- 1Laboratorio de Ecología Costera, Centro de Investigación Científica de Yucatán, A.C. Unidad de Ciencias del Agua, Cancún, Mexico
- 2Laboratorio FICOTOX, Departamento de Oceanografía Biológica, Centro de Investigación Científica y de Educación Superior de Ensenada, Ensenada, Mexico
- 3Laboratorio de Toxinas Marinas y Aminoácidos, Centro de Investigaciones Biológicas del Noroeste, La Paz, Mexico
- 4Centro Universitario de Investigaciones Oceanológicas, Universidad de Colima, Manzanillo, Mexico
In the present study the abundance of epiphytic dinoflagellates was evaluated at two coral reef sites of the natural protected areas, Arrecife de Puerto Morelos and Isla Contoy, located in the northern Mesoamerican Reef System of the Mexican Caribbean. Abundances were monitored from April to December, 2015 on two genera of macroalga belonging to different functional groups: Dictyota and Amphiroa. In general, the total abundance of dinoflagellates was higher in Puerto Morelos on both macroalgae. Ostreopsis cf. marina and O. heptagona were the dominant species. Relative abundance of these species varied from 8 to 99% of total epiphytic dinoflagellates. Maximum abundances at Puerto Morelos were registered in April, with 33,801 cells·g−1 on Dictyota and 6,264 cells·g−1 on Amphiroa. In Isla Contoy the maximum abundance was 16,006 cells·g−1 and it was detected on Dictyota during December. Other dinoflagellate genera were more abundant during the warmer period (May–September) in both locations. Prorocentrum was the second most abundant genus and was represented by six species (P. hoffmannianum, P. lima, P. belizeanum, P. elegans, P. emarginatum, and P. rathymum). The maximum pooled abundance of Prorocentrum species was 4,144 cells·g−1 on Dictyota in August. Coolia spp. did not reach abundances higher than 1,000 cells·g−1 and Gambierdiscus spp. only exceeded 100 cells·g−1 during August. Mean water temperature in Isla Contoy was significantly lower than that of Puerto Morelos during the entire study period. There was a negative correlation between the water temperature and the abundance of O. cf. marina. The dominance of Ostreopsis in the Caribbean is significant because of its capability to produce palytoxin analogs and its potential role in ciguatera fish poisoning outbreaks in the region. This is the first study that reports blooms of Ostreopsis in Mexican Caribbean coral reefs, a fact that emphasizes the significance of this genus at global scale.
Introduction
There is the general assumption that ciguatera fish poisoning (CFP) outbreaks are linked to benthic dinoflagellate blooms, especially of toxic species of Gambierdiscus genus (Chinain et al., 1999; Turquet et al., 2001). However, the ecological processes that occur between the benthic harmful algae blooms and intoxication in humans are not as clear as planktonic harmful algae blooms (HABs), which can be more easily detected, especially those that color the water. For a beter understanding of the ciguatera phenomenon, it is necessary to increase the knowledge of the factors that control the abundance of toxin-producing epiphytic dinoflagellates, such as the influence of environmental variability, host-epiphytic dynamics, herbivory patterns on the macroalgae substrates, trophic relationships between vectors and even the preferences of human consumption on top predator fishes.
Most research has focused on the environmental variability and host-epiphytic dynamics through the analysis of the experimental responses of benthic dinoflagellates to variations of temperature, irradiance, nutrient concentration and salinity (Bomber et al., 1988; Chinain et al., 1999; Hales et al., 1999; Anderson et al., 2008; Lartigue et al., 2009; Kibler et al., 2015), as well as the substrate preference of some dinoflagellate species (Lobel et al., 1988; Bomber et al., 1989; Nakahara et al., 1996; Parsons and Preskitt, 2007; Parsons et al., 2011; Rains and Parsons, 2015), and the monitoring of dinoflagellate abundance on several substrates and macroalgae (Morton and Faust, 1997; Vila et al., 2001; Okolodkov et al., 2007, 2014; Moreira et al., 2012; Cohu et al., 2013). Also, other features such as depth, the water motion and habitat structure have been studied in relation to abundance and occurrence of the benthic dinoflagellates (Richlen and Lobel, 2011; Cohu et al., 2013; Boisnoir et al., 2018; Yong et al., 2018).
These investigations demonstrate that in many cases, benthic dinoflagellate abundances are influenced by increased temperature (Tester et al., 2010; Kibler et al., 2015). On this basis, seasonal variability of water temperature has received special attention as a predictive variable of dinoflagellate abundance and ciguatera outbreaks (Chinain et al., 1999; Tosteson, 2004; Chateau-Degat et al., 2005; Gingold et al., 2014). Although temperature could be the most important variable that explains changes in the abundance of benthic dinoflagellates, other factors could account for the dominance of a particular species. Particularly, macroalgal species could control the population dynamics of associated dinoflagellates. Macroalgae could promote or inhibit dinoflagellate growth through providing shelter or surface for attachment (Bomber et al., 1989; Parsons et al., 2011; Rains and Parsons, 2015).
The historical relationship between dinoflagellates and macroalgae may be facing a nonreversible change due to the replacement of macroalgae coastal communities as consequence of eutrophication and climate change (McCook, 1999). The impact of these phenomena on benthic communities is especially evident in tropical coral reefs ecosystems (Hoegh-Guldberg et al., 2007). These ecosystems constitute a common habitat for toxin-producing dinoflagellates (Yasumoto et al., 1980; Chinain et al., 1999). Hard corals are key species in these ecosystems because they function as shelter for a variety of species. Coralline macroalgae are associated with the coral in healthy coral reefs (Björk et al., 1995). However, coral cover is decreasing dramatically and their skeletons are being colonized by fleshy opportunistic macroalgae (Kohler and Kohler, 1992). This implies that macroalgae cover can reach high cover percentages and, in turn, constitute an extensive substratum for epiphytic dinoflagellates. Therefore, it is necessary to understand if the replacement of coralline macroalgae communities with fleshy macroalgae communities also changes the epiphytic dinoflagellate assemblage inhabiting them.
In the Mexican Caribbean coast, the CFP is a syndrome with a relatively high incidence. The main vector of ciguatera toxins is the great barracuda (Sphyraena barracuda) (Arcila-Herrera et al., 1998), but other fishes have also been involved in outbreaks. The presence of several potentially toxic species of the genera Gambierdiscus, Prorocentrum, Ostreopsis, Coolia, and Amphidinium has been registered in the Mexican Caribbean (Hernandez-Becerril and Almazan-Becerril, 2004; Almazán-Becerril et al., 2015, 2016).
In the coral reefs of the Mexican Caribbean fleshy macroalgae cover is higher than 50% and may reach 90% at some sites (Delgado-Pech, 2016). Dictyota is one of the dominant genera and may account for the 80% of the total macroalgae cover at some sites and periods. This implies that, as dominant macroalgae, Dictyota could increase the density of dinoflagellates in absolute terms (cells·m2).
The aim of this work is to characterize taxonomic composition and abundance of dinoflagellate community in two functionally distinct algae: Dictyota and Amphiroa at two sites located at the northern portion of the Mesoamerican Coral Reef Barrier. Each genera of macroalgae represent different states of coral reef health. Dictyota represents an impacted coral reef and Amphiroa a healthy one.
