- 1Programa de Pós-Graduação em Oceanografia Física, Química e Geológica, Instituto de Oceanografia, Universidade Federal do Rio Grande, Rio Grande, Brazil
- 2Instituto de Pesquisas Jardim Botânico do Rio de Janeiro, Rio de Janeiro, Brazil
One of the most important contributions of crustose coralline algae (CCA) to some coral reefs is their structural role in sunlit habitats, but in the Atlantic southernmost coral reef, Abrolhos, these algae are also important components of living communities covering larger areas than corals. Little is known about their competence in occupying reef space and consequently their ecological role. This work compared two CCA species along reef sites and habitats and their responses to different irradiance levels. To study colonization, epoxy disks were placed at four sites and three habitats (reef base, reef flat, and reef edge). Crustose coralline individual pieces were glued onto epoxy disks and their relative growth was estimated. Productivity responses to irradiance levels found on reef habitats was measured on incubated samples. In general, CCA were less abundant than filamentous algae and non-calcareous crusts. Crustose algae showed no seasonal or spatial pattern in cover, contrasting with erect algae that differed in biomass among sites depending on season. Differences among habitats were only found for CCA. The dominant coralline Porolithon onkodes was more productive and grew faster than Lithophyllum stictaeforme at high irradiance level and both species were inhibited at low light. Dominance of P. onkodes in shallow and sunlit reefs was explained by its preference for high-light environments.
Introduction
The crustose coralline algae (CCA, Corallinales Rhodophyta) on coral reefs can cover large areas, such as in the Africa (McClanahan et al., 2001b), Australia (Fabricius and De’ath, 2001), Caribbean (Adey and Vassar, 1975; McClanahan et al., 2001a; Williams and Polunin, 2001), Fiji (Littler and Littler, 1997), Hawaii (Vroom et al., 2005), and Brazil (Figueiredo, 1997; Gherardi and Bosence, 2001; Villas-Bôas et al., 2005). Studies have confirmed that together with corals, CCA are important components of the reef framework in shallow environments exposed to strong wave action in the Caribbean (Macintyre, 1997) and Brazilian reefs (Kikuchi and Leão, 1997; Leão and Dominguez, 2000; Gherardi and Bosence, 2001; Leão and Kikuchi, 2001; Lei et al., 2018). The cementation of reefs by CCA maintains reef complexity, reduces erosion (Littler, 1972; Steneck, 1986) and increases resistance to herbivore action due to calcium carbonate deposited in the cell walls (Pitlik and Paul, 1997).
According to models of seaweed (algal) form and function, proposed to describe the distribution and abundance of seaweeds, CCA are classified as a unique functional group that dominates highly productive environments with exposure to high levels of disturbance (Littler and Littler, 1980, 1984; Steneck, 1988; Steneck and Dethier, 1994; Mariath et al., 2013). Morphological characteristics of coralline algae indicate adaptations to many environmental and biological factors, such as wave exposure, light intensity, sediment deposition, competition, and herbivory (Steneck, 1986, 1988; Steneck and Dethier, 1994).
Herbivory is regarded as one of the main processes maintaining the structure and diversity of tropical reefs (Bellwood et al., 2004). In tropical coral ecosystems, herbivorous fishes play key roles in the carbon and energy flux of food webs and are considered some of the primary determinants of the benthic structure (Clements et al., 2009). Herbivorous fish assemblages, dominated by surgeonfishes (Acanthuridae) and parrotfishes (Labridae: Scarini), are widely regarded for their importance in the control of the settlement, growth, and spread of benthic seaweeds (Steneck, 1988; Horn, 1989).
Several descriptive studies have related patterns of distribution and abundance of these algae to environmental parameters that influence coral reefs (Adey and Vassar, 1975; Littler et al., 1995; Steneck, 1997; Fabricius and De’ath, 2001; Figueiredo and Steneck, 2002). The biological limits of CCA were analyzed for a few temperate and subtropical species, showing influence of temperature and light intensity (Adey, 1970; Edyvean and Ford, 1987; Matsuda, 1989; Leukart, 1994), competition and herbivory (Steneck et al., 1991; Keats et al., 1994; Figueiredo et al., 1996). In contrast, experimental studies that test the effect of environmental factors on growth and production of CCA on coral reefs are less common and consist of studies of irradiance (Littler, 1973), sediments (Kendrick, 1991), and herbivory (Steneck and Adey, 1976; Figueiredo, 1997).
Among the most important reef builders on the Atlantic Ocean are the CCA Porolithon onkodes (Heydrich) Foslie and Lithophyllum stictaeforme (J.E. Areschoug) Hauck, which form algal crests on reefs edges (Adey, 1975; Steneck and Adey, 1976; Tâmega et al., 2014; Spotorno-Oliveira et al., 2015). These two coralline species have distinct growth forms: P. onkodes is encrusting and L. stictaeforme has branched thalli. Both species are commonly found on shallow reefs of the Abrolhos Archipelago in Brazil (Figueiredo and Steneck, 2002; Tâmega and Figueiredo, 2007; Tâmega et al., 2014). This study aims to describe the early colonization and growth of CCA on the reef flat, edge and base of sheltered and exposed sites in summer and winter, testing the responses of the two most common coralline species to different light levels.
Preliminary observations of seaweeds on the reef community of Abrolhos Archipelago generated three hypotheses that were tested in field and laboratory experiments:
H1: CCA are more abundant than other form-functional seaweeds groups in early stages of colonization in any of the studied reef habitats, sites and seasons.
H2: Encrusting CCA have faster marginal growth than branched CCA in any of the studied reef habitats, sites, and seasons.
H3: Encrusting CCA are more productive than branched CCA in environments under high irradiance.
Materials and Methods
Study Sites
The present study was conducted in Abrolhos Archipelago (17° 57′–17° 59′S and 38° 41′–38° 43′W; Figure 1) about 70 km off North-eastern Brazil. This archipelago is composed by five volcanic islands bordered by fringing reefs and is included in the Abrolhos Marine National Park. The average seawater temperature ranges from 23 to 27°C (Muehe, 1988) and salinity from 36.5 to 36.7 (Muehe, 1988). In autumn and winter (from March to September), winds are mostly from the south. In spring and summer (September to February), winds are from the east to north. Tides range from ± 2.4 to 0.1 m in the north (Porto de Ilhéus) and ± 1.8 to 0.0 m in the south (Barra do Riacho) (Muehe, 1988).
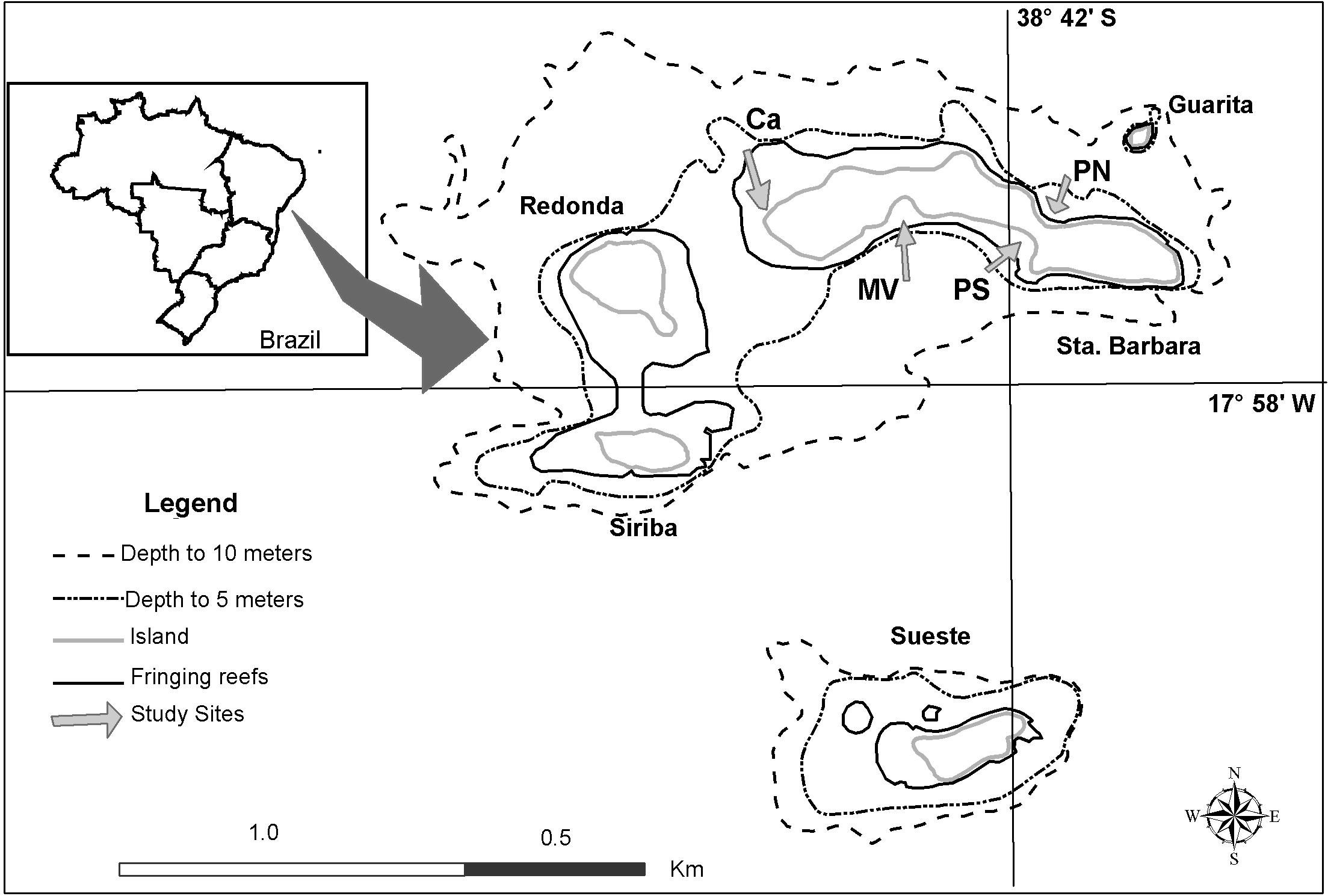
Figure 1. Study sites in the Abrolhos Marine National Park: Porto Norte (PN), Caldeiros (Ca), Mato Verde (MV), and Porto Sul (PS).
Study sites at Santa Barbara Island were selected according to reef orientation: Porto Norte (N), Caldeiros (NW), Mato Verde (SW), and Porto Sul (S) (Figure 1). Studies were run during two periods: December 2001 to March 2002 (austral summer) and July 2002 to October 2002 (austral winter and spring). Three habitats were studied: reef flat, edge, and slope base in Mato Verde, during austral winter. Reef flats are usually exposed to air on extreme spring low tides. Reef bases are at 4 to 5 m depth. Reefs have a gradual slope on Porto Norte and Porto Sul, while at Caldeiros and Mato Verde they have steep slopes from 50°. Sites were compared only at the reef edge in both seasons, where corallines are more abundant (Figueiredo, 1997; Tâmega and Figueiredo, 2007).
