- 1Palaeoceanology Unit, Faculty of Geosciences, University of Szczecin, Szczecin, Poland
- 2National Marine Fisheries Research Institute, Gdynia, Poland
Dreissena polymorpha and D. rostriformis bugensis are freshwater Ponto-Caspian bivalve species, at present widely distributed in Europe and North America. In the Szczecin Lagoon (a southern Baltic coastal lagoon), the quagga was recorded for the first time in 2014 and found to co-occur with the zebra mussel, a long-time resident of the Lagoon. As the two species are suspected of being competitors where they co-occur, their population dynamics was followed at a site the new immigrant was discovered (station ZS6, northern part of the Lagoon) by collecting monthly samples in 2015–2017. The abundance and biomass of the two congeners showed wide fluctuations, significant differences being recorded between months within a year and between years. The abundance and biomass proportions between the two congeners changed from an initial domination of the newcomer quagga until mid-2015 to a persistent domination of the zebra mussel throughout the remainder of the study period. Both the abundance and biomass of the two dreissenids showed a number of significant associations with environmental variables, notably with salinity, chlorophyll a content, and temperature. The co-occurrence of the two dreissenids in the Lagoon is discussed in the context of their invasion stage; it is concluded that while the quagga seems to have achieved the “outbreak” stage, the zebra mussel, an “accommodated” invader present prior to the quagga immigration, reverted to that stage.
Introduction
Co-occurrence of congeneric species, observed in many ecosystems, is of interest for both theoretical and practical reasons (Gotelli, 2000; Sfenthourakis et al., 2005; Veech, 2014). It is generally assumed that co-occurrence may lead to co-existence (HilleRisLambers et al., 2012) as a result of competitive interactions, whereby the sympatric occurrence of the two congeners is possible on account of their resource partitioning (Walter, 1991) both in space (spatial separation, e.g., Pigot and Tobias, 2013; the use of different food resources, e.g., Labropoulou and Eleftheriou, 1997) and in time (different timing of reproduction; e.g., Dettman et al., 2003). Co-existence of congeners is of a special importance when a non-indigenous species (a “newcomer”) appears in an area already occupied by a congener with an established population (the “resident”). The increasing rate of species migrations and biological invasions worldwide (Lockwood et al., 2007; Nentwig, 2008; Rilov and Crooks, 2009) present an opportunity to follow the course of events associated with immigration of one species to an area already colonized by a congener. Such an opportunity presented itself in 2014 when the quagga (Dreissena rostriformis bugensis Andrusov, 1897) was first recorded in the Szczecin Lagoon, a coastal water body at the southern Baltic Sea coast, which already supported a population of a congener, the zebra mussel [D. polymorpha (Pallas, 1771)] (Woźniczka et al., 2016).
The two dreissenids are freshwater Ponto-Caspian bivalves of a considerable invasive potential (Nalepa and Schloesser, 1993; Karatayev et al., 1997, 2014a; Nalepa and Schloesser, 2014), which have colonized European and North American waters (Zhulidov et al., 2004, 2010; Karatayev et al., 2014a, 2015). The zebra mussel expansion in Europe began at the turn of the 18th century (Thienemann, 1950), much earlier than the onset of the D. rostriformis bugensis spread in the 1940s (e.g., Orlova et al., 2004; Popa and Popa, 2006; Molloy et al., 2007; Bij de Vaate, 2010; Stańczykowska et al., 2010; Zhulidov et al., 2010; Heiler et al., 2013; Matthews et al., 2014). In the Szczecin Lagoon, D. polymorpha has been present since the time regular biological observations in the area began in the 19th century. Despite its non-indigenous species status (Olenin et al., 2017), the zebra mussel has become a permanent and abundant component of the Lagoon’s biota (Wolnomiejski and Witek, 2013). When the quagga was first recorded in the Lagoon, it was already abundant and co-occurring with D. polymorpha (Woźniczka et al., 2016).
Numerous literature data from Europe and North America demonstrate that the sudden appearance of D. rostriformis bugensis, like previously that of D. polymorpha, can lead to ecosystem alteration and changes in indigenous communities (Karatayev et al., 1997; Ricciardi and MacIsaac, 2000; Nalepa et al., 2010). Both in North America and in its native area, it took D. rostriformis bugensis a relatively short time (5–10 years) to displace D. polymorpha (e.g., Mills et al., 1996; Orlova et al., 2004; Ricciardi and Whoriskey, 2004; Karatayev et al., 2015). The zebra mussel’s replacement by D. rostriformis bugensis in the Great Lakes was explained by invoking a number of possible mechanisms. One involves genetic adaptations of D. rostriformis bugensis to colonize new areas, which makes it possible for the species to migrate from deeper cooler waters to shallow areas (Mills et al., 1996; Karatayev et al., 2015). It has also been suggested that reproduction of D. rostriformis bugensis begins at temperatures lower than those needed by D. polymorpha, that is earlier in the season, which facilitates the former species’ success in the “race” to colonize suitable areas (Roe and MacIsaac, 1997; Claxton and Mackie, 1998). Still other hypotheses refer to differences between the two species in their filtration rates, feeding and energy efficiency. According to Diggins (2001), the filtration rate of D. rostriformis bugensis is higher than that of D. polymorpha, which is advantageous for the former, particularly under limited food resources. In addition, the energy efficiency of D. rostriformis bugensis is higher than that of D. polymorpha, resulting in a higher growth rate at a high food supply (Baldwin et al., 2002; Stoeckmann, 2003). Is it then likely that the zebra mussel, an “old” immigrant and the resident in the Szczecin Lagoon, might be replaced by D. rostriformis bugensis?
To assess (and possibly predict) effects of species, particularly immigrants, upon communities and ecosystems, the functional approach is regarded as especially valuable at present (Flynn et al., 2011; Karatayev et al., 2015). Functionally, the two congeners are considered interchangeable in terms of their community or ecosystem effects (Keller et al., 2007; Ward and Ricciardi, 2007; Higgins and Vander Zanden, 2010; Kelley et al., 2010; Karatayev et al., 2015). They are fairly similar in their life traits (e.g., a high reproductive potential, free-swimming planktonic larvae, sessile mode of life effected by byssus attachment to the substrate, efficient filtration) (Karatayev et al., 2015). Consequently, they are successful colonizers and invaders, and may reach a dominant status in a newly settled area. On the one hand, they are perceived as representing the most invasive freshwater species in the northern hemisphere (Nalepa and Schloesser, 1993, 2014; Karatayev et al., 2014a, 2015). On the other, the dreissenid presence in water bodies is ecologically important as the bivalves, on account of their mode of life and suspension feeding, contribute significantly to the functioning of aquatic ecosystems (e.g., Fréchette and Bourget, 1985; Stańczykowska and Planter, 1985; Smit et al., 1993; Stewart et al., 1998; Daunys et al., 2006; Radziejewska et al., 2009; Nalepa and Schloesser, 2014; Karatayev et al., 2015). Both dreissenids are the so-called ecosystem engineers as, at a mass occurrence, their aggregations form complex three-dimensional structures consisting of both live individuals and empty shells, generating microhabitats and enhancing biodiversity of the associated sessile and motile benthos (Karatayev et al., 2002; Vanderploeg et al., 2002; Gutierrez et al., 2003; Hecky et al., 2004; Zaiko et al., 2009). The bivalves’ filter-feeding reduces the eutrophication symptoms by eliminating suspended particulates from the water column, whereas their feces and pseudofaeces deposited on the bottom supply organic matter to the sediment and boost microbial processes in it (Izvekova and Lvova-Katchanova, 1972; Stewart et al., 1998, 1999; Bially and MacIsaac, 2000; Ward and Ricciardi, 2007; Stańczykowska et al., 2010).
Although the two species are similar, they are by no means identical. To understand their ecology and effects on ecosystems they live or have appeared in, it is necessary to obtain insights into their distribution and population dynamics (Karatayev et al., 2015). Therefore, as an initial step toward exploring effects of the new immigrant on its resident congener and on the recipient ecosystem in general, this study focused on following the dynamics of the two dreissenid populations in the Lagoon in terms of their abundance and biomass from the time point when the new immigrant was first recorded in the Lagoon.
Materials and Methods
Area of Study
The Szczecin Lagoon (Figure 1), one of the three southern Baltic Sea lagoons, is a major part of the Odra River estuarine system (Wolnomiejski and Witek, 2013). It is divided into two sub-basins, the Kleines Haff in the west (in Germany) and the Wielki Zalew (“Great Lagoon”) in the east (in Poland). From the south, the Lagoon is fed by the Odra River; in the north, it is connected – via three straits (the Peene, Świna and Dziwna) – with the Pomeranian Bay in the Baltic Sea. The Lagoon is a brackish transitional water body the hydrography of which is shaped by the interplay of riverine runoff from the Odra and periodic intrusions of the seawater from the Pomeranian Bay. As a result, the Lagoon’s salinity changes in time and space (in the south–north direction) from PSU 0.3 to 4.5 (Radziejewska and Schernewski, 2008). With its mean depth of 3.8 m, the Lagoon is a shallow water body; the maximum natural depth is 8.5 m, whereas the artificially dredged navigation channel leading to the port of Szczecin is 10.5 m deep (Radziejewska and Schernewski, 2008). The central part of the Wielki Zalew is surrounded by a belt of sandy shallows (1–1.5 m depth) which extend from the shores into the Lagoon; steep slopes of the shallows descend to the muddy areas of the central basin (Wolnomiejski and Witek, 2013). Both the shallows and their slopes have been known to support dense aggregations of D. polymorpha (Wiktor, 1969; Wolnomiejski and Witek, 2013) and – as it turned out in 2014 – also mixed aggregations of D. polymorpha and D. rostriformis bugensis (Woźniczka et al., 2016).
The Szczecin Lagoon has been for years subjected to a heavy anthropogenic pressure, with all the consequences, including pronounced eutrophication (Radziejewska and Schernewski, 2008). It is also used as a busy shipping area, with navigation lanes leading to the large Baltic Sea ports of Świnoujście and Szczecin. The estuarine system of the Lagoon is also connected – via the Odra and a network of canals – with the European waterways, including the catchments of the Vistula and Elbe, and indirectly with those of the Rhein and Danube. The location of the Lagoon and the busy ship’s traffic has resulted in a fairly high contribution of non-indigenous species to the Lagoon’s biota (Gruszka, 1999; Wawrzyniak-Wydrowska and Gruszka, 2005; Radziejewska and Schernewski, 2008; Wolnomiejski and Witek, 2013), the estuary itself constituting potentially a source area for further spread of those species in the Baltic Sea catchment (Gruszka, 1999).
Following the first record of D. rostriformis bugensis in November 2014, the two dreissenids were sampled monthly (March to November or December) in 2015, 2016, and 2017 at station ZS6 in the northern part of the Lagoon (Figure 1). Due to water level changes in the Lagoon, the station depth varied between 3 and 4.5 m.
Sampling
The bivalves were sampled with a 625 cm2 Van Veen grab, three samples being collected at each site on each sampling occasion. The three samples were sieved individually on an 0.5 mm mesh size sieve and the sieving residue was fixed with buffered 10% formalin. The samples were then sorted, the bivalves were identified and counted, and subsequently dried for 12 h at 105°C and weighed to determine the dry-weight based biomass.
Synoptically with dreissenid sampling, environmental variables of the water column (temperature, salinity, pH, dissolved oxygen content, chlorophyll a content) and the sediment (silt/clay and organic matter content) were determined; these measurements are summarized in Table 1.
