- 1Hawai‘i Coral Reef Initiative, Social Science Research Institute, University of Hawai‘i, Honolulu, HI, United States
- 2Center for Ocean Solutions, Stanford University, Stanford, CA, United States
- 3School of Geography and the Environment, University of Oxford, Oxford, United Kingdom
- 4The Nature Conservancy, Indo-Pacific Resource Centre, South Brisbane, QLD, Australia
- 5Pristine Seas, National Geographic Society, Washington, DC, United States
- 6Department of Biology, University of Hawai‘i, Honolulu, HI, United States
- 7National Center for Ecological Analysis and Synthesis, University of California, Santa Barbara, Santa Barbara, CA, United States
Coral reef managers currently face the challenge of mitigating global stressors by enhancing local ecological resilience in a changing climate. Effective herbivore management is one tool that managers can use in order to maintain resilience in the midst of severe and frequent bleaching events. One recommended approach is to establish networks of herbivore management areas (HMAs), which prohibit the take of herbivorous reef fishes. However, there is a need to develop design principles to guide planning and implementation of these HMAs as a resilience-building tool. We refine available guidance from fully protected marine protected area (MPA) networks and developed a set of 11 biophysical design principles specifically for HMAs. We then provide a case study of how to apply these principles using the main Hawaiian Islands. We address site-specific considerations in terms of protecting habitats, including ecologically critical areas, incorporating connectivity, and addressing climate and local threats. This synthesis integrates core marine spatial planning concepts with resilience-based management and provides actionable guidance on the design of HMAs. When combined with social considerations, these principles will support spatial planning in Hawai‘i and could guide the future design of HMA networks globally.
Introduction
Coral reefs are among the most diverse and complex ecosystems in the world and provide ecosystem services to millions of coastal residents in nearly 100 nations (Moberg and Folke, 1999), yet are the marine ecosystem most vulnerable to climate change (Hughes et al., 2017b). Climate change is adversely affecting coral reefs in multiple ways including sea level rise, acidification, and ocean warming, which can act in concert with local stressors. For example, climate-induced coral bleaching events together with local stressors (e.g., overfishing, land-based pollution, and coastal development) can result in potential for regime shifts from coral-dominated to macroalgae-dominated systems, leading to a loss of biodiversity as well as ecosystem goods and services (Ateweberhan et al., 2013; Graham et al., 2013; Hoegh-Guldberg et al., 2017).
Enhancing the ecological resilience of coral reefs has become a central focus for managers worldwide as the frequency and severity of climate-induced impacts increases (Baker et al., 2008; Van Hooidonk et al., 2016; Hughes et al., 2017a). Improving coral reef resilience relies on fostering two central components: the ability of coral reefs to both resist and recover from ecological disturbances (Holling, 1973). To achieve increased resilience through conservation planning, managers must reduce local stressors while fostering key resilience processes throughout their jurisdiction, known as resilience-based management (Graham et al., 2013; Anthony et al., 2015).
Herbivory is a critical ecological process that underpins the ability of corals to recover from disturbances (Bellwood et al., 2004) and resist regime shifts to algal-dominated reef states (Graham et al., 2013). Herbivores prevent algal overgrowth (e.g., thick turfs and macroalgae) that can inhibit coral settlement, survival, thereby reducing reef structural complexity (Hixon, 2015). Increasing herbivore biomass has also been demonstrated as a critical tool for maintaining ecosystem function and resilience leading to increases in fish grazing, crustose coralline algae (CCA), coral recruitment and cover (Mumby, 2006; Hughes et al., 2007; Smith et al., 2010).
Herbivore management areas (HMAs) are spatially managed areas where the take of herbivorous fishes and invertebrates (e.g., sea urchins) is prohibited while other extractive and non-extractive uses are allowed. Increasing herbivorous fish biomass, particularly through the use of spatial networks have been dominant recommendations of resilience-based management (McLeod et al., 2009; Graham et al., 2013; Green et al., 2014; Chung et al., 2019). Herbivores are good candidates for spatial strategies because of their high site fidelity (Howard et al., 2013) and in previous usage spatial management and herbivory showed a strong connection to shaping benthic communities (Graham et al., 2011). Herbivory maintains space for coral recruit settlement, a key process for coral recovery after disturbances and bleaching events (Hughes, 1994; McClanahan, 2000; Mumby et al., 2007). Thus, HMAs have several qualities that suggest they would be an effective tool to prevent ecosystem shifts and increase the resilience of coral reef ecosystems (McClanahan et al., 2012; Graham et al., 2013; Mumby et al., 2014; Bozec et al., 2016).
Consecutive and unprecedented mass coral bleaching events in 2014 and 2015 in the main Hawaiian Islands ignited a new conversation about how local managers can mitigate the effects of climate change. Exposure and severity of temperature stress during these two consecutive events was variable across the state, with some coastlines far exceeding levels previously observed (Figure 1). For example in 2015, areas along the west coast of Hawai‘i island (known as west Hawai‘i) reached 16 degree heating weeks (DHW), which is double the level of accumulated temperature stress expected to trigger widespread bleaching and significant coral mortality (NOAA Coral Reef Watch, 2015). Following these bleaching events, the average coral loss along west Hawai‘i was 50% (Kramer et al., 2016). Substantial mortality was also reported around the islands of Maui and O‘ahu. Subsequently, concerns about the resilience of coral reefs in Hawai‘i spurred local resource managers to consider long-term intervention measures.
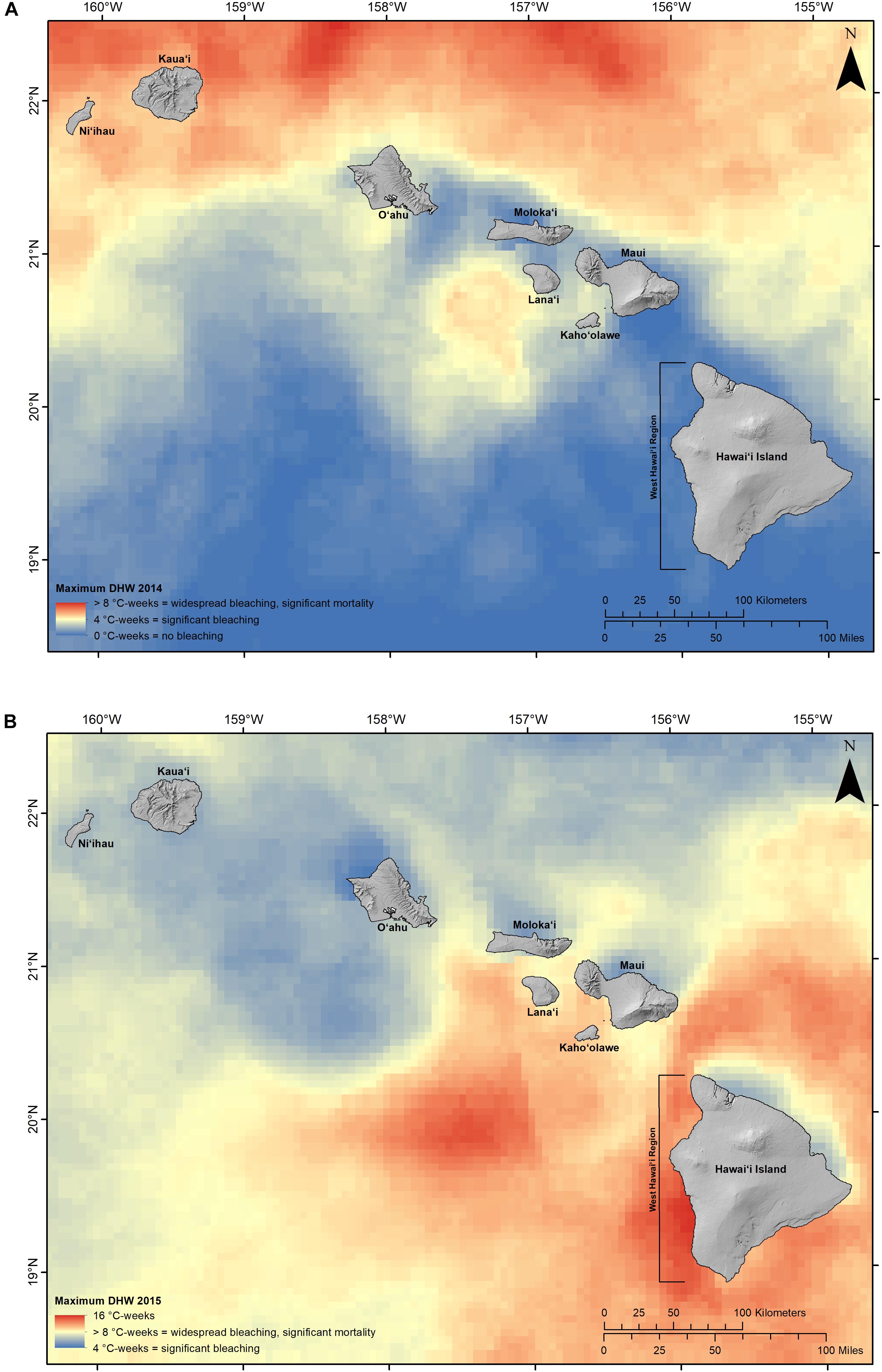
Figure 1. The maximum degree heating week (DHW) observed in 2014 (A) and 2015 (B) across the main Hawaiian Islands (climate data source: NOAA coral reef watch).