Colonization
The colonization of CCA and erect seaweeds was followed at all study sites, 10 replicate disks made of epoxy (Tubolit), 75 mm diameter, were fixed by epoxy to the substratum at each study site. After 3 months each disk was recovered and stored in a plastic bag with formalin solution (4% in seawater). The cover of colonizing seaweeds was analyzed using a Petri dish marked with 47 random points, placed on the surface of each disk. Seaweeds were separated in functional form groups (Littler and Littler, 1984; Steneck and Dethier, 1994) and also identified to species level when possible. Sorted samples were oven dried at 60°C for 72 h until their mass was constant (not changing with time).
Colonizing seaweeds can be limited by herbivores such as parrotfishes (Labridae: Scarini), that are able to excavate calcified thalli with their fused teeth (Steneck, 1986).
Growth Rates
Growth was compared between two CCA, L. stictaeforme and P. onkodes. CCA were collected with a hammer and chisel and shaped by cutting pliers to obtain 20 mm diameter and 3 ml volume disks. A single disk naturally free of epiphytes was fixed onto an epoxy disk of 30 mm diameter (for each crustose coralline species, n = 10 per location). Disks of both CCA species were interspersed, fixed to the reef with epoxy and left for the same period as colonization disks. The initial and final sizes of CCA were estimated by averaging two perpendicular diameters measured with calipers. The formula used to calculate the marginal relative growth rate (RGR) was:
which is based on the difference between the logarithms of final and initial average diameter (D) over time (t), following recommendations of Kain (1987). L. stictaeforme also had thickness (vertical) growth measured but in P. onkodes only marginal (horizontal) growth was measured as it was considered that vertical growth was not sufficient to be detected in this species during such a short deployment time. CCA species were identified following Tâmega et al. (2014).
Productivity
To estimate the competence of the two most abundant CCA species under different irradiance levels, oxygen produced by CCA through net photosynthesis was measured at different levels of light intensities naturally found at each habitat. The irradiance at each site was measured with a Li-Cor light meter (LI-1000) linked to a terrestrial and submersible sensor to estimate the percentage of incident irradiance reduced by the water column. In the field, the irradiance was measured at midday hours in the summer on sunny days. The light levels of habitats were reproduced in the laboratory by 4, 6, or 10 fluorescent lamps (daylight, 20 watts each), and in the field by shading with 1 or 3 layers of black plastic grids, with 3 mm mesh diameter.
Fragments of the two species of CCA were collected and cleaned of epiphytes with brush and forceps. Seawater was filtered through coarse filter paper in the field or by Millipore 0.47 μm pore and sterilized at boiling point to fill 300 ml BOD bottles. Seaweed samples of standard size (diameter 20 mm) and volume of 3 ml were distributed among clear BOD bottles (with or without shades) to measure O2 production. One clear BOD bottle with only seawater was used as an initial reference for O2 production. Number of replicates varied between 8 and 12, in the laboratory and field experiments, respectively. In the laboratory experiments, CCA samples were acclimated to light levels lower than the ones found at the reef base, 2 weeks prior to the experiments. Dissolved oxygen readings were measured by a sensor with a stirrer linked to an oximeter (YSI 5000). Net photosynthesis was calculated to estimate primary productivity using the modified formula of Thomas (1988):
At the end of experiments, CCA were decalcified by a solution of 10% nitric acid and oven dried at 60°C to adjust O2 production readings to sample weight. Incubation time (4 h) was previously tested and showed no difference from either 2 or 6 h, though shorter times resulted in negative readings at some light levels. Pigment concentration of CCA species was assumed to be similar because only sunlit specimens were used in experiments. Nutrients and carbon depletion did not interfere with results. Similar levels of nitrate, phosphate, and alkalinity between incubated samples and surrounding seawater were found for P. onkodes after 4 h of incubation (pers. comm. S. M. Ribeiro). Levels of irradiance used in field tests corresponded to ones mentioned for CCA in shallow reefs (Littler and Littler, 1984), being close to saturated photosynthesis (623 and 408 μmol m−2 s−1), photo-inhibition (1093 and 716 μmol m−2 s−1), or to levels below those that might limit photosynthesis (218 and 143 μmol m−2 s−1). In the laboratory, irradiance levels corresponded to those found at the reef base and within cryptic habitats (218, 107, and 68 μmol m−2 s−1).
Statistical Analysis
Variance homogeneity was tested using Cochrans test before performing analysis of variance (ANOVA) and, when necessary, data were transformed (Underwood, 1997). Uni or bi-factorial orthogonal ANOVAs were used to test differences between treatments in experiments. Multiple comparison of means was performed using Tukey’s test.
Results
Colonization
The most common erect seaweeds found on colonization disks were filamentous algae (Cladophora dalmatica, Sphacelaria tribuloides, Polysiphonia scopulorum, Ceramium byssoides), foliose algae (Enteromorpha flexuosa, Padina gymnospora, Colpomenia sinuosa, Dictyota ciliolata, Dictyota mertensii, Dictyota menstrualis), non-calcareous crusts (cyanobacteria, crusts) and calcareous coralline crusts.
The abundance of coralline crusts was different among sites independent of seasons (Figures 2A,B). These groups of algae were abundant at all sites, except Caldeiros. Foliose algae showed similar distribution pattern. A significant interaction between seasons and sites was found for filamentous algae and non-calcareous crusts (Figures 2A,B, ANOVA F = 2.99; p ≤ 0.05 and F = 2.99; p ≤ 0.05, respectively). The filamentous algae were abundant in almost all sites during both seasons, except in Caldeiros (ANOVA p ≤ 0.0001 and p ≤ 0.01). In contrast, non-calcareous crusts were more abundant in Caldeiros in both seasons (ANOVA p ≤ 0.0001 and p ≤ 0.0001).
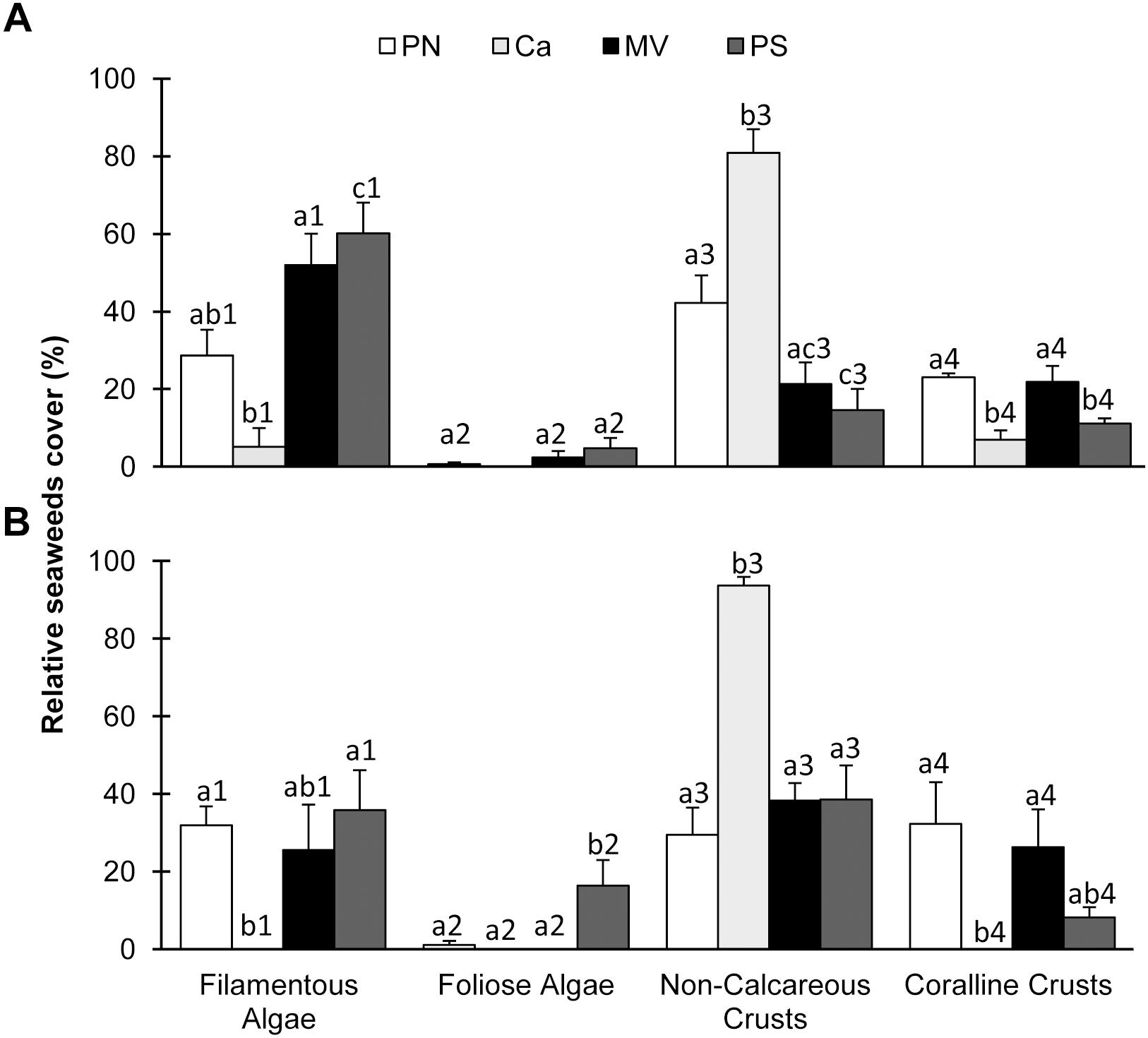
Figure 2. Seaweeds cover at study sites on reef edge, in summer (A) and winter (B). Means ± standard error. Different letters above bars indicate significant differences among means detected by Tukey’s test (p < 0.05).
