 10.5194/bg-7-217-2010
Pfannkuche, O. (1993). Benthic response to the sedimentation of particulate organic matter at the BIOTRANS station, 47 N, 20 W. Deep Sea Res. Part II Top. Stud. Oceanogr. 40, 135–149. doi: 10.1016/0967-0645(93)90010-K
Plante-Cuny, M.-R., and Bodoy, A. (1987). Biomasse et production primaire du phytoplancton et du microphytobenthos de deux biotopes sableux (Golfe de Fos, France). Oceanol. Acta 10, 223–237.
Polymenakou, P. N., Lampadariou, N., Mandalakis, M., and Tselepides, A. (2009). Phylogenetic diversity of sediment bacteria from the southern Cretan margin, Eastern Mediterranean Sea. Syst. Appl. Microbiol. 32, 17–26. doi: 10.1016/j.syapm.2008.09.006
Puig, P., Palanques, A., and Martín, J. (2014). Contemporary sediment-transport processes in submarine canyons. Ann. Rev. Mar. Sci. 6, 53–77. doi: 10.1146/annurev-marine-010213-135037
Pusceddu, A., Bianchelli, S., Martín, J., Puig, P., Palanques, A., Martín, J., et al. (2014). Chronic and intensive bottom trawling impairs deep-sea biodiversity and ecosystem functioning. Proc. Natl. Acad. Sci. U.S.A. 111, 8861–8866. doi: 10.1073/pnas.1405454111
Pusceddu, A., Bianchellia, S., Canals, M., Sanchez-Vidal, A., De Madron, X. D., Heussner, S., et al. (2010). Organic matter in sediments of canyons and open slopes of the Portuguese, Catalan, Southern Adriatic and Cretan Sea margins. Deep Sea Res. Part 1 Oceanogr. Res. Papers 57, 441–457. doi: 10.1016/j.dsr.2009.11.008
Quaiser, A., Zivanovic, Y., Moreira, D., and López-García, P. (2011). Comparative metagenomics of bathypelagic plankton and bottom sediment from the Sea of Marmara. ISME J. 5, 285–304. doi: 10.1038/ismej.2010.113
Quast, C., Pruesse, E., Yilmaz, P., Gerken, J., Schweer, T., Yarza, P., et al. (2013). The SILVA ribosomal RNA gene database project: improved data processing and web-based tools. Nucleic Acids Res. 41, D590–D596. doi: 10.1093/nar/gks1219
Ramirez-Llodra, E., Brandt, A., Danovaro, R., De Mol, B., Escobar, E., German, C. R., et al. (2010). Deep, diverse and definitely different: unique attributes of the world’s largest ecosystem. Biogeosciences 7, 2851–2899. doi: 10.5194/bg-7-2851-2010
Restrepo-Ortiz, C. X., Auguet, J. C., and Casamayor, E. O. (2014). Targeting spatiotemporal dynamics of planktonic SAGMGC-1 and segregation of ammonia-oxidizing thaumarchaeota ecotypes by newly designed primers and quantitative polymerase chain reaction. Environ. Microbiol. 16, 689–700. doi: 10.1111/1462-2920.12191
Rex, M. A., and Etter, R. J. (2010). Deep-Sea Biodiversity: Pattern and Scale. Cambridge, MA: Harvard University Press.
Ristova, P. P., Wenzhöfer, F., Ramette, A., Felden, J., and Boetius, A. (2015). Spatial scales of bacterial community diversity at cold seeps (Eastern Mediterranean Sea). ISME J. 9:1306. doi: 10.1038/ismej.2014.217
Román, S. (2017). Ecology and Biodiversity of the Deep-Sea Meiobenthos from the Blanes Canyon and its Adjacent Slope (NW Mediterranean). Ph.D. thesis, University of Barcelona, Barcelona.
Román, S., Vanreusel, A., Ingels, J., and Martin, D. (2018). Nematode community zonation in response to environmental drivers in Blanes Canyon (NW Mediterranean). J. Exp. Mar. Biol. Ecol. 502, 111–128. doi: 10.1016/j.jembe.2017.08.010
Román, S., Vanreusel, A., Romano, C., Ingels, J., Puig, P., Company, J. B., et al. (2016). High spatiotemporal variability in meiofaunal assemblages in Blanes Canyon (NW Mediterranean) subject to anthropogenic and natural disturbances. Deep Sea Res. Part I Oceanogr. Res. Papers 117, 70–83. doi: 10.1016/j.dsr.2016.10.004
Romano, C., Coenjaerts, J., Flexas, M. M., Zúñiga, D., Vanreusel, A., Company, J. B., et al. (2013a). Spatio-temporal variability of meiobenthic density in the Blanes submarine canyon (NW Mediterranean). Prog. Oceanogr. 118, 159–174.
