- 1Department of Earth and Environmental Sciences, University of Pavia, Pavia, Italy
- 2Institute of Marine Sciences, University of Portsmouth, Portsmouth, United Kingdom
- 3National Research Council, Institute of Marine Sciences of Ancona, Ancona, Italy
- 4Department of Biology, University of Pisa, Pisa, Italy
- 5ENEA Marine Environment Research Centre, La Spezia, Italy
- 6School of Engineering, University of Portsmouth, Portsmouth, United Kingdom
- 7Stazione Zoologica Anton Dohrn, Integrative Marine Ecology Department, Naples, Italy
Coralline algae represent the most important bioconstructors in the Mediterranean Sea and are currently impaired by the effects of climate change (CC), particularly by global warming and ocean acidification (OA). We studied the effects of these two drivers on Ellisolandia elongata, an intertidal coralline algae that is known to host a rich biodiversity of associated fauna. We cultured turfs of E. elongata in experimental conditions of increased temperature and OA (using the values of the IPCC scenario RCP- 8.5 expected for 2100: actual mean temperature +3°C and pH = 7.78), and estimated alteration of algal linear growth and community structure, focusing especially on peracarid crustaceans and annelids. Our findings revealed a decrease in linear growth, yet with no significant changes on structural integrity, and a simplification of associated community, in particular for peracarids. Our study contributes to understand community-level response to CC drivers, highlighting the vulnerability of the fauna associated to an important Mediterranean marine habitat.
Introduction
Current increase in carbon dioxide due to anthropogenic forcing (Collins et al., 2013) is dramatically affecting the oceans. The increase in greenhouse gasses has led to a rise of the global temperature average on Earth’s surface by 0.7°C during the last century and according to scenario RCP – 8.5 (IPCC, 2014), it is expected to rise by 3°C by 2100. About 93% of the excess heat accumulated in the Earth system ends up in the ocean, causing ocean warming (Bindoff et al., 2013; Rhein et al., 2013). The ocean’s capacity to act as an important sink for anthropogenic carbon over the past few decades has also caused a decrease in ocean pH, with major changes in the seawater chemistry, namely an increase of bicarbonate and a decrease of carbonate ions as well as of the saturation state of calcium carbonate. Among marine ecosystems, bioconstructions are especially vulnerable to CC, and in particular to the decrease in pH driven by the ongoing carbon uptake from the atmosphere to the ocean (Martin and Gattuso, 2009; Bijma et al., 2013; Kamenos et al., 2013). Structurally complex, bioconstructions host macroinvertebrate communities characterized by remarkable abundance and species richness (Crowder and Cooper, 1982). In fact, they increase benthic diversity by providing hard substrates with a complex architecture for the species to settle on, hide and protect, thus the resulting assemblages are highly diverse and taxonomically complex (Jokiel et al., 2008). Previous studies showed that the physical characteristics of habitats affect community structure (MacArthur and MacArthur, 1961; Lawton, 1983; Ellner et al., 2001), with several studies demonstrating the linear relationship between increase in habitat complexity and increase in the diversity and abundance of its associated fauna (Kohn and Leviten, 1976; Heck and Wetstone, 1977; Downes et al., 1998). The reasons of this linear relationship are multiple: the complexity of the structure increases the number of possible refuge areas and niches (MacArthur and MacArthur, 1961; Schoener, 1974) and the structure itself can also affect biological processes and environmental factors such as competitive interactions (Fletcher and Underwood, 1987) and impact of disturbances (e.g., mitigation of wave action) (Dommasnes, 1968; Whorff et al., 1995). Bioconstructional organisms provide the bases for many other ecosystem processes, making them pivotal for conservation (Crain and Bertness, 2006; Ingrosso et al., 2018).
On rocky intertidal shores around the world, one of the main group of bioconstructional organisms is represented by articulated coralline algae (Stewart, 1982; Dye, 1993; Benedetti-Cecchi and Cinelli, 1994), which often form complex, extremely dense and highly branched turfs that are considered the apex of algal structural complexity (Davenport et al., 1999). Even though the coralline turf is highly variable, with the fronds length and density differing at small spatial scale, they still can host high-densities of macrofaunal organisms, up to 250,000 individuals per m2 (Kelaher et al., 2001), with annelid polychaetes and crustaceans peracarids representing the dominant macrofaunal groups (Musco, 2012).
Coralline algae not only do support high biodiversity (Jones et al., 1997; Gattie et al., 2003; Kuffner et al., 2008), but they contribute to the global inorganic carbon budgets in shallow water ecosystems (Foster, 2001; Martin and Gattuso, 2009), hence they are receiving renewed attention across the ecological and geological sciences, mainly owing to the threat of CC. This is mainly due to the mineralogical composition of their thalli, based on high Mg-Calcite, the most soluble CaCO3 polymorph compared to calcite and aragonite. Several studies have analyzed the effect of pH and temperature on coralline algae, showing a stronger effect of temperature if compared with that of CO2 concentration (Martin and Gattuso, 2009; Martin et al., 2013). Previous studies have shown a negative effect of global warming (GW) on recruitment (Kuffner et al., 2008), growth (Jokiel et al., 2008), and calcification (Gao et al., 1993; Semesi et al., 2009) of coralline algae leading to an increasing susceptibility to grazing by bioeroders (Steneck, 1986). Thus, GW and OA could have a dramatic consequence on species distributions (Harley et al., 2012; Bijma et al., 2013), possibly causing shifts in their abundance and geographical boundaries (Díez et al., 2012). Most of the studies so far have been focusing on the effect of CC on the physiology of the algae, often neglecting the organisms living within their fronds. In fact, synergism between acidification and warming exacerbates the negative effects on ecosystem associated communities, and is expected to cause a shift toward a less diverse ecosystem in terms of species richness and spatial heterogeneity, directly affecting the lower levels of the food web and eventually reducing productivity and trophic energy (Kleypas et al., 2006; Smale et al., 2011; Bijma et al., 2013; Sunday et al., 2017).
This experimental study was designed to mimic the environmental conditions predicted for the year 2100 by the RCP 8.5 (IPCC, 2014) and to analyze the responses of the coralline alga Ellisolandia elongata (Rodophyta, Corallinales) and its associated fauna. Ellisolandia elongata, formerly known under the name Corallina elongata (see Cormaci et al., 2017 for an appraisal of synonymies) is widely distributed in the North-Eastern Atlantic and in the whole Mediterranean Sea (Bressan and Babbini, 2003), where it represents one of the most important bioconstructors. It can create tridimensional biogenic structures which are developed on vertical shady rock faces, from the surface to one meter deep, in high energy areas (i.e., rocky bottoms exposed to waves and currents) (Bressan and Babbini, 2003).
In particular, we analyzed the combined effects of GW and OA on: (1) E. elongata linear extension; (2) E. elongata structural integrity; (3) community structure of the associated fauna.
This study aimed to approach the responses to climate change (CC) at the community level, and highlighted the vulnerability to the dominant combination of climate drivers occurring at specific habitats. Despite its importance, the response to global changes at community level has been still poorly investigated, thus, the need for field observations and long-term experiments to understand the emergence of different levels of biological responses and their feedbacks is a priority, in order to design appropriate mitigation actions (Pörtner et al., 2014).
Materials and Methods
Target Algal Species
Ellisolandia elongata (J. Ellis and Solander) K. R. Hind and G. W. Saunders is a red alga belonging to the order Corallinales, family Corallinaceae. Reddish-lilac to whitish-pink in color, it has branching, pinnate flexible fronds and an erect thallus attached to the rocky substrate by a crustose holdfast (Figure 1). The fronds are made of small calcified segments (intergenicula), which are separated from one another by uncalcified nodes (genicula) (Babbini and Bressan, 1997; Bressan and Babbini, 2003).
Being adapted to variable habitats (e.g., the intertidal rocky pools of the northeastern Atlantic), this species can experience large fluctuations in the physico-chemical factors, and therefore it has been suggested to have advantages, under the current GW and OA scenario, over other coralline algae adapted to more stable environments (Egilsdottir et al., 2013).
Study Site
The study area is located in the Gulf of La Spezia (North-West Mediterranean Sea), within the Natural Regional Park of Portovenere and the Islands (Palmaria, Tino, and Tinetto). The presence of vertical rocky walls as well as sandy shoals, exposed and sheltered sites, make the Gulf an important source of marine biodiversity promoted and maintained by several ecosystem engineers, including seagrass such as Posidonia oceanica and calcifying bioconstructors such as coralline algae, corals and bryozoans (Cocito et al., 2000, 2002; Peirano et al., 2005; Nannini et al., 2015). The sampling site is located in the Palmaria Island (surface area: 1.89 km2), at the locality ‘Cala Grande’ (north-western side of the island: 44° 2.366′ N, 9° 50.519′ E; Figure 2). This area is characterized by an extremely limited tidal range, around 10–40 (up to 50) cm. The salinity in this area is rather constant, around 36.8–36.9 PSU (Gasparini et al., 2009), whereas surface temperatures range between 12 and 13°C in winter (minimum values) and the maximum value of 26–27°C in summer (maximum values). The minimum–maximum values recorded in March–April 2015 were 13–15°C; in the end of September–October 2015 20–24°C. Mean pH value was 8.1. Due to seawater circulation, current intensity (velocity: 4 cm s-1), and wave actions (height: 20–100 cm), this site is one of the most exposed to seawater physical forces (Ciuffardi et al., 2013).
Sampling and Sample Preparation
The experiment was carried out between July and October 2015. In July, four areas, approximately 10 m far from each other, were randomly allocated on vertical rocks at 50 cm of depth. Using hammer and chisel, we collected 84 samples (5 cm × 5 cm each) of substrate covered by E. elongata turfs. The collected turfs were then placed in separate bags filled with seawater and transported to the laboratory inside a thermal-box. Out of the 84 samples originally collected, 12 (3 per area, haphazardly chosen) were preserved in ethanol for the identification of the associated fauna, while 72 samples were placed into a thermal bath (150 L) for the acclimatization to the laboratory condition (1 week). At the end of the week, the turfs were stained using Alizarin Red S (Andrake and Johansen, 1980), which has been used for many decades for in vivo labeling (Holcomb et al., 2013; Bensimon-Brito et al., 2016), by placing them in aerated (airstone pump -Mouse Air Pump 4, Delta, United States) plastic bags with a solution of 0.25 g L-1 Alizarin Red S staining (Fluka, Sigma-Aldrich) for 24 h at 20°C in 12:12 h light-dark cycle. At the end of the staining period, eight experimental aquaria (four for control condition, C1–C4, and four for treatment conditions, T1–T4; Figure 3) were set up with nine E. elongata turfs each by placing the bags straight into the aquaria, in order to avoid the loss of the alga-associated fauna. The alizarin was progressively washed out by in conditions of continuum water exchange, up to its complete removal from the aquaria. Once cleaned, the aquaria were progressively set up to the following experimental conditions: control – current temperature and pH conditions of the Gulf of La Spezia (24.76 ± 0.83°C in August, 23.15 ± 0.90°C in September and 20.57 ± 0.83°C in October; 385 ppmv/pH 8.1; see Supplementary Table S1); treatment – increased temperature (mean monthly temperature +3°C) and decreased pH (pH = 7.75) according to the 2100 scenario (IPCC – scenario RCP- 8.5). For details on the experimental design, system set-up and environmental conditions within the system see Supporting Information S1, S2 and Table S1.