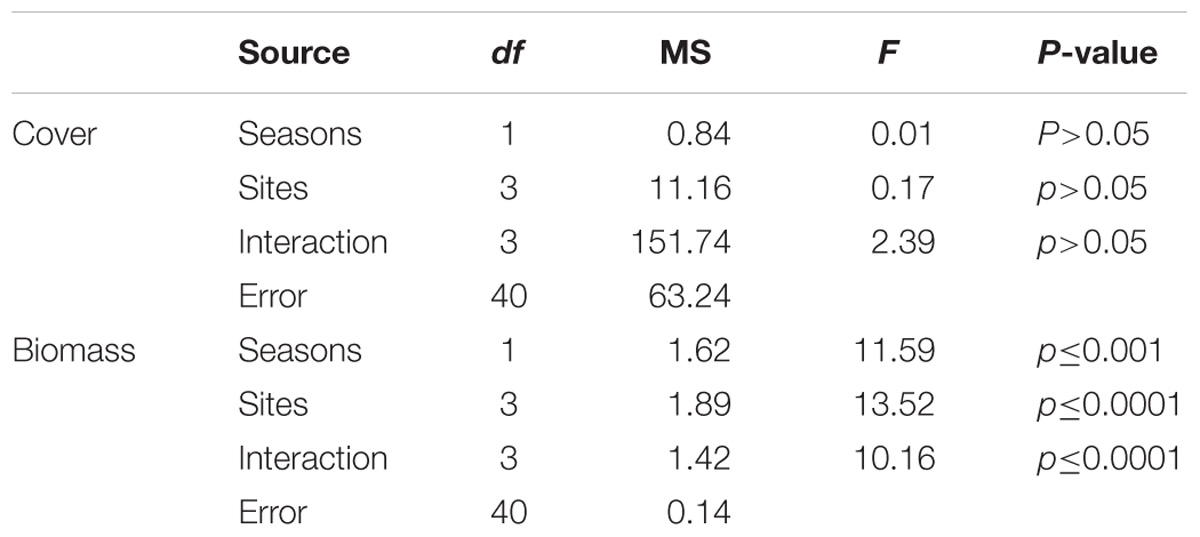
Table 1. Analysis of variance for algae colonization in relation to total cover and total biomass in study sites in summer and winter (n = 8).
A significant interaction was observed for biomass of colonizing algae between sites and seasons (Figures 3A,B and Table 1). Total biomass was different among sites in summer and in winter (ANOVA F = 5.68; p ≤ 0.05 and F = 7.11; p ≤ 0.001, respectively). There was less biomass on Caldeiros than on most sites in summer and higher biomass in Porto Sul than on most sites in winter. In relation to total cover of algae, there were no significant interactions or differences in colonization among sites or seasons, in summer the total cover ranged from 90 to 97%, and in winter from 90 to 99%.
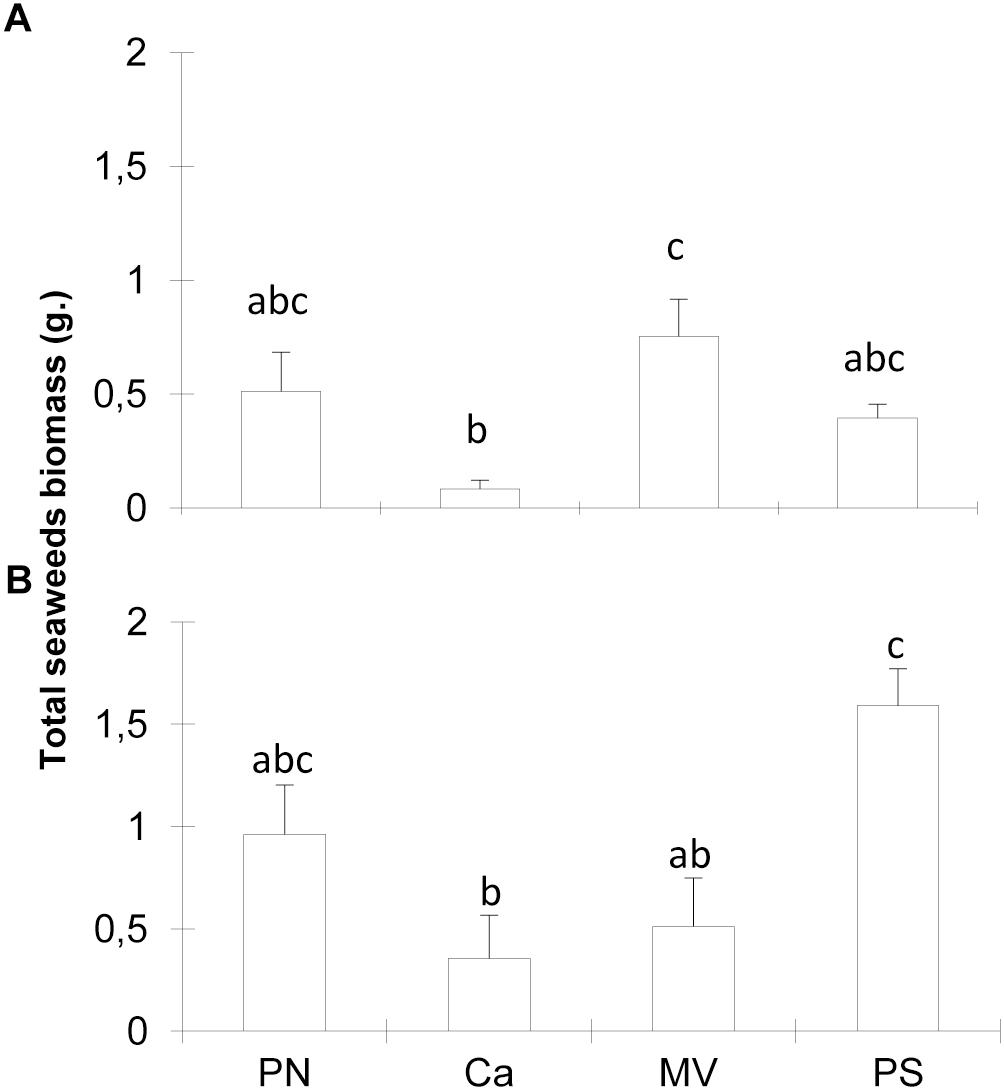
Figure 3. Seaweeds biomass at study sites on reef edge, in summer (A) and winter (B). Means ± standard error. Different letters above bars indicate significant differences among means detected by Tukey’s test (p < 0.05).
There was a significant difference in the total macroalgal biomass among habitats (Figure 4A, F = 4.85, p ≤ 0.05), which was higher at the reef base and lower at both reef flat and edge. Similarly, total algae cover did not differ significantly among reef habitats (ANOVA F = 0.65; p > 0.05). In relation to algae functional groups, coralline crusts were present at the reef base and edge, but absent on the reef flat (Figure 4B, ANOVA F = 5.37; p ≤ 0.01). Filamentous algae were abundant at the reef base and edge and absent on the reef flat (ANOVA F = 10.74; p ≤ 0.001). Foliose algae were present only at the reef base (ANOVA F = 3.38; p ≤ 0.05). Non-calcareous crusts were abundant in all habitats, and were dominant at the reef flat (ANOVA F = 38.56; p ≤ 0.0001).
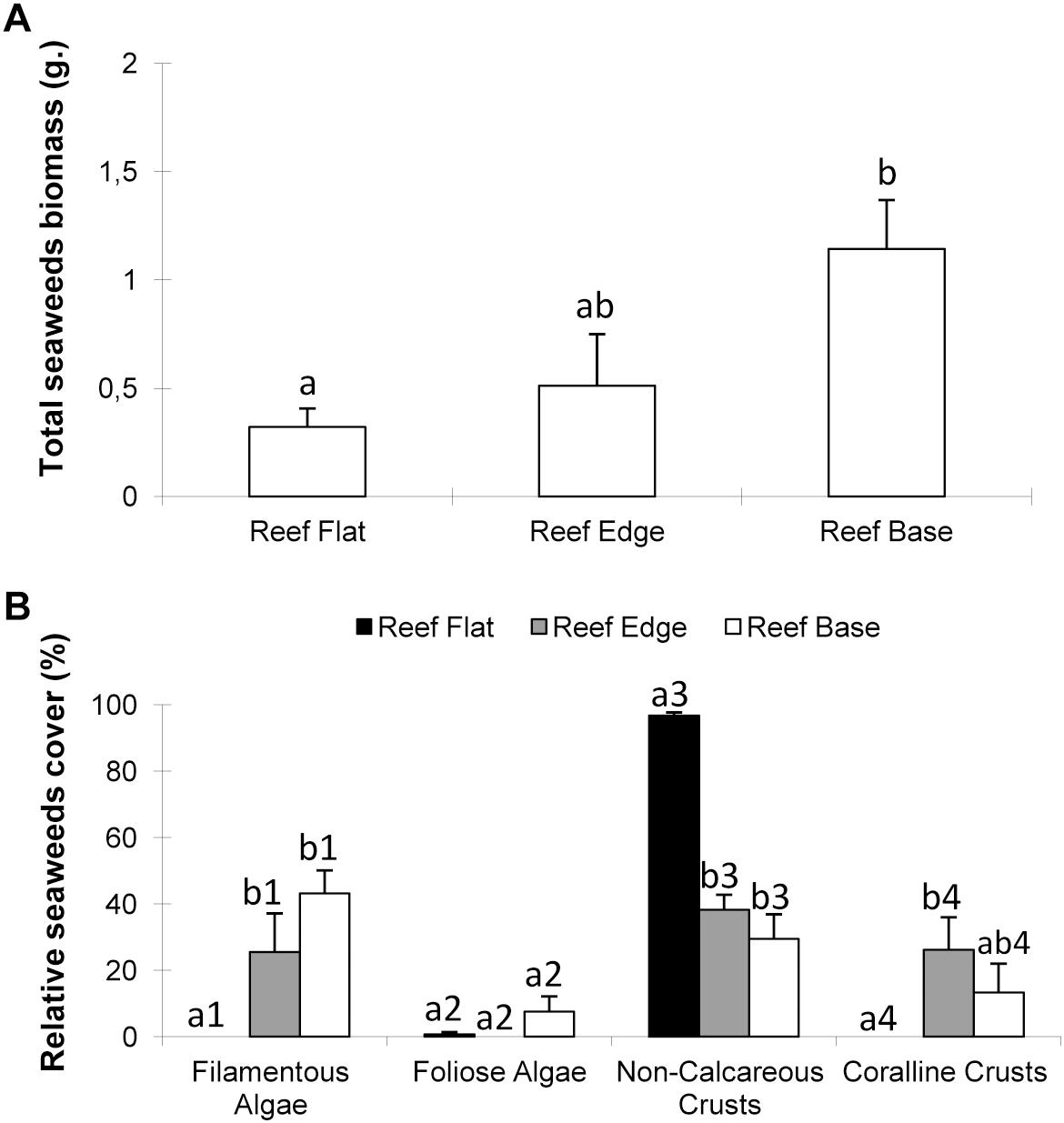
Figure 4. Seaweeds biomass (A) and seaweeds cover (B) in different habitats at Mato Verde. Means ± standard error. Different letters above bars indicate significant differences among means detected by Tukey’s test (p < 0.05).