Materials and Methods
Study Area
This study was carried out from April to December 2015 at two reef sites located in the northern Mexican Caribbean: Isla Contoy (IC) and Puerto Morelos (PM). Both sites are protected natural areas of the Mesoamerican Reef Barrier System (Figure 1). Ixlaché reef is located 1 km south of IC. It is a small reef 800 m long and 60 m wide. Depth varies between 0.5 and 3 m, macroalgae cover is 60%, and is influenced by coastal currents, waves, tides, and winds predominantly from the southeast and polar fronts. On the other hand, the Bonanza reef site is part of the PM reef; it is a shallow area of <2 m depth and macroalgae cover is higher than 75%.
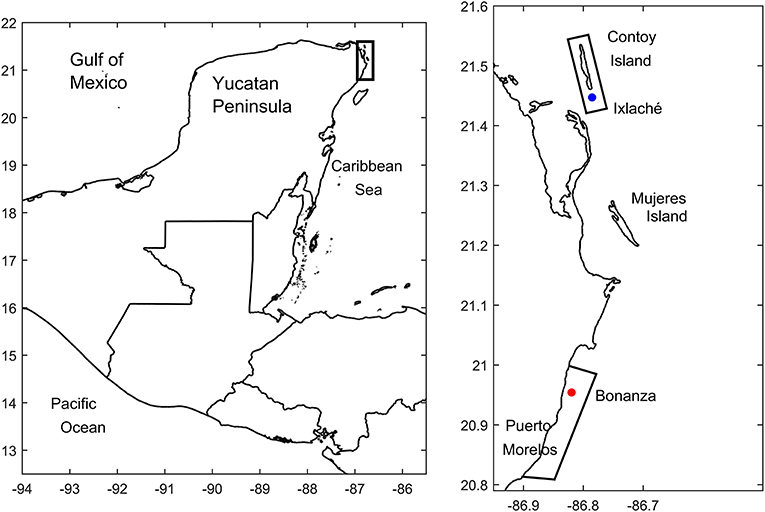
Figure 1. Zone of study. Blue point corresponds to the Ixlaché Reef located at the Parque Nacional Isla Contoy, and the red point corresponds to La Bonanza reef at the Parque Nacional Arrecife de Puerto Morelos. Natural Protected Areas are enclosed with a frame.
Substrate Selection
Two macroalgae species were selected for this study, each representing a distinct functional group (Littler et al., 1983; Steneck and Deither, 1994). Amphiroa is a slow-growing rate coralline articulated macoalgae present at relatively low cover in the sites of study. Dictyota is fast-growing rate fleshy, foliose macroalgae with a high cover. Both sites selected for this study are macroalgae-dominated reefs where Dictyota, Turbinaria, Lobophora, and Laurencia are very common, although patches of the coralline algae Amphiroa still remain.
Collection of Macroalgae Samples
At each site, 10 specimens of each genus of macroalgae were collected. The distance between collected specimens was at least 10 m according to Lobel et al. (1988). Collected macroalgae were stored in plastic bags with seawater and placed into a cooler with water of the site to maintain the temperature. Algae were fixed with formaldehyde at 4% final concentration and stored until analysis.
In the laboratory, macroalgae samples were strongly shaken during 2 min to detach epiphytic dinoflagellates. Macroalgae were separated from the seawater and the excess of water was removed with absorbent paper. Macroalgae were weighed and fixed in formaldehyde. A total of 400 samples were processed.
Environmental Variables
Water temperature was recorded every 3 h during the study period. HOBO Pro-v2 sensors were placed at the reef sites anchored at the level of the macroalgae mats. For nutrient determinations, water samples were collected with a 50 ml syringe, previously washed with a 10% HCl solution, connected to a filter holder containing a Whatman® membrane filter of 0.22 μm size pore and 2.5 mm diameter. Filtered seawater was recovered into a 30 ml Nalgene® polypropylene bottles that were stored in a cooler with ice. Once in the laboratory the samples were stored at −80°C. The quantification of NO (NO + NO) and PO was performed by using a Skalar® segmented flux autoanalyzer following the techniques described by Strickland and Parsons (1972) and Grasshoff et al. (1983).
Abundance and Identification of Epiphytic Dinoflagellates
The abundance of epiphytic dinoflagellates was estimated with the help of a Carl Zeiss inverted microscope model Axiovert 40 CFL at 10X magnification using a Sedgewick-Rafter counting chamber. Abundance is expressed in cells·g−1 of wet weight macroalgae (Reguera et al., 2016).
For dinoflagellate species identification the following specialized literature was used: Fukuyo (1981), Faust (1990, 1993, 1994, 1995, 1997, 1999), Faust et al. (1996), Litaker et al. (2009), and Almazán-Becerril et al. (2015, 2016).
Dinoflagellate observations and measurements were made with an Axio Imager A2 Zeiss microscope at 40X magnification using the differential interference contrast and epifluorescence techniques. Photographs of the specimens were taken with an AxioCam ICc1 digital camera coupled to the microscope. The image processing was carried out with the software Axiovision®.
Statistic Analysis
The non-parametric Mann–Whitney U-test (α = 0.05) was used to compare the dinoflagellate abundance at each sampling date between sites, and the non-parametric Wilcoxon rank test (α = 0.05) was used to evaluate the potential differences between sites during the whole period of sampling by paired data. The Spearman rank correlation was applied to describe the community structure on basis of the associations between species in both macroalgae. The association of temperature and nutrient concentration was assessed using the same correlation test.
Results
Dinoflagellate Species
We recorded species of the genera Gambierdiscus, Coolia, Prorocentrum, Ostreopsis, Amphidinium, Bysmatrum, and Sinophysis. Identification of the species of Gambierdiscus and Coolia is difficult based only on the characters observed (size and shape of the cells) during the evaluation of abundance. Therefore, hereafter the Gambierdiscus and Coolia specimens will be referred as Gambierdiscus spp. and Coolia spp. Five species of Ostreopsis were observed but only two of them accounted for more of the 95% of the abundance of this genus. These two morphotypes of Ostreopsis, were clearly differentiated during the counting and were designated as O. cf. marina and O. heptagona. Finally, six Prorocentrum species were found: P. belizeanum, P. emarginatum, P. hoffmannianum, P. lima. P. cf. levis, and P. rathymum. Gambierdiscus spp. Coolia spp., Ostreopsis cf. marina, O. heptagona, and the six Prorocentrum species were the most abundant groups found in the samples, therefore, the analysis was constrained to these taxa. Figure 2 shows the main species recorded in the zone of study.