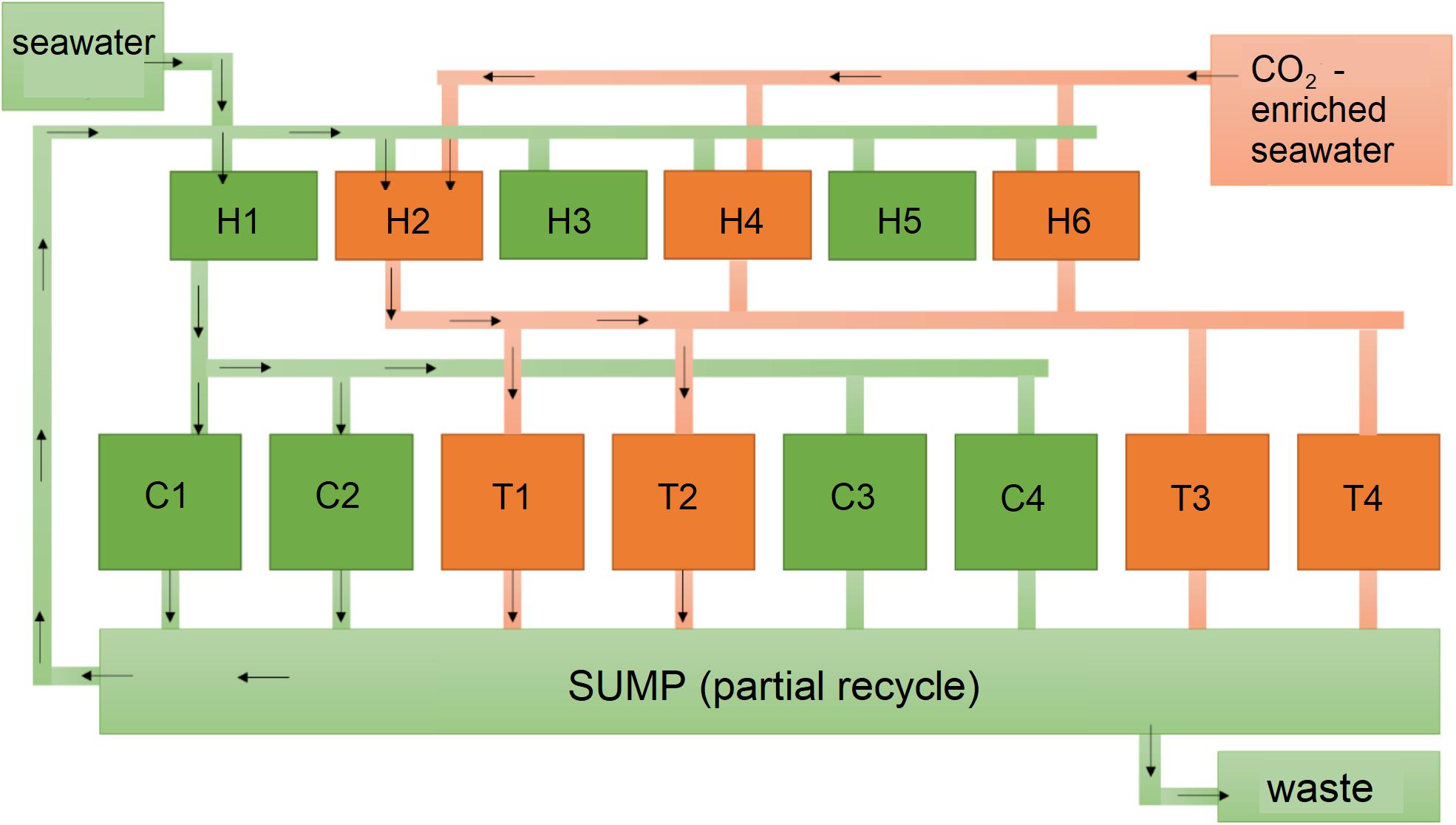
Figure 3. Scheme of the experimental system. Green: control, orange: treatment; H: header tanks; C: control aquaria; T: treatment aquaria. Arrows indicate flow direction.
Linear Growth
From each of the four control aquaria and each of the four treatment aquaria, three E. elongata turfs were sampled after 1 month (on August 24th), three after 2 months (September 22nd), and the last three after 3 months (October 22nd). For each aquarium and each sampling event, 20 fronds were randomly selected from the three turfs and measured to estimate E. elongata linear extension. In particular, after collection the fronds were rinsed with deionized water, dried naturally for 24 h, and then photographed under the stereomicroscope (AZ 100, Nikon, Japan; lens 1x with camera DS-U1, Nikon, Japan), then the distance between the red band left after the staining and the new frond apex was measured using ImageJ® software (Figure 4a).
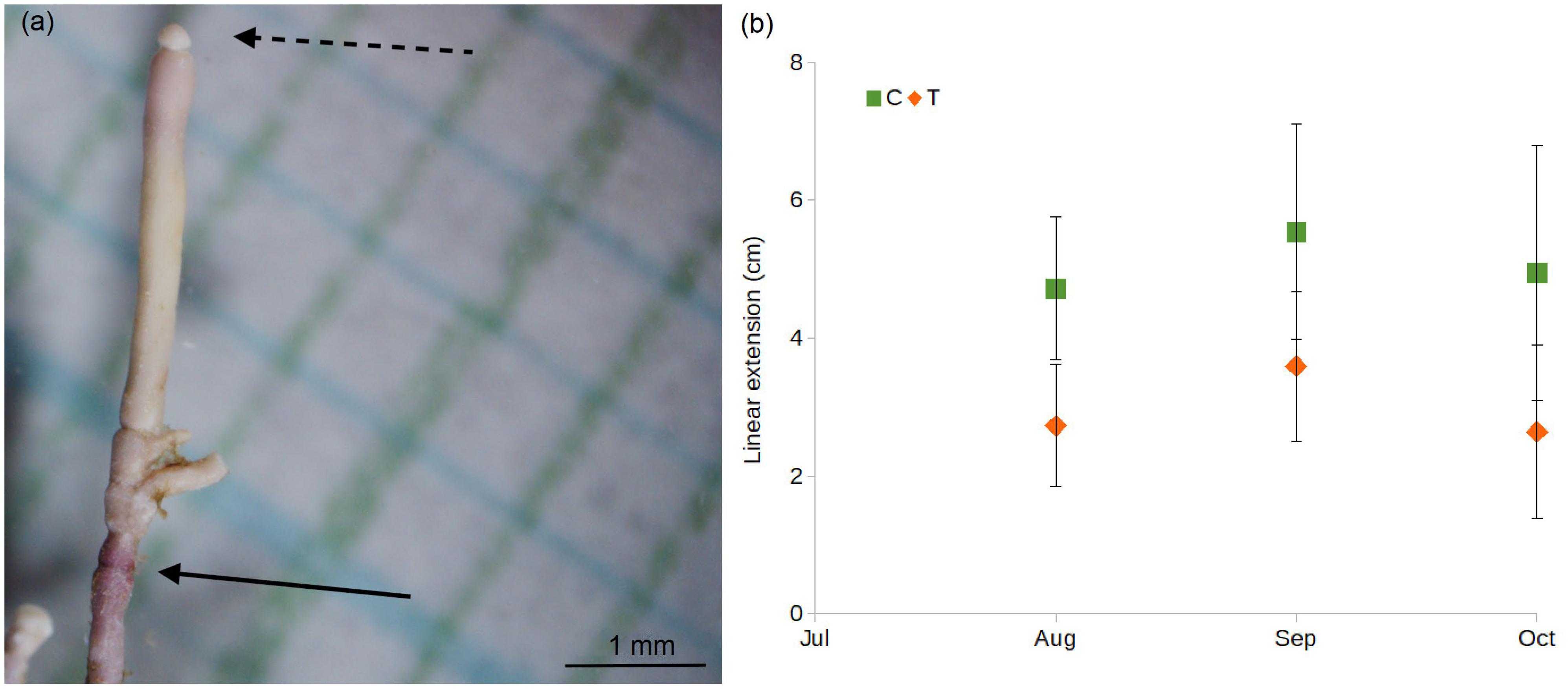
Figure 4. (a) Frond of E. elongata stained with Alizarin Red. Continuous arrow indicates red line of the staining. Dotted arrow indicates the frond tip. Linear extension (i.e., portion of frond grown after the staining) is comprised between dotted and continuous arrow. (b) Linear growth of E. elongata (mean ± SD; N = 80: 20 fronds × 4 aquaria) measured after 1 month (August), 2 months (September), and 3 months (October).
Structural Integrity
Nanoindentation on all samples was performed using Nanotest (Platform 3) indentation instrument (Micromaterials, Ltd., Wrexham, United Kingdom). Instrument description and its working principle is in detailed explained in Beake and Leggett (2002), Beake et al. (2002). Indents were done using standard Berkovich diamond indenter in a load-controlled mode. Maximum force was set to 5 mN, loading and unloading rates were set each to 0.01 mN/s, holding time of 30 s at maximum load was set to minimize the influence of creep. Measurements were performed on the top of three genicula of each algae branch (three branches per set). A matrix of 60 to 120 indents with 50 μm space between each indent was set on each geniculum to measure the distribution of mechanical properties, such as hardness and elastic modulus (see section “Statistical Analyses”). To identify the position of an indent, an integrated optical microscope was used before and after indentation experiments.
Associated Fauna
From each of the four control (C) aquaria and four treatment (T) aquaria, three turfs were sampled on August 24th, three on September 22nd and finally, three on October 22nd, 2015, meaning that at each month 24 turfs were collected in total (12 C + 12 T). Each sample was put into a separate container and preserved in ethanol. To remove the associated macrofauna from the algal fronds, the sample was washed with tap water, while being gently rubbed, and subsequently sieved (0.5 mm mesh size). The sequence of rubbing-washing-sieving was repeated at least three times to ensure the removal of all the vagile fauna associated to E. elongata. After this, the sample was observed under a stereoscope (AZ100, Nikon, Japan) to ensure the complete removal of all individuals.
When each turf sample was cleared by the fauna, the volume of the sample was used as standard unit to compare samples with different size (i.e., tridimensional structures of different size = difference in habitat availability = difference in abundance of associated taxa) created by each algal turf. Each sample was immersed in a 1000 cm3 beaker, filled with tap water, and the volume was calculated as difference between final and initial volume (Pereira et al., 2006; Izquierdo and Guerra-García, 2011).
Samples of associated vagile fauna were preserved in 70% ethanol and analyzed under a stereomicroscope (WILD M5A, Heerbrugg); individuals were counted and divided in different higher-rank taxonomic groups, including both calcifying (i.e., molluscs, crustaceans, echinoderms) and non-calcifying organisms (i.e., polychaetes, nematodes). The only exception was represented by the bivalve Mytilus galloprovincialis Lamarck 1819, a semi-sessile species representing the most abundant mollusc in natural conditions, which was included within mollusc counting. Peracarid crustaceans and polychaete annelids, representing the dominant component of the vagile assemblage, were identified at species level and counted. The abundance of each taxon was standardized to the volume of the sample to obtain relative abundance values.