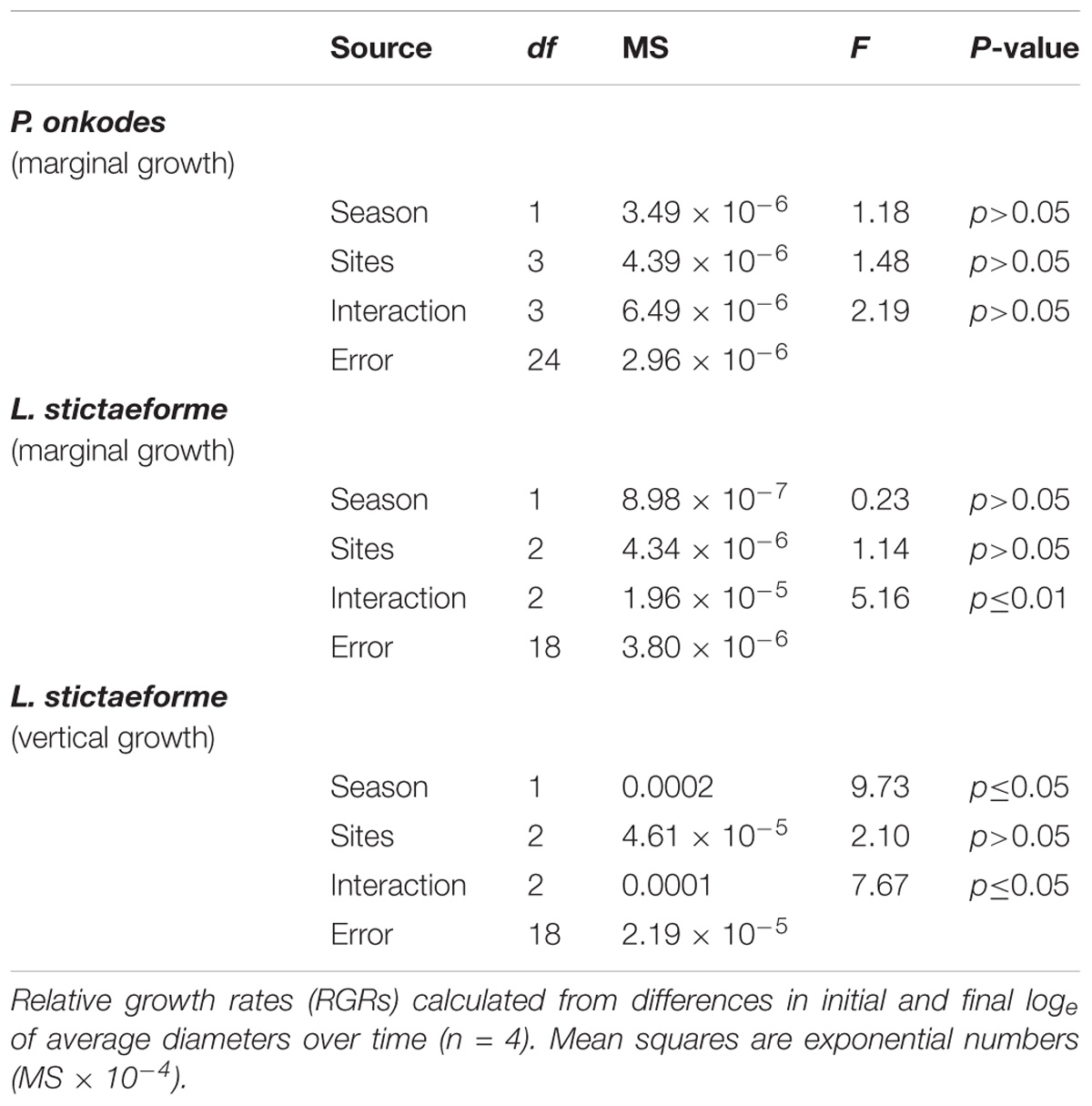
Table 2. Analysis of variance for marginal growth rate of Porolithon onkodes and Lithophyllum stictaeforme and vertical growth of L. stictaeforme in study sites in summer and winter.
Growth Rates
The absolute marginal growth ranged from 0.01 to 0.28 mm for P. onkodes and 0.01 to 0.13 mm for L. stictaeforme. The vertical growth of this second species varied from 0.02 to 0.23 mm. Marginal relative growth of P. onkodes between seasons and among sites though was not significantly different, a small growth was observed at Caldeiros in winter (Figures 5A,B and Table 2). Marginal relative growth of L. stictaeforme, had a strong significant interaction among sites and seasons, growth was negative in Caldeiros differing from all other sites only in winter, (Figures 5C,D and Table 2). Comparing seasons in Caldeiros, growth was positive in summer and negative in winter (ANOVA F = 30.35; p ≤ 0.001). In Mato Verde and Porto Sul growth was negative in summer and positive in winter, but differences between seasons were not significant (ANOVA F = 2.10; p > 0.05 and F = 1.70; p > 0.05, respectively). In summer growth could not be measured at Porto Norte because samples were lost due to strong wave surge.
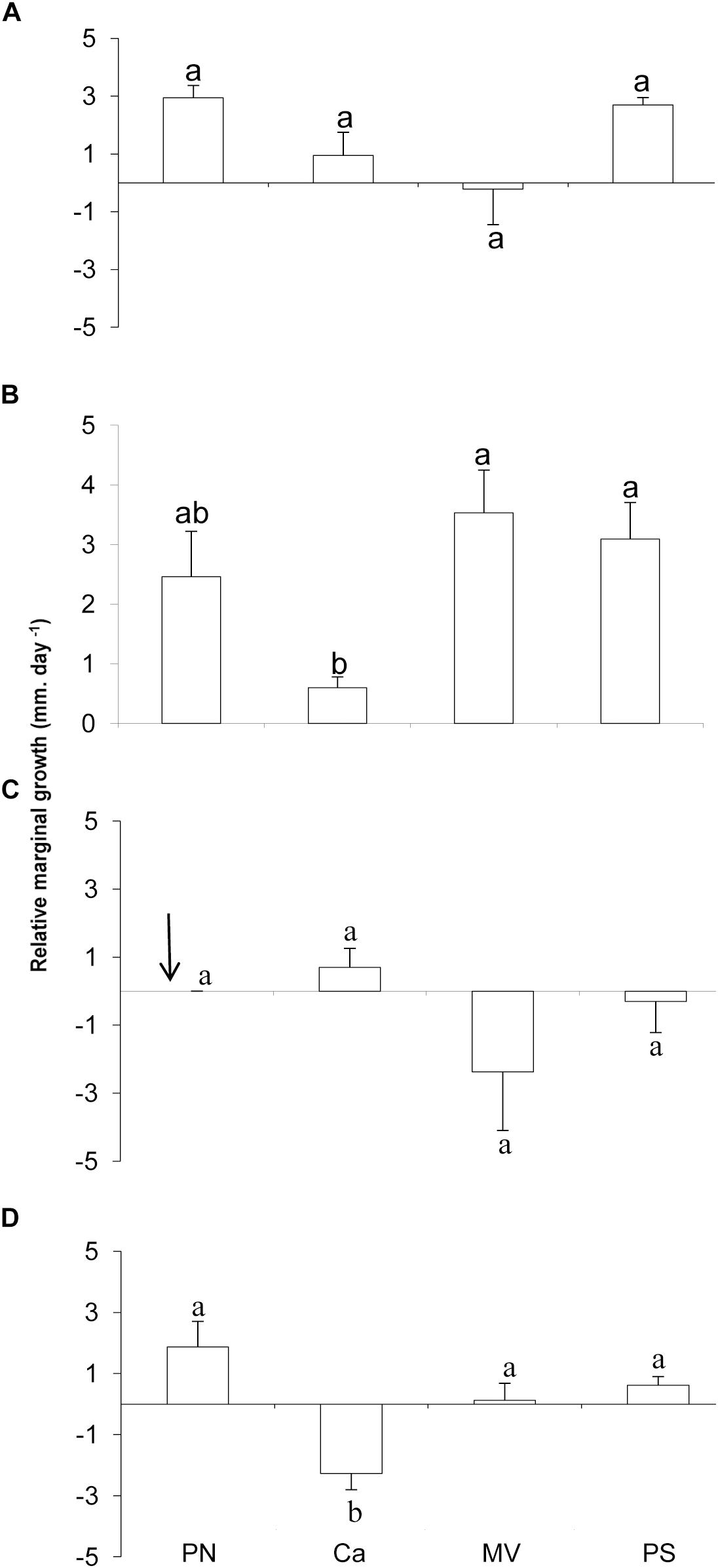
Figure 5. Relative marginal growth of Porolithon onkodes at study sites on reef edge, in summer (A) and winter (B), and Lithophyllum stictaeforme in summer (C) and winter (D). Arrow indicates missing data. Means ± standard error. Different letters above bars indicate significant differences among means detected by Tukey’s test (p < 0.05).
Vertical growth rate of L. stictaeforme showed a similar pattern as marginal growth with a significant interaction among seasons and sites. Again Caldeiros showed different growth rates from all other sites but only in winter. (Figures 6A,B and Table 2). In Caldeiros, there was a positive growth in summer and negative in winter. (ANOVA F = 60.77; p ≤ 0.001). In Mato Verde and Porto Sul there was no significative difference between summer and winter (respectively, ANOVA F = 1.49; p > 0.05 and F = 0.81; p > 0.05).