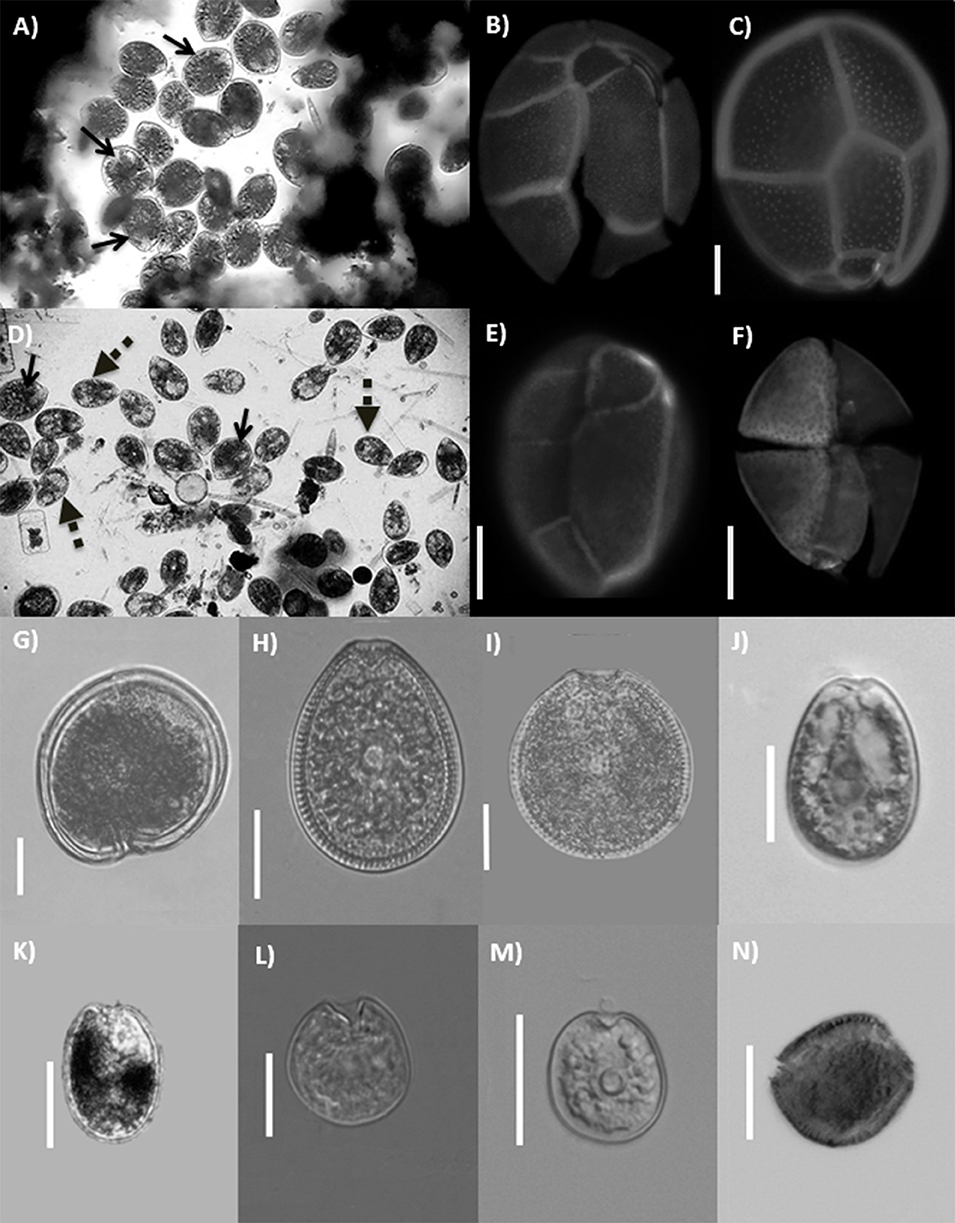
Figure 2. Species of benthic dinoflagellates found in the study zone. (A) Ostreopsis marina bloom from IC. (B) Epifluorescence images of O. marina epitheca and (C) hipotheca. (D) Ostreopsis heptagona bloom from PM. Note the presence of some cells of O. marina (arrows). (E) Epifluorescence images of O. heptagona epitheca and (F) hipotheca. (G) Gambierdiscus sp. (H) Prorocentrum hoffmannianum. (I) Prorocentrum belizeanum. (J) Prorocentrum lima. (K) Prorocentrum rathymum. (L) Prorocentrum emarginatum. (M) Prorocentrum cf. levis. (N) Coolia sp. Scale bar = 20μm.
Abundance Variability
Taking into account the entire sampling period, total abundance of epiphytic dinoflagellates was higher at PM on both algae (Tables 1, 2; Figure 3). However, when the total abundance and the abundance by group were compared at each sampling date, it became evident that the numeric differences were not always significant for all groups (Table 3). Total dinoflagellate abundances on Amphiroa were significantly higher at PM during all sampling dates (p < 0.05, Mann–Whitney test) except for Sep17 and Nov09 when no differences were detected. In Dictyota total abundances were higher in PM during the entire study period, except on Jun22 when no differences were detected.
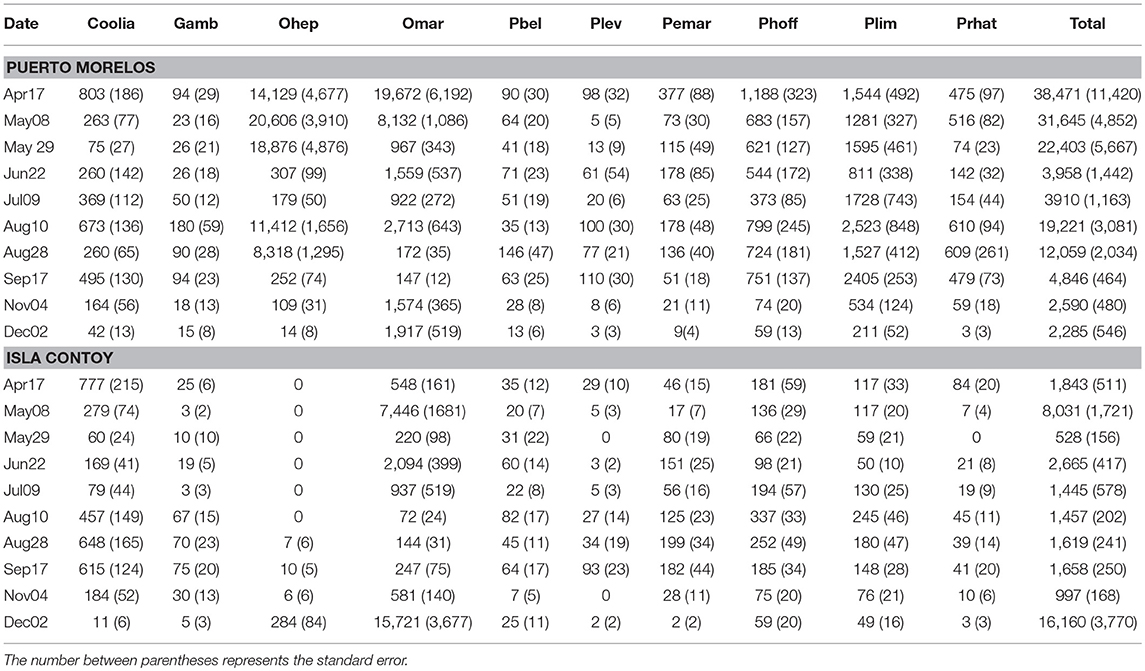
Table 1. Mean abundance (in cells·g−1) by date of the main taxonomic groups of benthic dinoflagellates on the macroalgae Dictyota.