Statistical Analyses
An orthogonal design was employed to test the null hypotheses that (1) linear extension of E. elongata fronds and (2) their structural integrity and (3) the abundance and diversity of the fauna associate to E. elongata turfs did not vary at different spatial and temporal scales under different experimental conditions (control: current monthly mean temperature and pH = 8.10; treatment: the monthly mean temperature +3°C and pH = 7.75) through time (3 months). Each experimental condition has been replicated four times (e.g., four aquaria for control and four for treatment). For the structural integrity analysis only three replicates were used.
Linear Extension
Differences in linear extension between the levels of the factors ‘condition’ (fixed; two levels: C and T) and ‘time’ (random; three levels: August, September, October) were estimated using the generalized linear mixed model (GLMM). The factor ‘aquaria’ (e.g., random) had been tested separately and excluded from the analyses due to the non-significant differences encountered.
Normality and homoscedasticity were assessed by visually checking the residuals distributions and relation versus predicted values (Zuur et al., 2007). A more formal Levene’s test was also used to assess the variance homogeneity. When the test was significant (p < 0.05), transformations (square root, logarithmic) were applied. In the case of failure of the transformation, the more stringent criterion of α < 0.01 was applied (Underwood, 1997). Post hoc Student-Newman-Keuls (SNK) tests were performed whenever a significant difference was found. These analyses were performed using Statistica® v.7.
Nano Indentation
During nanoindentation experiments a series of force vs. displacement curves were recorded. The analysis was performed using analytical software provided by MicroMaterials, where the unloading portion of the curve was fitted to a power law function to determine hardness and elastic modulus of algae samples (Oliver and Pharr, 1992).
Sample hardness (H) was calculated from the maximum load (Fmax) and projected area of contact, Ac, determined through a series of indentations at different loads on calibration sample of fused silica, by:
Young’s modulus (or elastic modulus), E, of the sample was determined from
where v is the Poisson’s ratio of the sample, Er is the reduced modulus of the sample derived from the load vs. displacement curves (Oliver and Pharr, 1992), vi is the Poisson’s ratio of the indenter (0.07) and Ei is the Young’s modulus for the indenter (1141 GPa). As Poisson’s ratio of algae are not known, reduced indentation modulus (Er) will be reported in this paper.
Maps of elastic modulus and hardness were generated to determine the distribution of the mechanical properties. These maps were further processed by eliminating values obtained on epoxy resin as well as where surface defects interfered with points of measurement. Statistical histograms of modulus and hardness were also obtained, and mean values were calculated (Figure 5).
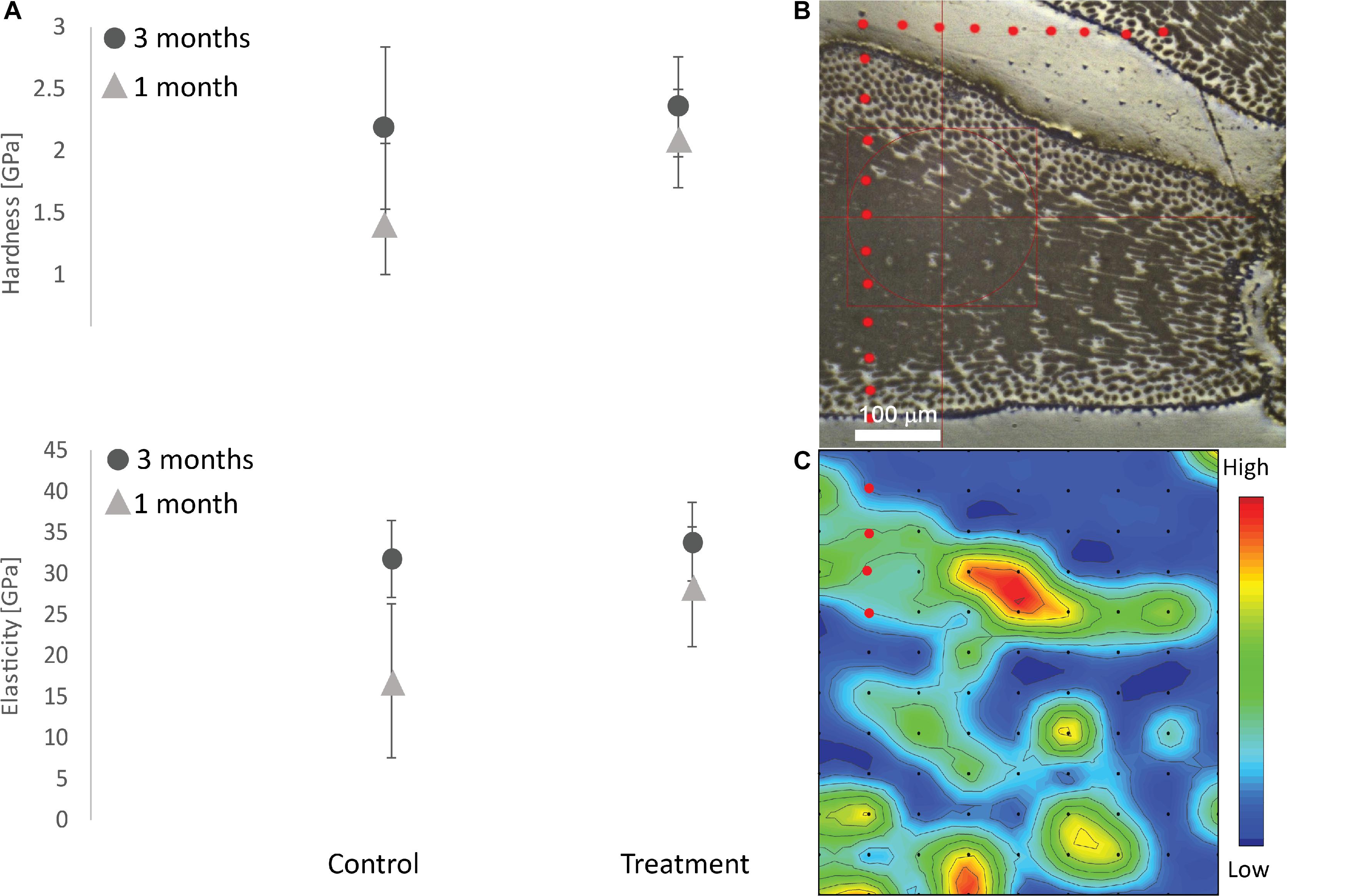
Figure 5. Structural analysis of the E. elongata skeleton. (A) Elastic modulus and hardness on thalli growing under different conditions and times (H: C1month = 1.41 ± 1.4; C3months = 2.18 ± 0.69; T1month = 2.41 ± 1.1; T3months = 2.4 ± 0.7; E: C1month = 19.9 ± 16.2; C3months = 31.7 ± 8.09; T1month = 28.4 ± 12.5; T3months = 33.8 ± 8.3; N = 3 fronds, 60 × 120 indents each). (B) Optical images of a section of the skeleton. Red dots represent the frame of the indents. (C) Example of the distribution of the hardness within the skeleton. Red dots represent the frame of the indents.
Associated Fauna
Relative abundances of taxonomic groups were compared for the two factors ‘condition’ and ‘time’ (see section “Linear Extension”) by using multifactorial ANOVA (Underwood, 1997). Rare taxonomic groups were excluded from the analysis. Prior the application of multifactorial ANOVA, a data exploring protocol (Zuur et al., 2010) was applied to test for presence of anomalous values, heterogeneity of variance and dependence among observations. In case of violation of ANOVA assumptions, generalized least squares (GLSs) method (Aitken, 1935) based on restricted maximum likelihood (REML) was applied according to Zuur et al. (2009) protocol. The method allowed the corrections of the heterogeneity of residuals respect to the factors, thus respecting normality and homoscedastic assumptions.
The model selection was based on Akaike Information Criterion (AIC; Akaike, 1974) and the model with lower AIC value was selected. These analyses were performed with R © (R Core Team, 2016).
The effects of the experimental conditions on the vagile fauna community structure were also tested via permutational multivariate analysis of variance (PERMANOVA; Anderson, 2001), based on a Bray–Curtis similarity matrix. Rare species were removed from the data matrix. Data were transformed via square root, then zero-Adjusted Bray–Curtis method (Clarke et al., 2006) was applied to create a dissimilarity matrix. This approach was necessary in order to avoid indefinite values caused by the presence of double zero in the main matrix. The PERMANOVA test was applied to clarify differences between treatments and among months, and when a significant factor was found, a pairwise test was applied to identify the source of the differences. The SIMPER test was used to identify species mostly contributing to the dissimilarity between the significant factors obtained by PERMANOVA. A non-metric multi-dimensional scaling (nMDS) was plotted for each of the two groups, showing the variation in time of the peracarids and polychaetes under C and T conditions. These analyses were carried out on Primer 6.1.13 (Clarke and Gorley, 2006, 2015).
Results
Linear Extension
The measurement of 480 E. elongata fronds [20 fronds × 8 aquaria (4C + 4T) × 3 months] showed that the species maintained a positive growth trend after 1 month (July–August) as well as after 2 months (July–September) in both C and T conditions (Figure 4b), with an increase from August to September of ΔAug-Sept = 0.84 cm and ΔAug-Sept = 0.87 cm, respectively. At the end of the experiment (October), after 3 months of exposure, the linear extension was 4.94 ± 1.85 cm (C) and 2.64 ± 1.26 cm (T). Compared to the beginning of the experiment (July), in October the fronds maintained a positive growth in C conditions (ΔAug-Oct = 0.22) whereas a decrease under T condition was shown (ΔAug-Oct = -0.08 cm).
Data analyses revealed significant differences between conditions (F1 = 177.48, p < 0.01) and among months (F2 = 11.99, p < 0.01), and post hoc (SNK) showed significant differences after 1 and 2 months of exposure, in August and September (p < 0.01).
Structural Integrity
For the structural integrity analysis of the algal thallus, only the second genicula of each branch were analyzed in order to have full calcified cells. The hardness (H) and the elastic modulus (E) of the thallus in C condition after 1 month were 1.41 ± 1.4 SD GPa and 19.86 ± 16.92 SD Gpa, respectively, while after 3 months they were 2.18 ± 0.70 SD GPa and 31.80 ± 8.09, respectively (Figure 5A). Paired t-test showed no significant difference between 1 and 3 months of culturing (H: p = 0.31, df = 2; E: p = 0.19, df = 2) in C conditions. No significant difference was also found in the algae cultured in T conditions (Paired t-test-H: p = 0.69, df = 2; E: p = 0.52, df = 2): H and E of the thallus after 1 month were 2.41 ± 1.1 SD GPa and 28.40 ± 12.50 SD Gpa, respectively, while after 3 months they were 2.36 ± 0.70 SD GPa and 33.86 ± 8.30 SD GPa. No significant difference in the overall structural integrity (E and H) was found between C and T after both 1 and 3 months (Paired t-test-H 1 month: p = 0.64, df = 2; H 3 months: p = 0.81, df = 2; Paired t-test-E 1 month: p = 0.66, df = 2; H 3 months: p = 0.82, df = 2).