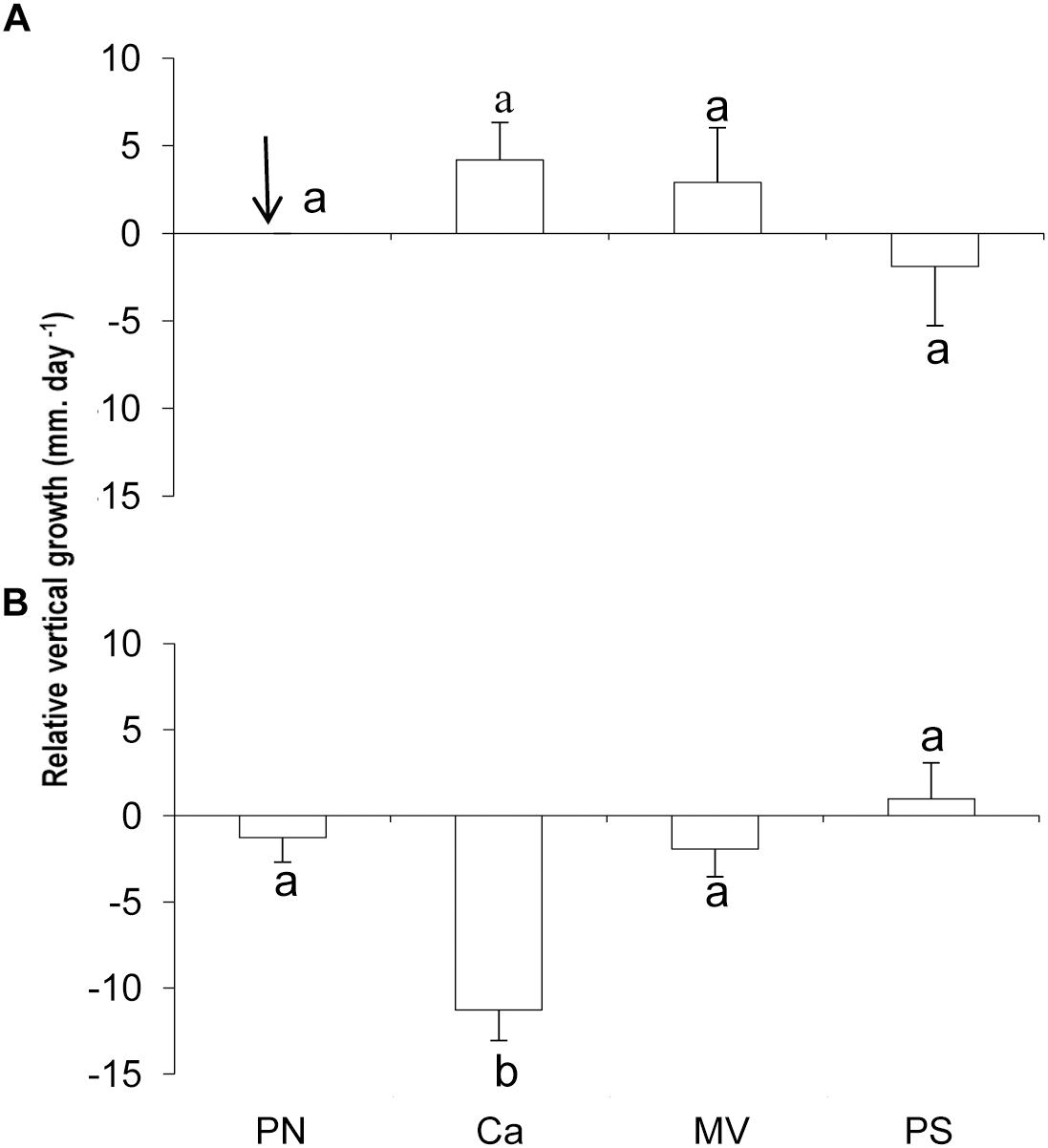
Figure 6. Relative vertical growth of L. stictaeforme at study sites on reef edge, in summer (A) and winter (B). Missing data (↓). Means ± standard error. Different letters above bars indicate significant differences among means detected by Tukey’s test (p < 0.05).
Comparing marginal relative growth among habitats there was a significant difference for P. onkodes (Figure 7A, ANOVA F = 11.76; p ≤ 0.001), being higher on the reef edge and reef base and zero on reef flat. However, L. stictaeforme, had an inconspicuous marginal growth on both edge and base and zero on the reef flat, so differences was not significant among habitats. (Figure 7B, ANOVA F = 0.008; p > 0.05). There was also no significant difference in vertical growth for L. stictaeforme among habitats (ANOVA F = 0.67; p > 0.05), since growth was negative on both edge and base and zero on the reef flat. Epiphyte algae overgrew some coralline crusts but this cover was not enough to interfere with their growth in any habitat.
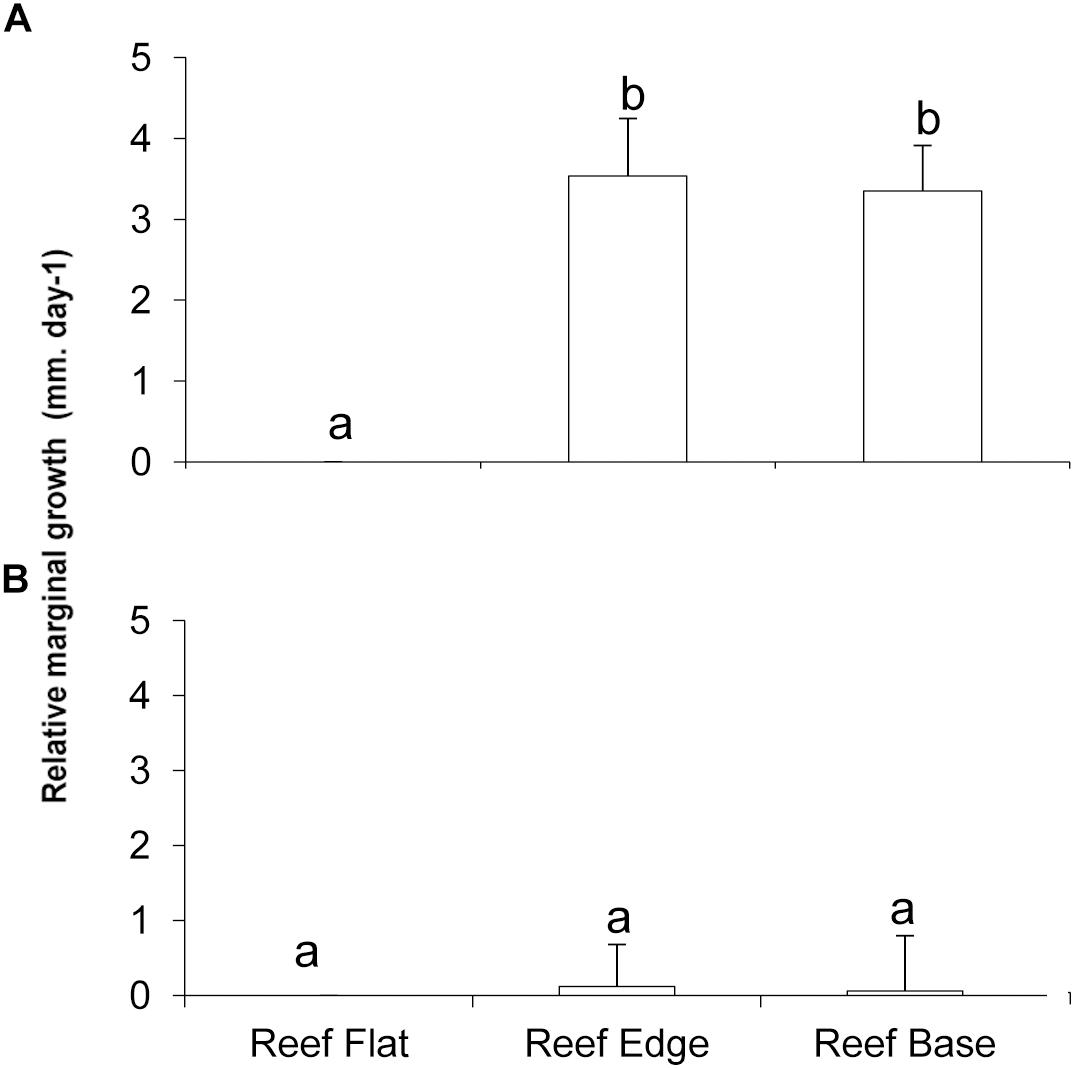
Figure 7. Relative marginal growth of P. onkodes (A) and L. stictaeforme (B) in different habitats at Mato Verde. Means ± standard error. Different letters above bars indicate significant differences among means detected by Tukey’s test (p < 0.05).
Productivity
The maximum irradiance measured was 1975 μmol m−2 s−1 on the reef flat, 1125 μmol m−2 s−1 on the reef edge and 869 μmol m−2 s−1 on the reef base, at midday on a sunny day in summer. Considering light attenuation of 56% by water column on a spring tide, irradiance on reef base could reach as low as 105 μmol m−2 s−1 in late afternoon. Under the conditions of low irradiance simulated in the laboratory, it was observed that crustose coralline species presented significant differences at the three levels tested (218, 107, and 68 μmol m−2 s−1), O2 production being greater for P. onkodes than L. stictaeforme (Figure 8A, ANOVA F = 7.81; p ≤ 0.005 and F = 19.42; p ≤ 0.0001, respectively). In contrast, exposure to the lowest irradiance level, 68 μmol m−2 s−1, resulted in negative production in both species (Figure 8A).
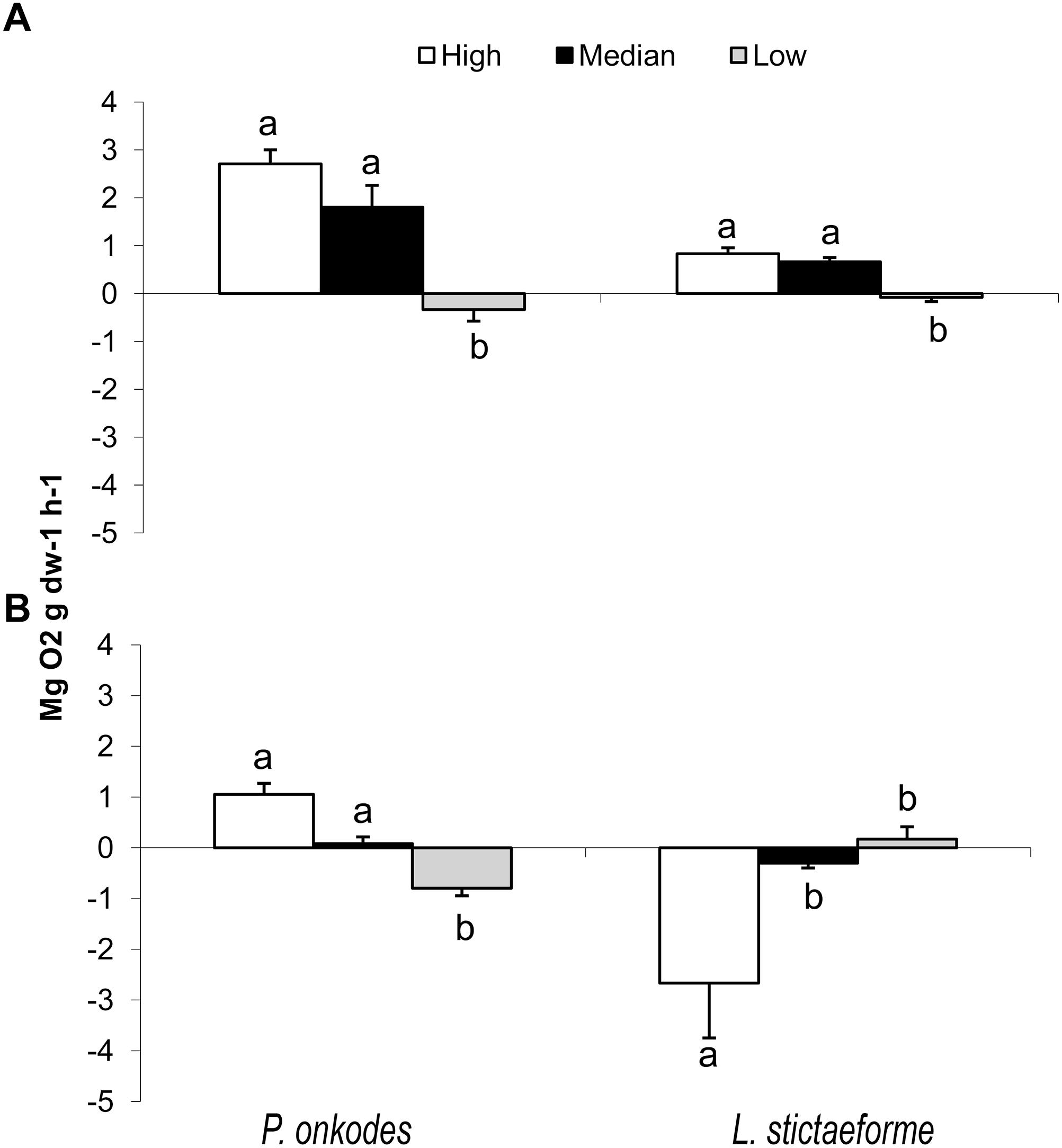
Figure 8. Responses of P. onkodes and L. stictaeforme to irradiance at high, median, and low levels in the laboratory (A) and in the field (B) showing the net photosynthesis. Means ± standard error. Different letters above bars indicate significant differences among means detected by Tukey’s test (p < 0.05).