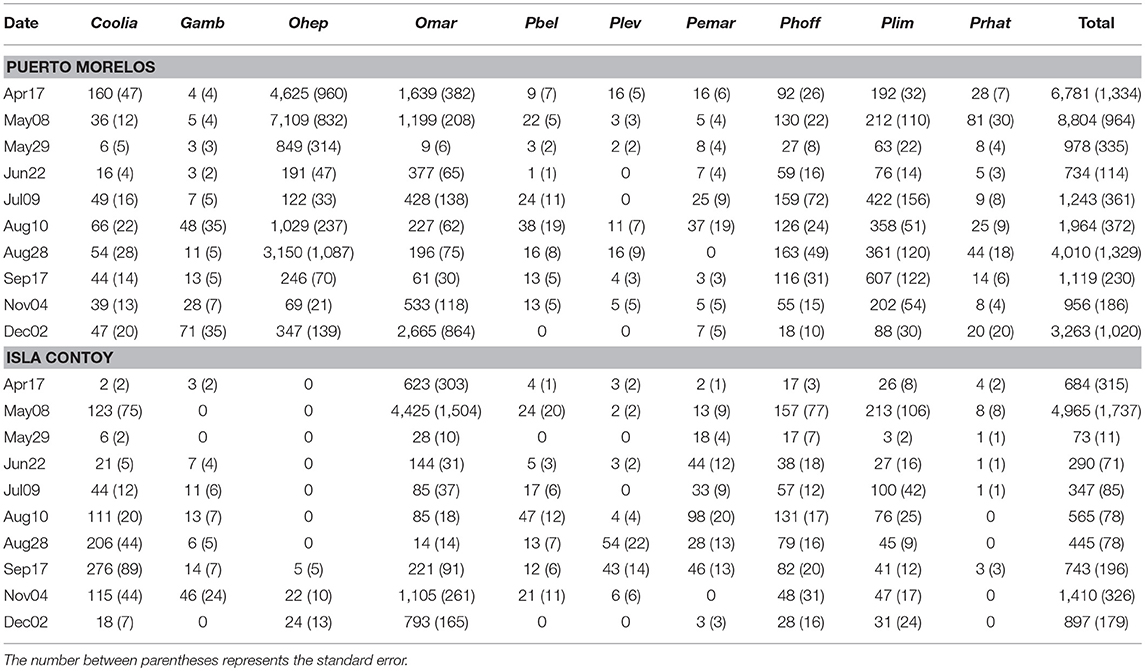
Table 2. Mean abundance (in cells·g−1) by date of the main taxonomic groups of benthic dinoflagellates on the macroalgae Amphiroa.
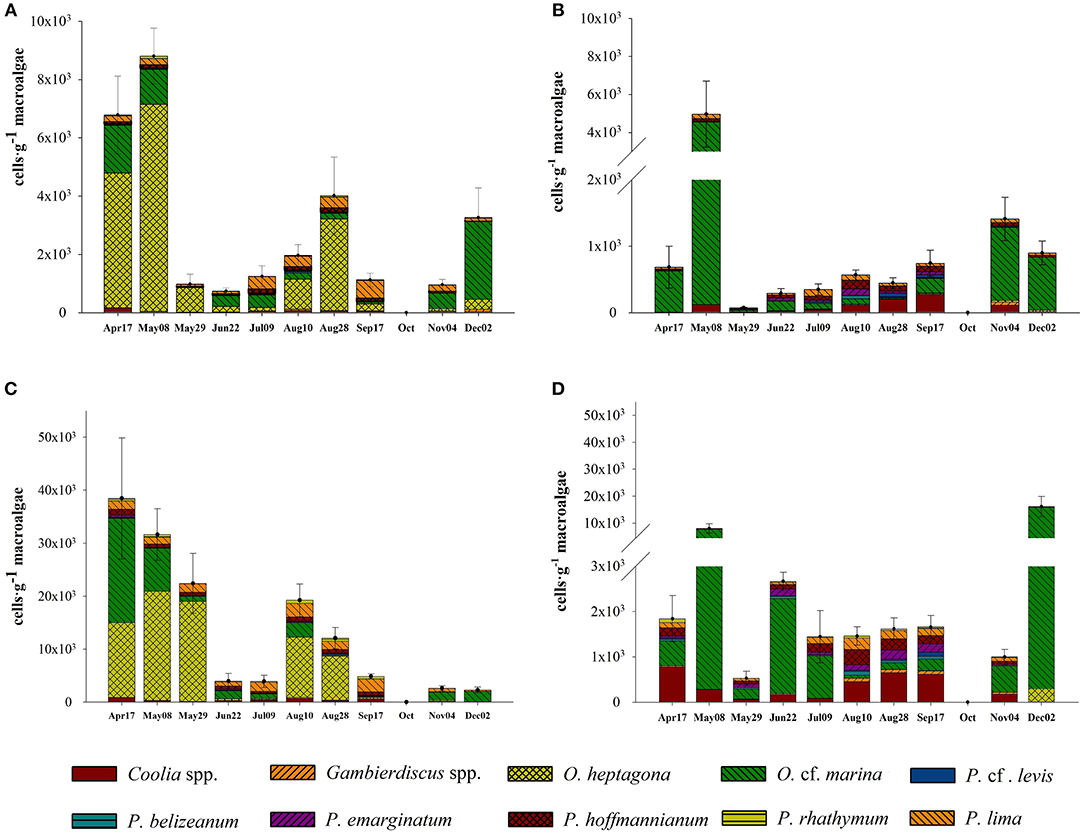
Figure 3. Variability of total benthic dinoflagellate abundance on (A) Amphiroa at Puerto Morelos and (B) Isla Contoy, and on Dictyota at (C) Puerto Morelos and (D) Isla Contoy.
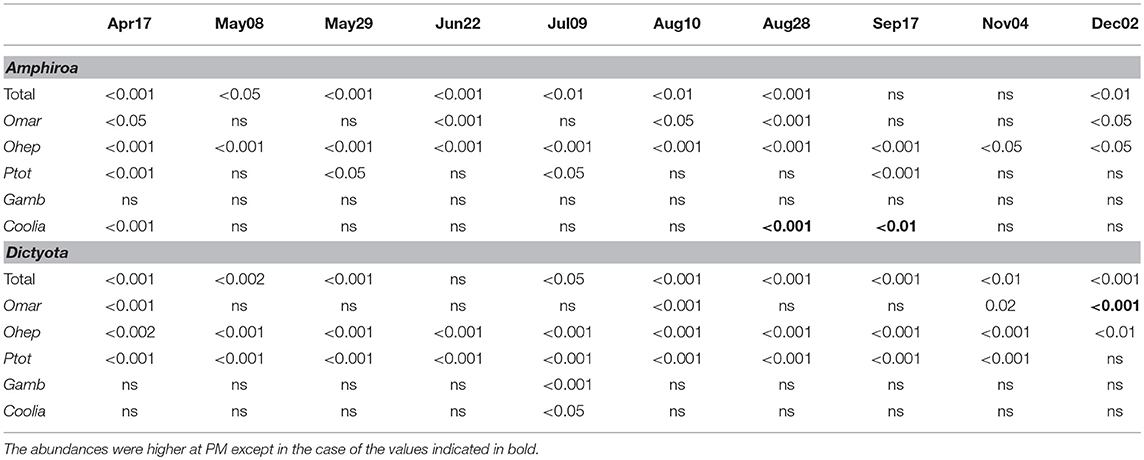
Table 3. P-values of the abundances comparisons of the main taxonomic groups of epiphytic dinoflagellates at each sampling date between sites (Mann–Whitney test, α = 5%).