Associated Fauna
The macrofauna found on E. elongata turfs at Palmaria was characterized by dominance of molluscs and crustaceans over other groups (Figure 6A).
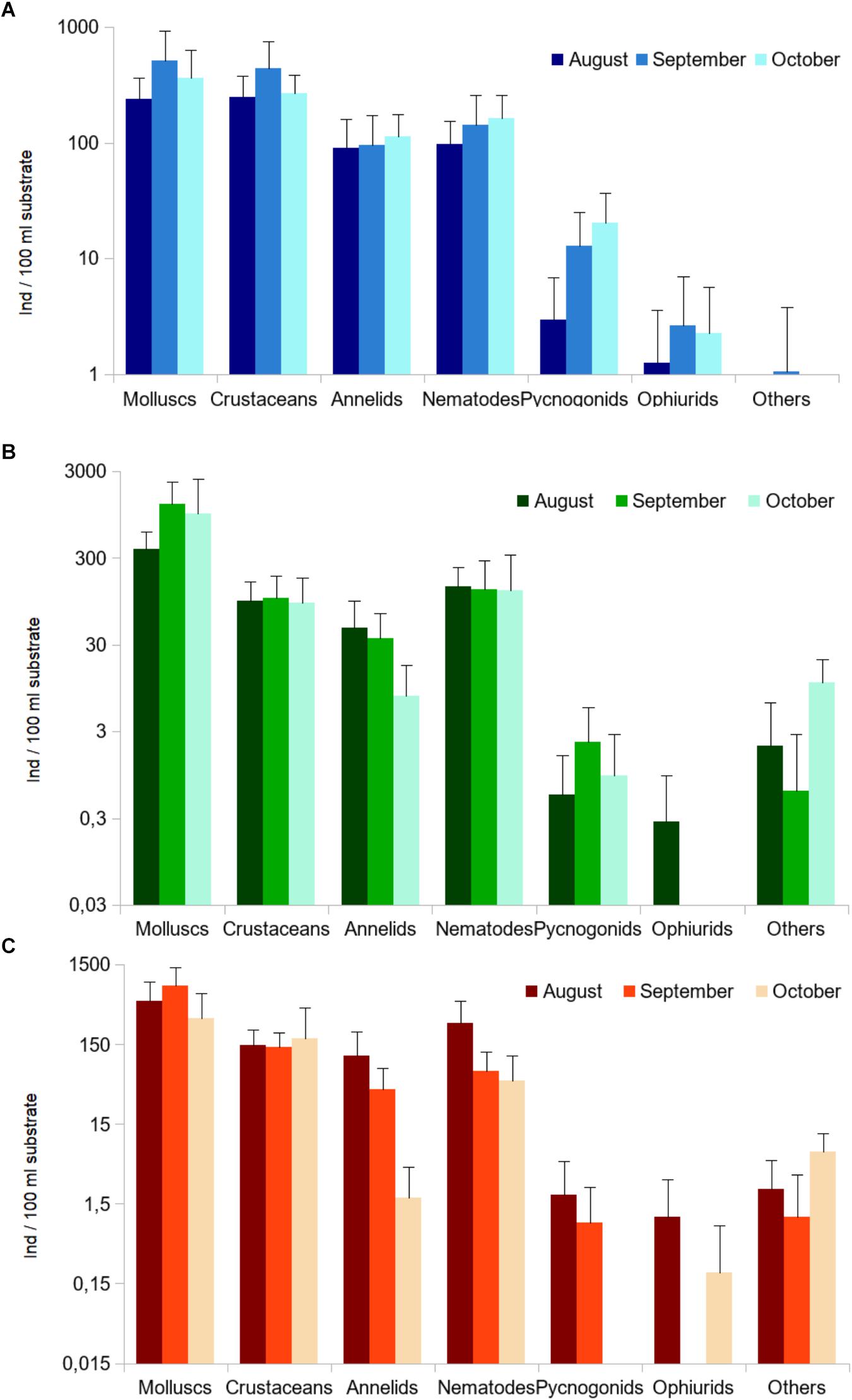
Figure 6. Relative abundance (mean ± SD) of the main taxa associated to E. elongata, from August to October 2015 (on a logarithmic scale; please note that scales differ among graphs); (A) Palmaria island (N = 15: 5 sites × 3 replicates), (B,C) laboratory aquaria (N = 12: 4 aquaria × 3 replicates), under (B) control and (C) treatment conditions. Number of individuals standardized over a 100 ml volume of substrate. The category “others” includes nemerteans, sipunculids, and platyhelmints.
A similar pattern could be observed in the laboratory aquaria, both in C (Figure 6B) and T conditions (Figure 6C), although prominence of molluscs was more striking in the laboratory than in the field. For most groups, relative abundance was much higher in C than in T aquaria.
When looking at the species composition, peracarid crustaceans associated to E. elongata at Palmaria island were highly diverse, exhibiting 23 different species (see Supplementary Table S2; see also Supporting Information S3 for an appraisal on some interesting faunal entities found in the samples).
In each sample, composed by a single turf of E. elongata, we found 22 ± 14 species on average per sample (values standardized over 100 cm3 volume; Figure 7A). In the laboratory samples, the total number of peracarid species was lower than in the field, namely 7.5 ± 6.0 species on average (over 100 ml), but with differences among months, both in C and T aquaria (Figures 7B,C). As regards annelids, the analysis to species level showed that each sample had 14.5 ± 9.0 species on average (over 100 ml volume; Figure 7A; see also Supplementary Table S3). The laboratory aquaria hosted a notably high richness of polychaete species (30), with 7.5 ± 7.0 species on average per sample, yet with differences among months (Figures 7B,C).
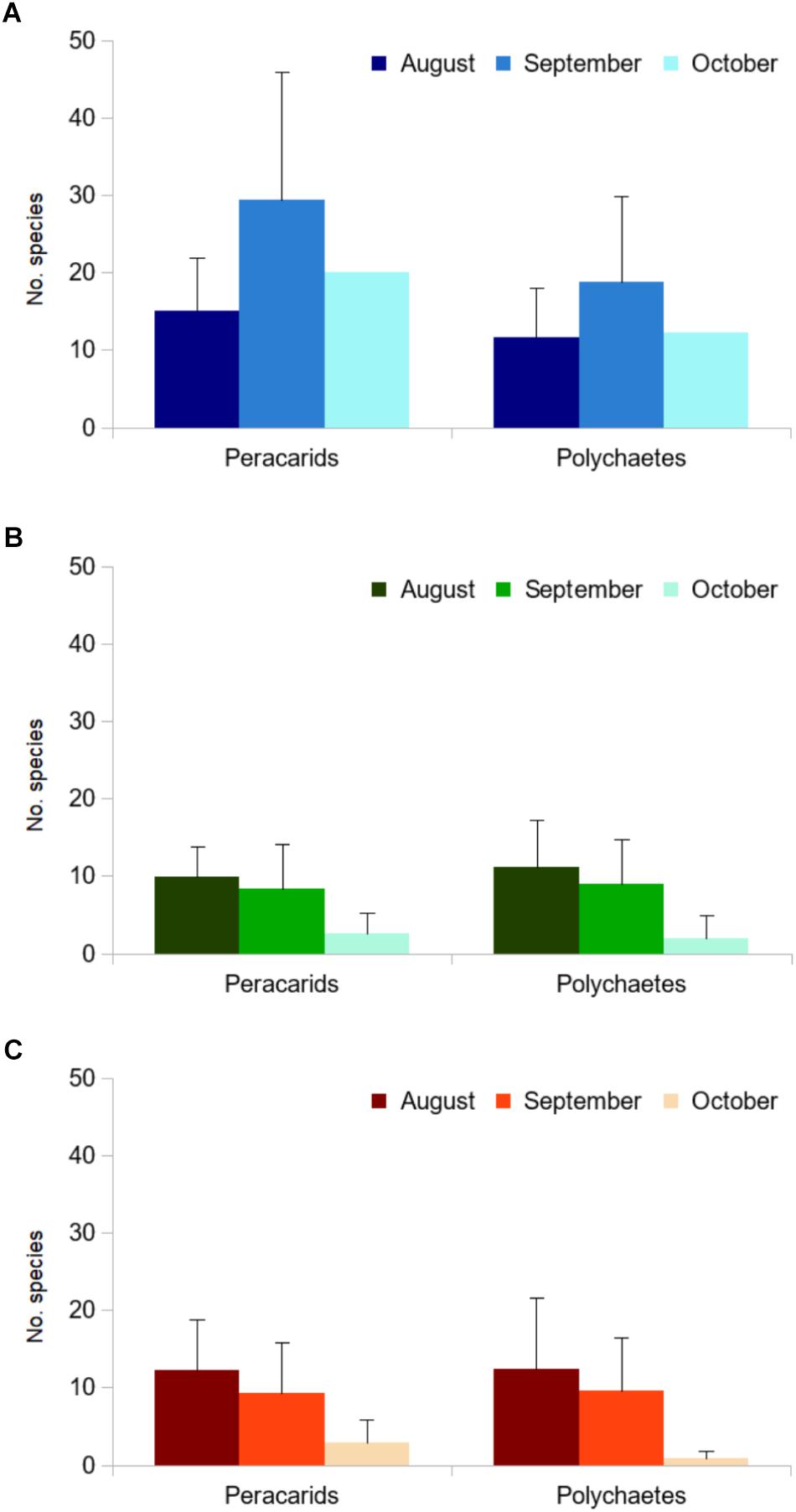
Figure 7. Number of peracarid and polychaete species (mean ± SD) associated to E. elongata, from August to October 2015. (A) Palmaria island (N = 15: 5 sites × 3 replicates), (B,C) laboratory aquaria (N = 12: 4 aquaria × 3 replicates), under (B) control and (C) treatment conditions. Number of species standardized over a 100 ml volume of substrate.
Analysis of variance based on abundance values of the macro-groups revealed only two significant responses of single taxa to treatment (C vs. T conditions) and months (August vs. September vs. October). The abundance of crustaceans differed significantly between C and T aquaria, and polychaetes decreased significantly their abundance with time (Table 1). No significant differences emerged for molluscs and nematodes; other groups were not tested due to insufficient number of specimens.
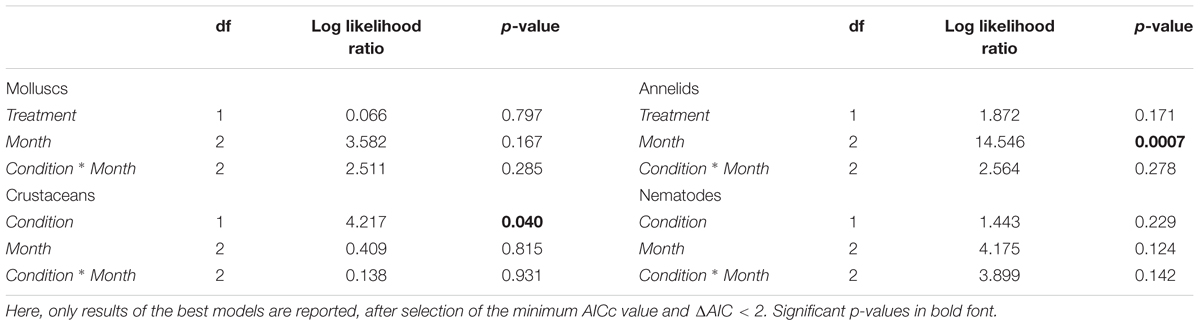
Table 1. Results of linear multifactorial ANOVA (method GLS and REML) based on abundance values of the four major benthic groups investigated, tested for two orthogonal factors: condition (two levels: control and treatment) and month (three levels: August, September, October).