In the field, irradiance effects on oxygen produced by P. onkodes presented significative difference among levels, being positive at 1093 (high), 623 (median), and negative at 218 (low light level) μmol m−2 s−1, (Figure 8B, ANOVA F = 30.75; p ≤ 0.0001). In contrast, L. stictaeforme exposed to equivalent irradiance levels, had a negative O2 production under high irradiances of 716 and 408 μmol m−2 s−1 and positive under the lowest one of 143 μmol m−2 s−1 (Figure 8B, ANOVA F = 4.79; p ≤ 0.05).
Discussion
Colonization
In the Abrolhos Archipelago shallow reefs are mainly dominated by the CCA P. onkodes and L. stictaeforme (Figueiredo and Steneck, 2002; Tâmega and Figueiredo, 2007; Tâmega et al., 2014; Amado-Filho et al., 2018). However, during early stages of colonization erect seaweeds may smother coralline crusts. Filamentous algae generally are small in size, being less than 5 mm in height, and have low biomass (Steneck and Watling, 1982; Hackney et al., 1989), so their proliferation in summer may contribute little to final biomass, unless they form dense turfs. Nevertheless, filamentous algae were abundant colonizers covering reef edges of most sites, except during winter in Caldeiros. This absence may be related to competition by preemption of space (Olson and Lubchenco, 1990) by the non-calcareous crusts as these crusts are thinner and might have faster growth when compared to thick CCA (Dethier, 1994). Though the non-calcareous crusts were common early colonizers in all sites and seasons they were not common on the mature reef communities. These crusts occur seasonally during the development of communities but may be less successful than other fast growing ephemeral algae that may shade or sweep the substratum (Dethier, 1981). The CCA are long living crusts and are more resistant than non-calcareous crusts to consumption by grazers (Steneck and Dethier, 1994) and generally dominate in environments under moderate herbivore pressure (Steneck and Watling, 1982; Hackney et al., 1989; Figueiredo, 1997). However, intense herbivory could result in an inversion of this pattern in favor of non-calcareous crusts, which have faster growth (Steneck et al., 1991). In relation to the dominant algae groups, the colonization patterns found generally agreed with those found by others in Abrolhos’ reef communities. Algae turf is one of the most abundant functional groups, ranging from 15 to 80% cover in both early colonization and in mature reef communities (Figueiredo, 1997; Villaça and Pitombo, 1997; Tâmega and Figueiredo, 2007).
Biomass of colonizing algae was higher on the reef base and edge than on the reef flat. The base and edge were covered by filamentous, foliose, non-calcareous crusts, and CCA. The absence of filamentous algae on reef flats may be related to the inability of their propagules to support prolonged desiccation during low tide, as diurnal low tides are more frequent in autumn and winter (Hackney et al., 1989). Species of Polysiphonia can lose 25% of water in its thallus in only 1 or 2 h and 75 up to 90% in longer periods when exposed to air (Kain and Norton, 1990). Foliose algae were present only on the reef base, which may act as a refuge from high hydrodynamic conditions as these algae are easily removed by wave action (Norton, 1991) and as refuge for fishes (mainly Acanthuridae and Labridae: Scarini) that is reduced when depth increases (Steneck, 1997; Figueiredo and Steneck, 2002; Tâmega and Figueiredo, 2007).
Coralline crusts colonized reef edges and bases but did not grow on the reef flat, similar to non-calcareous crusts. Despite both crustose groups being able to survive most disturbances (Dethier, 1994; Steneck and Dethier, 1994), coralline crusts are easily bleached when exposed to air although they can be protected from high light intensities by epiphyte cover (Figueiredo et al., 2000). The success of CCA depends not only on the capacity of recruits to resist disturbance, but also on their ability to occupy space through marginal vegetative growth.
Growth Rates
The absolute marginal growth of P. onkodes and L. stictaeforme ranged from 0.01 to 0.28 mm and 0.01 to 0.13 mm per day, respectively, the vertical growth being 0.023 to 0.23 mm per day for the latter species. These values were close to daily rates found for the first species in the Brazilian reef: Abrolhos (0.02 to 0.17 mm, Figueiredo, 1997); Rocas Atoll (0.01 to 0.05 mm, Villas-Bôas et al., 2005), and other reef forming species in the Caribbean region (0.07 mm for P. onkodes and 0.07–0.12 mm for Neogonioliton megacarpum in Adey and Vassar, 1975), but were higher than those found in Pacific reefs (P. onkodes 0.02 mm, Matsuda, 1989). Coralline crust growth is comparatively slower in temperate environments (0.02 to 0.04 mm, Huvé appud Adey, 1970; Leukart, 1994). However, studies elsewhere have based their estimates of growth on small individuals during early colonization and thus, they proportionally detected greater marginal extension rates.
In general, marginal growth of P. onkodes crusts was positive at all sites in both seasons, except in Mato Verde in summer. Cover of epiphytic algae, especially non-calcareous crusts may have influenced crustose coralline growth because they were abundant when growth was reduced or absent. In contrast, marginal growth of L. stictaeforme crusts were negative or absent in most sites in both seasons, but significantly higher in Caldeiros in summer. This result can also be related to epiphytic cover mainly by non-calcareous crusts and articulated calcareous algae. Comparing reef habitats, lateral growth of P. onkodes was positive on the reef edge and base, but zero on the reef flat, which could be explained by desiccation plus high epiphytic cover of non-calcareous crusts. The minute or imperceptible marginal growth of L. stictaeforme might also have resulted from desiccation and epiphytes, which out-competed coralline crusts on the reef flats.
Lithophyllum stictaeforme develops well at depths around 0.3 to 4 m in regions where wave action is greater (Steneck and Adey, 1976). In shallow habitats, protected from waves, the CCA need herbivores to remove epiphytes, especially turf algae (Steneck, 1997), which may limit their growth (Williams and Carpenter, 1990; Figueiredo et al., 2000). The mechanism of sloughing epithelial cells to remove epiphytes (e.g., Masaki et al., 1984; Keats et al., 1997) is efficient in less productive environments, such as under canopies (Figueiredo et al., 1997) and probably in cryptic environments and at great depths (Steneck, 1997). In the sites at the Abrolhos, it was observed that epiphytic seaweeds were drastically reduced on P. onkodes on the reef edge and base, where herbivorous fishes are more frequently seen (Figueiredo and Steneck, 2002; Tâmega et al., 2016). However, epiphytes proliferated on L. stictaeforme in all habitats, probably because branches of these algae protect epiphytes against potential herbivory by acanthurids. In contrast, the vertical growth of L. stictaeforme was negative on the reef base and edge probably due to herbivory by parrotfishes, which are more adapted to consume branches and large portions of coralline crusts. The ramified forms of L. stictaeforme attract these fishes, resulting in a drastic loss of biomass (Steneck and Adey, 1976). Preliminary experiments with transplants of L. stictaeforme in Abrolhos demonstrated high consumption rates (40 to 80% of its branches to 12 h, unpublished data). Studies demonstrated that tropical seaweeds could survive and grow following ingestion by surgeonfishes and parrotfishes in the Caribbean (Vermeij et al., 2013) and Brazil (Tâmega et al., 2016), suggesting that these fishes contribute to the dispersion of seaweeds on reefs.
Productivity
In the laboratory P. onkodes usually responded better than L. stictaeforme under low irradiance, but under 68 μmol m−2 s−1 both species showed negative net O2 production, which indicates that this level is beneath their compensation point (Bessell-Browne et al., 2017). In the field P. onkodes was more productive under irradiance levels up to 623 μmol m−2 s−1, but in contrast to the lab, showed negative growth when exposed to 218 μmol m−2 s−1.
Lithophyllum stictaeforme responded positively, as expected, to equivalent levels of irradiance (143 μmol m−2 s−1), but was photo-inhibited under irradiance up to 408 μmol m−2 s−1. The encrusting coralline algae P. onkodes not only grew better but was also more productive in environments subjected to high irradiance, when compared to L. stictaeforme.
Deposited sediments can limit incident light. Sediments are more easily trapped among turf algae than among other erect macroalgal thalli. In fact, sediments on disks tended to accumulate more on the reef base, where filamentous turfs are more abundant than on the reef flat (unpublished data). According to Fabricius and De’ath (2001), sedimentation increases even more with a slight reef slope, resulting in a decrease of CCA abundance. Filamentous turfs that trap sediments might also cause damage to the encrusting coralline algae because sediments create an anoxic area over the epithelial cells, suffocating these crusts (Connell and Slatyer, 1977; Kendrick, 1991; Steneck, 1997). Crustose corallines were as abundant on the reef edge as on the reef base, thus it was assumed that the deposition of sediments trapped by erect seaweeds was too low to affect CCA.