In PM, the maximum mean abundance recorded in Dictyota was 38,471 ± 11,420 cells·g−1 on Apr16. In the case of Amphiroa, the maximum abundance was 8,803 ± 964 cells·g−1 and was recorded on May08. At IC the maximum density of dinoflagellates on Dictyota occurred on Dec02 (16,160 ± 3,770 cells·g−1), and on Amphiroa the highest abundance was 16,160 ± 3,770 cells·g−1 in May08. In PM, the maximum individual abundance was registered on Dictyota during Apr16 (112,293 cells·g−1).
Dominant species during most of the period were Ostreopsis heptagona and O. cf. marina Therefore, the variability of total abundance of epiphytic dinoflagellates were due the variation in the contribution of these species at each site. In fact, the contribution of Ostreopsis species varied linearly with total abundance (Figures 4A,B).
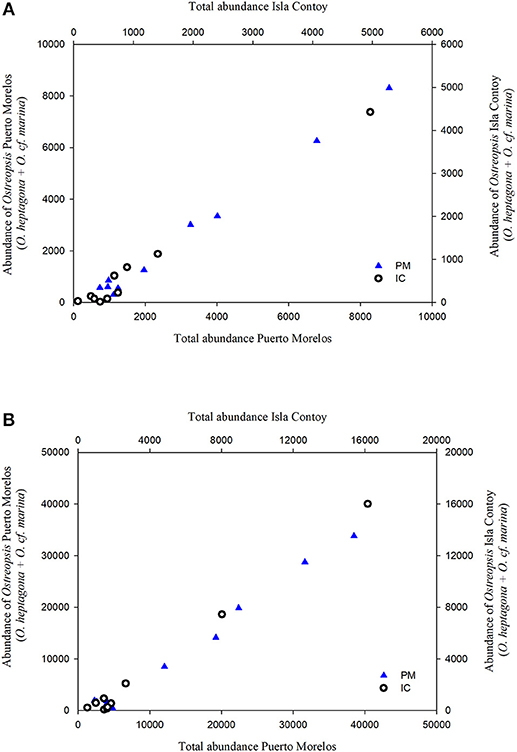
Figure 4. Relationship between the contribution of Ostreopsis species abundance in cells·g−1 (O. marina + O. heptagona) with total abundance on Amphiroa (A) and Dictyota (B).
The contribution of O. cf. marina was important at both sites, but O. heptagona was important only in PM because this species was absent at IC during almost the entire sampling period. O. cf. marina and O. heptagona reached maximum densities of 53,231 and ~46,462 cells·g−1 on a Dictyota sample during Apr16.
The contribution of Gambierdiscus spp. to total abundance was marginal on both macroalgae and at both sites: from 0 to 3.26% on Amphiroa, and from 0 to 4.6% on Dictyota. The highest abundance of this group on Dictyota was found at PM on Aug10 (178 ± 60 cells·g−1); and on Amphiroa the maximum abundance was observed on Dec02 (71 ± 38 cells·g−1).
The most abundant species of Prorocentrum were P. hoffmannianum and P. lima on both algae, representing the largest fraction of this genus (50–94%). Prorocentrum was important (65% of total abundance) when Ostreopsis abundances were low. Maximum abundance of all Prorocentrum species was registered at PM on Dictyota (4,144 cells·g−1).
The contribution of Coolia spp. to total abundance was lowest at PM. This genus did not present a contribution higher than 10% on both algae. In contrast, in IC on some dates it reached more than 30% on both macroalgae. The abundance of this group was lower than 1,000 cells·g−1on, and its abundance on Amphiroa was always lower than 300 cells·g−1.
Associations Between Species
There were more correlations between species on Dictyota than on Amphiroa in both sites (Table 4). Prorocentrum lima, P. belizeanum, and P. hoffmannianum were correlated with each other on Amphiroa at both sites but not on Dictyota. Also P. rathymum was correlated with O. heptagona on Amphiroa at PM. Given that this species was almost absent at IC, the correlation did not exist at this site. At Isla Contoy, P. hoffmannianum, and P. cf. levis were correlated with Coolia spp. The association between P. lima and P. hoffmannianum was the only association detected in both algae at both sites. On Dictyota, the community structure was more complex because it included the correlations of Coolia with Gambierdiscus, and each of these genera with the Prorocentrum species. The negative correlations of O. marina (with Gambierdiscus, P. emarginatum, and P. hoffmannianum) were particularly important, but this only occurred at IC.
Temperature and Nutrients
The temperature varied from 24.04 to 30.20°C at IC and from 26.68 to 29.7°C at PM. Warmer temperatures were registered between August and September, and April was the coldest month in both sites (Figure 5). Over the entire sampling period, mean water temperature was slightly lower at IC (28.25°C) than at PM (28.74°C). Although small, this difference was significant (p < 0.05, Wilcoxon test). On Apr17, the temperature was 1.46°C higher at PM (27.90°C) than IC (26.44°C). Over the rest of the sampling period, the temperature differences between sites were lower than 1°C. After May29, the temperature decreased almost 1°C at both sites, followed by a gradual increase until the maximum value in August–September. This behavior is common in the zone and is related to the influence of the polar fronts coming from the north from December to February, although during the year of study, polar fronts occurred until July. For example, the event which occurred during June 12–19 was intense enough to decrease the temperature recorded on Jun22.
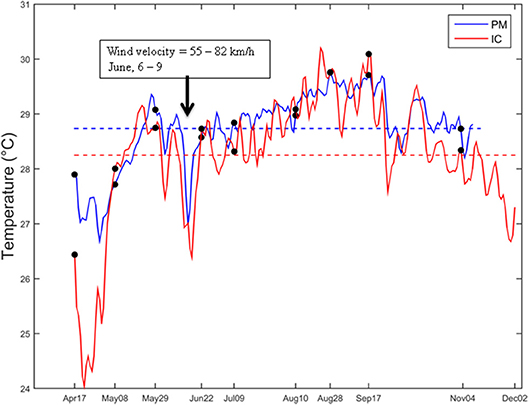
Figure 5. Variability of the water temperature at Puerto Morelos (blue line), and Isla Contoy (red line). Dotted lines indicate the mean water temperature of each series. The black arrow indicates a period of an intense polar front occurred in the zone during 6–9 June (winds 55–87 km/h). These events have the potential to remove a great fraction of macroalgae in the zone. Data of temperature were acquired every 3 h. Temperature series were filtered and binned to have only one data per day. Sensors were placed at the same depth (~1.5 m) in both sites to make data comparable.
High ( ) concentrations were measured on both sites with mean values of 8.0 and 7.8 μmol·l−1 for PM and IC, respectively, but no significant differences were detected along the entire period of study. Conversely, concentration was different between sites: 2.3 μmol·l−1 at IC and 2.4 μmol·l−1 in PM (p < 0.05, Wilcoxon test). and showed a high correlation at PM (r = 0.95) but not for IC. Temperature did not correlate with nutrients at PM nor IC (p > 0.05, Spearman rank correlation).