The PERMANOVA analysis on the community structure of macro-groups, based on the Bray–Curtis similarity index, showed no significant response to the experimental conditions (C vs. T), yet highlighting differences in the abundance of the taxa along the 3 months of experiment (Table 2).
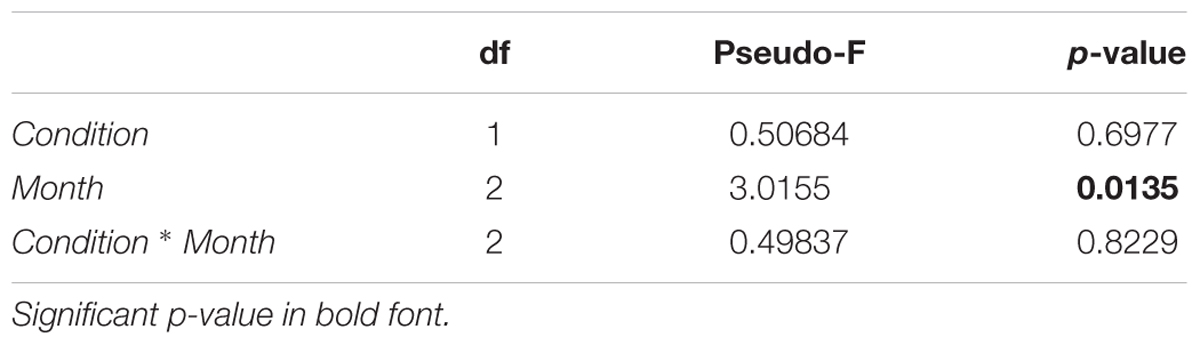
Table 2. Results of PERMANOVA based on Bray–Curtis similarities for macro-groups, tested for two orthogonal factors: condition (two levels: control and treatment) and month (three levels: August, September, October).
A different picture emerged when analyzing more in depth the species composition of peracarids and polychaetes assemblages with PERMANOVA. The interaction between condition and month was significant in shaping the community structure of peracarids (Table 3). The pairwise test applied to the interaction factor showed clear differences between all the considered pairs of months, indicating a shift in the peracarid community along the period, under both conditions. The SIMPER analysis identified the species providing the highest contributions to the dissimilarity among months and between experimental conditions: the amphipods Elasmopus pocillimanus and Jassa marmorata, and the isopod Paranthura costana. In particular, E. pocillimanus gave the highest contribution (up to 44.0%) to the dissimilarity between C and T conditions across months, while J. marmorata and P. costana contributed up to 20.6 and 36.7%, respectively. However, the two latter ones decreased in abundance under T conditions, showing a negative response to the simulated conditions of reduced pH and increased temperature. Conversely, the average abundance of E. pocillimanus was higher in T than in C aquaria.
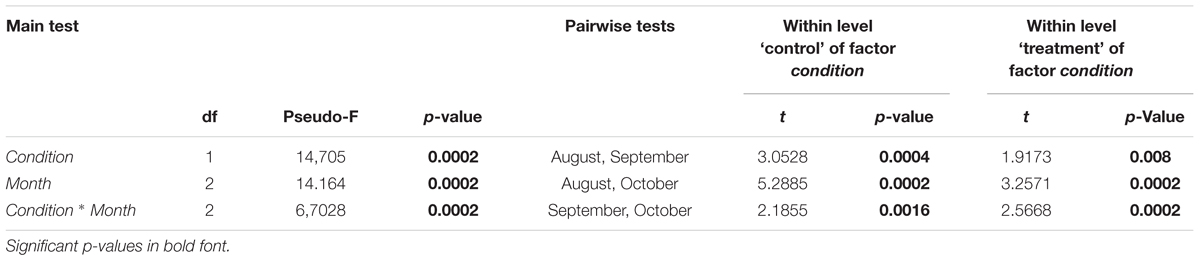
Table 3. Results of PERMANOVA based on Bray–Curtis similarities for peracarids: main test (left) and pairwise test (right), tested for two orthogonal factors: condition (two levels: ‘control’ and ‘treatment’) and month (three levels: August, September, October).
For polychaetes, instead, the interaction did not appear significant, despite significant differences observed for both factors (condition and month; Table 4). The pairwise test applied to the month factor showed significant differences between all the considered pairs of months, reflecting the progressive reduction in the polychaete abundances along the experiment period (they were absent from 11 replicates out of 24 at the end of the experiment, in October). According to the SIMPER test, the species mostly contributing to the dissimilarity among months were Syllis gracilis (19.7% between August and September and 24.7% between August and October), Amphiglena mediterranea (18.0% between August and September and 19.3% between August and October) and Perinereis cultrifera (15.0% between August and September and 18.6% between August and October). In fact, their average abundance progressively decreased with time. The nMDS revealed some separation between control and treatment samples for the peracarid assemblage (Figure 8A), with their dissimilarity increasing with time, thus confirming that the interaction between the two factors (condition × month) is important in shaping the peracarid assemblages. This pattern is less clear when the polychaetes assemblage is taken into account, possibly because of the low number of specimens recorded in the majority of replicates (Figure 8B).

Table 4. Results of PERMANOVA based on Bray–Curtis similarities for polychaetes: main test (left) and pairwise test (right), tested for two orthogonal factors: condition (two levels: ‘control’ and ‘treatment’) and month (three levels: August, September, October).
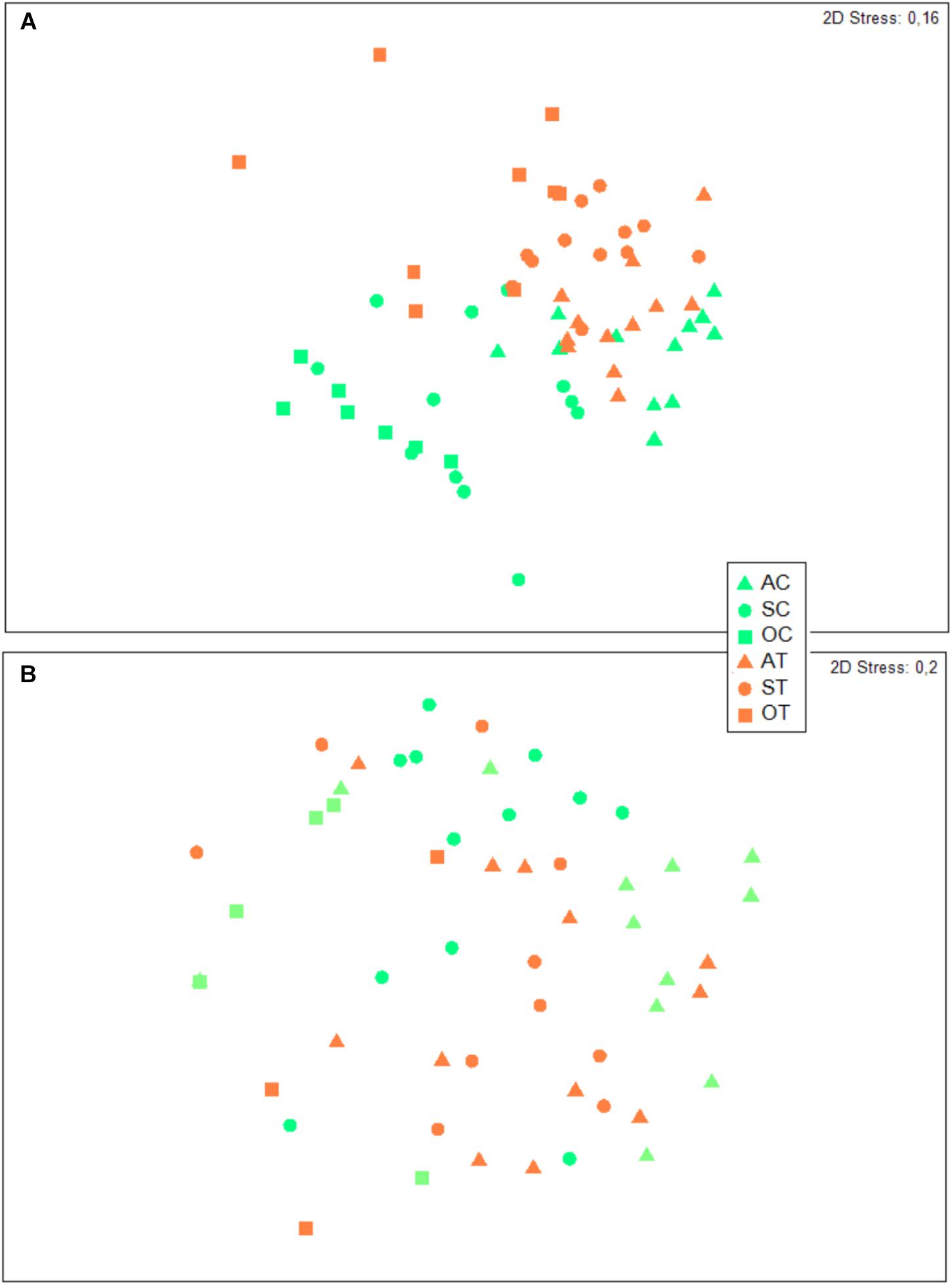
Figure 8. Non-metric multidimensional scaling plots of peracarids (A) and polychaetes (B) assemblages associated to E. elongata in experimental aquaria. A = August; S = September; O = October; C = Control; T = Treatment.
Discussion
In the Mediterranean Sea, previous studies on the effects of either warming or acidification on benthic communities have focused on very specific study-cases, reporting mass mortality events after heat wave events (e.g., Garrabou et al., 2009) and simplification of community structure along gradients of decreasing pH observed at volcanic CO2 vent habitats (e.g., Cigliano et al., 2010; Kroeker et al., 2011; Porzio et al., 2011). Our study instead explores the combined effect of both pH and temperature alterations, experimentally exposing the organisms to values expected for 2100 (IPCC, 2014 –RCP- 8.5). We target the habitat-forming alga E. elongata and its associated fauna, finding that high CO2 and high temperature conditions are responsible for the decreased linear growth rate in the alga and of structural changes in the benthic community; this effect is even more evident when taking into account the specific composition of selected taxonomic groups.
Physiological and structural responses withstanding the effects of ocean acidification have been already identified in coralline algae (Ragazzola et al., 2013, 2016; McCoy and Ragazzola, 2014), but little is still known on the algal structural responses in high CO2 and high temperature environments.
In this study E. elongata showed a decrease in linear growth rates under high CO2 and high temperature but, interestingly with no significant changes in the structural integrity of the skeleton. Due to their very densely packed and highly branched fronds, coralline algae represent the extreme end of algal structural complexity (Coull and Wells, 1983; Davenport et al., 1999). The unchanged structural integrity of the skeleton could represent a strong selective advantage allowing E. elongata to survive in changing environments. The partial preservation of the structural complexity, although hindered by the reduction in linear growth rate, could still provide a suitable habitat for the associated fauna.