On the reef edge, crusts of P. onkodes are twice as abundant as branched L. stictaeforme (Tâmega and Figueiredo, 2007), which is apparently more common in cryptic habitats, such as reef crevices. In all experiments, flat crusts of P. onkodes responded better than branched crusts of L. stictaeforme to high irradiances, showing marginal growth rates up to four times faster in sunlit areas. In fact, fused branches of L. stictaeforme may indicate photoinibition of branch tips exposed directly to sunlight (Littler and Littler, 2000). Competition for space between these coralline crusts might be regulated not only by their intrinsic capacity to grow and respond to different light conditions, but also by their resistance to herbivores. It has been shown that L. stictaeforme is more likely to be consumed by Labridae: Scarini (Steneck and Adey, 1976); when transplanted to the reef edge the consumption was about 50 to 60% in the study area (unpublished data).
Conclusion
In summary, although there exist differences in biological limits between CCA, L. stictaeforme coexists with P. onkodes in habitats with high light intensity probably due to self-shading by its dense branches. L. stictaeforme showed high abundance only in cryptic reef habitats, such as crevices and beneath reefs with negative slopes. P. onkodes grew faster and was more productive under high light levels. Therefore, P. onkodes can be regarded as a plant adapted to high light environments and L. stictaeforme adapted to low light ones, where saturations levels are around 500 and 150 μmol m−2 s−1, respectively (Lüning, 1990). These CCA are highly dependent on light, as has been demonstrated for CCA in tropical and temperate environments(Adey, 1970; Leukart, 1994).
Coralline crusts were not the most common algae among early colonizers, rather filamentous turfs and non-calcareous crusts dominated the studied reefs. They are, however, usually more abundant in mature communities, possibly because of their greater resistance to disturbances (Steneck and Dethier, 1994). Changes in community structure in coral reefs are highly determined by a continuous gradient of grazing intensity (Hackney et al., 1989; Williams and Carpenter, 1990). Herbivory of coralline crusts in Abrolhos was lower (up to 10% of fish bite marks, unpublished data) when compared to former studies (up to 40% of fish bite marks, Figueiredo, 1997). Parrotfishes are one the most important and abundant herbivorous fish families on tropical reefs (Steneck, 1988) and form shoals with large individuals in the Abrolhos Archipelago (Ferreira and Gonçalves, 1999, 2006). In Abrolhos, Scarus trispinosus, one of the most abundant scarinae, has been reported to forage intensely on CCA (Ferreira and Gonçalves, 1999, 2006; Tâmega et al., 2016). We suggest that branches of L. stictaeforme might be preferentially consumed (Steneck and Adey, 1976) because its protruding branches seemed to attract herbivorous fishes (field observations), thus explaining the higher abundance of L. stictaeforme in reef refuges and its rarity on reef edges and flats. Parrotfishes are the only fish group able to excavate CCA (Steneck, 1983, 1986), but articulated calcareous, filamentous algae and a large amount of detritus are also important items in their diet (Ferreira and Gonçalves, 2006).
Author Contributions
FT and MF conceived the study, conducted the analysis, and wrote the manuscript.
Conflict of Interest Statement
The authors declare that the research was conducted in the absence of any commercial or financial relationships that could be construed as a potential conflict of interest.
Acknowledgments
We would like to thank the staff of the National Marine Park of Abrolhos who supported field work, Abrolhos Turismo for helping with boat transportation, Tubolit for providing epoxy putty, and the Brazilian Navy for their hospitality. Instituto Brasileiro do Meio Ambiente – IBAMA issued a research the license IBAMA 026/2000 for collecting samples. We are also grateful to Gary Andrew Kendrick from University of Western Australia for improving the English version and for their important comments. Comments and suggestions from the Editor NS and two reviewers improved the early version of the manuscript.
References
Adey, W. H. (1970). The effects of light and temperature on growth rates in boreal-subartic crustose coralline algae. J. Phycol. 6, 269–276. doi: 10.1371/journal.pone.0027973
Adey, W. H. (1975). The algal ridges and coral reefs of St. Croix, their structure and Holocene development. Atoll Res. Bull. 187, 1–66. doi: 10.5479/si.00775630.187.1
Adey, W. H., and Vassar, J. M. (1975). Colonization, succession and growth rates of tropical crustose coralline algae (Rhodophyta, Cryptonemiales). Phycologia 14, 55–69. doi: 10.2216/i0031-8884-14-2-55.1
Amado-Filho, G. M., Bahia, R. G., Mariath, R., Jesionek, M. B., Moura, R. L., Bastos, A. C., et al. (2018). Spatial and temporal dynamics of the abundance of crustose calcareous algae on the southernmost coral reefs of the western Atlantic (Abrolhos Bank, Brazil). Algae 33, 85–99. doi: 10.4490/algae.2018.33.2.25
Bellwood, D. R., Hughes, T. P., Folke, C., and Nyström, M. (2004). Confronting the coral reef crisis. Nature 429, 827–833. doi: 10.1038/nature02691
Bessell-Browne, P., Negri, A. P., Fisher, R., Clode, P. L., and Jones, R. (2017). Impacts of light limitation on corals and crustose coralline algae. Sci. Rep. 7:11553. doi: 10.1038/s41598-017-11783-z
Clements, K. D., Raubenheimer, D., and Choat, J. H. (2009). Nutritional ecology of marine herbivorous fishes: ten years on. Funct. Ecol. 23, 79–92. doi: 10.1111/j.1365-2435.2008.01524.x
Connell, J. H., and Slatyer, R. O. (1977). Mechanisms of succession in natural communities and their role in community stability and organization. Am. Nat. 3, 1119–1144. doi: 10.1086/283241
Dethier, M. N. (1981). Heteromorphic algal life histories: the seasonal pattern and response to herbivore of the brown crust, Ralfsia californica. Oecologia 49, 333–339. doi: 10.1007/BF00347594
Dethier, M. N. (1994). The ecology of intertidal algal crusts: variation within a functional group. J. Exp. Mar. Biol. Ecol. 177, 37–71. doi: 10.1016/0022-0981(94)90143-0
Edyvean, R. G. J., and Ford, H. (1987). Growth rates of Lithophyllum incrustans (Corallinales, Rhodophyta) from south west Wales. Br. Phycol. J. 22, 139–146. doi: 10.1080/00071618700650161
Fabricius, K., and De’ath, G. (2001). Enviromental factors associated with the spatial distribution of crustose coralline algae on the Great Barrier Reef. Coral Reefs 19, 303–309. doi: 10.1007/s003380000120
Ferreira, C. E. L., and Gonçalves, J. E. A. (1999). The unique Abrolhos reef formation (Brazil): need for specific management strategies. Coral Reefs 18:352. doi: 10.1007/s003380050211
Ferreira, C. E. L., and Gonçalves, J. E. A. (2006). Community structure and diet of roving herbivorous reef fishes in the Abrolhos Archipelago, South Western Atlantic. J. Fish Biol. 69, 1–19. doi: 10.1111/j.1095-8649.2006.01220.x
Figueiredo, M. A. O. (1997). “Colonization and growth of crustose coralline algae in Abrolhos, Brazil,” in Proceedings of the 8th International Coral Reef Symposium, Vol. 1, eds H. A. Lessios and I. G. Macintyre (Panama: Smithsonian Tropical Research Institute), 689–694.
Figueiredo, M. A. O., Kain, J. M., and Norton, T. A. (1996). Biotic interactions in the colonization of crustose coralline algae by epiphytes. J. Exp. Mar. Biol. Ecol. 199, 303–318. doi: 10.1016/0022-0981(96)00018-4
Figueiredo, M. A. O., Kain, J. M., and Norton, T. A. (2000). Responses of crustose coralline algae to epiphyte and canopy cover. J. Phycol. 36, 17–24. doi: 10.1046/j.1529-8817.2000.98208.x
Figueiredo, M. A. O., Norton, T. A., and Kain, J. M. (1997). Settlement and survival of epiphytes on two intertidal crustose coralline algae. J. Exp. Mar. Biol. Ecol 213, 247–260. doi: 10.1016/S0022-0981(96)02766-9
Figueiredo, M. A. O., and Steneck, R. S. (2002). “Floristic and ecological studies of crustose coralline algae on Brazil’s Abrolhos reefs,” in Proceedings of the Ninth International Coral Reef Symposium, Panama, 493–498.
Gherardi, D. F. M., and Bosence, D. W. J. (2001). Composition and community structure of the coralline algal reefs from Atol das Rocas, South Atlantic, Brazil. Coral Reefs 19, 205–219. doi: 10.1007/s003380000100
Hackney, J. M., Carpenter, R. C., and Adey, W. H. (1989). Characteristic adaptations to grazing among algal turfs on a Caribbean coral reef. Phycologia 28, 109–119. doi: 10.2216/i0031-8884-28-1-109.1
Horn, M. H. (1989). Biology of marine herbivorous fishes. Oceanogr. Mar. Biol. Annu. Rev. 27, 167–272.
Kain, J. M. (1987). Seasonal growth and photoinhibition in Plocamium cartilagineum (Rhodophyta) off the Isle of Man. Phycologia 26, 88–99. doi: 10.2216/i0031-8884-26-1-88.1
Kain, J. M., and Norton, T. A. (1990). “Marine ecology,” in The Biology of the Red Algae, eds K. M. Cole and R. Sheath (Cambridge: Cambridge University Press), 377–422.
Keats, D. W., Knight, M. A., and Pueschel, C. M. (1997). Antifouling effects of epithallial shedding in three crustose coralline algae (Rhodophyta, Coralinales) on a coral reef. J. Exp. Mar. Biol. Ecol. 213, 281–293. doi: 10.1016/S0022-0981(96)02771-2
Keats, D. W., Mathews, I., and Maneveldt, G. (1994). Competitive relationships and coexistence in a guild of crustose algae in eullitoral zone, Cape Province, South Africa. S. Afr. J. Bot. 60, 108–113. doi: 10.1016/S0254-6299(16)30640-8
Kendrick, G. A. (1991). Recruitment of coralline crust and filamentous turf algae in the Galapagos archipelago: effect of simulated scour, erosion and accretion. J. Exp. Mar. Biol. Ecol. 147, 47–63. doi: 10.1016/0022-0981(91)90036-V
Kikuchi, R. K. P., and Leão, Z. M. A. N. (1997). “Rocas (southwestern equatorial Atlantic, Brazil): an atol built primarily by coralline algae”in Proceedings Of The International Coral Reef Symposium, Panama, 731–736.