Effect of Environmental on Dinoflagellate Abundances
The correlation between temperature and the abundance of O. cf. marina is shown in Figure 6. At both sites and on both algae this species showed a negative correlation with temperature (p < 0.05, Spearman rank correlation). Maximum abundances were detected at temperatures between 27 and 28°C, independent of the macroalgae substrate. In contrast, Prorocentrum lima and P. emarginatum were positively correlated with temperature at IC and only on Dictyota: r = 0.66 and r = 0.79, respectively. A negative correlation between O. heptagona and concentration was found at PM on both algae (r = −0.67 and r = 0.7 on Amphiroa and Dictyota, respectively). Correlations with only were observed at IC with the abundances of P. emarginatum, P. rathymun, and Coolia spp. on Dictyota (Table 5).
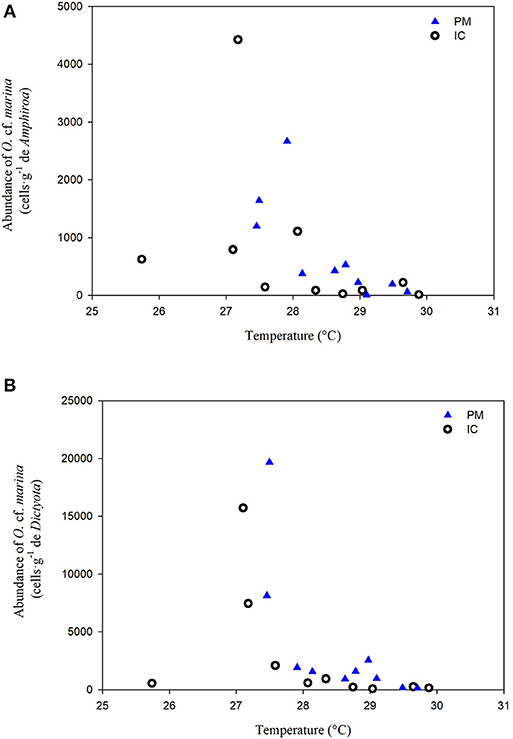
Figure 6. Relationship between Ostreopsis marina abundance and water temperature on (A) Amphiroa and (B) Dictyota. The highest abundance of this species occurred between 27–28°C in both sites on both macroalgae.
Discussion
Taxonomic Composition of Epiphytic Dinoflagellates
Describing the taxonomic composition of epiphytic dinoflagellates is more complicated now than in the past because at present there are more described species for each of the benthic genera. Pioneer studies on benthic dinoflagellates considered all specimens with the typical shape of Gambierdiscus to be G. toxicus, or all those individuals similar to Coolia as C. monotis. However, Faust (1995) and Litaker et al. (2009) increased to four the richness of the genus Gambierdiscus in the Caribbean: G. belizeanus, G. caribaeus, G. carolinianus, and G. carpenteri. Also the number of Coolia species increased after reports of the presence of C. tropicalis, C. santacroce, C. malayensis, and C. areolata (Faust, 1995; Karafas et al., 2015; Almazán-Becerril et al., 2016). Since the morphological identification of each of these species involves the inspection and analysis of the thecal plates, which is not a practical procedure given the high number of samples to analyse, it was necessary to join the species of each genus and consider them as taxonomic groups, although the ecological behavior of individual species of the same genus remain unclear.
Prorocentrum has the highest richness among the benthic-epiphytic thecate dinoflagellates, and the Caribbean harbors a great fraction of these species (Faust, 1990, 1993, 1994, 1997), however, just some of them are common in the macroalgae samples which allow an easier identification of the specimens. Prorocentrum lima, P. hofmmaniannum, P. belizeanum. P. rathymum, and P. emarginatum are well-recognized under light microscope as different species.
Ostreopsis taxonomy represents a challenge due to the lack of specific morphological characters for species discrimination (Penna et al., 2005; Parsons et al., 2012), however, in this study two Ostreopsis species were identified as O. heptagona and O. cf. marina. In the first case, the contact between the plates 4′ and 4″ (according to the interpretation of Besada et al., 1982) was an adequate characteristic to identify this species. The other species was referred as O. cf. marina because the original description of this species made by Faust (1999) matches with the characteristics of the specimens observed in this study. A closely related morphological species is O. lenticularis, also reported for the Caribbean (Ballantine et al., 1988; Moreira et al., 2012, 2017), which has nearly the same shape (Fukuyo, 1981; Faust, 1999). However, the size reported for this species in the literature is consistently lower than the size of the specimens observed in this study. Therefore, the discrimination of the specimens of these two species under microscope during counting was based on the size and shape of the specimens and was ratified by plate analysis of some cells in several samples. As consequence, the taxonomic composition of epiphytic dinoflagellates cannot be unequivocally determined by using the standard methodology (rough morphometric discrimination), and for purposes of practicality and comparability it is necessary to work with this “schematic” community.
The taxonomic composition of the epiphytic dinoflagellate community on the surveyed algae of this study is quite similar to those reported in similar studies in the Caribbean (Morton and Faust, 1997; Delgado et al., 2006; Boisnoir et al., 2018) and other regions of the North Atlantic like the south of the Gulf of Mexico (Okolodkov et al., 2007, 2014) or Florida (Bomber et al., 1989) and even in the Pacific (Parsons and Preskitt, 2007; Richlen and Lobel, 2011) although each species (or genus) did not contribute in the same proportion to the total abundance. For example, Delgado et al. (2006) found that P. lima and G. toxicus were more abundant than O. lenticularis and C. monotis, whereas Boisnoir et al. (2018) reported that Ostreopsis spp. and Prorocentrum spp. were numerically more abundant than Coolia and Gambierdiscus. In the Gulf of Mexico, Okolodkov et al. (2007) found that depending of the site and the date P. lima or C. monotis could dominate the community, and in the Yucatan coast, Prorocentrum species, particularly P. rathymum was the dominant species on seagrass meadows present in Yucatan coast (Okolodkov et al., 2014).
Abundance and Seasonal Patterns
The results of this study demonstrate that all the taxonomic groups were present on both algae at both localities, excepting O. heptagona which was almost absent at IC with only a few cells observed between September and December. As the distribution of this species comprises the Gulf of Mexico, Florida and the Caribbean (Bomber et al., 1989; Faust et al., 1996), its low abundance at IC during the period of study could be the effect of a local characteristic of the site, because at the same time a high number of specimens were detected at PM.
The short period of sampling of this study was not enough to determinate seasonal patterns for any species of dinoflagellate (although positive significant correlations were found between temperature and P. lima and P. emarginatum) and it appears that more time is necessary to characterize seasonal variability. For example, Ballantine et al. (1988) found evidence of seasonality in Gambierdiscus and O. lenticularis in Puerto Rico in a 3-year study. Also, Chinain et al. (1999) used a 5-year time series to describe the seasonality of Gambierdiscus spp. at Tahiti, and Chateau-Degat et al. (2005) used an 8-year study in French Polynesia to describe the seasonal behavior of Gambierdiscus. Higher abundances were observed during April and May 08 on both algae in PM, and in May 08 for both algae at IC, additionally the higher abundance in Dictyota at IC was detected on Dec02.