Romano, C., Fanelli, E., D’Anna, G., Pipitone, C., Vizzini, S., Mazzola, A., et al. (2016). Spatial variability of soft-bottom macrobenthic communities in northern Sicily (Western Mediterranean): contrasting trawled vs. untrawled areas. Mar. Environ. Res. 122, 113–125. doi: 10.1016/j.marenvres.2016.10.002
Romano, C., Voight, J., Company, J. B., Plyuscheva, M., and Martin, D. (2013b). Submarine canyons as the preferred habitat for wood-boring species of Xylophaga (Mollusca, Bivalvia). Prog. Oceanogr. 118, 175–187. doi: 10.1016/j.pocean.2013.07.028
Romano, C., Voight, J. R., Pérez-Portela, R., and Martin, D. (2014). Morphological and genetic diversity of the wood-boring Xylophaga (Mollusca, Bivalvia): new species and records from deep-sea Iberian canyons. PLoS One 9:e102887. doi: 10.1371/journal.pone.0102887
Rzeznik-Orignac, J., Puisay, A., Derelle, E., Peru, E., Le Bris, N., and Galand, P. E. (2018). Co-occurring nematodes and bacteria in submarine canyon sediments. PeerJ 6:e5396. doi: 10.7717/peerj.5396
Sardà, F., Company, J. B., Bahamón, N., Rotllant, G., Flexas, M. M., Sánchez, J. D., et al. (2009). Relationship between environment and the occurrence of the deep-water rose shrimp Aristeus antennatus (Risso, 1816) in the Blanes submarine canyon (NW Mediterranean). Prog. Oceanogr. 82, 227–238. doi: 10.1016/j.pocean.2009.07.001
Schauer, R., Bienhold, C., Ramette, A., and Harder, J. (2010). Bacterial diversity and biogeography in deep-sea surface sediments of the South Atlantic Ocean. ISME J. 4, 159–170. doi: 10.1038/ismej.2009.106
Sebastián, M., Auguet, J. C., Restrepo-Ortiz, C. X., Sala, M. M., Marrasé, C., and Gasol, J. M. (2018). Deep ocean prokaryotic communities are remarkably malleable when facing long-term starvation. Environ. Microbiol. 20, 713–723. doi: 10.1111/1462-2920.14002
Sevastou, K., Lampadariou, N., Polymenakou, P. N., and Tselepides, A. (2013). Benthic communities in the deep Mediterranean Sea: exploring microbial and meiofaunal patterns in slope and basin ecosystems. Biogeosciences 10, 4861–4878. doi: 10.5194/bg-10-4861-2013
Shannon, C. (1948). A mathematical theory of communication. Bell Syst. Tech. J. 27, 379–423; 623–656. doi: 10.1002/j.1538-7305.1948.tb01338.x
Sintes, E., Bergauer, K., De Corte, D., Yokokawa, T., and Herndl, G. J. (2013). Archaeal amoA gene diversity points to distinct biogeography of ammonia-oxidizing Crenarchaeota in the ocean. Environ. Microbiol. 15, 1647–1658. doi: 10.1111/j.1462-2920.2012.02801.x
Soetaert, K., Vincx, M., and Heip, C. (1995). Nematode community structure along a Mediterranean shelf-slope gradient. Mar. Ecol. 16, 189–206. doi: 10.1111/j.1439-0485.1995.tb00405.x
Sonthiphand, P., Hall, M. W., and Neufeld, J. D. (2014). Biogeography of anaerobic ammonia-oxidizing (anammox) bacteria. Front. Microbiol. 5:399. doi: 10.3389/fmicb.2014.00399
Stoddard, S. F., Smith, B. J., Hein, R., Roller, B. R., and Schmidt, T. M. (2014). rrn DB: improved tools for interpreting rRNA gene abundance in bacteria and archaea and a new foundation for future development. Nucleic Acids Res. 43, D593–D598. doi: 10.1093/nar/gku1201
Stuart, C. T., Rex, M. A., and Etter, R. J. (2003). in Large-Scale Spatial And Temporal Patterns of Deep-Sea Benthic Species Diversity, ed. P. A. Tyler (Amsterdam: Elsevier), 295–312.