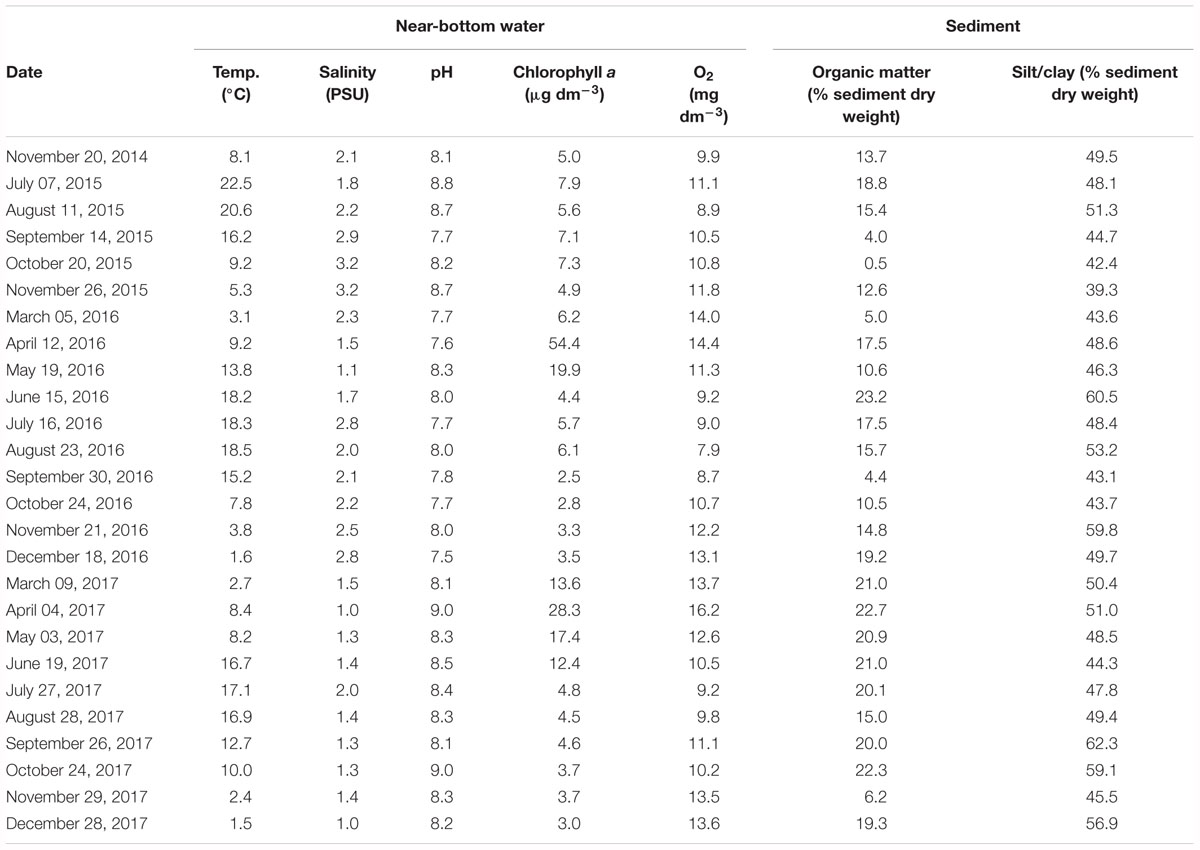
Table 1. Sampling dates and characteristics of the near-bottom water layer (temperature, salinity, pH, chlorophyll a content, dissolved oxygen content) and sediment (organic matter and silt/clay contents) at the Szczecin Lagoon station ZS6 sampled in 2014–2017.
The near-bottom (0.5 m above the bottom) water temperature, salinity, pH, dissolved oxygen content, and chlorophyll a content (a measure of the quantity of biogenic suspended particulates and a proxy of phytoplankton biomass) were measured with an ENDECO YSI 6600 device equipped with appropriate sensors. To characterize the sediment, the silt/clay fraction (a MALVERN 300 laser grain size analyser, 0.3–200 μm) and the organic matter content (loss on ignition at 550°C; Bale and Kenny, 2005) in a sediment sample collected separately with the Van Veen grab were determined.
Statistical Treatment
The near-bottom water characteristics (temperature, salinity, pH, chlorophyll a content, dissolved oxygen content) were processed with the Principal Components Analysis (PCA) to identify those variables which accounted for most of the variation in the data set. The PCA was run, on log-transformed and normalized values, using the Statistica v. 12 software (Statistica, Poland).
Differences in the two dreissenids’ population metrics of interest for this study (abundance and biomass) were assessed using the three-level nested ANOVA for uneven groups. The first level (group effect) involved the abundance or biomass of a species, and the null hypothesis tested was that the mean abundances or biomasses did not differ significantly between the species. The second level (sub-group effects) tested the null hypothesis of there being no significant differences in mean abundance or biomass between the years within species. The third level (sub-sub-group effects) tested the null hypothesis of no significant differences in mean abundance or biomass between months within a year. The analyses were run, using the algorithm available at http://www.biostathandbook.com/nestedanova.html (McDonald, 2014) on log(x+1)-transformed abundance and biomass data.
Relationships between the bivalve abundance or biomass, as the dependent variables, and the near-bottom water characteristics mentioned before, as the independent or explaining variables, were explored using the multiple regression analysis (Stanisz, 2007). When some of the variables were co-linear or non-significant, step-wise regression (forward or backward) was applied. The forward step-wise regression involves gradual adding, to the list of the explaining variables, of those variables which affect the dependent variable the most. The procedure was repeated until the best model was obtained. The backward step-wise regression involves gradual removal from the model, consisting of all the potential variables, of those variables which, at a given step, were the least important for the dependent variable, until the best model is obtained (Stanisz, 2007). The regression was run for t0 (environmental data collected synoptically to bivalve sampling) and for t-1 (environmental data preceding the actual abundance or biomass value by a time lag of 1 month). The analyses were conducted with the Statistica v. 12 software (Statistica, Poland).
Results
Environment
The near-bottom water temperature (Table 1) in each year varied seasonally, from 1.5°C in December 2017 to 22.5°C in July 2015. A between-year comparison showed each season of 2015 to be warmer than the respective seasons of 2016 and 2017.
The near-bottom water salinity (Table 1) ranged from PSU 1.0 in April and December 2017 to PSU 3.2 in October and November 2015. In 2015, salinities were somewhat higher than in other years. Noteworthy were markedly reduced salinities at ZS6 in 2016, even in the autumn (PSU 1–1.4) when northerly and north-westerly winds, usually prevalent at that time, are known to enhance seawater intrusions into the Lagoon (Meyer and Lampe, 1999; Bangel et al., 2004).
The near-bottom water pH (Table 1) ranged from 7.5 in December 2016 to a maximum of 9.0 recorded in October 2017.
The near-bottom chlorophyll a content was found to range from about 2.5 (September 2016) to 54.4 μg dm-3 (April 2016) and varied markedly in time (between months and years) (Table 1). Regarding the temporal variability (between-months differences), a similar general pattern was observed in 2016 and 2017 (the measurements in both years were taken from March until December), with a pronounced peak in spring (April and May). The chlorophyll a content in April 2016, however, was almost twice that in April 2017. In addition, much higher chlorophyll a contents were recorded in individual months of 2015, compared to the respective records in other years.
The near-bottom mean dissolved oxygen contents (Table 1) varied from 7.9 (August 2016) to 14.4 mg dm-3 (April 2016). No instances of oxygen deficiency were recorded, although the contents observed in August 2016 were fairly low, compared to the oxygen situation at other times.
The sediment showed a very high silt/clay content, from 39.3% in November 2015 to 62.3% in September 2017 (Table 1), and contained some admixture of fine sand. The sediment was usually strongly enriched in organic matter (from 0.5% in October 2015 to 23.2% in June 2016) (Table 1), the enrichment being dependent on sedimentation of organic material from the water column (Radziejewska et al., in prep.) and hence a distinct between-month variation. The enrichment was noticeably higher in 2017 than in the two previous years.
The PCA of the environmental variables (Figure 2) showed the characteristics of the water column in its near-bottom part to change primarily in time. The sampling events were grouped mainly along the principal component 1 (PC1), and a separation between “warm” (June, July, and September, on the right-hand side of PC1) and “cold” (April, March, October, and November on the left) months is fairly distinct in the plot. PC1, which accounted for 50.4% of the total variation in the data, combined the near-bottom water temperature and the dissolved oxygen content. The second principal component (PC2), accounting for 22.7% of variation in the data, combined salinity, pH and the chlorophyll a content, and separated the sampling events temporally as well, with the “warm” months being grouped above the main axis and the “cold” ones below it.
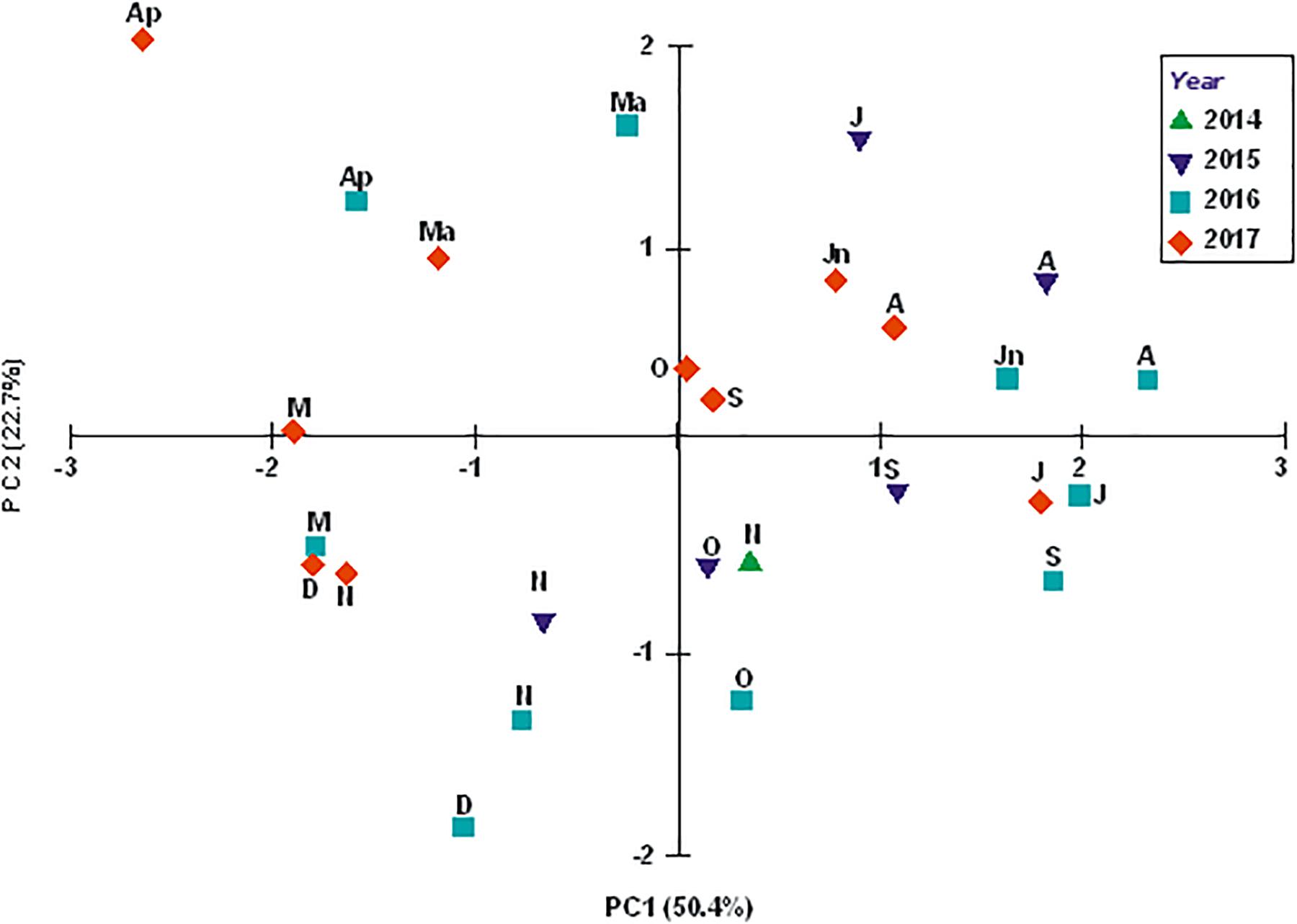
Figure 2. Environmental variables-based PCA plot for sampling events (months of 2014, 2015, 2016, and 2017) at station ZS6. Letters next to symbols denote months (M, March; Ap, April; Ma, May; Jn, June; J, July; A, August; S, September; O, October; N, November; and D, December).