In Hawai‘i, the department of land and natural resources (DLNR) division of aquatic resources (DAR) is responsible for “managing, conserving, and restoring the state’s aquatic resources and ecosystems for present and future generations” (Department of Land and Natural Resources [DLNR], 2018). In 2016, DAR initiated the development of The Hawai‘i Coral Bleaching Recovery Plan, which evaluated alternative management options following the major bleaching events through a combination of a systematic literature review and global and local expert opinion. Establishing a network of HMAs was identified as the top-ranked intervention based on its potential to promote coral recovery and management effectiveness (Chung et al., 2019).
Currently, there are 84 existing marine protected areas (MPAs) across the main Hawaiian Islands. However, only a few of these areas provide full protection for herbivorous fishes, most provide only partial or no herbivore protection, and only one (the Kahekili HMA on Maui) was specifically designed to manage herbivores (Friedlander et al., in press) (Figure 2). Furthermore, the existing MPAs were not designed as an ecologically connected network, but rather were built piecemeal and is reflective of various needs to manage user conflict, safeguard protected species, or on the wishes of local communities (Friedlander et al., 2014).
However, the success of some individual MPAs (Friedlander et al., 2007a,b; Williams et al., 2016) indicates that a network of HMAs could be a useful tool for managing herbivores and coral reef resilience in Hawai‘i. For example, the existing Marine Life Conservation Districts, where fishing and consumptive uses are limited (and in many completely prohibited), generally have higher herbivore biomass, larger overall fish size, and higher biodiversity than adjacent areas of similar habitat quality (Friedlander et al., 2007a,b). Furthermore, in the first 6 years of herbivore management at the Kahekili HMA, the single example of an existing HMA in the main Hawaiian Islands, mean parrotfish and surgeonfish biomass increased by 139 and 28%, respectively, macroalgal cover remained low, CCA (a settlement habitat for coral larvae) increased from 2 to 15%, and coral cover stabilized from a declining trend (Williams et al., 2016).
Despite global and Hawai‘i-specific recommendations to use HMAs as a tool for increasing coral reef resilience, there is currently a lack of practical guidance on how to apply this theoretical approach. In this study, we identify biophysical principles for designing a network of HMAs as a climate adaptation tool. Where design principles are defined as guidelines that provide specific advice on how to design a MPA network to achieve its objectives (Green et al., 2013). To demonstrate how to apply this concept, we use Hawai‘i as a case study to explore unique place-based factors that could guide site-specific implementation. This process will guide the future configuration and placement of HMAs to help build ecological resilience in Hawai‘i, and could inform similar processes worldwide.
Materials and Methods
We developed biophysical criteria for designing networks of HMAs using the following process often applied to design MPA networks in other tropical marine ecosystems worldwide (e.g., see Fernandes et al., 2005; Green et al., 2009; Wilson et al., 2011).
First, we defined clear and measurable objectives, which are necessary to effectively design, monitor and evaluate MPAs (Botsford et al., 2003; IUCN World Commission on Protected Areas, 2008). We defined our objectives based on the scientific literature regarding what HMA networks could achieve ecologically, and the objectives of The Hawai‘i Coral Bleaching Recovery Plan. Our objectives for designing a network of HMAs were to maintain and increase coral reef resilience by maintaining and/or increasing herbivore biomass, abundance, and functional diversity.
The next step was to identify design criteria, which provided specific advice on how to design the HMA network to achieve its objectives. Typically, MPA networks are designed using two types of design principles: biophysical principles aimed at achieving ecological objectives by taking key biological and physical processes into account; and socioeconomic, cultural and governance (SECG) principles aimed at maximizing benefits and minimizing costs to local communities and industries and aligning with legal, political and institutional requirements (e.g., see Fernandes et al., 2005; Green et al., 2009; Wilson et al., 2011).
As a starting point, we adapted biophysical principles for fully protected, or no-take, MPA networks that aim to rebuild fish stocks, conserve biodiversity, and mitigate climate impacts designing networks of HMAs. These biophysical principles were intended to contribute to a larger design process that includes incorporating local knowledge, complementing human uses and values, and adhering to legal and political requirements. Thus implementation will also require the development of SECG design principles, which are currently being developed through a similar process for Hawai’i. Once completed, the biophysical and SECG design principles can be used in tandem to design the HMA network to meet multiple ecological and social objectives (Klein et al., 2008).
We developed biophysical design principles for HMAs by adapting and refining existing principles for designing networks of no-take marine MPAs to rebuild fish stocks, conserve biodiversity, and mitigate climate impacts (McLeod et al., 2009; Green et al., 2014, 2015). Fully protected MPAs are powerful tools for enhancing fish stocks within their boundaries and providing fisheries benefits outside their boundaries (Gaines et al., 2010; Baskett and Barnett, 2015; Lubchenco and Grorud-Colvert, 2015). We then adapted and refined the design guidelines to provide specific criteria for designing networks of HMAs as a climate change adaptation tool. Design principles are currently being developed through a similar process and, once completed, can be used in tandem with the biophysical principles to design HMA networks. Finally, we reviewed the best available information regarding the biophysical environment in Hawai’i to identify how these design criteria could to be used to design a network of HMAs in the main Hawaiian Islands.
Results
We developed 11 biophysical principles across five categories to design a network of HMAs to maintain and increase coral reef resilience by maintaining and/or increasing herbivore biomass, abundance, and functional diversity (Table 1). We also provide specific considerations for applying these principles to design a network of HMAs in the main Hawaiian Islands based on local conditions.
Habitats
Different herbivore species use different habitats. Thus, protection of all species and maintenance of the health, integrity and resilience of the ecosystem can only be achieved if adequate examples of each major is protected in HMAs (adapted from Green et al., 2014). Where the percentage of each habitat to be protected will depend on several factors including habitat condition, fishing pressure and if there is additional effective protection (fisheries management) outside of HMAs (reviewed in Green et al., 2014). Thus to maximize benefits to fisheries management and biodiversity in the face of climate change, HMAs should encompass at least 20–40% of each type of herbivore habitat (e.g., see Airamé et al., 2003; Fernandes et al., 2005; McLeod et al., 2009; Green et al., 2014), depending on the local situation (Green et al., 2014).