Sturdivant, S. K., Díaz, R. J., and Cutter, G. R. (2012). Bioturbation in a declining oxygen environment, in situ observations from Wormcam. PLoS One 7:e34539. doi: 10.1371/journal.pone.0034539
Sunagawa, S., Coelho, L. P., Chaffron, S., Kultima, J. R., Labadie, K., Salazar, G., et al. (2015). Structure and function of the global ocean microbiome. Science 348:1261359. doi: 10.1126/science.1261359
Thiel, H. (1978). Benthos in Upwelling Regions. Upwelling Ecosystems. Berlin: Springer, 124–138. doi: 10.1007/978-3-642-66985-9_11
Vanreusel, A., Vandenbossche, I., and Thiermann, F. (1997). Free-living marine nematodes from hydrothermal sediments: similarities with communities from diverse reduced habitats. Mar. Ecol. Prog. Ser. 157, 207–219. doi: 10.3354/meps157207
Vila-Costa, M., Sharma, S., Moran, M. A., and Casamayor, E. O. (2013). Diel gene expression profiles of a phosphorus limited mountain lake using metatranscriptomics. Environ. Microbiol. 15, 1190–1203. doi: 10.1111/1462-2920.12033
Watanabe, K., Kodama, Y., and Harayama, S. (2001). Design and evaluation of PCR primers to amplify bacterial 16S ribosomal DNA fragments used for community fingerprinting. J. Microbiol. Methods 44, 253–262. doi: 10.1016/S0167-7012(01)00220-2
Wemheuer, F., Taylor, J., Daniel, R., Johnston, E. L., Meinicke, P., Thomas, T., et al. (2018). Tax4Fun2: a R-based tool for the rapid prediction of habitat-specific functional profiles and functional redundancy based on 16S rRNA gene marker gene sequences. bioRxiv [Preprint]. doi: 10.1101/490037
Wickham, H. (2009). ggplot2: Elegant Graphics For Data Analysis. New York, NY: Springer. doi: 10.1007/978-0-387-98141-3
Yu, T., Li, M., Niu, M., Fan, X., Liang, W., and Wang, F. (2018). Difference of nitrogen-cycling microbes between shallow bay and deep-sea sediments in the South China Sea. Appl. Microbiol. Biotechnol. 102, 447–459. doi: 10.1007/s00253-017-8594-9
Zinger, L., Amaral Zettler, L., Fuhrman, J., Horner Devine, M. C., Huse, S., Sogin, M., et al. (2011). Global patterns of bacterial beta-diversity in seafloor and seawater ecosystems. PLoS One 6:e24570. doi: 10.1371/journal.pone.0024570
Keywords: submarine canyon, open slope, deep-sea, microbial communities, bacteria, archaea, biogeochemistry, sediments
Citation: Román S, Ortiz-Álvarez R, Romano C, Casamayor EO and Martin D (2019) Microbial Community Structure and Functionality in the Deep Sea Floor: Evaluating the Causes of Spatial Heterogeneity in a Submarine Canyon System (NW Mediterranean, Spain). Front. Mar. Sci. 6:108. doi: 10.3389/fmars.2019.00108
Received: 04 September 2018; Accepted: 21 February 2019;
Published: 19 March 2019.
Edited by:
Ramiro Logares, Institute of Marine Sciences (ICM), SpainReviewed by:
Rohit Ghai, Biology Centre (ASCR), CzechiaAnders Lanzen, Centro Tecnológico Experto en Innovación Marina y Alimentaria (AZTI), Spain
Copyright © 2019 Román, Ortiz-Álvarez, Romano, Casamayor and Martin. This is an open-access article distributed under the terms of the Creative Commons Attribution License (CC BY). The use, distribution or reproduction in other forums is permitted, provided the original author(s) and the copyright owner(s) are credited and that the original publication in this journal is cited, in accordance with accepted academic practice. No use, distribution or reproduction is permitted which does not comply with these terms.
*Correspondence: Rüdiger Ortiz-Álvarez, rudigerortiz@gmail.com
†These authors have contributed equally to this work