Dreissenid Abundance
The mean abundance of D. polymorpha at ZS6 was found to range from 1573.3 ± 351.39 (September 2015) to 101642.7 ± 9192.73 ind. m-2 (October 2016) (Supplementary Table S1). After a period of rather low abundances lasting until July 2016, a boom was observed in August–September, followed by a decline resulting in abundances being fairly leveled-out, albeit at a level much higher than that observed prior to August 2016, throughout 2017.
The mean abundance of D. rostriformis bugensis was found to range from 3141.3 ± 1719.71 ind. m-2 (July 2017) to 26869.3 ± 2644.91 ind. m-2 (August 2015) (Supplementary Table S1). Like those of the zebra mussel, the quagga abundance fluctuated considerably over time (Figure 3), the fluctuations being particularly dynamic in 2015–2016. The quagga population peaked in August 2015 and again in May 2016, to decline thereafter down to fairly low levels maintained throughout 2017.
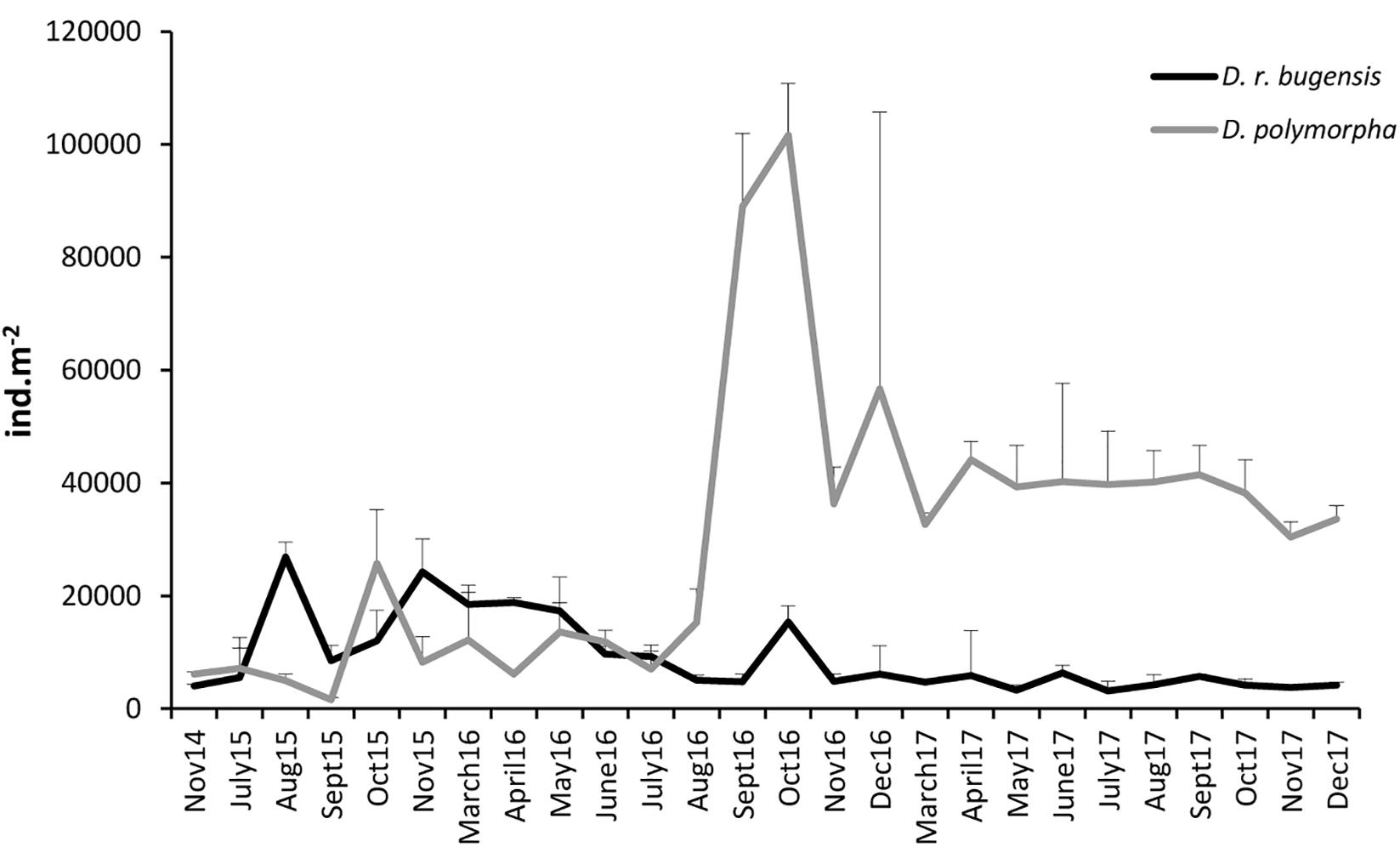
Figure 3. Changes in mean abundances of D. polymorpha and D. r. bugensis in 2014–2017 at station ZS6; error bars denote standard deviations.
When analyzed together, the abundances of the two dreissenids at ZS6 were observed to change out of synchrony (correlation coefficient r = -0.15) (Figure 3), with peak abundances coinciding (albeit at very different levels) only in October 2016. Initially (from August 2015 until July 2016), the quagga was a dominant congener (the abundance ratio of ca. 6:4 in favor of D. rostriformis bugensis), but as of August 2016 the zebra mussel took over, its abundances being several times higher than those of the quagga.
Differences in abundance between the two species proved non-significant (group effect, p > 0.05) (Table 2). On the other hand, differences in abundance between years in each species (sub-group effect) proved highly significant (p < 0.01), very highly significant differences (p < 0.001) being observed between months in each year (Table 2).
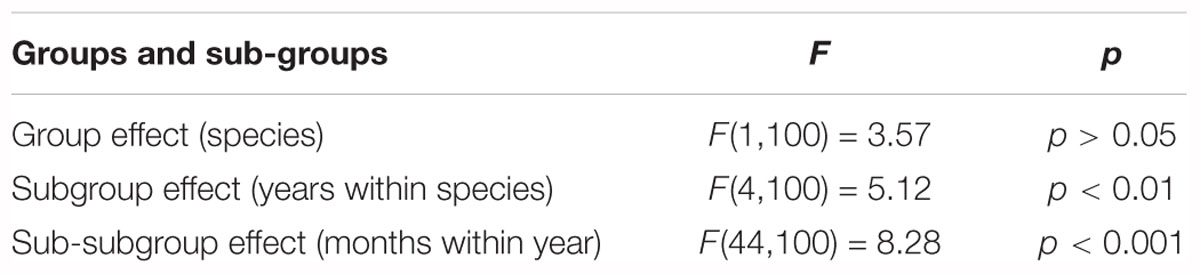
Table 2. Three-level nested ANOVA (among-species difference as the group effect) for abundances of D. polymorpha and D. rostriformis bugensis.
Dreissenid Abundance Versus Environmental Variables
The regression analysis for dreissenid abundance at t0 and t-1 (abundance time-lagged by 1 month relative to records of environmental characteristics) (Table 3) showed significant associations (p < 0.05; p < 0.001) for D. polymorpha. In 2015, the significant variable was the chlorophyll a content at t0 (a very high coefficient of determination, 0.92; model p < 0.05), no significant associations being found for t-1. In 2016, the zebra mussel abundance showed to be significantly related to temperature; however, the model with one variable explained as little as 45% of the variation in the abundance. No significant association was found for t-1. In 2017, the significant variables explaining 89% (p < 0.001) of the variability in abundance were the temperature and salinity. The 2017 time-lagged model included salinity and chlorophyll a which explained 68% (p < 0.05) of the variability in abundance.
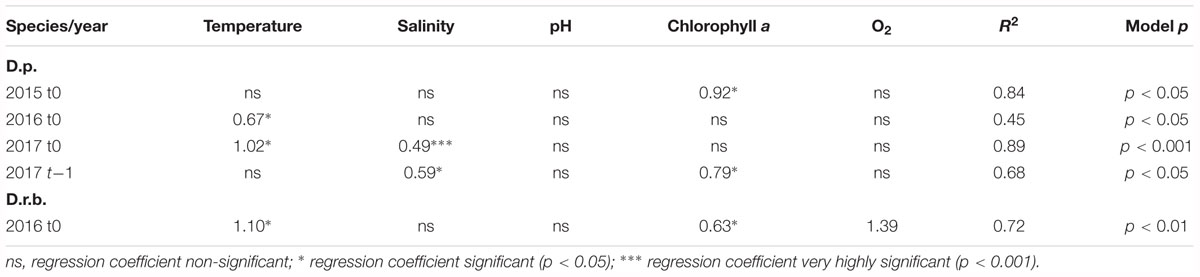
Table 3. Multiple regression analysis for relationships between dreissenid abundances (D.p., D. polymorpha; D.r.b., D. rostriformis bugensis) and environmental variables (at t0 and t-1) in the near-bottom water layer; significant regression coefficients shown only.
The D. rostriformis bugensis abundance at ZS6 showed significant associations with environmental variables in 2016 only, the significant variables including the near-bottom water temperature, chlorophyll a and dissolved oxygen contents which, taken together, explained 72% (p < 0.01) of the variability in abundance at t0.
Dreissenid Biomass
The mean dry weight biomass of D. polymorpha at ZS6 was found to range from 123.4 ± 55.30 (April 2016) to 2603.3 ± 504.91 g m-2 (July 2017) (Figure 4). Like the abundances, the biomass fluctuated extensively throughout the period of study (Figure 4): after a biomass peak in October 2015, a steep decline was observed and a low biomass prevailed until September 2016 when the biomass started to increase steadily until the summer of 2017 (July–August).