In Hawai‘i, it is important to represent habitat heterogeneity that occurs at multiple scales from individual reefs to the entire MHI within the network. Spatial pattern metrics that describe seascape structure and habitat complexity have been used to describe relationships between habitat structure and reef fish assemblages (Wedding and Friedlander, 2008; Wedding et al., 2008, 2011), with more complex seascape structure associated with greater abundance, species richness, and biomass of reef fishes. These areas with high habitat heterogeneity should be a priority to include in HMAs.
Throughout the MHIs, parrotfish distributions are significantly correlated with areas of high rugosity, coral cover, non-turf macroalgae, and CCA (Howard et al., 2009) and in particular shallow (5–10 m) spur and groove habitat (Ong and Holland, 2010). Zebrasoma flavescens, a common surgeonfish, is known to forage in shallow complex reef flat and boulder habitats (Claisse et al., 2011). Herbivore density is also highest in shallow, backreef habitat (Friedlander and Parrish, 1998a), whereas biomass shows a negative relationship with macroalgal cover (Friedlander et al., 2007b). Thus, areas that have features and a high diversity of habitats used by a range of herbivorous fishes and functional groups should be prioritized for inclusion in HMAs (modified from Fernandes et al., 2005; Green et al., 2009, 2014).
Protection of each type of habitat in at least three widely separated HMAs will also minimize the risk that all examples will be adversity impacted by the same disturbance (e.g., mass coral bleaching or hurricanes: see Green et al., 2014). Thus, if some protected habitats survive the disturbance, they can act as a source of larvae to facilitate recovery in other areas.
Critical Areas
Areas that are critical to the life history of herbivores should be included in the HMA network. These include concentrated feeding and spawning grounds or areas with ecosystem processes on which herbivores depend (Green et al., 2014). Herbivores have been classified into groups based on their functional role as grazers, scrapers, or browsers, so a combination of their unique ecological roles will be critical to build resilience (Choat et al., 2004; Hixon, 2015).
In Hawai‘i, the distribution of herbivorous fishes varies by habitat regime, which is driven by ecological and biophysical characteristics (Donovan et al., 2018). In addition, current herbivore biomass naturally varies considerably across the archipelago driven by differences in benthic habitat cover, physical characteristics, and oceanography (Gorospe et al., 2018). HMAs should be prioritized for inclusion in the HMA network that have a naturally high diversity of functional groups and a high herbivore biomass to maximize benefit for coral reef resilience.
While many species of herbivores are considered overfished in Hawai‘i, reductions in fishing pressure could result in high gains in some areas and should be prioritized within the network. There is strong evidence of overfishing in the MHI when compared with the neighboring, unpopulated northwestern Hawaiian Islands (Friedlander and DeMartini, 2002) as well as historical levels on the main Hawaiian Islands (Friedlander and DeMartini, 2002; Williams et al., 2011; Howard et al., 2013; Heenan et al., 2016). In a recent stock assessments, surgeonfishes and parrotfishes had the highest number (83 and 50%, respectively) of species with low spawning potential ratio (SPR) values, which defines overexploitation (Nadon, 2017). However, there is also substantial spatial variation in the predicted ability of nearshore areas to recover from fishing pressure (Gorospe et al., 2018; Stamoulis et al., 2018). These hotspots for fisheries recovery should be prioritized for inclusion in the network of HMAs.
It is also important to ensure that herbivores will be protected at all life-history phases within the HMA network. In particular, herbivores are concentrated in areas that are important for their various ecological needs (e.g., nursery, sheltering, feeding, and spawning areas), and protecting these critical habitats can yield significant benefits for conserving herbivore functional groups (Green et al., 2014; Weeks et al., 2017). Thus, critical areas for herbivores during spawning and nursery stages, as well as feeding and sheltering, should also be prioritized for protection within a network of HMAs. For example, spawning aggregations of Acanthurus nigrorus have been observed in large boulder habitat 7–10 m deep near a steep (25–30 m) dropoff (Schemmel and Friedlander, 2017), and larval surgeonfishes (e.g., Acanthurus triostegus) are known to leave the pelagic stage and enter very shallow water in Hawai’i, often in tide pools where they grow to juvenile size in these shallow-water refugia (Randall, 1961).
Connectivity
To accommodate larval connectivity in the sizing and spacing of herbivore management areas, barriers to gene flow across the MHI must be considered. Compared with most Indo-Pacific coral reef ecosystems, Hawai‘i’s reefs, are relatively isolated and likely mostly self-seeding (Halford and Caley, 2009). Multispecies dispersal barriers have been documented within the MHI between island groups corresponding to major ocean channels (Toonen et al., 2011; Wren et al., 2016). Within islands, studies of existing MPAs in Hawai‘i indicate the potential for management areas to support not only protected reef areas but also benefit neighboring unprotected reefs through enhanced reproductive output and adult spillover (Christie et al., 2010; Stamoulis and Friedlander, 2013). For example, Christie et al. (2010) found that the distance of Zebrasoma flavescens larval dispersal ranged between 15 and 184 km along the coast of West Hawai‘i. Lastly, coral reef community structure in Hawai‘i is primarily driven by wave exposure (Dollar, 1982) with sheltered areas maintaining larger fish populations (Friedlander and Parrish, 1998b). These characteristics emphasize the need for stratification between and within islands to achieve evenness in larval dispersal across the network. Therefore, we suggest that HMAs should be replicated within major shores (e.g., north, east, south, and west) on each major island, and spaced appropriately to the geography and biophysical attributes of the nearshore region (e.g., prevailing currents, wave forcing) to ensure connectivity among HMAs and fished areas.
In addition to larval connectivity, adult movements should be considered throughout the network. However spatial use patterns are variable as some herbivores in Hawai‘i are site-associated most of the time, while others make periodic forays for specific activities. For example, Zebrasoma flavescens occupy shallow depths (3–6 m deep) during the day (Williams et al., 2009) then make considerable crepuscular migrations to deeper waters up to 600 m away from foraging to spawning and sheltering sites (Claisse et al., 2011). Similarly, parrotfishes, especially large individuals, also make forays at crepuscular hours and rely heavily on the availability of nocturnal holes for shelter (Meyer et al., 2010; Howard et al., 2013). These intermittent movements should be captured in the size of HMAs, extending to the full depth range (1–30 m) and ensuring multiple areas per coastline.
Movements of adult and juvenile herbivorous fishes, which range from resident to long-ranging species, should also be considered when considering the size of individual HMAs (Table 2). Multiple species of small-bodied surgeonfishes and parrotfishes are resident in a small (0.14 km2) marine reserve in Kâne’ohe Bay, O‘ahu (Meyer and Holland, 2005; Bierwagen et al., 2017; Stamoulis et al., 2017). The bluespine unicornfish (Naso unicornis), a medium-sized herbivorous fish, demonstrated daily movement patterns in Hawai‘i less than 1 km (Meyer and Holland, 2005). Large-bodied adult herbivorous fishes often have larger home ranges (Holland et al., 1993) and seek refuge commensurate with their body size (Friedlander and Parrish, 1998a). For instance (Howard et al., 2013) found persistent mean adult fish home range sizes for large-bodied parrotfish to range between 834 and 2,279 m2 depending on depth. Chubs (Kyphosus spp.) are unique in that they have much larger home ranges than many other reef fishes (Eristhee, 2001; Pillans et al., 2017), with rare individuals observed to make trans-island movements over 300 km in Hawaiian waters (Sakihara et al., 2015). Ultimately, reef physiography will be a critical consideration in MPA network design in Hawai‘i as it has been observed to play an important role in home range movement (Holland et al., 1996).