Leão, Z. M. A. N., and Dominguez, J. M. L. (2000). Tropical coast of Brazil. Mar. Pollut. Bull. 41, 112–122. doi: 10.1016/S0025-326X(00)00105-3
Leão, Z. M. A. N., and Kikuchi, R. K. P. (2001). The Abrolhos reefs of Brazil. Ecol. Stud. 144, 83–96. doi: 10.1007/978-3-662-04482-7_7
Lei, X., Huang, H., Lian, J., Zhou, G., and Jiang, L. (2018). Community structure of coralline algae and its relationship with environment in Sanya reefs, China. Aquat. Ecosyst. Health 21, 19–29. doi: 10.1080/14634988.2018.1432954
Leukart, P. (1994). Field and laboratory studies on depth dependence, seasonality and light requirement of growth in three species of crustose coralline algae (Rhodophyta, Corallinales). Phycologia 33, 281–290. doi: 10.2216/i0031-8884-33-4-281.1
Littler, M. M. (1973). The population and community structure of Hawaiian fringing-reef crustose corallinaceae (Rhodophyta, Cryptonemiales). J. Exp. Mar. Biol. Ecol. 11, 103–120. doi: 10.1016/0022-0981(73)90050-6
Littler, M. M., and Littler, D. S. (1980). The evolution of thallus form and survival strategies in benthic marine macroalgae: field and laboratory tests of a functional form model. Am. Nat. 116, 25–44. doi: 10.1086/283610
Littler, M. M., and Littler, D. S. (1984). Relationships between macroalgal functional form groups and substrata stability in a subtropical rocky intertidal system. J. Exp. Mar. Biol. Ecol. 74, 13–34. doi: 10.1016/0022-0981(84)90035-2
Littler, M. M., and Littler, D. S. (1997). “Disease-induced mass mortality of crustose coralline algae on coral reefs provides rationale for the conservation of herbivorous fish stocks,” in Proceedings of the Eighth International Coral Reefs Symposium, Panama, 719–724.
Littler, M. M., and Littler, D. S. (2000). Caribean Reef Plant. An Identification Guide to the Reef Plants of the Caribbean. Boca Raton, FL: Off Shore Grafics, Inc.
Littler, M. M., Littler, D. S., and Taylor, P. R. (1995). Selective herbivore increases biomass of its prey: a chiton-coralline reef-building association. Ecology 76, 1666–1681. doi: 10.2307/1938167
Lüning, K. (1990). Seaweeds. Their Environmental, Biogeography, and Ecophysiology. New York, NY: John Wiley and Sons.
Macintyre, I. G. (1997). “Reevaluating the role of crustose coralline algae in the construction of the coral reefs,” in Proceedings of the 8th International Coral Reef Symposium, Panama, 725–730.
Mariath, R., Riosmena-Rodriguez, R., and Figueiredo, M. A. O. (2013). Succession of crustose coralline red algae (Rhodophyta) on coralgal reefs exposed to physical disturbance in the southwest Atlantic. Helgol. Mar. Res. 67, 687–696. doi: 10.1007/s10152-013-0354-3
Masaki, T., Fujita, D., and Hagen, N. T. (1984). The surface ultrastructure and epithallium shedding of crustose coralline algae in an “isoyake” area of southwestern Hokkaido, Japan. Hydrobiologia 117, 219–223.
Matsuda, S. (1989). Succession and growth rates of incrusting crustose coralline algae (Rhodophyta, Cryptonemiales) in the upper fore-reef enviromental off Ishigaki Island, Ryukyu Islands. Coral Reefs 7, 185–195. doi: 10.1007/BF00301597
McClanahan, T. R., McField, M., Huitric, M., Bergman, K., Sala, E., Nyström, et al. (2001a). Responses of algae, corals and fish to the reduction of macroalgae in fished and unfished patch reefs of Glovers Reef Atoll, Belize. Coral Reefs 19, 367–379. doi: 10.1007/s003380000131
McClanahan, T. R., Muthinga, N. A., and Mangi, S. (2001b). Coral and algal changes after the 1998 coral bleching: interaction with reef management and herbivores on Kenyan reefs. Coral Reefs 19, 380–391. doi: 10.1007/s003380000133
Muehe, D. (1988). O arquipélago dos Abrolhos: geomorfologia e aspectos gerais. An. Inst. Geoc. 11, 90–100.
Norton, T. A. (1991). Conflicting constraints on the form of intertidal algae. Br. Phycol. J. 26, 203–218. doi: 10.1080/00071619100650191
Olson, A. M., and Lubchenco, J. (1990). Competition in seaweeds: linking plant traits to competitive outcomes. J. Phycol. 26, 1–6. doi: 10.1111/j.0022-3646.1990.00001.x
Pitlik, T. J., and Paul, V. J. (1997). “Effects of toughness, calcite level, and chemistry of crustose coralline algae (Rhodophyta, Corallinales) on grazing by the parrot fish Chorurus sordidus,” in Proceedings of the 8th International Coral Reef Symposium, Panama, 701–706.
Spotorno-Oliveira, P., Figueiredo, M. A. O., and Tâmega, F. T. S. (2015). Coralline algae enhance the settlement of the vermetid gastropod Dendropoma irregulare (d’Orbigny, 1842) in the southwestern Atlantic. J. Exp. Mar. Biol. Ecol. 471, 137–145. doi: 10.1016/j.jembe.2015.05.021
Steneck, R. S. (1983). Escalating herbivory and resulting adaptive trends in calcareous algal crusts. Paleobiology 9, 44–61. doi: 10.1017/S0094837300007375
Steneck, R. S. (1986). The ecology of coralline algal crusts: convergent patterns and adaptive strategies. Ann. Rev. Ecol. Syst. 17, 273–303. doi: 10.1146/annurev.es.17.110186.001421
Steneck, R. S. (1988). “Herbivore on coral reefs: a synthesis,” in Proceedings of the 8th International Coral Reef Symposium, Panama, 37–49.
Steneck, R. S. (1997). “Crustose corallines, other algal functional grups, herbivores and sediments: complex interactions along reef productivity gradients,” in Proceedings of the 8th International Coral Reef Symposium, Vol. 1, eds H. A. Lessios and I. G. Macintyre (Panama: Smithsonian Tropical Research Institute), 695–700.
Steneck, R. S., and Adey, W. H. (1976). The role of environment in control of morphology in Lithophyllum congestum, a Caribbean algal ridge builder. Bot. Mar. 19, 197–215. doi: 10.1515/botm.1976.19.4.197
Steneck, R. S., and Dethier, M. N. (1994). A functional group approach to the structure of algal-dominated communities. Oikos 69, 476–498. doi: 10.2307/3545860
Steneck, R. S., Hacker, S. D., and Dethier, M. N. (1991). Mechanisms of competitive dominance between crustose coralline algae: an herbivore-mediated competitive reversal. Ecology 72, 938–950. doi: 10.2307/1940595
Steneck, R. S., and Watling, L. E. (1982). Feeding capabilities and limitations of herbivorous molluscs: a functional group approach. Mar. Biol. 68, 299–319. doi: 10.1007/BF00409596
Tâmega, F. T. S., and Figueiredo, M. A. O. (2007). Distribution of crustose coralline algae (Corallinales, Rhodophyta) in the Abrolhos reefs, Bahia, Brazil. Rodriguésia 58, 941–947. doi: 10.1111/j.1529-8817.2007.00431.x
Tâmega, F. T. S., Figueiredo, M. A. O., Ferreira, C. E. L., and Bonaldo, R. M. (2016). Seaweed survival after consumption by the greenbeak parrotfish, Scarus trispinosus. Coral Reefs 35, 329–334. doi: 10.1007/s00338-015-1373-0
Tâmega, F. T. S., Riosmena-Rodriguez, R., Mariath, R., and Figueiredo, M. A. O. (2014). Nongeniculate coralline red algae (Rhodophyta: Corallinales) in coral reefs from Northeastern Brazil and a description of Neogoniolithon atlanticum sp. nov. Phytotaxa 190, 277–298. doi: 10.11646/phytotaxa.190.1.17
Thomas, M. L. H. (1988). “Photosynthesis and respiration of aquatic macroflora using the light and dark bottle oxygen method and dissolved oxygen analyzer,” in Experimental Phycology: a Laboratory Manual, eds C. S. Lobban, D. J. Chapman, and B. P. Kremer (Cambridge: Cambridge University Press), 64–77.
Underwood, A. J. (1997). Experiments in Ecology. Logical Design and Interpretation using Analysis of Variance. Cambridge: Cambridge University Press.
Vermeij, M. J. A., van der Heijden, R. A., Olthuis, J. G., Marhaver, K. L., Smith, J. E., and Visser, P. M. (2013). Survival and dispersal of turf algae and macroalgae consumed by herbivorous coral reef fishes. Oecologia 171, 417–425. doi: 10.1007/s00442-012-2436-3
Villaça, R., and Pitombo, F. B. (1997). Benthic communities of shallow-water reefs of Abrolhos, Brazil. Rev. Bras Oceanogr. 45, 35–43. doi: 10.1590/S1413-77391997000100004
Villas-Bôas, A. B., Figueiredo, M. A. O., and Villaça, R. C. (2005). Colonization and growth of crustose coralline algae (Corallinales Rhodophyta) on the Rocas Atoll. Braz. J. Ocean. 53, 147–156. doi: 10.1590/S1679-87592005000200005
Vroom, P. S., Page, K. N., Peyton, K. A., and Kukea-Shultz, J. K. (2005). Spatial heterogeneity of benthic community assemblages with an emphasis on reef algae at French frigate shoals, Northwestern Hawaiian Island. Coral Reefs 24, 574–581. doi: 10.1007/s00338-005-0028-y
Williams, I. D., and Polunin, N. V. C. (2001). Large-scale associations between macroalgal cover and grazer biomass on mid-depth reefs in the Caribbean. Coral Reefs 19, 358–366. doi: 10.1007/s003380000121
Keywords: reef habitats, irradiance levels, Abrolhos, Brazil, crustose coralline algae, growth rates, colonization, productivity
Citation: Tâmega FTS and Figueiredo MAO (2019) Colonization, Growth and Productivity of Crustose Coralline Algae in Sunlit Reefs in the Atlantic Southernmost Coral Reef. Front. Mar. Sci. 6:81. doi: 10.3389/fmars.2019.00081
Received: 05 October 2018; Accepted: 12 February 2019;
Published: 28 February 2019.
Edited by:
Nadine Schubert, Federal University of Santa Catarina, BrazilReviewed by:
Mirta Teichberg, Leibniz Centre for Tropical Marine Research (LG), GermanyBonnie Lewis, University of Glasgow, United Kingdom
Copyright © 2019 Tâmega and Figueiredo. This is an open-access article distributed under the terms of the Creative Commons Attribution License (CC BY). The use, distribution or reproduction in other forums is permitted, provided the original author(s) and the copyright owner(s) are credited and that the original publication in this journal is cited, in accordance with accepted academic practice. No use, distribution or reproduction is permitted which does not comply with these terms.
*Correspondence: Frederico T. S. Tâmega, fredtamega@gmail.com