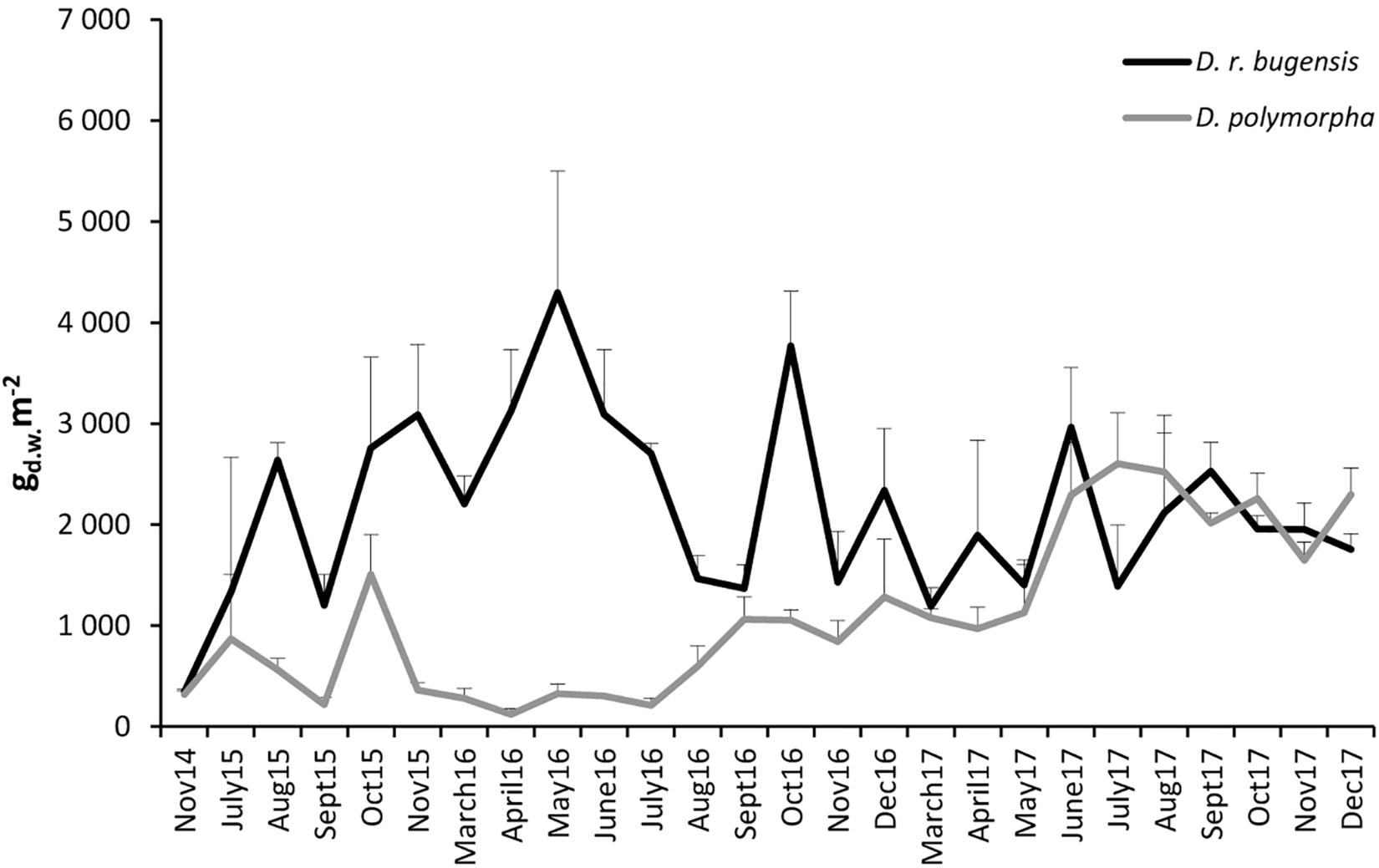
Figure 4. Changes in mean biomasses of D. polymorpha and D. r. bugensis in 2014–2017 at station ZS6; error bars denote standard deviations.
The mean dry weight biomass of D. rostriformis bugensis was found to range from 345.6 ± 7240 (November 2014) to 4297.8 ± 1202.20 g m-2 (May 2016) (Figure 4). Like those of the zebra mussel, the quagga biomass fluctuated during the period of study (Figure 4), with pronounced peaks in May 2016, October 2016, and June 2017.
The biomasses of the two congeners at ZS6 were observed to follow different paths of variation, without any synchrony (correlation coefficient r = -0.09) (Figure 4). The quagga biomasses were higher (initially very much so) than the biomass of the zebra mussel until May 2017, when the two congeners – notwithstanding some differences, particularly in July – showed fairly similar biomasses.
Like in the case of abundance, between-species changes in biomass proved non-significant (group effect, p > 0.05) (Table 4). On the other hand, each species showed very highly significant biomass differences between years (sub-group effect; p < 0.001) and between months within a year (sub-subgroup effect; p < 0.001) (Table 4).
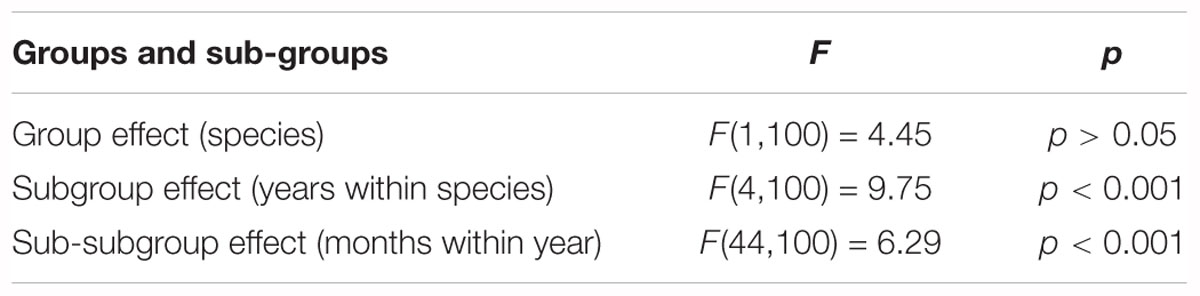
Table 4. Three-level nested ANOVA (among-species difference as the group effect) for biomasses of D. polymorpha and D. rostriformis bugensis.
Dreissenid Biomass Versus Environmental Variables
The regression analysis for dreissenid biomass at t0 and t-1 (biomass time-lagged by 1 month relative to records of environmental characteristics) (Table 3) showed no significant associations between the D. polymorpha biomass and environmental variables in 2015. In 2016 and 2017, the near-bottom water temperature, chlorophyll a and dissolved oxygen contents at t0 were found to account jointly for 70 (p < 0.05) and 89% (p < 0.001) of the biomass variation, respectively.
The D. rostriformis bugensis biomass, like that of D. polymorpha, showed significant associations with environmental variables in 2016 and 2017 only. The time-lagged biomass in 2016 was found to be significantly related to the dissolved oxygen content (47% of the variability explained), the near-bottom temperature being a significant variable at t0 in 2017 (57%, p < 0.05).
Discussion
Dreissenid Abundance and Biomass
The abundances and biomasses of both dreissenids were found to fluctuate extensively. Such wide fluctuations seem to be well known from dreissenid populations worldwide (Strayer and Malcom, 2006; Stańczykowska et al., 2010) and are typical of sessile bivalve populations in general (e.g., Ardisson and Bourget, 1991). In the Szczecin Lagoon, the zebra mussel population abundances and biomass have been observed to fluctuate since the time when observations of the population in the Lagoon began (the early 1950s) (Wiktor, 1962, 1969). In the 1950s–1960s, the zebra mussel was a very abundant component of the Szczecin Lagoon biota, the abundances being estimated at more than 110 thou. ind. m-2 (Wiktor, 1962, 1969). In the 1980s, the D. polymorpha abundance in the Lagoon was reported to decline, and the population was even thought to have disappeared from the area (Piesik, 1992; Masłowski, 1993). The population decline at that time was associated with increased anthropogenic pressure, primarily the progressing eutrophication (Piesik, 1992). However, surveys conducted in 2000–2004 (Woźniczka and Wolnomiejski, 2005) showed D. polymorpha to be still an abundant component of the Lagoon’s biota and to occur primarily on the slopes of shallows (depth range of 2.5–4.5 m) with average abundances of several thousand ind. m-2. And yet, the 2005 survey (Wolnomiejski and Woźniczka, 2008) revealed a distinct (even fourfold) decline in the Lagoon’s zebra mussel population. The authors quoted ascribed the decline to the habitat’s trophic capacity being exceeded due to excessive growth of the population in the preceding years, which resulted in the food resources being depleted by intensive filtration of the large population. This “population boom and bust” is fairly common in various sessile populations (Strayer and Malcom, 2006). Considerable fluctuations in the D. polymorpha abundance were reported from the Kleines Haff (the eastern part of the Szczecin Lagoon), too: Fenske et al. (2010) found that, between 1993 and 2007, the zebra mussel abundance and biomass declined and the area occupied by the bivalves shrank. And yet, Stańczykowska et al. (2010) regarded the D. polymorpha population in the Lagoon as “stable,” i.e., persistent, the fluctuations of abundance notwithstanding. Even with large differences from 1 year to the next, the fluctuations are, in the opinion of Stańczykowska et al. (2010), typical of eutrophic areas. The short-term instability, such as observed in the abundances of D. polymorpha in this study (3 years of observations) could then be a part of the normal longer-term pattern of variability in the area.
Changes in abundance and biomass of D. rostriformis bugensis have been studied much less extensively than those of the zebra mussel. Nevertheless, a large variability has been reported as well, ascribed (particularly with regard to the abundance) to an invasion stage and the depth of the bivalve’s occurrence in a reservoir (Mills et al., 1996; Zhulidov et al., 2004; Jones and Ricciardi, 2005; Nalepa et al., 2009, 2010; Wong et al., 2012).
Fluctuations in the abundance of sessile bivalve populations, including those of dreissenids, have been explained by invoking effects of internal and/or external drivers. The former involve primarily recruitment and mortality (e.g., Cockrell et al., 2015; Polsenaere et al., 2017) and intra-specific competition (e.g., Naddafi et al., 2010; D’Hont et al., 2018) as mechanisms of population regulation (Krebs, 1995). In the D. polymorpha population studied here, recruitment might have played a role, as the abundance peaks coincided with the appearance of new cohorts, visible as increased numbers of small (<8 mm) individuals in the population (Wawrzyniak-Wydrowska et al., in prep.). However, the timing of the new individuals’ appearance differed between years, as the peaks occurred in different months (cf. Figure 3).
External drivers include abiotic environmental factors and biotic pressures. The former, at least the suite of environmental factors analyzed in this study, did not produce a uniform pattern of responses: abundance correlated with different parameters each year, and the biomass was significantly related to the near-bottom water temperature, chlorophyll a content, and dissolved oxygen content (cf. Tables 3, 5).
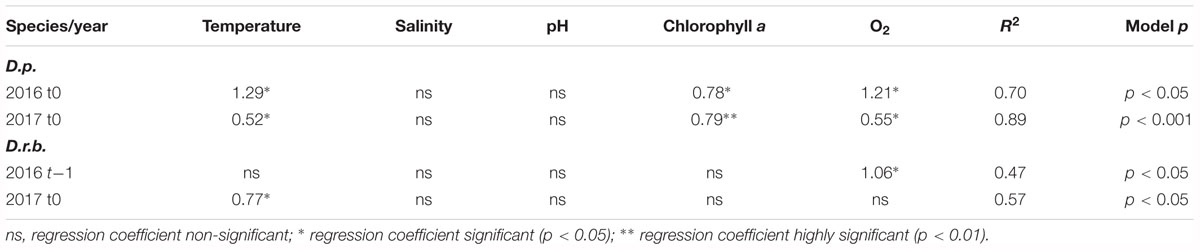
Table 5. Multiple regression analysis for relationships between dreissenid biomasses (D.p., D. polymorpha; D.r.b., D. rostriformis bugensis) and environmental variables (at t0 and t-1) in the near-bottom water layer; significant regression coefficients shown only.