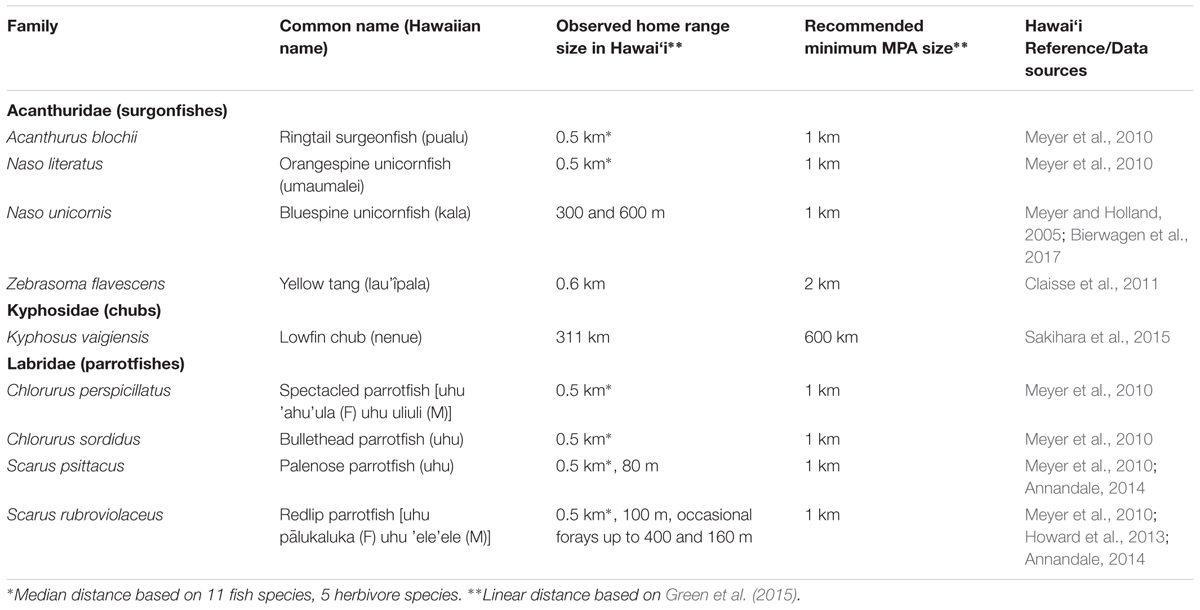
Table 2. Recommended minimum MPA size for herbivorous fishes in Hawai‘i, based on Green et al. (2015) and Weeks et al. (2017).
In addition, ontogenetic patterns of habitat use by herbivores should be considered in network design. Depth has a strong correlation to fish assemblages in the main Hawaiian Islands (Friedlander et al., 2007b) and herbivore biomass has been observed to be highest at the relatively shallow depth range of 4.3–7.2 m (Friedlander and Parrish, 1998a). Furthermore, Kane and Tissot (2017) reports that herbivorous fishes in west Hawai‘i are not abundant below 30 m, suggesting priority should be given to nearshore waters 1–30 m deep. However, in various life stages, herbivorous fish have been observed to move between shallow to deep reef habitats (Ortiz and Tissot, 2008; Ortiz and Tissot, 2012), highlighting the need for adjacent areas of deep aggregate coral habitat and shallow nearshore habitat (such as rubble and turf-rich boulders) to be included within HMAs.
Green et al. (2015) recommend that fully protected MPAs should cover at least twice the length of coastline that focal species adults and juveniles require. To accommodate the full range of movements of herbivorous fishes in Hawai‘i, each HMA should be sized to accommodate large-bodied parrotfish movements, covering no less than 2 km of the coastline. Large distances traveled by chub species can be accommodated through placement of multiple HMAs per coastline.
Climate Considerations
Given changing climatic conditions, it will be important to protect ecological communities that are more likely to be resilient to changes in climate and ocean change, as well as those that may be particularly vulnerable and need more protection. The network should therefore encompass a combinations of reefs that have either (a) areas that have withstood bleaching in that past or are thought to more likely to withstand bleaching in the future (i.e., climate refugia) (McClanahan et al., 2007; McLeod et al., 2012; Magris et al., 2015), (b) areas currently at high risk of regime risks (e.g., areas with recent high exposure to elevated temperatures) (Graham et al., 2015; Magris et al., 2015), and (c) a distribution of areas that spreads the risk to address uncertainty regarding how conditions may change (Salm et al., 2006; Green et al., 2009, 2014).
In Hawai‘i, there is a lack of long-term information on the effect of bleaching events as the 2014 and 2015 events were unprecedented in their extreme and widespread effects. Because of this, refugia should be based on biophysical drivers that were observed to correlate with areas either resisting or quickly recovering from the bleaching events. Based on mortality and recovery data synthesized through the Hawai‘i Coral Bleaching Collaborative, areas with high weekly temperature variability were found to better resist and immediately recover from high temperature stress. Thus, the HMA network should include the upper quantile of values (top 25%) of these areas to capture potential climate refugia.
To maximize potential reef recovery from past bleaching, HMAs should prioritize areas with highest exposure to accumulated temperature stress following the consecutive bleaching events must be addressed. To maximize recovery potential of coral communities in Hawai‘i, the network should prioritized to include areas of the state in Maui, Moloka‘i, Lana‘i, and west Hawai‘i that experienced the highest bleaching stress (NOAA Coral Reef Watch, 2015). The network should include a portion of nearshore areas within these islands that reached >8 DHW during the event, which is the level at which widespread bleaching and substantial mortality is expected to occur. This is important to help build the resilience of coral communities that have had the highest exposure to coral bleaching impacts to date and are in the most need for additional management.
Future climate risk should also be mitigated by spreading HMAs areas both across and within island units (Salm et al., 2006; Green et al., 2009, 2014: see Principle 2). Differences in exposure between the 2014 and 2015 bleaching events suggest future exposure will also be variable across the entire archipelago. Modeling suggests annual severe bleaching starting between 2030 and 2040 in the MHI, with variable effects across islands (Van Hooidonk et al., 2016). To spread the climate risk, the design should include multiple HMAs. Stratifying and replicating HMAs within the network will support the natural process of adaptation to climate change and lessen the possibility of major ecological impacts to the entire network from individual disturbances.
Local Threats
Nutrient input has been shown to increase algal biomass, trigger invasive blooms, and result in reef decline in Hawaiian waters (Smith et al., 1981), particularly when combined with decreased herbivory (Smith et al., 2001). Areas with high sedimentation can suppress herbivory on coral reefs (Bellwood and Fulton, 2008) and increased sediment loads may result in more persistent algal coverage (Goatley and Bellwood, 2013; Goatley et al., 2016). In Hawai‘i, sources of land-based pollution of particular concern include sedimentation from erosion (both natural and human-induced), nutrient flux from on-site sewage disposal systems, agriculture and golf-course runoff, and urban runoff from impervious surfaces (Lecky, 2016; Wedding et al., 2018). Effects of land-based pollution on coral health vary spatially with leeward, sheltered reefs having a stronger relationship to watershed health compared to windward, exposed coastlines with enhanced mixing (Rodgers et al., 2012). Therefore, where possible, it will be important to avoid placing HMAs in areas strongly affected by land-based pollution. In addition, the network should be implemented to complement land-based management strategies to support reef resilience. In Hawai‘i, the Division of Forestry and Wildlife has identified priority watershed areas covering forests on each island (Sustainable Hawai‘i Initiative, 2018). HMAs should be paired with these watershed restoration areas to align priorities between management bodies.
Discussion
Here we provide, for the first time, biophysical principles to guide the design of HMAs to maintain and increase coral reef resilience. We also provide a case study of how to apply these principles in the unique biophysical environment of Hawai’i. The next step in planning for a network of HMAs across the main MHI is to incorporate socioeconomic, cultural, and governance (SECG) considerations to balance ecological and human aspects of the design. This more holistic set of guidance can then be used to conduct a systematic spatial planning process where data related to each principle can be mapped and analyzed simultaneously using marine reserve design software such as Marxan.
The social context is particularly crucial to success of HMAs in Hawai‘i, where herbivorous fishes are a valued nearshore food resource, critical to both commercial and non-commercial fisheries and where patterns of use are geographically variable (Friedlander and Parrish, 1998a; Williams and Ma, 2013; Grafeld et al., 2017; McCoy et al., 2018). This provides an opportunity to use spatial estimates of herbivorous fish catch to balance human use with conservation and fisheries management goals.