These fluctuations were dominated by the contribution of Ostreopsis species which was largely variable and increased linearly with total abundance. The high abundance of Ostreopsis species was also reported at the Caribbean in Cuba (Moreira et al., 2012, 2017) and Puerto Rico (Ballantine et al., 1988) where O. lenticularis reached higher abundances than the reported in this study (Table 6). When the abundance of Ostreopsis species was removed from the data, the contribution of Coolia spp., P. lima, and P. hoffmannianum were more important, while the abundance of Gambierdiscus spp. and the rest of Prorocentrum species was almost marginal. Prorocentrum lima is a conspicuous member of benthic dinoflagellate communities in the Caribbean and Gulf of Mexico where it attaches to a variety of algae and seagrasses and at some sites could be the dominant species with maximum abundances above 10,000 cells·g−1 on phaeophyte algae (Delgado et al., 2006), and up to 29,756 cells·g−1 of Thalassia testudinum (Okolodkov et al., 2007). Also, at Florida Keys, Bomber et al. (1989) found that P. lima was more abundant on green algae Penicillus capitatus and Avrainvillea nigricans as compared to other brown or red algae species.
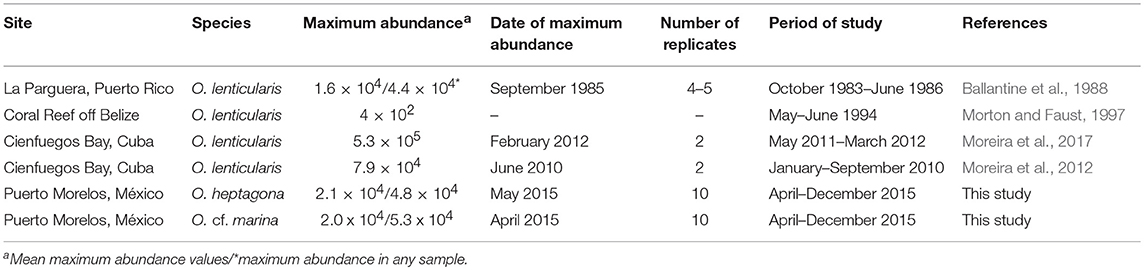
Table 6. Abundances from the literature of Ostreopsis species on the macroalgae Dictyota in the Caribbean basin.
The case of Gambierdiscus abundance is remarkable because the species of this genus and those of Fukuyoa are thought to be primarily responsible for the toxin production involved in ciguatera intoxications. Although there is an intrinsic difficulty in comparing abundances between different studies based on number of cells normalized by wet weight of macroalgae due to the high variability of weight to surface area ratios between the different groups of host macroalgae (Lobel et al., 1988), there are some reports of high abundances of Gambierdiscus spp. on Dictyota in the Caribbean. For example, Ballantine et al. (1988) reported a maximum abundance slightly higher than 2,000 cells·g−1 in Puerto Rico and Morton and Faust (1997) found 400 cells·g−1 in Belize. Also, Bomber et al. (1989) reported a maximum mean value of 8,191 ± 300 cells·g−1 in Florida. In contrast, the Gambierdiscus abundances found on Dictyota in this study were similar to the values reported by Lobel et al. (1988) at the Caribbean Island St. Barthélemy (5–56 cells·g−1). The factors that control the abundance of Gambierdiscus populations in the zone remain to be solved.
In the Caribbean high abundances of Ostreopsis lenticularis has been reported for Puerto Rico (Ballantine et al., 1988) and Cuba (Moreira et al., 2012, 2017), but no reports of high densities exist for O. marina and O. heptagona in this region. Table 5 shows that maximum densities recorded for Ostreopsis species in the Caribbean vary from 102 to 105 cells·g−1 in Dictyota. Indeed, the highest abundance was recorded in Cuba (531,000 cel·g−1) that is two times the maximum value reported by Ballantine et al. (1988) for the same species at Laurel Reef site, in Puerto Rico (235,803 cells·g−1). High abundances have been reported for O. siamensis (Shears and Ross, 2009) at New Zealand coasts (1.4 × 106 cells·g−1 of Carpophyllum plumosum) and for O. cf. ovata at the Mediterranean (Cohu et al., 2013) where the maximum abundance recorded was 8.54 × 106 cells·g−1 of Dictyota spp. These results demonstrate that at temperate and tropical latitudes Ostreopsis species can reach high abundances, emphasizing the potential of Ostreopsis species to produce large benthic blooms globally (Rhodes, 2011).
Community Structure on Dictyota and Amphiroa
In terms of abundance, both algae are used as substrate by all species. However, in terms of community structure, correlation between species can be used to know if the substrate offers common advantages for different species, or if the increase of one species implies the decrease of another. The results of this study suggest that the structure of the epiphytic dinoflagellate community is different on each of the host algae Amphiroa and Dictyota, and there are even differences in the same alga between sites. These findings suggest that Prorocentrum species and Coolia constitute the basic structure on Amphiroa, and on Dictyota the structure is given by Prorocentrum, Gambierdiscus and Coolia that share the habitat. In addition, O. marina presents habitat separation with Prorocentrum and Gambierdiscus. A negative correlation between Ostreopsis and Prorocentrum was reported by Richlen and Lobel (2011) in the Pacific, but Boisnoir et al. (2018) found the contrary in the Eastern Caribbean. Also, the habitat separation between Gambierdiscus and Ostreopsis has been reported by Bomber et al. (1989) in Florida, but in the Caribbean, Ballantine et al. (1988) did not find evidence of correlation between these genera. The dominance of Ostreopsis over the other genera of dinoflagellates could be related to its preference to sites with little water motion, as these are shallow sites (Richlen and Lobel, 2011; Cohu et al., 2013; Boisnoir et al., 2018)
The Effect of Environment on the Population Dynamics
The distance between study sites is nearly 50 km; both sites are shallow and located in the northern region of the Mesoamerican Barrier Reef System. Therefore it is reasonable to suppose that these sites also undergo the same climatological and oceanographic conditions, generating similar abundances and taxonomic compositions of dinoflagellate populations. However, the results showed some important differences. For example, the differences in temperature are low but significant and this could be explained by the climate patterns. The region presents three seasons: (1) the season influenced by polar fronts (locally called “nortes”) between December and February, (2) the dry season between March to May, and (3) the rainy season between June and November. The presence of a polar front can occur year-round (Henry, 1979), but its intensity is higher during winter. During 2015 the cold fronts were intense even during July (2015). Figure 5 shows the temperature series in the study sites. Both series have the same behavior but the magnitude of the variations is higher at IC than PM. The main effect of the polar fronts on the shallow aquatic ecosystems is the abrupt decrease of water temperature, but also the generation of strong movement of the surface layer of water. The energy of polar fronts decreases as they flow southward, therefore their effect on the coral reef systems should be higher at IC than PM. Since the energy of polar fronts removes host macroalgae, the turnover of dinoflagellate populations could be higher at IC, preventing their accumulation and resulting in higher abundances at PM. As the nutrient concentration remains high year-round, Dictyota can recover its biomass and abundance in short periods, providing surface for the attachment of Ostreopsis, which also grows rapidly (Ballantine et al., 1988). This implies that Dictyota and Ostreopsis are opportunistic genera exploiting an expanding habitat produced by the phase shift in the coral reefs.