Certain associations inferred from results of the multiple regression analysis (cf. Tables 3, 5) are amenable to a more in-depth exploration. For example, in 2015 and 2017, the zebra mussel abundance produced significant relationships with chlorophyll a, a proxy of phytoplankton biomass and the trophic status of a water body. As shown in a number of studies (Dorgelo, 1993; Sprung, 1995a,b; Schneider et al., 1998), the trophic status as well as the quality and density of the bioseston (which includes the phytoplankton) strongly affects the growth rate of D. polymorpha, the bivalves growing better under eutrophic than meso-oligotrophic conditions, and Jantz and Neumann (1992) reported a highly significant correlation between the zebra mussel growth rate and the chlorophyll a content. As suspension feeders, the two dreissenids rely on ambient phytoplankton for food. In a eutrophic water body such as the Szczecin Lagoon, the phytoplankton densities are usually high (Wolnomiejski and Witek, 2013), so the filter feeders should not experience food limitation. However, the phytoplankton in the Lagoon frequently features the presence of cyanobacteria, and cyanobacteria blooms of different intensity are a regularly observed effect. It may be conceivable that the presence of cyanobacteria limits the availability of phytoplankton as food for both dreissenids. Bierman et al. (2005) demonstrated selective rejection of cyanobacteria from the phytoplankton food ingested by the zebra mussel, while Fernald et al. (2007) found high cyanobacterial biomasses to be associated with low zebra mussel filtration rate (and hence a weakened feeding ability). Paradoxically, the presence of the zebra mussel may even promote cyanobacteria (Nogaro and Steinman, 2013; Gaskill and Woller-Skar, 2018). As demonstrated by Boegehold et al. (2018) in a laboratory study, reproduction potential, spawning and fertilization success of the quagga was greatly reduced in the presence of cyanobacteria. Although no effects of cyanobacteria on in situ reproduction of invasive dreissenids have been identified yet, there is a possibility of reproductive consequences for wild populations.
Salinity emerged as another environmental factor producing significant associations with the abundance of D. polymorpha (cf. Table 3). Although the two species show a low salinity tolerance, D. polymorpha is somewhat more tolerant (up to above PSU 6) than D. rostriformis bugensis (<PSU 5) (Karatayev et al., 1998, 2014a; Garton et al., 2014), hence a significant association of the factor with the zebra mussel abundance.
The near-bottom water temperature was still another environmental variable that could be associated with fluctuations in the two species’ abundances and biomass. Generally, D. polymorpha has a wider temperature tolerance than D. rostriformis bugensis, with the upper limit being reported as 33 and 31°C, respectively, the lower limit being set at 0°C for both species (McMahon, 1996; Karatayev et al., 1998, 2014a). The temperature range recorded in the Lagoon during the period of study (1.5–22.5°C) would hardly be limiting for either species. The temperature could, however, affect the timing of the onset of reproduction and larval settlement (Karatayev et al., 1998, 2014a; Garton et al., 2014). The associations between the dreissenids’ abundances and biomass, produced by the multiple regression, were, however, weaker than those involving the salinity and chlorophyll a content.
The dissolved oxygen content in the Szczecin Lagoon does not seem to be a factor limiting the dreissenid occurrence in the area, as the near-bottom water was generally well-oxygenated throughout the period of study (cf. Table 1). The water column in the Lagoon, a shallow reservoir, is usually well-mixed, and hypoxia has been occasional only (Wolnomiejski and Witek, 2013). As the multiple regression analysis identified dissolved oxygen content to form significant associations with the dreissenid abundance/biomass in 2016 and 2017, it is possible that the association was a result of the lower-than-usual oxygen contents recorded in August 2016 at ZS6 (cf. Table 1).
Among the external biotic pressures affecting the dreissenid populations, predation by waterfowl and fish (Mörtl et al., 2010; Wong et al., 2013) and parasitic infestations (Molloy et al., 1997; Karatayev et al., 2000; Mastitsky and Gagarin, 2004; Tyutin, 2005) have been most frequently referred to. The zebra mussel and quagga individuals sampled in the Lagoon were found to be parasite-free (Żbikowska, pers. comm.). On the other hand, the waterfowl predation could be an important factor affecting the population size and inducing its fluctuations. The zebra mussel in the Lagoon is known to be a food resource, particularly in winter, for the tufted duck (Aythya fuligula), the coot (Fulica atra) and the greater scaup (Aythya marila) (Piesik, 1974, 1983; Fenske et al., 2013; Marchowski et al., 2015), all abundant in the area. The fish predators include the roach (Rutilus rutilus), white bream (Blicca bjoerkna), vimba (Vimba vimba). and the invasive round goby (Neogobius melanostomus) (Kublickas, 1959; Fenske et al., 2013), a species that has already formed a local reproducing population in the River Odra estuary (Czugała and Woźniczka, 2010). The evidence from other areas (Naddafi and Rudstam, 2014; Foley et al., 2017) indicates, however, that the round goby predation, preferential with respect to the quagga where the bivalve’s abundance has exceeded that of the zebra mussel, seems to affect the population demography (size structure) rather than the abundance. Other zebra mussel predators in the Lagoon include the crayfish Orconectes limosus and the Chinese mitten crab (Eriocheir sinensis) (Fenske et al., 2013). On the other hand, as reported from the Dutch lakes IJsselmeer and Markermeer, even where the two congeners co-occur, the quagga is selectively preyed upon by the wintering tufted duck, scaup, pochard and goldeneye, and is their main food item in the area (Noordhuis et al., 2010; Van Eerden and De Leeuw, 2010). However, no estimates of predation impact on either dreissenid in the Lagoon are available as yet.
Predation on dreissenid larvae may be another external biotic driver affecting the population size and inducing fluctuations in both abundance and biomass. Dreissenid larvae are known to be ingested by large planktonic copepods (e.g., Liebig and Vanderploeg, 1995) as well as by the conspecific adult bivalves (e.g., MacIsaac et al., 1991). The lack of data on this mechanism of population regulation underscores the importance of long-term observations on invasive species (Lucy, 2006).
Another line of reasoning with which to seek the underpinnings of wide fluctuations in the population size of new immigrants/invaders invokes generalizations concerning stages of invasion. Based on 12 years of observations of the zebra mussel in Ireland, Zaiko et al. (2014) developed a generalized scheme of invasion progression, whereby the arrival of an invader is followed by its establishment, expansion, outbreak (with sub-stages of late expansion, peak abundance, and early decline), and eventually accommodation; they provided also direct (semi-quantitative) and indirect (qualitative) characteristics of each stage. Zaiko et al. (2014), like Strayer and Malcom (2006), stressed the importance of long-term observations for an appropriate assessment of invader population fluctuations. Following this line of reasoning and referring to older data (Wiktor, 1962, 1969; Piesik, 1992; Masłowski, 1993; Woźniczka and Wolnomiejski, 2005; Wolnomiejski and Woźniczka, 2008), it may be assumed that D. polymorpha had reached the accommodation phase in the Lagoon prior to the appearance of D. rostriformis bugensis. It is likely that immigration of the quagga has perturbed the zebra mussel accommodation, as abundance fluctuations as well as certain demographic traits (Wawrzyniak-Wydrowska et al., in prep.) and asynchrony between changes in abundance and biomass point to the population reverting periodically to various sub-stages of the outbreak. According to the classification of Zaiko et al. (2014), the stage of the D. rostriformis bugensis invasion in the Lagoon can be regarded as the outbreak, with its sub-stages (late expansion, peak abundance, and decline) detectable throughout the study. It will be interesting to follow further developments in the status of the two populations in the lagoon, to find out, inter alia, whether the pattern known from other areas where longer-term observations have been made (Zaiko et al., 2014; D’Hont et al., 2018) would be repeated in the Lagoon.
“New” Versus “Old” Immigrant
For an immigration of a non-indigenous species to be successful in a new area, that species has to survive there, establish a population and persist (Olenin et al., 2017). This may be difficult when the area has already been colonized by a congener. Species within the same genera are usually similar to each other in many respects (Brionez-Fourzán, 2014); for example, they share a trophic level, for which reason competitive exclusion is expected to take place (Scott, 2009). However, closely related congeners do co-occur in many systems, which is evidently the case of the two dreissenids in the Szczecin Lagoon. Since it was first revealed in the Lagoon in 2014 (Woźniczka et al., 2016), D. rostriformis bugensis has apparently fulfilled the three conditions of success spelled out above: the species survived in the Lagoon, established a population (with sub-populations sampled in this study), and seems to have persisted. Thus, it presents an interesting case of co-occurring, at a local scale, with a congener representing the same trophic level. The emerging question is whether the two congeners will co-exist in the Lagoon. As stated by Chesson (2000), the essential criterion for coexistence is the “invasibility”; this criterion requires that a species be able to increase in abundance, from a low level, whereas the other species maintains its typical abundance (Brionez-Fourzán, 2014).
The 3 years of observations reported here seem to confirm that the invasibility criterion has been fulfilled, as the quagga did increase in abundance (cf. Figure 3). After its first record at ZS6 in November 2014, the abundance ratio of the two dreissenids was in favor of the zebra mussel (4:6) and remained so in the first half of 2015 (until July). Subsequently, until May 2016, the proportion shifted in favor of the quagga, to change again to a more or less even ratio (June and July 2016), following which the quagga abundance declined and the ratio changed again in favor of the zebra mussel (75–95% of the combined dreissenid abundance). A similar ratio (85–92%), in favor of the zebra mussel, was maintained throughout 2017.
Since the onset of observations the quagga was a clear dominant in terms of biomass, despite a marked decline in its abundance in the consecutive months of 2015 and 2016. Even at the peak zebra mussel numerical domination in October 2016 (the D. polymorpha abundance was 7.5 times that of D. rostriformis bugensis), the quagga biomass was 3.5 times that of the zebra mussel. In 2017, despite a marked decline in the quagga abundance, the biomass ratio was more or less even. This effect was resulted from the quagga population being dominated by larger (older) bivalves (Wawrzyniak-Wydrowska et al., in prep.).
The changes in population sizes of the two dreissenids are to some extent consistent with a general pattern of events following an invasion (see above). The quagga is still at the early stage of invasion, although probably not at the initial phase, because – when first recorded (November 2014) – the individuals found were of a size suggesting they were about 3 years old, so the immigration must have occurred earlier. Nevertheless, the early stage of invasion implies a lower adaptability to the conditions of the Szczecin Lagoon. The differences between the two species may, on the one hand, reflect internal regulation mechanisms, and on the other, may be an outcome of feedbacks resulting from inter-specific competition for space and food as well as cyclicity in reproductive potential and settlement success (Strayer and Malcom, 2006; Pace et al., 2010; D’Hont et al., 2018).
Conclusion and Outlook
In 2014–2017, we followed the dynamics, in terms of abundance and biomass, of two non-indigenous dreissenids, D. polymorpha and D. rostriformis bugensis co-occurring at a station in the Szczecin Lagoon. We managed to observe how the latter clearly established itself in the area, was co-occurring and possibly was entering co-existence with the former. We consider it important to record changes in both populations at the initial stage of the new immigrant’s establishment, the more so that D. rostriformis bugensis shows – like in other areas where more long-term data are available (e.g., D’Hont et al., 2018) – signs of becoming dominant over the already established D. polymorpha. It, however, remains to be seen what the two populations are being driven by in the Szczecin Lagoon.
Considering the “known unknowns” in the co-occurrence of the two dreissenids in the Lagoon, it seems necessary to continue observations on changes in their populations and to explore their respective population structures. Longer-term studies on demography of both species will be helpful in shedding light on interactions between them and in forming predictions regarding the fate of the two species in the area. It will be particularly interesting to learn whether the quagga will be persistently successful in colonizing the Lagoon, as was the case in other areas it migrated into where, in a relatively short time of 5–10 years, it outcompeted the zebra mussel (e.g., Mills et al., 1996; Orlova et al., 2004; Ricciardi and Whoriskey, 2004; Karatayev et al., 2015), although the latter may still persist and feature prominently in the Lagoon’s ecosystem, as it has done in. e.g., Lake Erie (cf. Karatayev et al., 2014b).
Author Contributions
BW-W developed the plan of research. BW-W, AW, and AS conducted the field work. BW-W, AS, and AW processed the samples. BW-W and TR analyzed the data. All authors contributed to writing the manuscript.