Community co-managed areas are relevant in the Hawai‘i context and could contribute to the success of newly implemented herbivore management areas. In 2016, the first community-based subsistence fisheries area (CBSFA) was established at Hā‘ena, Kauai. Several other communities are currently pursuing CBSFA designation, some with herbivore protections included, and many others are participating in grassroots, community-based stewardship. This community interest could be leveraged to appropriately place HMAs along coastlines where they would be welcomed and supported, rather than those where they may be misaligned with the community’s interests (Friedlander et al., 2013, 2014; Ayers and Kittinger, 2014). We recommend pursuing both an ecologically and socially connected network of HMAs appropriately designed in areas of high community involvement and support.
Design of a cohesive network of MPAs is currently underway as part of the state’s Marine 30 × 30 – an effort to achieve “30% effective management in Hawai‘i’s nearshore marine waters by 2030” (Sustainable Hawai‘i Initiative, 2018). One objective of this initiative is to increase reef resilience through improved spatial management. The biophysical design principles that we provide could be applied to prioritize specific nearshore areas to protect herbivorous fishes, promote recovery from coral bleaching, and build ecological resilience. Final placement and design of HMAs in Hawai‘i could be woven into this process through collaborative planning with stakeholders and the public rulemaking process.
Coral reefs worldwide are experiencing the negative effects of global climate change. Predictions of more frequent and severe bleaching events in Hawai‘i and globally (Van Hooidonk et al., 2016) are a catalyst for local managers to implement resilience concepts within their jurisdictions and improve long-term ecological resilience. The fate of coral reefs depends on our ability to develop appropriate management solutions. Maintaining herbivory has been recognized as a key strategy in both prevention of and recovery from coral reef phase shifts (Bellwood et al., 2006; Jouffray et al., 2015; McLeod et al., 2019). Developing biophysical design principles for networks of HMAs are a step in the translation of a highly recommended resilience-based management practice, which includes strengthening protection of herbivores (Graham et al., 2013; Anthony et al., 2015). Refining existing management practices and tools, including how to design and plan networks of MPAs, is one way that managers can begin implementing resilience-based management at a local scale.
Author Contributions
AC, LW, and GG conceived the original research concept and led initial writing of the manuscript. AC refined the manuscript and developed the table of design principles and the herbivorous fish movement table. AG provided guidance on the methods to develop the design principles and the fish movement table, and made significant refinements to several drafts of the manuscript. AF and MH provided input to early concepts of the manuscript and contributed detailed edits of preliminary drafts. AM supported the writing of preliminary drafts, created the map of existing herbivore management areas, and assisted with editing later manuscript drafts.
Conflict of Interest Statement
The authors declare that the research was conducted in the absence of any commercial or financial relationships that could be construed as a potential conflict of interest.
Acknowledgments
The authors would like to thank the Hawai‘i Office of Planning Coastal Zone Management Program and the National Oceanic and Atmospheric Administration (Award No. NA14NOS4190079) as well as the Pacific Islands Climate Change Cooperative, with funding from the US Fish and Wildlife Service for supporting this work. Thanks to Mary Donovan, Jamie Gove, and Tom Oliver for their work on data related to this publication and for their contributions to early renditions of the design principles. A sincere mahalo to the staff of the Hawai‘i Coral Reef Initiative for providing critical administrative and program management assistance.
References
Airamé, S., Dugan, J., Lafferty, K., Leslie, H., McArdle, D., and Warner, R. (2003). Applying ecological criteria to marine reserve design: a caste study from the California channel Islands. Ecol. Appl. 13, 170–184. doi: 10.1890/1051-0761(2003)013[0170:AECTMR]2.0.CO;2
Annandale, S. F. (2014). Diel Movement Patterns of Scarus Rubroviolaceus and Scarus Psittacus Examined Using Acoustic Telemetry in Puako. Ph.D. thesis, Doctoral dissertation, University of Hawai‘i at Hilo, HI: Hilo.
Anthony, K., Marshall, P., Abdulla, A., Beeden, R., Bergh, C., Black, R., et al. (2015). Operationalizing resilience for adaptive coral reef management under global environmental change. Glob. Change Biol. 21, 48–61. doi: 10.1111/gcb.12700
Ateweberhan, M., Feary, D., Keshavmurthy, S., Chen, A., Schleyer, M., and Sheppard, C. (2013). Climate change impacts on coral reefs: synergies with local effects, possibilities for acclimation, and management implications. Mar. Poll. Bull. 74, 526–539. doi: 10.1016/j.marpolbul.2013.06.011
Ayers, A., and Kittinger, J. (2014). Emergence of co-management governance for Hawai‘i coral reef fisheries. Glob. Environ. Change 28, 251–262. doi: 10.1016/j.gloenvcha.2014.07.006
Baker, A., Glynn, P., and Riegl, B. (2008). Climate change and coral reef bleaching: an ecological assessment of long-term impacts, recovery trends and future outlook. Estuar. Coast. Shelf Sci. 80, 435–471. doi: 10.1016/j.ecss.2008.09.003
Baskett, M., and Barnett, L. (2015). The ecological and evolutionary consequences of marine reserves. Annu. Rev. Ecol. Evol. Syst. 46, 49–73. doi: 10.1146/annurev-ecolsys-112414-054424
Bellwood, D., and Fulton, C. (2008). Sediment-mediated suppression of herbivory on coral reefs: decreasing resilience to rising sea-levels and climate change? Limnol. Oceanogr. 53, 2695–2701. doi: 10.4319/lo.2008.53.6.2695
Bellwood, D., Hughes, T., Folke, C., and Nyström, M. (2004). Confronting the coral reef crisis. Nature 429, 827–833. doi: 10.1038/nature02691
Bellwood, D., Hughes, T., and Hoey, A. (2006). Sleeping functional group drives coral reef recovery. Curr. Biol. 16, 2434–2439. doi: 10.1016/j.cub.2006.10.030
Bierwagen, S., Price, D., Pack, A., and Meyer, C. (2017). Bluespine unicornfish (Naso unicornis) are both natural control agents and mobile vectors for invasive algae in a Hawaiian marine reserve. Mar. Biol. 164:25. doi: 10.1007/s00227-016-3049-x
Botsford, L., Micheli, F., and Hastings, A. (2003). Principles for the design of marine reserves. Ecol. Appl. 13, S25–S31. doi: 10.1890/1051-0761(2003)013[0025:PFTDOM]2.0.CO;2
Bozec, Y., O’Farrell, S., Bruggemann, J., Luckhurst, B., and Mumby, P. (2016). Tradeoffs between fisheries harvest and the resilience of coral reefs. Proc. Natl. Acad. Sci. U.S.A. 113, 4536–4541. doi: 10.1073/pnas.1601529113
Choat, J., Robbins, W., and Clements, K. (2004). The trophic status of herbivorous fishes on coral reefs. Mar. Biol. 145, 445–454. doi: 10.1007/s00442-016-3753-8
Christie, M., Tissot, B., Albins, M., Beets, J., Jia, Y., Ortiz, D., et al. (2010). Larval connectivity in an effective network of marine protected areas. PLoS One 5:e15715. doi: 10.1371/journal.pone.0015715
Chung, A., Oliver, T., Gove, J., Gorospe, K., White, D., Davidson, K., et al. (2019). Translating resilience-based management theory to practice for coral bleaching recovery in Hawai‘i. Mar. Policy 99, 58–68. doi: 10.1016/j.marpol.2018.10.013
Claisse, J., Clark, T., Schumacher, B., McTee, S., Bushnell, M., Callan, C., et al. (2011). Conventional tagging and acoustic telemetry of a small surgeonfish, Zebrasoma flavescens, in a structurally complex coral reef environment. Environ. Biol. Fishes 91, 185–201. doi: 10.1007/s10641-011-9771-9
Costa, B. M., and Kendall, M. S. (2016). “Marine biogeographic assessment of the main Hawaiian Islands,” Bureau of Ocean Energy Management and National Oceanic and Atmospheric Administration, (New Orleans, LA: Office of the Environment), 35.