However, the ecological costs of a possible shift in the energy budget from growth extension to maintaining structural integrity, like in Lithothamnion glaciale (Ragazzola et al., 2016), will need further investigations. For instance, during this experiment we were not able to assess if the decrease in linear growth rates was related to a decrease in the branching of the algae, which would in turn reduce the space availability for the macrofauna.
We verified that in the Palmaria island, the habitat-creator species E. elongata hosts a diverse and highly abundant community of small mobile invertebrates, as observed in similar habitats elsewhere (e.g., see Cowles et al., 2009; Guerra-García et al., 2009, 2012; Izquierdo and Guerra-García, 2011; Zakhama-Sraieb et al., 2011; Berthelsen et al., 2015). Besides the high number of individuals recorded from field turfs, belonging to various taxa (up to several 1000 individuals per 100 cm3 of substrate), our samples host peculiar communities of crustacean peracarids and polychaetes and possibly even endemic species (see Supporting Information S3 and Tables S2, S3).
When exposed to high CO2 and high temperature environment, the whole associated community, when identified at a low taxonomic level, did not show a clearly discernible response, but the two rich assemblages of peracarids and polychaetes were used as models to study the effects of CC drivers. In fact, the analysis of the species composition within at least one selected taxon (peracarids) did prove that reduced pH and increased temperature can affect community structure, thus providing empirical evidence to the predictions made by Sunday et al. (2017) regarding the effects of GW and OA on communities associated to habitat-forming species, based on a literature review. In particular, here peracarid crustaceans exhibited significant changes in species composition under treatment conditions, also depending on the exposure time to altered pH and temperature. In almost all treatment samples, the species dominating the assemblages until the end of the experiment (October) was the amphipod E. pocillimanus, which has already been reported to withstand stressful environmental conditions (Sparla et al., 1993; Zakhama-Sraieb et al., 2011), including low pH (Scipione et al., 2017). Under a control condition, instead, different species were dominant: the isopod P. costana, a predator, and the amphipod J. marmorata, a suspension feeder. Interestingly, most isopods that occurred in the control aquaria (the herbivorous Dynamene spp., Ischyromene lacazei, the fish parasite Gnathia sp., the predator Kupellonura serritelson), were very rare or absent under treatment conditions. This could be possibly explained by the fact that the exoskeleton of isopods is characterized by high magnesium calcite (Munguia and Alenius, 2013), thus more soluble to OA (Andersson et al., 2008), possibly confirming previous findings on isopods vulnerability to CC (Munguia and Alenius, 2013; Garrard et al., 2014; Wood et al., 2014; Turner et al., 2016). In particular, Turner et al. (2016) observed that some isopod species alter their metabolism to regulate the internal acid–base balance under natural low pH conditions (at CO2 vents), but it has also been suggested that indirect effects of acidification, such as changes in food quality, or competition and predation interactions (Garrard et al., 2014) may affect the isopods’ response to OA. Given the large number of isopod species inhabiting marine ecosystems and the wide range of trophic functions they express (Poore and Bruce, 2012), as well as their role in transferring energy from the benthic to the pelagic compartment (as food item for fish), it would be important to better elucidate their response to changing environmental conditions.
As regards polychaetes, it was shown that under natural low pH conditions (CO2 vents) they exhibit species-specific responses to OA, which are related to their functional traits (Gambi et al., 2016). However, in our study it was not possible to quantify the response of this taxon to the treatment conditions, since the aquarium effect covered the possible effects of lowered pH – increased temperature. In fact, although PERMANOVA highlighted significant differences between controls and treatments, at the end of the 3-month experiments, too few individuals survived both in the control and treatment aquaria to highlight any clear pattern.
Possible explanations could be related to the effect of Alizarine Red S staining treatment, which has been shown to negatively affect the growth of corals (Holcomb et al., 2013), or type of feeding provided in the aquaria (Chlorella sp. mix, see Supporting Information S1), which may not have been suitable for the carnivorous polychaete species (e.g., syllids and phyllodocids) included in the assemblage. Moreover, while the majority of peracarid crustaceans reported in this study have direct development with parental care, which allows for a successful recruitment under experimental conditions, the majority of the reported polychaetes are characterized by pelagic reproductive phases (epitoke stages, pelagic larvae, or both), and a natural mortality under experimental conditions would not be compensated by the recruitment. Different reproductive strategies might then account for the different response of the two taxonomic groups.
Our results partially overlap with those obtained by Hale et al. (2011) with a similar experiment, yet we acknowledge that in our case community responses are less obvious. In Hale et al. (2011), however, the treatment conditions may have been exacerbated by the fact that invertebrates had been grown on artificial substrates (nylon pan scourers), whereas the natural substrate (living algal turfs) used in the present experiment could have mitigated the effect of acidification, through local alteration of the water chemistry at the micro-habitat scale. Further testing is required to clarify this aspect, as well as to test whether reduced pH and increased temperature, when combined, exert an additive, antagonistic, or synergistic effect (Crain et al., 2008).
Conclusion
Our study shows that the species-rich Mediterranean assemblages associated to E. elongata should be considered at risk. The combined effect of low pH and high temperature that are expected for the future can impair algal growth and generate structural changes at the level of the associated benthic community, especially concerning selected taxonomic groups. Under future CC scenario, a rich and diverse mobile associated fauna shifts to a more simplified and depleted community. Despite preliminary and subject to the limitations of experimental conditions, our results show (i) that E. elongata is able to somewhat counter-act the effect of combined stressors (acidification and increased temperature); and (ii) that these stressors cause shifts in the associated assemblages toward a less diverse structure, with possible dominance of the more opportunistic species. These conclusions suggest two important pathways of further investigation: (1) the loss of some species and the resilience of others, at different trophic levels, need to be better understood in order to clarify the potential of species interactions in mediating the effects of CC; (2) analogously to seagrass meadows (Koweek et al., 2018), plastic and metabolic responses of calcareous, habitat-forming algae are key elements that need to be explored for studying mitigation strategies at local scale.
Author Contributions
FR, AM, and CL designed the study. MN carried out the experimental work with help from GC and CL. AM, MCM, FG, JL, AC, and LM did the taxonomical work. CJ and JZ performed the analyses on structural integrity. CL, MN, CV, and AM performed statistical analyses with help from JL and LM. FR and AM drafted the first version of this manuscript assisted by CL. All authors contributed to the later versions of the manuscript and approved the final version for publication.
Funding
The present project was supported by internal funding of ENEA, University of Pavia and University of Portsmouth.
Conflict of Interest Statement
The authors declare that the research was conducted in the absence of any commercial or financial relationships that could be construed as a potential conflict of interest.
Acknowledgments
The authors would like to thank students who contributed to the project: Tirzah Bottomley, Maurizio Florio, Maria Murcia Diaz, Lorenzo Pasculli. CL would like to thank her colleagues at ENEA: G. Raiteri, A. Bordone, A. Peirano, and S. Cocito for the technical and scientific support. Three reviewers have provided significant inputs to this work through constructive criticism.
Supplementary Material
The Supplementary Material for this article can be found online at: https://www.frontiersin.org/articles/10.3389/fmars.2019.00106/full#supplementary-material
References
Aitken, A. C. (1935). On least squares and linear combinations of observations. Proc. R. Stat. Soc. 55, 42–48.
Akaike, H. (1974). A new look at the statically model identification. IEEE Trans. Automation Control 19, 716–723. doi: 10.1109/TAC.1974.1100705
Anderson, M. J. (2001). A new method for non-parametric multivariate analyses of variance. Aust. J. Ecol. 26, 32–46.
Andersson, A. J., Mackenzie, F. T., and Bates, N. R. (2008). Life on the margin: implications of ocean acidification on Mg-calcite, high latitude and cold-water marine calcifiers. Mar. Ecol. Prog. Ser. 373, 265–273. doi: 10.3354/meps07639
Andrake, W., and Johansen, H. W. (1980). Alizarin red dye as marker for measuring assemblages: an experimental study from Mediterranean rocky shore. J. Exp. Mar. Biol. Ecol. 215, 271–290.
Babbini, L., and Bressan, G. (1997). Recensement des corallincées de la Mer Mediteranée et consideration sphytogeographiques. Bibl. Phycol. 1, 103–121.
Beake, B., and Leggett, G. J. (2002). Nanoindentation and nanoscratch testing uniaxially and biaxially drawn poly (ethylene terephtalate) film. Polymer 43, 319–327. doi: 10.1016/S0032-3861(01)00600-0
Beake, B., Leggett, G. J., and Alexander, M. R. (2002). Characterization of the mechanical properties of plasma-polymerized coatings by nanoindentation and tribology. J. Mater. Sci. 37, 4919–4927. doi: 10.1023/A:1020830717653
Benedetti-Cecchi, L., and Cinelli, F. (1994). Recovery of patches in an assemblage of geniculate coralline algae: variability at different successional stages. Mar. Ecol. Prog. Ser. 110:9. doi: 10.3354/meps110009
Bensimon-Brito, A., Cardeira, J., Dionísio, G., Huysseune, A., Cancela, M. L., and Witten, P. E. (2016). Revisiting in vivo staining with alizarin red S-a valuable approach to analyse zebrafish skeletal mineralization during development and regeneration. BMC Dev. Biol. 16:2. doi: 10.1186/s12861-016-0102-4
Berthelsen, A. K., Hewitt, J. E., and Taylor, R. B. (2015). Coralline turf-associated fauna are affected more by spatial variability than by host species identity. Mar. Biodivers. 45, 689–699. doi: 10.1007/s12526-014-0270-z
Bijma, J., Pörtner, H. O., Yesson, C., and Rogers, A. D. (2013). Climate change and the oceans–What does the future hold? Mar. Pollut. Bull. 74, 495–505. doi: 10.1016/j.marpolbul.2013.07.022
Bindoff, N. L., Stott, P. A., AchutaRao, K. M., Allen, M. R., Gillett, N., Gutzler, D., et al. (2013). “Detection and attribution of climate change: from global to regional,” in Climate Change 2013: The Physical Science Basis. Contribution of Working Group I to the Fifth Assessment Report of the Intergovernmental Panel on Climate Change, eds T. F. Stocker, D. Qin, G.-K. Plattner, M. Tignor, S. K. Allen, J. Boschung, et al. (Cambridge, MA: Cambridge University Press), 867–952.
Bressan, G., and Babbini, L. (2003). Biodiversità marina delle coste italiane: corallinales del mediterraneo: guida alla determinazione. Biol. Mar. Mediterr. 10(Suppl. 2), 1–237.
Cigliano, M., Gambi, M. C., Rodolfo-Metalpa, R., Patti, F. P., and Hall-Spencer, J. M. (2010). Effects of ocean acidification on invertebrate settlement at volcanic CO2 vents. Mar. Biol. 157, 2489–2502. doi: 10.1007/s00227-010-1513-6
Ciuffardi, T., Giuliani, A., Barsanti, M., Bordone, A., Cerrati, G., Di Nallo, G., et al. (2013). Quarant’anni di Dati Oceanografici a Cura Del Centro Ricerche Ambiente Marino ENEA S. Teresa: Il Quadro Del Golfo di La Spezia. Distribuzione Storica Dei Dati Dal 1973 al 2013. ENEA Technical Report No. 19. Kista: ENEA.