Funding
This study was supported by the Faculty of Geosciences, University of Szczecin, Szczecin, Poland within the framework of the statutory funds for research.
Conflict of Interest Statement
The authors declare that the research was conducted in the absence of any commercial or financial relationships that could be construed as a potential conflict of interest.
The handling Editor is currently organizing a Research Topic with one of the authors TR, and confirms the absence of any other collaboration.
Acknowledgments
Thanks are due to our skipper, Mr. Mieczysław Ziarkiewicz, for his much-appreciated assistance with sampling. We appreciate greatly the input received from three reviewers whose comments helped us to improve the manuscript.
Supplementary Material
The Supplementary Material for this article can be found online at: https://www.frontiersin.org/articles/10.3389/fmars.2019.00076/full#supplementary-material
References
Ardisson, P. L., and Bourget, E. (1991). Abundance, growth, and production estimation of the blue mussel Mytilus edulis on moored navigation buoys in the estuary and northwestern gulf of St. Lawrence. Can. J. Fish. Aquat. Sci. 48, 2408–2419. doi: 10.1139/f91-282
Baldwin, B. S., Mayer, M. S., Dayton, J., Pau, N., Mendilla, J., Sullivan, M., et al. (2002). Comparative growth and feeding in zebra and quagga mussels (Dreissena polymorpha and Dreissena bugensis): implications for North American lakes. Can. J. Fish. Aquat. Sci. 59, 680–694. doi: 10.1139/f02-043
Bale, A. J., and Kenny, A. J. (2005). “Sediment Analysis and Seabed Characterisation,” in Methods for the Study of Marine Benthos, 3 Edn, eds A. Eleftheriou and A. McIntyre (Oxford: Blackwell Science), 43–86. doi: 10.1002/9780470995129.ch2
Bangel, H., Schernewski, G., Bachor, A., and Landsberg-Uczciwek, M. (2004). “Spatial pattern and long-term development of water quality in the Oder Estuary,” in The Oder Estuary – Against the Background of the European Water Framework Directive, eds G. Schernewski and T. Dolch (Rostock: Institut für Ostseeforschung Warnemünde), 17–65.
Bially, A., and MacIsaac, H. J. (2000). Fouling mussels (Dreissena spp.) colonize soft sediments in lake erie and facilitate benthic invertebrates. Freshw. Biol. 43, 85–97. doi: 10.1046/j.1365-2427.2000.00526.x
Bierman, V. J. Jr., Kaur, J., DePinto, J. V., Feist, T. J., and Dilks, D. W. (2005). Modeling the role of zebra mussels in the proliferation of blue-green algae in saginaw bay, lake huron. J. Great Lakes Res. 31, 32–55. doi: 10.1016/S0380-1330(05)70236-7
Bij de Vaate, A. (2010). Some evidence for ballast water transport being the vector of the quagga mussel (Dreissena rostriformis bugensis Andrusov, 1897) introduction into Western Europe and subsequent upstream dispersal in the River Rhine. Aquat. Invasions 5, 207–209. doi: 10.3391/ai.2010.5.2.13
Boegehold, A. G., Johnson, N. S., Ram, J. L., and Kashian, D. R. (2018). Cyanobacteria reduce quagga mussel (Dreissena rostriformis bugensis) spawning and fertilization success. Freshw. Sci 37, 510–518. doi: 10.1086/698353
Brionez-Fourzán, P. (2014). Differences in life-history and ecological traits between co-occurring Panulirus spiny lobsters (Decapoda, Palinuridae). ZooKeys 457, 289–311. doi: 10.3897/zookeys.457.6669
Chesson, P. (2000). Mechanisms of maintenance of species diversity. Annu. Rev. Ecol. Evol. Syst. 31, 343–366. doi: 10.1146/annurev.ecolsys.31.1.343
Claxton, W. T., and Mackie, G. L. (1998). Seasonal and depth variations in gametogenesis and spawning of Dreissena polymorpha and Dreissena bugensis in eastern Lake Erie. Can. J. Zool. 76, 2010–2019. doi: 10.1139/z98-150
Cockrell, M. L., Bernhardt, J. R., and Leslie, H. M. (2015). Recruitment, abundance, and predation on the blue mussel (Mytilus edulis) on northeastern estuarine rocky shores. Ecosphere 6:art18. doi: 10.1890/ES14-00176.1
Czugała, A., and Woźniczka, A. (2010). The River odra estuary – another baltic sea area colonized by the round goby Neogobius melanostomus Pallas, 1811. Aquat. Invasions 5(Suppl. 1), S61–S65. doi: 10.3391/ai.2010.5.S1.014
Daunys, D., Zemlys, P., Olenin, S., Zaiko, A., and Ferrarin, C. (2006). Impact of the zebra mussel Dreissena polymorpha invasion on the budget of suspended matter in a shallow lagoon ecosystem. Helgol. Mar. Res. 60, 113–120. doi: 10.1007/s10152-006-0028-5
Dettman, J. R., Jacobson, D. J., Turner, E., Pringle, A., and Taylor, J. W. (2003). Reproductive isolation and phylogenetic divergence in neurospora: comparing methods of species recognition in a model eukaryote. Evolution 57, 2721–2741. doi: 10.1111/j.0014-3820.2003.tb01515.x
D’Hont, A., Gittenberger, A., Hendriks, A. J., and Leuven, R. S. E. W. (2018). Drivers of dominance shifts between invasive ponto-caspian dreissenids Dreissena polymorpha (Pallas, 1771) and Dreissena rostriformis bugensis (Andrusov, 1897). Aquat. Invasions 13, 449–462. doi: 10.3391/ai.2018.13.4.03
Diggins, T. P. (2001). A seasonal comparison of suspended sediment filtration by quagga (Dreissena bugensis) and zebra mussels (D. polymorpha). J. Great Lakes Res. 27, 457–466. doi: 10.1016/S0380-1330(01)70660-0
Dorgelo, J. (1993). “Growth and population structure of the zebra mussel (Dreissena polymorpha) in Dutch lakes different in trophic state,” in Zebra Mussels: Biology, Impacts, and Control, eds T. F. Nalepa and D. W. Schloesser (Ann Arbor, MI: Lewis Publishers), 79–94.
Fenske, C., Dahlke, S., Riel, P., and Woźniczka, A. (2010). “Dynamics of mussel beds in the Szczecin Lagoon,” in From Brazil to Thailand – New Results in Coastal Research, eds K. Schwarzer, K. Schrottke, and K. Stattegger (Rostock: EUCC - Die Küsten Union Deutschland e.V), 51–58.
Fenske, C., Zaiko, A., Wozniczka, A., Dahlke, S., and Orlova, M. I. (2013). “Variation in length-frequency distributions of zebra mussels (Dreissena polymorpha) within and between three Baltic Sea subregions Szczecin Lagoon, Curonian Lagoon, and Gulf of Finland,” in Quagga and Zebra Mussels: Biology, Impacts and Control, eds T. F. Nalepa and D. W. Schlosser (Boca Raton, FL: CRC Press Taylor), 725–740.
Fernald, S. H., Caraco, N. F., and Cole, J. J. (2007). Changes in cyanobacterial dominance following the invasion of the zebra mussel Dreissena polymorpha: long-term results from the hudson river estuary. Estuaries Coasts 30, 163–170. doi: 10.1007/BF02782976
Flynn, D. F. B., Mirotchnick, N., Matthew, M. J., Palmer, J. I., and Naeem, S. (2011). Functional and phylogenetic diversity as predictors of biodiversity–ecosystem-function relationships. Ecology 92, 1573–1581. doi: 10.1890/10-1245.1
Foley, C. J., Andree, S. R., Pothoven, S. A., Nalepa, T. F., and Höök, T. O. (2017). Quantifying the predatory effect of round goby on saginaw bay dreissenids. J. Great Lakes Res. 43, 121–131. doi: 10.1016/j.jglr.2016.10.018
Fréchette, M., and Bourget, E. (1985). Energy flow between the pelagic and benthic zones: factors controlling particulate organic matter available to an intertidal mussel bed. Can. J. Fish. Aquat. Sci. 42, 1158–1165. doi: 10.1139/f85-143
Garton, D. W., McMahon, R., and Stoeckmann, A. M. (2014). “Limiting Environmental Factors and Competitive Interactions between Zebra and Quagga Mussels in North America,” in Quagga and Zebra Mussels: Biology, Impacts, and Control, 2nd Edn, eds T. F. Nalepa and D. W. Schloesser (Boca Raton, FL: CRC Press), 695–703.
Gaskill, J. A., and Woller-Skar, M. M. (2018). Do invasive dreissenid mussels influence spatial and temporal patterns of toxic Microcystis aeruginosa in a low-nutrient Michigan lake? Lake Reserv. Manag. 34, 244–257. doi: 10.1080/10402381.2018.142358
Gotelli, N. J. (2000). Null model analysis of species co-occurrence patterns. Ecology 81, 2606–2621. doi: 10.1890/0012-9658(2000)081[2606:NMAOSC]2.0.CO;2
Gruszka, P. (1999). The river odra estuary as a gateway for alien species immigration to the baltic sea basin. Acta Hydrochim. Hydrobiol. 27, 374–382. doi: 10.1002/(SICI)1521-401X(199911)27:5<374::AID-AHEH374>3.0.CO;2-V
Gutierrez, J. L., Jones, C. G., Strayer, D. L., and Iribarne, O. O. (2003). Mollusks as ecosystem engineers: the role of shell production in aquatic habitats. Oikos 101, 79–90. doi: 10.1034/j.1600-0706.2003.12322.x
Hecky, R. E., Smith, R. E. H., Barton, D. R., Guilford, S. J., Taylor, W. D., Charlton, M. N., et al. (2004). The nearshore phosphorus shunt: a consequence of ecosystem engineering by dreissenids in the laurentian great lakes. Can. J. Fish. Aquat. Sci. 61, 1285–1293. doi: 10.1139/f04-065
Heiler, K. C. M., Bij de Vaate, A., Ekschmitt, K., von Oheimb, P. V., Albrecht, C., and Wilke, T. (2013). Reconstruction of the early invasion history of the quagga mussel (Dreissena rostriformis bugensis) in Western Europe. Aquat. Invasions 1, 53–57. doi: 10.3391/ai.2013.8.1.06
Higgins, S. N., and Vander Zanden, M. J. (2010). What a difference a species makes: a meta-analysis of dreissenid mussel impacts on freshwater ecosystems. Ecol. Monogr. 80, 179–196. doi: 10.1890/09-1249.1
HilleRisLambers, J., Adler, P. B., Harpole, W. S., Levine, J. M., and Mayfield, M. M. (2012). Rethinking community assembly through the lens of coexistence theory. Annu. Rev. Ecol. Evol. Syst. 43, 227–248. doi: 10.1146/annurev-ecolsys-110411-160411
Izvekova, E. I., and Lvova-Katchanova, A. A. (1972). Sedimentation of suspended matter by Dreissena polymorpha Pallas and its subsequent utilization by Chironomidae larvae. Pol. Arch. Hydrobiol. 19, 203–210.
Jantz, B., and Neumann, D. (1992). “Shell growth and population dynamics of Dreissena polymorpha in the River Rhine,” in The Zebra Mussel Dreissena Polymorpha. Limnologie till, eds D. Neumann and H. A. Jenner (Stuttgart: Gustav Fischer Verlag), 49–66.