Department of Land and Natural Resources [DLNR] (2018). State of Hawai‘i, Division of Aquatic Resources. Available at: http://dlnr.hawaii.gov/dar/
Dollar, S. (1982). Wave stress and coral community structure in Hawai‘i. Coral Reefs 1, 71–81. doi: 10.1002/ecy.1884
Donovan, M., Friedlander, A., Lecky, J., Jouffray, J., Williams, G., Wedding, L., et al. (2018). Combining fish and benthic communities into multiple regimes reveals complex reef dynamics. Sci. Rep. 8:16943. doi: 10.1038/s41598-018-35057-4
Eristhee, N. (2001). Home range size and use of space by Bermuda chub Kyphosus sectatrix (L.) in two marine reserves in the Soufriere marine management area, St Lucia, West Indies. J. Fish Biol. 59, 129–151. doi: 10.1006/jfbi.2001.1754
Fernandes, L., Day, J., Lewis, A., Slegers, S., Kerrigan, B., Breen, D., et al. (2005). Establishing representative no-take areas in the great barrier reef: large-scale implementation of theory on marine protected areas. Conserv. Biol. 19, 1733–1744. doi: 10.1111/j.1523-1739.2005.00302.x
Friedlander, A., Brown, E., and Monaco, M. (2007a). Coupling ecology and GIS to evaluate efficacy of marine protected areas in Hawai‘i. Ecol. Appl. 17, 715–730.
Friedlander, A., Brown, E., and Monaco, M. (2007b). Defining reef fish habitat utilization patterns in Hawai‘i: comparisons between marine protected areas and areas open to fishing. Mar. Ecol. Prog. Ser. 351, 221–233. doi: 10.3354/meps07112
Friedlander, A., and DeMartini, E. (2002). Contrasts in density, size, and biomass of reef fishes between the northwestern and the main Hawaiian Islands: the effects of fishing down apex predators. Mar. Ecol. Prog. Ser. 230, 253–264. doi: 10.3354/meps230253
Friedlander, A., Donovan, M., Koike, H., Murakawa, P., and Goodell, W. (in press). Characteristics of effective marine protected areas in Hawai‘i. Aquat. Conserv.
Friedlander, A., and Parrish, J. (1998a). Habitat characteristics affecting fish assemblages on a Hawaiian coral reef. J. Exp. Mar. Biol. Ecol. 224, 1–30. doi: 10.1016/S0022-0981(97)00164-0
Friedlander, A., and Parrish, J. (1998b). Temporal dynamics of fish communities on an exposed shoreline in Hawai‘i. Environ. Biol. Fishes 53, 1–18. doi: 10.1023/A:1007497210998
Friedlander, A., Shackeroff, J., and Kittinger, J. (2013). Customary marine resource knowledge and use in contemporary Hawai’i 1. Pac. Sci. 67, 441–460. doi: 10.2984/67.3.10
Friedlander, A., Stamoulis, K., Kittinger, J., Drazen, J., and Tissot, B. (2014). Understanding the scale of marine protection in Hawai‘i. Adv. Mar. Biol. 69, 153–203. doi: 10.1016/B978-0-12-800214-8.00005-0
Gaines, S., White, C., Carr, M., and Palumbi, S. (2010). Designing marine reserve networks for both conservation and fisheries management. Proc. Natl. Acad. Sci. U.S.A. 107, 18286–18293. doi: 10.1073/pnas.0906473107
Goatley, C., and Bellwood, D. (2013). Ecological consequences of sediment on high-energy coral reefs. PLoS One 8:e77737. doi: 10.1371/journal.pone.0077737
Goatley, C., Bonaldo, R., Fox, R., and Bellwood, D. (2016). Sediments and herbivory as sensitive indicators of coral reef degradation. Ecol. Soc. 21:29. doi: 10.5751/ES-08334-210129
Gorospe, K., Donahue, M., Heenan, A., Gove, J., Williams, I., and Brainard, R. (2018). Local biomass baselines and the recovery potential for Hawaiian coral reef fish communities. Front. Mar. Sci. 5:162. doi: 10.3389/fmars.2018.00162
Grafeld, S., Oleson, K., Teneva, L., and Kittinger, J. (2017). Follow that fish: uncovering the hidden blue economy in coral reef fisheries. PLoS One 12:e0182104. doi: 10.1371/journal.pone.0182104
Graham, N., Ainsworth, T., Baird, A., Ban, N., Bay, L., Cinner, J., et al. (2011). From microbes to people: tractable benefits of no-take areas for coral reef. Oceanogr. Mar. Biol. Annu. Rev. 49, 105–135. doi: 10.1201/b11009-4
Graham, N., Bellwood, D., Cinner, J., Hughes, T., Norström, A., and Nyström, M. (2013). Managing resilience to reverse phase shifts in coral reefs. Front. Ecol. Environ. 11, 541–548. doi: 10.1890/120305
Graham, N., Jennings, S., MacNeil, M., Mouillot, D., and Wilson, S. (2015). Predicting climate-driven regime shifts versus rebound potential in coral reefs. Nature 518, 94–97. doi: 10.1038/nature14140
Green, A., Fernandes, L., Almany, G., Abesamis, R., McLeod, E., Aliño, P., et al. (2014). Designing marine reserves for fisheries management, biodiversity conservation, and climate change adaptation. Coast. Manag. 42, 143–159. doi: 10.1080/08920753.2014.877763
Green, A., Maypa, A., Almany, G., Rhodes, K., Weeks, R., Abesamis, R., et al. (2015). Larval dispersal and movement patterns of coral reef fishes, and implications for marine reserve network design. Biol. Rev. 90, 1215–1247. doi: 10.1111/brv.12155
Green, A., Smith, S., Lipsett-Moore, G., Groves, C., Peterson, N., Sheppard, S., et al. (2009). Designing a resilient network of marine protected areas for Kimbe Bay, Papua New Guinea. Oryx 43, 488–498. doi: 10.1017/S0030605309990342
Green, A., White, A., Kilarski, S., Fernandes, L., Tanzer, J., Porfirio, M., et al. (2013). Designing Marine Protected Area Networks to Achieve Fisheries, Biodiversity, and Climate Change Objectives in Tropical Ecosystems: A Practitioner Guide. Cebu: The Nature Conservancy.
Halford, A., and Caley, M. (2009). Towards an understanding of resilience in isolated coral reefs. Glob. Change Biol. 15, 3031–3045. doi: 10.1111/j.1365-2486.2009.01972.x
Hawai‘i Monitoring and Research Collaborative (2018). Hawai‘i Monitoring and Research Collaborative Database [database].
Heenan, A., Hoey, A., Williams, G., and Williams, I. (2016). Natural bounds on herbivorous coral reef fishes. Proc. R. Soc. B Biol. Sci. 283:20161716. doi: 10.1098/rspb.2016.1716
Hixon, M. (2015). “Reef fishes, seaweeds, and corals: a complex triangle,” in Coral Reefs in the Anthropocene, ed. C. Birkeland (Dordrecht: Springer), 195–215. doi: 10.1007/978-94-017-7249-5_10
Hoegh-Guldberg, O., Poloczanska, E., Skirving, W., and Dove, S. (2017). Coral reef ecosystems under climate change and ocean acidification. Front. Mar. Sci. 4:158. doi: 10.3389/fmars.2017.00158
Holland, K., Lowe, C., and Wetherbee, B. (1996). Movements and dispersal patterns of blue trevally (Caranx melampygus) in a fisheries conservation zone. Fish. Res. 25, 279–292. doi: 10.1016/0165-7836(95)00442-4
Holland, K., Peterson, J., Lowe, C., and Wetherbee, B. (1993). Movements, distribution and growth rates of the white goatfish Mulloides flavolineatus in a fisheries conservation zone. Bull. Mar. Sci. 52, 982–992.