Clarke, K. R., Somerfield, P. J., and Chapman, M. G. (2006). On resemblance measures for ecological studies, including taxonomic dissimilarities and a zero-adjusted bray-curtis coefficient for denuded assemblages. J. Exp. Mar. Biol. Ecol. 330, 55–80. doi: 10.1016/j.jembe.2005.12.017
Cocito, S., Bedulli, D., and Sgorbini, S. (2002). Distribution patterns of the sublittoral epibenthic assemblages on a rocky shoal in the Ligurian Sea (NW Mediterranean). Sci. Mar. 66, 175–181. doi: 10.3989/scimar.2002.66n2175
Cocito, S., Ferdeghini, F., Morri, C., and Bianchi, C. N. (2000). Patterns of bioconstruction in the cheilostome bryozoan Schizoporella errata: the influence of hydrodynamics and associated biota. Mar. Ecol. Prog. Ser. 192, 153–161. doi: 10.3354/meps192153
Collins, M., Knutti, R., Arblaster, J., Dufresne, J.-L., Fichefet, T., Friedlingstein, P., et al. (2013). “Long-term climate change: projections, commitments and irreversibility,” in Climate Change 2013: The Physical Science Basis. Contribution of Working Group I to the Fifth Assessment Report of the Intergovernmental Panel on Climate Change, eds T. F. Stocker, D. Qin, G.-K. Plattner, M. Tignor, S. K. Allen, J. Boschung, et al. (Cambridge: Cambridge University Press), 1029–1136.
R Core Team (2016). R: A Language and Environment for Statistical Computing. Vienna: R Foundation for Statistical Computing.
Cormaci, M., Furnari, G., and Alongi, G. (2017). Flora marina bentonica del Mediterraneo Rhodophyta (Rhodymeniophycidae escluse). Bollettino Accademia Gioenia di Scienze Naturali in Catania 50, 1–391.
Coull, B. C., and Wells, J. B. J. (1983). Refuges from fish predation: experiments with phytal meiofauna from the New Zealand rocky intertidal. Ecology 64, 1599–1609. doi: 10.2307/1937513
Cowles, A., Hewitt, J. E., and Taylor, R. B. (2009). Density, biomass and productivity of small mobile invertebrates in a wide range of coastal habitats. Mar. Ecol. Prog. Ser. 384, 175–185. doi: 10.3354/meps08038
Crain, C. M., and Bertness, M. D. (2006). Ecosystem engineering across environmental gradients: implications for conservation and management. AIBS Bull. 56, 211–218.
Crain, C. M., Kroeker, K., and Halpern, B. S. (2008). Interactive and cumulative effects of multiple human stressors in marine systems. Ecol. Lett. 11, 1304–1315. doi: 10.1111/j.1461-0248.2008.01253.x
Crowder, L. B., and Cooper, W. E. (1982). Habitat structural complexity and the interaction between bluegills and their prey. Ecology 63, 1802–1813. doi: 10.2307/1940122
Davenport, J., Butler, A., and Cheshire, A. (1999). Epifaunal composition and fractal dimensions of marine plants in relation to emersion. J. Mar. Biol. Assoc. 79, 351–355. doi: 10.1017/S0025315498000393
Díez, I., Muguerza, N., Santolaria, A., Ganzedo, U., and Gorostiaga, J. M. (2012). Seaweed assemblage changes in the eastern Cantabrian Sea and their potential relationship to climate change. Estuar. Coast. Shelf Sci. 99, 108–120. doi: 10.1016/j.ecss.2011.12.027
Dommasnes, A. (1968). Variations in the meiofauna of Corallina officinalis L. with wave exposure. Sarsia 34, 117–124. doi: 10.1080/00364827.1968.10413376
Downes, B. J., Lake, P. S., Schreiber, E. S. G., and Glaister, A. (1998). Habitat structure and regulation of local species diversity in a stony, upland stream. Ecol. Monogr. 68, 237–257. doi: 10.1890/0012-9615(1998)068[0237:HSAROL]2.0.CO;2
Dye, A. H. (1993). Recolonization of intertidal macroalgae in relation to gap size and molluscan herbivory on a rocky shore on the east coast of southern Africa. Mar. Ecol. Prog. Ser. 95, 263–263. doi: 10.3354/meps095263
Egilsdottir, H., Noisette, F., Noël, L., Olafsson, J., and Martin, S. (2013). Effects of pCO2 on physiology and skeletal mineralogy in a tidal pool coralline alga Corallina elongata. Mar. Biol. 160, 2103–2112. doi: 10.1007/s00227-012-2090-7
Ellner, S. P., McCauley, E., Kendall, B. E., Briggs, C. J., Hosseini, P. R., Wood, S. N., et al. (2001). Habitat structure and population persistence in an experimental community. Nature 412, 538–543. doi: 10.1038/35087580
Fletcher, W. J., and Underwood, A. J. (1987). Interspecific competition among subtidal limpets: effect of substratum heterogeneity. Ecology 68, 387–400. doi: 10.2307/1939270
Foster, M. S. (2001). Rhodoliths: between rocks and soft places. J. Phycol. 37, 659–667. doi: 10.1046/j.1529-8817.2001.00195.x
Gambi, M. C., Musco, L., Giangrande, A., Badalamenti, F., Micheli, F., and Kroeker, K. J. (2016). Distribution and functional traits of polychaetes in a CO2 vent system: winners and losers among closely related species. Mar. Ecol. Prog. Ser. 550, 121–134. doi: 10.3354/meps11727
Gao, K., Aruga, Y., Asada, K., Ishihara, T., Akano, T., and Kiyohara, M. (1993). Calcification in the articulated coralline alga Corallina pilulifera, with special reference to the effect of elevated CO2 concentration. Mar. Biol. 117, 129–132. doi: 10.1007/BF00346434
Garrabou, J., Coma, R., Bensoussan, N., Bally, M., Chevaldonné, P., Cigliano, M., et al. (2009). Mass mortality in Northwestern Mediterranean rocky benthic communities: effects of the 2003 heat wave. Glob. Chang. Biol. 15, 1090–1103. doi: 10.1111/j.1365-2486.2008.01823.x
Garrard, S., Gambi, M. C., Scipione, M. B., Lorenti, M., Zupo, V., Paterson, D. M., et al. (2014). Indirect effects may buffer negative responses of seagrass invertebrate communities to ocean acidifcation. J. Exp. Mar. Biol. Ecol. 461, 31–38. doi: 10.1016/j.jembe.2014.07.011
Gasparini, G. P., Abbate, M., Bordone, A., Cerrati, G., Galli, C., Lazzoni, E., et al. (2009). Circulation and biomass distribution during warm season in the Gulf of La Spezia (north-western Mediterranean). J. Mar. Syst. 78, 48–62. doi: 10.1016/j.jmarsys.2009.01.010
Gattie, D. K., Smith, M. C., Tollner, E. W., and McCutcheon, S. C. (2003). The emergence of ecological engineering as a discipline. Ecol. Eng. 20, 409–420. doi: 10.1073/pnas.1102712108
Guerra-García, J. M., Cabezas, P., Baeza-Rojano, E., Espinosa, F., and García-Gómez, J. C. (2009). Is the north side of the Strait of Gibraltar more diverse than the south side? A case study using the intertidal peracarids (Crustacea: Malacostraca) associated to the seaweed Corallina elongata. J. Mar. Biol. Assoc. 89, 387–397. doi: 10.1017/S0025315409002938
Guerra-García, J. M., Ros, M., Izquierdo, D., and Soler-Hurtado, M. M. (2012). The invasive Asparagopsis armata versus the native Corallina elongata: differences in associated peracarid assemblages. J. Exp. Mar. Biol. Ecol. 416, 121–128. doi: 10.1016/j.jembe.2012.02.018
Hale, R., Calosi, P., McNeill, L., Mieszkowska, N., and Widdicombe, S. (2011). Predicted levels of future ocean acidification and temperature rise could alter community structure and biodiversity in marine benthic communities. Oikos 120, 661–674. doi: 10.1111/j.1600-0706.2010.19469.x
Harley, C. D., Anderson, K. M., Demes, K. W., Jorve, J. P., Kordas, R. L., Coyle, T. A., et al. (2012). Effects of climate change on global seaweed communities. J. Phycol. 48, 1064–1078. doi: 10.1111/j.1529-8817.2012.01224.x
Heck, K. L. Jr., and Wetstone, G. S. (1977). Habitat complexity and invertebrate species richness and abundance in tropical seagrass meadows. J. Biogeogr. 4, 135–142. doi: 10.2307/3038158
Holcomb, M., Cohen, A. L., and McCorkle, D. C. (2013). An evaluation of staining techniques for marking daily growth in scleractinian corals. J. Exp. Mar. Biol. Ecol. 440, 126–131. doi: 10.1016/j.jembe.2012.12.003
Ingrosso, G., Abbiati, M., Badalamenti, F., Bavestrello, G., Belmonte, G., Cannas, R., et al. (2018). Mediterranean bioconstructions along the italian coast. Adv. Mar. Biol. 79, 61–136. doi: 10.1016/bs.amb.2018.05.001
IPCC (2014). Climate Change 2014: Synthesis Report. Contribution of Working Groups I, II and III to the Fifth Assessment Report of the Intergovernmental Panel on Climate Change. Available at: https://epic.awi.de/id/eprint/37530/.
Izquierdo, D., and Guerra-García, J. M. (2011). Distribution patterns of the peracarid crustaceans associated with the alga Corallina elongata along the intertidal rocky shores of the Iberian Peninsula. Helgol. Mar. Res. 65, 233–243. doi: 10.1007/s10152-010-0219-y
Jokiel, P. L., Rodgers, K. S., Kuffner, I. B., Andersson, A. J., Cox, E. F., and Mackenzie, F. T. (2008). Ocean acidification and calcifying reef organisms: a mesocosm investigation. Coral Reefs 27, 473–483. doi: 10.1371/journal.pone.0154844
Jones, C. G., Lawton, J. H., and Shachak, M. (1997). Positive and negative effects of organism as physical ecosystem engineers. Ecology 78, 1946–1957. doi: 10.1890/0012-9658(1997)078[1946:PANEOO]2.0.CO;2
Kamenos, N. A., Burdett, H. L., Aloisio, E., Findlay, H. S., Martin, S., Longbone, C., et al. (2013). Coralline algal structure is more sensitive to rate, rather than the magnitude, of ocean acidification. Glob. Chang. Biol. 44, 3621–3628. doi: 10.1111/gcb.12351
Kelaher, B. P., Chapman, M. G., and Underwood, A. J. (2001). Spatial patterns of diverse macrofaunal assemblages in coralline turf and their associations with environmental variables. J. Mar. Biol. Assoc. 81, 917–930. doi: 10.1017/S0025315401004842
Kleypas, J., Feely, R., Fabry, V., Langdon, C., Sabine, C. L., and Robbins, L. L. (2006). impacts of ocean acidification on coral reefs and other marine calcifiers: a guide for future research. Atmospher. Res. 18:88.