Jones, L. A., and Ricciardi, A. (2005). Influence of physicochemical factors on the distribution and biomass of invasive mussels (Dreissena polymorpha and Dreissena bugensis) in the St. Lawrence River. Can. J. Fish. Aquat. Sci. 62, 1953–1962. doi: 10.1139/f05-096
Karatayev, A. Y., Burlakova, L. E., Molloy, D. P., and Volkova, L. K. (2000). Endosymbionts of Dreissena polymorpha (Pallas) in Belarus. Int. Rev. Hydrobiol. 85, 543–559. doi: 10.1002/1522-2632(200011)85:5/6<543::AID-IROH543>3.0.CO;2-3
Karatayev, A. Y., Burlakova, L. E., and Padilla, D. K. (1997). The effects of Dreissena polymorpha (Pallas) invasion on aquatic communities in Eastern Europe. J. Shellfish Res. 16, 187–203.
Karatayev, A. Y., Burlakova, L. E., and Padilla, D. K. (1998). Physical factors that limit the distribution and abundance of Dreissena polymorpha (Pall.). J. Shellfish Res. 17, 1219–1235.
Karatayev, A. Y., Burlakova, L. E., and Padilla, D. K. (2002). “Impacts of zebra mussels on aquatic communities and their role as ecosystem engineers,” in Invasive Aquatic Species of Europe: Distribution, Impacts and Management, eds E. Leppäkoski, S. Gollasch, and S. Olenin (Dordrecht: Kluwer Academic Publishers), 433–446.
Karatayev, A. Y., Burlakova, L. E., and Padilla, D. K. (2014a). “General overview of zebra and quagga mussels what we do and do not know,” in Quagga and Zebra Mussels: Biology, Impacts, and Control, 2nd Edn, eds T. F. Nalepa and D. W. Schloesser (Boca Raton, FL: CRC Press), 695–703.
Karatayev, A. Y., Burlakova, L. E., Pennuto, C., Ciborowski, J., Karatayev, V. A., Juette, P., et al. (2014b). Twenty five years of changes in Dreissena spp. populations in Lake Erie. J. Great Lakes Res. 40, 550–559. doi: 10.1016/j.jglr.2014.04.010
Karatayev, A. Y., Burlakova, L. E., and Padilla, D. K. (2015). Zebra versus quagga mussels: a review of their spread, population dynamics, and ecosystem impacts. Hydrobiologia 746, 97–112. doi: 10.1007/s10750-014-1901-x
Keller, R. P., Drake, J. M., and Lodge, D. M. (2007). Fecundity as a basis for risk assessment of nonindigenous freshwater molluscs. Conserv. Biol. 21, 191–200. doi: 10.1111/j.1523-1739.2006.00563.x
Kelley, D. W., Herborg, L. M., and MacIsaak, H. J. (2010). “Ecosystem changes associated with Dreissena invasions: recent developments and emerging issues,” in The Zebra Mussel in Europe, eds G. van der Velde, S. Rajagopal, and A. Bij de Vaate (Leiden: Backhuys Publishers), 199–209.
Krebs, C. J. (1995). Two paradigms of population regulation. Wildlife Res. 22, 1–10. doi: 10.1071/WR9950001
Kublickas, A. (1959). “Feeding of benthophagous fish in the Curonian lagoon,” in Kursiu Marios, eds K. Jankevicius, I. Gasiûnas, A. Gediminas, V. Gudelis, A. Kublickas, and I. Maniukas (Vilnius: Lithuanian Academy of Sciences), 463–521.
Labropoulou, M., and Eleftheriou, A. (1997). The foraging ecology of two pairs of congeneric demersal fish species: importance of morphological characteristics in prey selection. J. Fish Biol. 50, 324–340. doi: 10.1111/j.1095-8649.1997.tb01361.x
Liebig, J. R., and Vanderploeg, H. A. (1995). Vulnerability of Dreissena polymorpha larvae to predation by great lakes calanoid copepods: the importance of the bivalve Shell. J. Great Lakes Res. 21, 353–358. doi: 10.1016/S0380-1330(95)71046-2
Lockwood, J. L., Hoopes, M. F., and Marchetti, M. P. (2007). Invasion Ecology. Hoboken, NJ: Blackwell Publishing.
Lucy, F. (2006). Early life stages of Dreissena polymorpha (zebra mussel): the importance of long-term datasets in invasion ecology. Aquat. Invasions 1, 171–182. doi: 10.3391/ai.2006.1.3.12
MacIsaac, H. J., Sprules, W. G., and Leach, J. H. (1991). Ingestion of small-bodied zooplankton by zebra mussels (Dreissena polymorpha): can cannibalism on larvae influence population dynamics? Can. J. Fish Aquat. Sci. 48, 2051–2060. doi: 10.1139/f91-244
Marchowski, D., Neubauer, G., Ławicki,Ł, Woźniczka, A., Wysocki, D., Guentzel, S., et al. (2015). The importance of non-native prey, the zebra mussel Dreissena polymorpha, for the declining greater scaup Aythya marila: a case study at a key european staging and wintering site. PLoS One 10:e0145496. doi: 10.1371/journal.pone.0145496
Masłowski, J. (1993). Long-term changes in the bottom macrofauna of the Szczecin Lagoon (north-western Poland). Acta Hydrobiol. 35, 341–355.
Mastitsky, S. E., and Gagarin, V. G. (2004). Nematodes which infect the mollusc Dreissena polymorpha (Bivalvia: Dreissenidae) in Narochanskie Lakes. Vestnik Belorusskogo Gosudarstvennogo Universiteta. Seriya 2, 22–25.
Matthews, J., Van der Velde, B., Bij de Vaate, A., Collas, F. P. L., Koopman, K. R., and Leuven, R. S. E. W. (2014). Rapid range expansion of the invasive quagga musselin relation to zebra mussel presence in The Netherlands and Western Europe. Biol. Invasions 16, 23–42. doi: 10.1007/s10530-013-0498-8
McDonald, J. H. (2014). Handbook of Biological Statistics, 3rd Edn. Baltimore, MD.: Sparky House Publishing.
McMahon, R. F. (1996). The physiological ecology of the zebra mussel, Dreissena polymorpha, in North America and Europe. Am. Zool. 36, 339–363. doi: 10.1016/j.fsi.2016.06.054
Meyer, H., and Lampe, R. (1999). The restricted buffer capacity of a south baltic estuary – the oder estuary. Limnologica 29, 242–248. doi: 10.1016/S0075-9511(99)80008-5
Mills, E. L., Rosenberg, G., Spidle, A. P., Ludyanskiy, M., Pligin, Y., and May, B. (1996). A review of the biology and ecology of the quagga mussel (Dreissena bugensis), a second species of freshwater dreissenid introduced to North America. Am. Zool. 36, 271–286. doi: 10.1093/icb/36.3.271
Molloy, D. P., Bij de Vaate, A., Wilke, T., and Giamberini, L. (2007). Discovery of Dreissena rostriformis bugensis (Andrusov 1897) in Western Europe. Biol. Invasions 9, 871–874. doi: 10.1007/s10530-006-9078-5
Molloy, D. P., Karatayev, A. Y., Burlakova, L. E., Kurandina, D. P., and Laruelle, F. (1997). Natural enemies of zebra mussels: predators, parasites and ecological competitors. Rev. Fish. Sci. 5, 27–97. doi: 10.1080/10641269709388593
Mörtl, M. S., Werner, K., and Rothhaupt, O. (2010). “Effects of predation by wintering water birds on zebra mussels and on associated macroinvertebrates,” in The Zebra Mussel in Europe, eds G. Van der Velde, S. Rajagopal, and A. Bij de Vaate (Leiden: Backhuys Publishers), 239–249.
Naddafi, R., Pettersson, K., and Eklöv, P. (2010). Predation and physical environment structure the density and population size structure of zebra mussels. J. N. Am. Benthol. Soc. 29, 444–453. doi: 10.1899/09-071.1
Naddafi, R., and Rudstam, L. G. (2014). Predation on invasive zebra mussel, Dreissena polymorpha, by pumpkinseed sunfish, rusty crayfish, and round goby. Hydrobiologia 721, 107–115. doi: 10.1007/s10750-013-1653-z
Nalepa, T. F., Fanslow, D. L., and Lang, G. A. (2009). Transformation of the offshore benthic community in Lake Michigan: recent shift from the native amphipod Diporeia spp. to invasive mussel Dreissena rostriformis bugensis. Freshw. Biol. 54, 466–479. doi: 10.1111/j.1365-2427.2008.02123.x
Nalepa, T. F., Fanslow, D. L., and Pothoven, S. A. (2010). Recent changes in density, biomass, recruitment, size structure, and nutritional state of Dreissena populations in southern Lake Michigan. J. Great Lakes Res. 36, 5–19. doi: 10.1016/j.jglr.2010.03.013
Nalepa, T. F., and Schloesser, D. W. (1993). Zebra Mussels Biology, Impacts, and Control. Boca Raton, FL: Lewis Publishers.
Nalepa, T. F., and Schloesser, D. W. (2014). Quagga and Zebra Mussels: Biology, Impacts, and Control, 2nd Edn. Boca Raton, FL: CRC Press.
Nentwig, W. (2008). “Pathways in Animal Invasion,” in Biological Invasion, ed. W. Nentwig (Berlin: Springer), 11–27.
Nogaro, G., and Steinman, A. D. (2013). Influence of ecosystem engineers on ecosystem processes is mediated by lake sediment properties. Oikos 123, 500–512. doi: 10.1111/j.1600-0706.2013.00978.x
Noordhuis, R., van Erden, M. R., and Roos, M. (2010). “Crash of zebra mussel, transparency and water bird populations in Lake Markermeer,” in The Zebra Mussel in Europe, eds G. van der Velde, S. Rajagopal, and A. bij de Vaate (Leiden: Backhuys Publisher), 265–277.
Olenin, S., Gollasch, S., Lehtiniemi, M., Sapota, M., and Zaiko, A. (2017). “Biological invasions”,” in Biological Oceanography of the Baltic Sea, eds P. Snoeijs-Leijonmalm, H. Schubert, and T. Radziejewska (Dordrecht: Springer Science+Business Media), 193–232. doi: 10.1007/978-94-007-0668-2_5
Orlova, M. I., Muirhead, J., Antonov, P. I., Shcherbina, G. K., Starobogatov, I., Biochino, G. I., et al. (2004). Range expansion of quagga mussels Dreissena rostriformis bugensis in the Volga River and Caspian Sea basin. Aquat. Ecol. 38, 561–573. doi: 10.1007/s10452-004-0311-y
Pace, M. L., Strayer, D. L., Fischer, D., and Malcom, H. M. (2010). Recovery of native zooplankton associated with increased mortality of an invasive mussel. Ecosphere 1, 1–10. doi: 10.1890/ES10-00002.1
Piesik, Z. (1974). The role of crayfish Orconectes limosus (Raf.) in extinction of Dreissena polymorpha subsisting on steelon-net. Pol. Arch. Hydrobiol. 21, 401–410.
Piesik, Z. (1983). Biology of Dreissena polymorpha (Pall.) settling on stylon nets and the role of this mollusk in eliminating the seston and the nutrients from the water-course. Pol. Arch. Hydrobiol. 30, 353–361.
Piesik, Z. (1992). The biological and ecological role of the fouling organisms inhabiting artificial substrates in various types of water. Rozpr. Stud. Uniw. Szczecińskiego 12, 127–128.
Pigot, A. L., and Tobias, J. A. (2013). Species interactions constrain geographic range expansion over evolutionary time. Ecol. Lett. 16, 330–338. doi: 10.1111/ele.12043
Polsenaere, P., Soletchnik, P., Le Moine, O., Gohin, F., Robert, S., Pépin, H.-F., et al. (2017). Potential environmental drivers of a regional blue mussel mass mortality event (winter 2014, Breton Sound, France). J. Sea Res. 123, 39–50. doi: 10.1016/j.seares.2017.03.005
Popa, O. P., and Popa, L. O. (2006). The most westward European occurrence point for Dreissena bugensis (Andrusov 1897). Malacol. Bohemoslov. 5, 3–5.