Holling, C. S. (1973). Resilience and stability of ecological systems. Annu. Rev. Ecol. Syst. 4, 1–23. doi: 10.1146/annurev.es.04.110173.000245
Howard, K., Claisse, J., Clark, T., Boyle, K., and Parrish, J. (2013). Home range and movement patterns of the redlip parrotfish (Scarus rubroviolaceus) in Hawaii. Mar. Biol. 160, 1583–1595. doi: 10.1007/s00227-013-2211-y
Howard, K. G., Schumacher, B. D., and Parrish, J. D. (2009). Community structure and habitat associations of Parrotfishes on Oahu, Hawai‘i. Environ. Biol. Fishes 85, 175–186. doi: 10.1007/s10641-009-9478-3
Hughes, T. (1994). Catastrophes, phase shifts, and large-scale degradation of a Caribbean coral reef. Science 265, 1547–1551. doi: 10.1126/science.265.5178.1547
Hughes, T., Barnes, M., Bellwood, D., Cinner, J., Cumming, G., Jackson, J., et al. (2017a). Coral reefs in the Anthropocene. Nature 546, 82–90. doi: 10.1038/nature22901
Hughes, T., Kerry, J., Álvarez-Noriega, M., Álvarez-Romero, J., Anderson, K., Baird, A., et al. (2017b). Global warming and recurrent mass bleaching of corals. Nature 543, 373–377. doi: 10.1038/nature21707
Hughes, T., Bellwood, D., Folke, C., Mccook, L., and Pandolfi, J. (2007). No-take areas, herbivory and coral reef resilience. Trends Ecol. Evol. 22, 1–3. doi: 10.1016/j.tree.2006.10.009
IUCN World Commission on Protected Areas (2008). Establishing Marine Protected Area Networks: Making It Happen. Washington DC: WCPA.
Jouffray, J., Nystrom, M., Norstrom, A., Williams, I., Wedding, L., Kittinger, J., et al. (2015). Identifying multiple coral reef regimes and their drivers across the Hawaiian archipelago. Philos. Trans. R. Soc. B Biol. Sci. 370:20130268.
Kane, C. N., and Tissot, B. N. (2017). Trophic designation and live coral cover predict changes in reef-fish community structure along a shallow to mesophotic gradient in Hawaii. Coral Reefs 36, 891–901. doi: 10.1007/s00338-017-1581-x
Klein, C., Chan, A., Kircher, L., Cundiff, A. J., Gardner, N., Hrovat, Y., et al. (2008). Striking a balance between biodiversity conservation and socioeconomic viability in the design of marine protected areas. Conserv. Biol. 22, 691–700. doi: 10.1111/j.1523-1739.2008.00896.x
Kramer, K., Cotton, S., Lamson, M., and Walsh, W. (2016). Coral Bleaching: Monitoring, Management Responses and Resilience. Available at: http://coralreefs.org/wp-content/uploads/2016/12/Session-30-Kramer_etal_ICRS_Final-1-2.pdf
Lecky, J. (2016). Ecosystem Vulnerability and Mapping Cumulative Impacts on Hawaiian Reefs. Honolulu, HI: University of Hawai‘i at Manoa.
Lubchenco, J., and Grorud-Colvert, K. (2015). Making waves: the science and politics of ocean protection. Science 350, 382–383. doi: 10.1126/science.aad5443
Magris, R., Heron, S., and Pressey, R. (2015). Conservation planning for coral reefs accounting for climate warming disturbances. PLoS One 10:e0140828. doi: 10.1371/journal.pone.0140828
McClanahan, T., Ateweberhan, M., Muhando, C., Maina, J., and Mohammed, J. M. (2007). Effects of climate and seawater temperature variation on coral bleaching and mortality. Ecol. Monogr. 77, 503–525. doi: 10.1371/journal.pone.0033353
McClanahan, T., Donner, S., Maynard, J., MacNeil, M., Graham, N., Maina, J., et al. (2012). Prioritizing key resilience indicators to support coral reef management in a changing climate. PLoS One 7:e42884. doi: 10.1371/journal.pone.0042884
McClanahan, T. R. (2000). Bleaching damage and recovery potential of Maldivian coral reefs. Mar. Poll. Bull. 40, 587–597. doi: 10.1016/S0025-326X(00)00064-3
McCoy, K., Williams, I., Friedlander, A., Ma, H., Teneva, L., and Kittinger, J. (2018). Estimating nearshore coral reef-associated fisheries production from the main Hawaiian Islands. PLoS One 13:e0195840. doi: 10.1371/journal.pone.0195840
McLeod, E., Anthony, K., Mumby, P., Maynard, J., Beeden, R., Graham, N., et al. (2019). The future of resilience-based management in coral reef ecosystems. J. Environ. Manag. 233, 291–301. doi: 10.1016/j.jenvman.2018.11.034
McLeod, E., Green, A., Game, E., Anthony, K., Cinner, J., Heron, S., et al. (2012). Integrating climate and ocean change vulnerability into conservation planning. Coast. Manag. 40, 651–672. doi: 10.1080/08920753.2012.728123
McLeod, E., Salm, R., Green, A., and Almany, J. (2009). Designing marine protected area networks to address the impacts of climate change. Front. Ecol. Environ. 7, 362–370. doi: 10.1890/070211
Meyer, C., and Holland, K. (2005). Movement patterns, home range size and habitat utilization of the Bluespine unicornfish, Naso unicornis (Acanthuridae) in a Hawaiian marine reserve. Environ. Biol. Fishes 73, 201–210. doi: 10.1007/s10641-005-0559-7
Meyer, C., Papastamatiou, Y., and Clark, T. (2010). Differential movement patterns and site fidelity among trophic groups of reef fishes in a Hawaiian marine protected area. Mar. Biol. 157, 1499–1511. doi: 10.1007/s00227-010-1424-6
Moberg, F., and Folke, C. (1999). Ecological goods and services of coral reef ecosystems. Ecol. Econ. 29, 215–233. doi: 10.1016/S0921-8009(99)00009-9
Mumby, P. (2006). Fishing, trophic cascades, and the process of grazing on coral reefs. Science 311, 98–101. doi: 10.1126/science.1121129
Mumby, P., Harborne, A., Williams, J., Kappel, C. V., Brumbaugh, D. R., Micheli, F., et al. (2007). Trophic cascade facilitates coral recruitment in a marine reserve. Proc. Natl. Acad. Sci. U.S.A. 104, 8362–8367. doi: 10.1073/pnas.0702602104
Mumby, P., Wolff, N., Bozec, Y., Chollett, I., and Halloran, P. (2014). Operationalizing the resilience of coral reefs in an era of climate change: mapping resilience. Conserv. Lett. 7, 176–187. doi: 10.1111/conl.12047
Nadon, M. O. (2017). Stock assessment of the coral reef fishes of Hawaii, 2016. U.S. Dep. Commer., NOAA Tech. Memo., NOAA-TM-NMFS-PIFSC-60, 212. doi: 10.7289/V5/TM-PIFSC-60
NOAA Coral Reef Watch (2015). Updated Daily. NOAA Coral Reef Watch Version 3.0 Daily Global 5-km Satellite Virtual Station Time Series Data for the main Hawaiian Islands. College Park, MD: Coral Reef Watch.