Kohn, A. J., and Leviten, P. J. (1976). Effect of habitat complexity on population density and species richness in tropical intertidal predatory gastropod assemblages. Oecologia 25, 199–210. doi: 10.1007/BF00345098
Koweek, D. A., Zimmerman, R. C., Hewett, K. M., Gaylord, B., Giddings, S. N., Nickols, K. J., et al. (2018). Expected limits on the ocean acidification buffering potential of a temperate seagrass meadow. Ecol. Appl. 28, 1694–1714. doi: 10.1002/eap.1771
Kroeker, K. J., Micheli, F., Gambi, M. C., and Martz, T. R. (2011). Divergent ecosystem responses within a benthic marine community to ocean acidification. Proc. Natl. Acad. Sci. U.S.A. 108, 14515–14520. doi: 10.1073/pnas.1107789108
Kuffner, I. B., Andersson, A. J., Jokiel, P. L., Rodgers, K. S., and Mackenzie, F. T. (2008). Decreased abundance of crustose coralline algae due to ocean acidification. Nat. Geosci. 1, 114–117. doi: 10.1038/ngeo100
Lawton, J. H. (1983). Plant architecture and the diversity of phytophagous insects. Annu. Rev. Entomol. 28, 23–39. doi: 10.1146/annurev.en.28.010183.000323
MacArthur, R. H., and MacArthur, J. W. (1961). On bird species diversity. Ecology 42, 594–598. doi: 10.2307/1932254
Martin, S., Cohu, S., Vignot, C., Zimmerman, G., and Gattuso, J. P. (2013). One-year experiment on the physiological response of the Mediterranean crustose coralline alga, Lithophyllum cabiochae, to elevated pCO2 and temperature. Ecol. Evol. 3, 676–693. doi: 10.1002/ece3.475
Martin, S., and Gattuso, J. P. (2009). Response of Mediterranean coralline algae to ocean acidification and elevated temperature. Glob. Chang. Biol. 15, 2089–2100. doi: 10.1002/ece3.475
McCoy, S. J., and Ragazzola, F. (2014). Skeletal trade-offs in coralline algae in response to ocean acidification. Nat. Clim. Change 4:719. doi: 10.1038/nclimate2273
Munguia, P., and Alenius, B. (2013). The role of preconditioning in ocean acidification experiments: a test with the intertidal isopod Paradella dianae. Mar. Freshw. Behav. Physiol. 46, 33–44. doi: 10.1080/10236244.2013.788287
Musco, L. (2012). Ecology and diversity of Mediterranean hard-bottom Syllidae (Annelida): a community-level approach. Mar. Ecol. Prog. Ser. 461, 107–119. doi: 10.3354/meps09753
Nannini, M., De Marchi, L., Lombardi, C., and Ragazzola, F. (2015). Effects of thermal stress on the growth of an intertidal population of Ellisolandia elongata (Rhodophyta) from N-W Mediterranean Sea. Mar. Environ. Res. 112, 11–19. doi: 10.1016/j.marenvres.2015.05.005
Oliver, W. C., and Pharr, G. M. (1992). An improved technique for determining hardness and elastic modulus using load and displacement sensing indentation experiments. J. Mater. Res. 7, 1564–1583. doi: 10.1557/JMR.1992.1564
Peirano, A., Abbate, M., Cerrati, G., Difesca, V., Peroni, C., and Rodolfo-Metalpa, R. (2005). Monthly variations in calix growth, polyptissue, and density banding of the Mediterranean scleractinian Cladocora caespitosa (L.). Coral Reefs 24, 404–409. doi: 10.1007/s00338-005-0020-6
Pereira, S. G., Lima, F. P., Queiroz, N. C., Ribeiro, P. A., and Santos, A. M. (2006). Biogeographic patterns of intertidal macroinvertebrates and their association with macroalgae distribution along the Portuguese coast. Hydrobiologia 555, 185–192. doi: 10.1007/s10750-005-1115-3
Poore, G. C., and Bruce, N. L. (2012). Global diversity of marine isopods (except Asellota and crustacean symbionts). PLoS One 7:e43529. doi: 10.1371/journal.pone.0043529
Pörtner, H. O., Karl, D. M., Boyd, P. W., Cheung, W., et al. (2014). “Ocean systems,” in Climate Change 2014: Impacts, Adaptation, and Vulnerability. Part A: Global and Sectoral Aspects. Contribution of Working Group II to the Fifth Assessment Report of the Intergovernmental Panel on Climate Change, eds C. Field, V. Barros, D. Dokken, K. Mach, M. Mastrandrea, T. Bilir, et al. (Cambridge, MA: Cambridge University Press).
Porzio, L., Buia, M. C., and Hall-Spencer, J. M. (2011). Effects of ocean acidification on macroalgal communities. J. Exp. Mar. Biol. Ecol. 400, 278–287. doi: 10.1016/j.jembe.2011.02.011
Ragazzola, F., Foster, L. C., Form, A. U., Büscher, J., Hansteen, T. H., and Fietzke, J. (2013). Phenotypic plasticity of coralline algae in a high CO 2 world. Ecol. Evol. 3, 3436–3446. doi: 10.1002/ece3.723
Ragazzola, F., Foster, L. C., Jones, C. J., Scott, T. B., Fietzke, J., Kilburn, M. R., et al. (2016). Impact of high CO2 on the geochemistry of the coralline algae Lithothamnion glaciale. Sci. Rep. 6:20572. doi: 10.1038/srep20572
Rhein, M., Rintoul, S. R., Aoki, S., Campos, E., Chambers, D., Feely, R. A., et al. (2013). “Observations: ocean,” in Climate Change 2013: The Physical Science Basis. Contribution of Working Group I to the Fifth Assessment Report of the Intergovernmental Panel on Climate Change, eds T. F. Stocker, D. Qin, G.-K. Plattner, M. Tignor, S. K. Allen, J. Boschung, et al. (Cambridge, MA: Cambridge University Press), 255–316.
Schoener, T. W. (1974). Resource partitioning in ecological communities. Science 185, 27–39. doi: 10.1126/science.185.4145.27
Scipione, M. B., Kroeker, K. J., Ricevuto, E., and Gambi, M. C. (2017). Amphipod assemblages along shallow water natural pH gradients: data from artificial substrata (Island of Ischia. Italy). Biodivers. J. 8, 469–470.
Semesi, I. S., Beer, S., and Björk, M. (2009). Seagrass photosynthesis controls rates of calcification and photosynthesis of calcareous macroalgae in a tropical seagrass meadow. Mar. Ecol. Prog. Ser. 382, 41–47. doi: 10.3354/meps07973
Smale, D. A., Wernberg, T., Peck, L. S., and Barnes, D. K. A. (2011). Turning on the heat: ecological response to simulated warming in the sea. PLoS One 6:e16050. doi: 10.1371/journal.pone.0016050
Sparla, M. P., Scipione, M. B., and Riggio, S. (1993). Peracarid Crustacea inhabiting aegagropylae of the red algae Rytiphloa tinctoria (Clemente) C. Ag. in the Stagnone Sound, western Sicily, Italy. Crustaceana 64, 1–17. doi: 10.1163/156854093X00018
Steneck, R. S. (1986). The ecology of coralline algal crusts: convergent patterns and adaptive strategies. Annu. Rev. Ecol. Syst. 17, 273–303. doi: 10.1146/annurev.es.17.110186.001421
Stewart, J. G. (1982). Anchor species and epiphytes in intertidal algal turf. Pacific Sci. 36, 45–59.
Sunday, J. M., Fabricius, K. E., Kroeker, K. J., Anderson, K. M., Brown, N. E., Barry, J. P., et al. (2017). Ocean acidification can mediate biodiversity shifts by changing biogenic habitat. Nat. Clim. Chang. 7, 81–85. doi: 10.1038/nclimate3161
Turner, L. M., Ricevuto, E., Gallucci, A. M., Lorenti, M., Gambi, M. C., and Calosi, P. (2016). Metabolic responses to high pCO2 conditions at a CO2 vent site in juveniles of a marine isopod species assemblage. Mar. Biol. 163:211. doi: 10.1007/s00227-016-2984-x
Underwood, A. J. (1997). Experiments in Ecology. Their Logical Design and Interpretation Using Analysis of Variance. Cambridge, MA: Cambridge University Press.
Whorff, J. S., Whorff, L. L., and Sweet, M. H. (1995). Spatial variation in an algal turf community with respect to substratum slope and wave height. J. Mar. Biol. Assoc. 75, 429–444. doi: 10.1017/S0025315400018282
Wood, H. L., Sköld, H. N., and Eriksson, S. P. (2014). Health and population-dependent effects of ocean acidification on the marine isopod Idotea balthica. Mar. Biol. 161, 2423–2431. doi: 10.1007/s00227-014-2518-3
Zakhama-Sraieb, R., Sghaier, Y. R., Guesmi, S., and Charfi-Cheikhrouha, F. (2011). Peracarid crustaceans associated with the red alga Corallina elongata in Rafraf, Tunisia (Mediterranean Sea). Crustaceana 84, 69–80. doi: 10.1163/001121610X538868
Zuur, A., Ieno, E. N., and Smith, G. M. (2007). Analyzing Ecological Data. New York, NY: Springer. doi: 10.1007/978-0-387-45972-1
Zuur, A., Ieno, E. N., Walker, N., Saveliev, A. A., and Smith, G. M. (2009). Mixed Effects Models and Extensions in Ecology With R. New York, NY: Springer.
Keywords: climate change, intertidal ecosystem, coralline algae, bioconstruction, biodiversity, peracarid crustaceans, polychaetes
Citation: Marchini A, Ragazzola F, Vasapollo C, Castelli A, Cerrati G, Gazzola F, Jiang C, Langeneck J, Manauzzi MC, Musco L, Nannini M, Zekonyte J and Lombardi C (2019) Intertidal Mediterranean Coralline Algae Habitat Is Expecting a Shift Toward a Reduced Growth and a Simplified Associated Fauna Under Climate Change. Front. Mar. Sci. 6:106. doi: 10.3389/fmars.2019.00106
Received: 29 October 2018; Accepted: 21 February 2019;
Published: 13 March 2019.
Edited by:
Julio Aguirre, University of Granada, SpainReviewed by:
Tamar Guy-Haim, Israel Oceanographic and Limnological Research, IsraelJason Michael Hall-Spencer, University of Plymouth, United Kingdom
Sophie Martin, Station Biologique de Roscoff, France
Copyright © 2019 Marchini, Ragazzola, Vasapollo, Castelli, Cerrati, Gazzola, Jiang, Langeneck, Manauzzi, Musco, Nannini, Zekonyte and Lombardi. This is an open-access article distributed under the terms of the Creative Commons Attribution License (CC BY). The use, distribution or reproduction in other forums is permitted, provided the original author(s) and the copyright owner(s) are credited and that the original publication in this journal is cited, in accordance with accepted academic practice. No use, distribution or reproduction is permitted which does not comply with these terms.
*Correspondence: Federica Ragazzola, federica.ragazzola@port.ac.uk
†These authors have contributed equally to this work