Radziejewska, T., Fenske, C., Wawrzyniak-Wydrowska, B., Woźniczka, A., and Gruszka, P. (2009). The zebra mussel (Dreissena polymorpha) and the benthic community in a coastal Baltic lagoon: another example of enhancement? Mar. Ecol. 30, 138–150. doi: 10.1111/j.1439-0485.2009.00313.x
Radziejewska, T., and Schernewski, G. (2008). “The Szczecin (Oder-) Lagoon,” in Ecology of Baltic Coastal Waters Series, ed. U. Schiewer (Heidelberg: Springer-Verlag), 115–129. doi: 10.1007/978-3-540-73524-3_5
Ricciardi, A., and MacIsaac, H. J. (2000). Recent mass invasion of the North American Great Lakes by Ponto-Caspian species. Trends Ecol. Evol. 15, 62–66. doi: 10.1016/S0169-5347(99)01745-0
Ricciardi, A., and Whoriskey, F. G. (2004). Exotic species replacement: shifting dominance of dreissenid mussels in the soulanges canal, upper St. Lawrence River, Canada. J. N. Am. Benthol. Soc. 23, 507–514. doi: 10.1899/0887-3593(2004)023<0507:ESRSDO>2.0.CO;2
Rilov, G., and Crooks, J. A. (2009). Biological Invasions in Marine Ecosystems, Ecological, Management, and Geographic Perspectives. Berlin: Springer Science. doi: 10.1007/978-3-540-79236-9
Roe, S. L., and MacIsaac, H. J. (1997). Deepwater population structure and reproductive state of quagga mussels (Dreissena bugensis) in Lake Erie. Can. J. Fish. Aquat. Sci. 54, 2428–2433. doi: 10.1139/f97-151
Schneider, D. W., Madon, S. P., Stoeckel, J. A., and Sparks, R. E. (1998). Seston quality controls zebra mussel (Dreissena polymorpha?) energetics in turbid rivers. Oecologia 117, 331–341. doi: 10.1007/s004420050666
Scott, L. E. (2009). Co-occurrence with a Congeneric Species Predicts Life History and Morphological Diversification in the Mexican Livebearing fish Poelicopsis baenschi. M.Sc. Thesis, Brigham Young University, Brigham.
Sfenthourakis, S., Tzanatos, E., and Giokas, S. (2005). Species co-occurrence: the case of congeneric species and a causal approach to patterns of species association. Glob. Ecol. Biogeogr. 15, 39–49. doi: 10.1111/j.1466-822X.2005.00192.x
Smit, H., Bij de Vaate, A., Reeders, H. H., Van Nes, E. H., and Noordhuis, R. (1993). “Colonization, ecology, and positive aspects of zebra mussels (Dreissena polymorpha) in The Netherlands,” in Zebra Mussels Biology, Impacts and Control, eds T. F. Nalepa and D. Schloesser (Boca Raton, FL: CRS Press), 55–77.
Sprung, M. (1995a). Physiological energetics of the zebra mussel Dreissena polymorpha in lakes I. Growth and reproductive effort. Hydrobiologia 304, 117–132. doi: 10.1007/BF02579417
Sprung, M. (1995b). Physiological energetics of the zebra mussel Dreissena polymorpha in lakes III. Metabolism and net growth efficiency. Hydrobiologia 304, 147–158. doi: 10.1007/BF02579419
Stańczykowska, A., Lewandowski, K., and Czarnoleski, M. (2010). “Distribution and densities of Dreissena polymorpha in Poland – past and present” in The Zebra Mussel in Europe, eds G. Van der Velde, S. Rajagopal, and A. Bij de Vaate (Leiden: Backhuys Publishers), 119–126.
Stańczykowska, A., and Planter, M. (1985). Role of mussel Dreissena polymorpha (Pall.) in N and P cycles in a lake ecosystem. Ekol. Pol. 33, 345–354.
Stanisz, A. (2007). Przystȩpny kurs statystyki z zastosowaniem STATISTICA PL na przykładach z medycyny. StatSoft Polska Kraków 2, 137–166.
Stewart, T. W., Gafford, J. C., Miner, J. G., and Lowe, R. L. (1999). Dreissena-shell habitat and antipredator behavior: combined effects on survivorship of snails co-occurring with molluscivorous fish. J. N. Am. Benthol. Soc. 18, 274–283. doi: 10.2307/1468465
Stewart, T. W., Miner, J. G., and Lowe, R. L. (1998). Quantifying mechanisms for zebra mussel effects on benthic macroinvertebrates: organic matter production and shell-generated habitat. J. N. Am. Benthol. Soc. 17, 81–94. doi: 10.2307/1468053
Stoeckmann, A. (2003). Physiological energetics of Lake Erie dreissenid mussels: a basis for the displacement of Dreissena polymorpha by Dreissena bugensis. Can. J. Fish Aquat. Sci. 60, 126–134. doi: 10.1139/f03-005
Strayer, D. L., and Malcom, H. (2006). Long term demography of a zebra mussel (Dreissena polymorpha). Freshw. Biol. 51, 117–130. doi: 10.1111/j.1365-2427.2005.01482.x
Tyutin, A. V. (2005). “Aboriginal parasitic systems following invasions of new aquatic species in the Rybinsk Reservoir (the Upper Volga, Russia),” in Proceedings of the Second International Symposium “Alien Species in Holarctic (Borok-2), eds Y. Y. Dgebuadze and Y. V. Slynko (Borok: CAB International), 131–132.
Van Eerden, M. R., and De Leeuw, J. (2010). “How Dreissena sets the winter scene for water birds: Dynamic interaction between diving ducks and zebra mussel,” in The Zebra Mussel in Europe, eds G. van der Velde, S. Rajagopal, and A. bij de Vaate (Leiden: Backhuys Publisher), 251–264.
Vanderploeg, H. A., Nalepa, T. F., Jude, D. J., Mills, E. L., Holeck, K. T., Liebig, J. R., et al. (2002). Dispersal and emerging ecological impacts of ponto-caspian species in the Laurentian Great Lakes. Can. J. Fish. Aquat. Sci. 59, 1209–1228. doi: 10.1139/f02-087
Veech, J. A. (2014). The pairwise approach to analysing species co-occurrence. J. Biogeogr. 41, 1029–1035. doi: 10.1111/jbi.12318
Walter, G. H. (1991). What is resource partitioning? J. Theor. Biol. 150, 137–143. doi: 10.1016/S0022-5193(05)80327-3
Ward, J. M., and Ricciardi, A. (2007). Impacts of Dreissena invasions on benthic macroinvertebrate communities: a meta-analysis. Divers. Distrib. 13, 155–165. doi: 10.1111/j.1472-4642.2007.00336.x
Wawrzyniak-Wydrowska, B., and Gruszka, P. (2005). Population dynamics of alien gammarid species in the River Odra estuary. Hydrobiologia 539, 13–25. doi: 10.1007/s10750-004-3081-6
Wiktor, J. (1962). Quantitative and qualitative investigations of the Szczecin Firth bottom fauna. Part II. Pr. Mor. Inst. Ryb. Gdynia 11, 81–112.
Wiktor, J. (1969). The biology of Dreissena polymorpha (Pall.) and its biological importance in the Firth of Szczecin. Stud. Mat. Mor. Inst. Ryb. Gdynia A, 1–88.
Wolnomiejski, N., and Witek, Z. (2013). The Szczecin Lagoon Ecosystem: The Biotic Community of the Great Lagoon and its Food Web Model. London: Versita Ltd. doi: 10.2478/9788376560502
Wolnomiejski, N., and Woźniczka, A. (2008). A drastic reduction in abundance of Dreissena polymorpha Pall. in the Skoszewska Cove (Szczecin Lagoon, River Odra estuary): effects in the population and habitat. Ecol. Quest. 9, 103–111. doi: 10.2478/v10090-009-0025-9
Wong, W. H., Gerstenberger, S., Baldwin, W., and Moore, B. (2012). Settlement and growth of quagga mussels (Dreissenia rostriformis bugensis Andrusov, 1897) in Lake Mead, Nevada-Arizona, USA. Aquat. Invasions 7, 7–19. doi: 10.3391/ai.2012.7.1.002
Wong, W. H., Gerstenberger, S. L., Hatcher, M. D., Thompson, D. R., and Schrimsher, D. (2013). Invasive quagga mussels can be attenuated by redear sunfish (Lepomis microlophus) in the Southwestern United States. Biol. Control. 64, 276–282. doi: 10.1016/j.biocontrol.2012.11.006
Woźniczka, A., Wawrzyniak-Wydrowska, B., Radziejewska, T., and Skrzypacz, A. (2016). The quagga mussel (Dreissena rostriformis bugensis Andrusov, 1897) – another ponto-caspian dreissenid bivalve in the southern Baltic catchment: the first record from the Szczecin Lagoon. Oceanologia 58, 154–159. doi: 10.1016/j.oceano.2015.12.002
Woźniczka, A., and Wolnomiejski, N. (2005). Zebra mussel (Dreissena polymorpha Pall.) in the river odra estuary: the current status. 5th Baltic Sea Sci. Congr. Sopot Poland 373–374.
Zaiko, A., Minchin, D., and Olenin, S. (2014). “The day after tomorrow”: anatomy of an ‘r’ strategist aquatic invasion. Aquat. Invasions 9, 145–155. doi: 10.3391/ai.2014.9.2.03
Zaiko, A., Olenin, S., and Daunys, D. (2009). Habitat engineering by the invasive zebra mussel Dreissena polymorpha (Pallas) in a boreal coastal lagoon: impact on biodiversity. Helgol. Mar. Res. 63, 85–94. doi: 10.1007/s10152-008-0135-6
Zhulidov, A. V., Kozhara, A. V., Scherbina, G. H., Nalepa, T. F., Protasov, A., Afanasiev, S. A., et al. (2010). Invasion history, distribution, and relative abundances of Dreissena bugensis in the Old World: a synthesis of data. Biol. Invasions 12, 1923–1940. doi: 10.1007/s10530-009-9641-y
Keywords: non-indigenous species, zebra mussel, quagga, lagoon, Baltic Sea
Citation: Wawrzyniak-Wydrowska B, Radziejewska T, Skrzypacz A and Woźniczka A (2019) Two Non-indigenous Dreissenids (Dreissena polymorpha and D. rostriformis bugensis) in a Southern Baltic Coastal Lagoon: Variability in Populations of the “Old” and a “New” Immigrant. Front. Mar. Sci. 6:76. doi: 10.3389/fmars.2019.00076
Received: 05 June 2018; Accepted: 11 February 2019;
Published: 28 February 2019.
Edited by:
Hendrik Schubert, University of Rostock, GermanyReviewed by:
Sven Dahlke, University of Greifswald, GermanyMartin Powilleit, University of Rostock, Germany
Copyright © 2019 Wawrzyniak-Wydrowska, Radziejewska, Skrzypacz and Woźniczka. This is an open-access article distributed under the terms of the Creative Commons Attribution License (CC BY). The use, distribution or reproduction in other forums is permitted, provided the original author(s) and the copyright owner(s) are credited and that the original publication in this journal is cited, in accordance with accepted academic practice. No use, distribution or reproduction is permitted which does not comply with these terms.
*Correspondence: Brygida Wawrzyniak-Wydrowska, YnJ5Z2lkYS53eWRyb3dza2FAdXN6LmVkdS5wbA==