Ong, L., and Holland, K. (2010). Bioerosion of coral reefs by two Hawaiian parrotfishes: species, size differences and fishery implications. Mar. Biol. 157, 1313–1323. doi: 10.1007/s00227-010-1411-y
Ortiz, D., and Tissot, B. (2008). Ontogenetic patterns of habitat use by reef-fish in a marine protected area network: a multi-scaled remote sensing and in situ approach. Mar. Ecol. Prog. Ser. 365, 217–232. doi: 10.3354/meps07492
Ortiz, D., and Tissot, B. (2012). Evaluating ontogenetic patterns of habitat use by reef fish in relation to the effectiveness of marine protected areas in West Hawai‘i. J. Exp. Mar. Biol. Ecol. 432–433, 83–93. doi: 10.1016/j.jembe.2012.06.005
Pillans, R., Babcock, R., Thomson, D., Haywood, M. E., Downie, R., Vanderklift, M., et al. (2017). Habitat effects on home range and schooling behaviour in a herbivorous fish (Kyphosus bigibbus) revealed by acoustic tracking. Mar. Freshw. Res. 68, 1454–1467. doi: 10.1071/MF16199
Randall, J. (1961). A contribution to the biology of the convict surgeonfish of the Hawaiian Islands, Acanthurus triostegus sandvicensis. Pac. Sci. 15,215–272.
Rodgers, K., Kido, M., Jokiel, P., Edmonds, T., and Brown, E. (2012). Use of integrated landscape indicators to evaluate the health of linked watersheds and coral reef environments in the Hawaiian Islands. Environ. Manag. 50, 21–30. doi: 10.1007/s00267-012-9867-9
Sakihara, T., Nishiura, L., Shimoda, T., Shindo, T., and Nishimoto, R. (2015). Brassy chubs Kyphosus vaigiensis display unexpected trans-island movement along inshore habitats. Environ. Biol. Fishes 98, 155–163. doi: 10.1007/s10641-014-0245-8
Salm, R., Done, T., and Mcleod, E. (2006). “Marine protected area planning in a changing climate,” in Coral Reefs and Climate Change: Science and Management, eds J. Phinney, O. Hoegh-Guldberg, J. Kleypas, W. Skirving, and A. Strong (Washington, DC: American Geophysical Union).
Schemmel, E., and Friedlander, A. (2017). Participatory fishery monitoring is successful for understanding the reproductive biology needed for local fisheries management. Environ. Biol. Fishes 100, 171–185. doi: 10.1007/s10641-016-0566-x
Smith, J., Hunter, C., and Smith, C. (2010). The effects of top–down versus bottom–up control on benthic coral reef community structure. Oecologia 163, 497–507. doi: 10.1007/s00442-009-1546-z
Smith, S., Kimmerer, W., Laws, E., Brock, R., and Walsh, T. (1981). Kaneohe bay sewage diversion experiment: perspectives on ecosystem responses to nutritional perturbation. Pac. Sci. 35, 279–395.
Stamoulis, K., Delevaux, J., Williams, I., Poti, M., Lecky, J., Costa, B., et al. (2018). Seascape models reveal places to focus coastal fisheries management. Ecol. Appl. 28, 910–925. doi: 10.1002/eap.1696
Stamoulis, K., and Friedlander, A. (2013). A seascape approach to investigating fish spillover across a marine protected area boundary in Hawai‘i. Fish. Res. 144, 2–14. doi: 10.1016/j.fishres.2012.09.016
Stamoulis, K., Friedlander, A., Meyer, C., Fernandez-Silva, I., and Toonen, R. (2017). Coral reef grazer-benthos dynamics complicated by invasive algae in a small marine reserve. Sci. Rep. 7:43819. doi: 10.1038/srep43819
Sustainable Hawai‘i Initiative (2018). Available at: https://governor.hawaii.gov/sustainable-hawaii-initiative/
Toonen, R., Andrews, K., Baums, I., Bird, C., Concepcion, G., Daly-Engel, T., et al. (2011). Defining boundaries for ecosystem-based management: a multispecies case study of marine connectivity across the Hawaiian Archipelago. J. Mar. Biol. 2011, 1–13. doi: 10.1155/2011/460173
Van Hooidonk, R., Maynard, J., Tamelander, J., Gove, J., Ahmadia, G., Raymundo, L., et al. (2016). Local-scale projections of coral reef futures and implications of the Paris agreement. Sci. Rep. 6:39666. doi: 10.1038/srep39666
Wedding, L., Lecky, J., Gove, J., Walecka, H., Donovan, M., Williams, G., et al. (2018). Advancing the integration of spatial data to map human and natural drivers on coral reefs. PLoS One 13:e0189792. doi: 10.1371/journal.pone.0189792
Wedding, L. M., and Friedlander, A. M. (2008). Determining the influence of seascape structure on coral reef fishes in Hawaii using a geospatial approach. Mar. Geod. 31, 246–266. doi: 10.1080/01490410802466504
Wedding, L. M., Friedlander, A. M., McGranaghan, M., Yost, R. S., and Monaco, M. E. (2008). Using bathymetric lidar to define nearshore benthic habitat complexity: implications for management of reef fish assemblages in Hawaii. Remote Sens. Environ. 112, 4159–4165. doi: 10.1016/j.rse.2008.01.025
Weeks, R., Green, A., Joseph, E., Peterson, N., and Terk, E. (2017). Using reef fish movement to inform marine reserve design. J. Appl. Ecol. 54, 145–152. doi: 10.1111/1365-2664.12736
Wedding, L. M., Lepczyk, C. A., Pittman, S. J., Friedlander, A. M., and Jorgensen, S. (2011). Quantifying seascape structure: extending terrestrial spatial pattern metrics to the marine realm. Mar. Ecol. Prog. Ser. 427, 219–232.
Williams, I., and Ma, H. (2013). Estimating Catch Weight of Reef Fish Species Using Estimation and Intercept Data from the Hawai‘i Marine Recreational Fishing Survey. Honolulu, HI: NOAA Pacific Islands Fisheries Science Center.
Williams, I., Richards, B., Sandin, S., Baum, J., Schroeder, R., Nadon, M., et al. (2011). Differences in reef fish assemblages between populated and remote reefs spanning multiple archipelagos across the central and western pacific. J. Mar. Biol. 2011:826234. doi: 10.1155/2011/826234
Williams, I., Walsh, W., Claisse, J., Tissot, B., and Stamoulis, K. (2009). Impacts of a Hawaiian marine protected area network on the abundance and fishery sustainability of the yellow tang, Zebrasoma flavescens. Biol. Conserv. 142, 1066–1073. doi: 10.1016/j.biocon.2008.12.029
Williams, I., White, D., Sparks, R., Lino, K., Zamzow, J., Kelly, E., et al. (2016). Responses of herbivorous fishes and benthos to 6 years of protection at the Kahekili herbivore fisheries management area, Maui. PLoS One 11:e0159100. doi: 10.1371/journal.pone.0159100
Wilson, J., Darmawan, A., Subijanto, J., Green, A., and Sheppard, S. (2011). Scientific Design of a Resilient Network of Marine Protected Areas. Lesser Sunda Ecoregion, Coral Triangle. Sanur: The Nature Conservancy.
Keywords: coral bleaching, marine protected areas, herbivore management, systematic conservation planning, coral reef resilience
Citation: Chung AE, Wedding LM, Green AL, Friedlander AM, Goldberg G, Meadows A and Hixon MA (2019) Building Coral Reef Resilience Through Spatial Herbivore Management. Front. Mar. Sci. 6:98. doi: 10.3389/fmars.2019.00098
Received: 14 September 2018; Accepted: 18 February 2019;
Published: 19 March 2019.
Edited by:
Michael Sweet, University of Derby, United KingdomReviewed by:
Carlos Eduardo Leite Ferreira, Universidade Federal Fluminense, BrazilDominic A. Andradi-Brown, World Wildlife Fund, United States
Copyright © 2019 Chung, Wedding, Green, Friedlander, Goldberg, Meadows and Hixon. This is an open-access article distributed under the terms of the Creative Commons Attribution License (CC BY). The use, distribution or reproduction in other forums is permitted, provided the original author(s) and the copyright owner(s) are credited and that the original publication in this journal is cited, in accordance with accepted academic practice. No use, distribution or reproduction is permitted which does not comply with these terms.
*Correspondence: Anne E. Chung, YW5uZWVyQGhhd2FpaS5lZHU=