Implications of Ostreopsis Blooms in the Caribbean Coral Reefs
The presence of Ostreopsis blooms on Dictyota along the Caribbean coasts has some ecological implications. First, toxicity has been reported in populations of O. lenticularis from Cuba and Puerto Rico (Ballantine et al., 1988; Moreira et al., 2012), as well as O. heptagona (Norris et al., 1985); in addition, some samples of O. cf. marina taken after the sampling period of this study showed toxicity on mouse bioassays and hemolytic activity (unpublished data). In fact, Ostreopsis species produce palytoxin-like compounds which are involved in human intoxications and possibly mass mortality of vertebrates and invertebrates (Ramos and Vasconcelos, 2010; Faimali et al., 2012; Patocka et al., 2017). Secondly, Dictyota is a macroalgae favored by the changes in the coral reef systems. In the sites studied these algae can form mats of several tens of square meters, at times reaching nearly 80% of cover (Delgado-Pech, 2016). However, the low palatability of Dictyota to larger benthic herbivores reduces grazing (Cruz-Rivera and Villareal, 2006) and prevents the flux of toxins to higher trophic levels. Dictyota mats are persistent, growing on other calcareous and coralline algae, corals and any other hard surface. Still, their attachment to the substrate is not firm enough to avoid detachment by the strong waves formed under the influence of polar fronts. During these events, the cover of Dictyota and the abundance of Ostreopsis decrease in the benthic environment, although, in the case of Ostreopsis, the cells (and their toxins) could remain in the water column and possibly enter the planktonic food web. If the coral-algal phase shift in the Caribbean results in dominance of Dictyota and other fleshy macroalgae, toxic Ostreopsis species could increase their abundance and distribution and cases of ciguatera and other syndrome of benthic origin could become a more serious public health problem in the region.
Conclusions
The taxonomic composition of epiphytic dinoflagellates inhabiting the macroalgae from the northern of the Mesoamerican Coral Reef Barrier was comprised of species of the genera Ostreopsis, Prorocentrum, Coolia, and Gambierdiscus.
Ostreopsis was the dominant genus in the sites studied. Particularly, O. cf. marina and O. heptagona reached maximum densities of 53,000 and 48,000 cells·g−1 on Dictyota. However, the period of sampling was not enough to detect seasonal trends of the dinoflagellate species.
Total abundances were higher in PM on both algae. These differences could be attributed to the intensity of environmental variables. For example, the lower temperature and the higher effect of polar fronts in IC could prevent the accumulation of epiphytic dinoflagellates.
O. marina showed a negative correlation with temperature between 27 and 30°C. On the contrary P. lima and P. emarginatum showed a positive correlation with temperature at IC on both macroalgae.
Nutrient concentrations and were higher in both sites during the whole period of study, but in the most of the cases their concentration was not correlated with the abundance of dinoflagellates, excepting O. heptagona which showed a negative correlation with concentration. On the contrary, P. emarginatum, P. rathymum, and Coolia spp. were positively correlated whit on Dictyota at IC.
The structure of epiphytic dinoflagellates was different between algae. There were more correlations between species on Dictyota than Amphiroa. The association between P. lima and P. hoffmannianum was the only one detected in both algae and in both sites.
The high cover of Dictyota in the study zone and the high abundance of O. cf. marina and O. heptagona on this macroalgae could represent a risk for human and marine fauna health if production of toxins is confirmed in this species.
The report of Ostreopsis blooms in the Mexican Caribbean coasts and the reports of blooms of this genus in other sites along the Caribbean basin emphasize the importance of this genus worldwide.
Author Contributions
AA-B conceived and designed the study, wrote, and edited the document. EI-S and BD-P collected data, processed samples, wrote and edited the document, and built the figures and tables. EG-M and EN-V interpreted results, wrote, and edited the document. AO-O quantified the nutrient concentrations, interpreted the results, and wrote and edited the document.
Funding
CONACyT Fellowship number 308153. CONANP, Convenio PROMOBI/PNAPM/01/2015. CONABIO, Project MQ001. Red Temática sobre Florecimientos Algales Nocivos (RedFAN) CONACyT 2015-2017 projects.
Conflict of Interest Statement
The authors declare that the research was conducted in the absence of any commercial or financial relationships that could be construed as a potential conflict of interest.
Acknowledgments
We thank to the National Protected Areas Comission (CONANP), specifically to the Parque Nacional Arrecife de Puerto Morelos and Parque Nacional Isla Contoy, and particularly, to the director of the areas Dra. Mary Carmen García Rivas. EI-S and BD-P thank to the Posgrado en Ciencias del Agua of CICY and to the RedFAN of CONACyT. Finally we thanks to Gabriela Resendiz for the figures of the study zone and temperature series, Jennifer Méndez for the epifluorescence images and to Eva Kozak for her support with english language.
References
Almazán-Becerril, A., Escobar-Morales, S., Irola-Sansores, E. D., and Delgado-Pech, B. (2016). “Morfología y taxonomía de los géneros Ostreopsis y Coolia en el Caribe Mexicano,” in Florecimientos Algales Nocivos en México, eds E. García-Mendoza, S. Quijano-Scheggia, A. Olivos-Ortiz, and E. J. Núñez-Vázquez (Ensenada: CICESE), 378–393.
Almazán-Becerril, A., Escobar-Morales, S., Rosiles-González, G., and Valadez, F. (2015). Benthic-epiphytic dinoflagellates from the northern portion of the Mesoamerican Reef System. Bot. Mar. 58, 115–128. doi: 10.1515/bot-2014-0093
Anderson, D. M., Burkholder, J. M., Cochlan, W. P., Glibert, P. M., Gobler, C. J., Heil, C. A., et al. (2008). Harmful algal blooms and eutrophication: examining linkages from selected coastal regions of the United States. Harmful Algae 8, 39–53. doi: 10.1016/j.hal.2008.08.017
Arcila-Herrera, H., Castello-Navarrete, A., Mendoza-Ayora, J., Montero-Cervantes, L., González-Franco, M. F., and Brito-Villanueva, W. O. (1998). Ten cases of ciguatera fish poisoning in Yucatan. Rev. Inv. Clin. 50, 149–152.
Ballantine, D. L., Tosteson, T. R., and Bardales, A. T. (1988). Population dynamics and toxicity of natural populations of benthic dinoflagellates in southwestern Puerto Rico. J. Exp. Mar. Biol. Ecol. 119, 201–212. doi: 10.1016/0022-0981(88)90193-1
Besada, E. G., Loeblich, L. A., and Loeblich, A. R. III. (1982). Observations on tropical, benthic dinoflagellates from ciguatera-endemic areas: Coolia, Gambierdiscus, and Ostreopsis. Bull. Mar. Sci. 32, 723–735.
Björk, M., Mohammed, S. M., Björklund, M., and Smesi, A. (1995). Coralline algae, important coral-reef builders threatened by pollution. Ambio 21, 502–505.
Boisnoir, A., Pascal, P. Y., Cordonnier, S., and Lemée, R. (2018). Depth distribution of benthic dinoflagellates in the Caribbean Sea. J. Sea Res. 135, 74–83. doi: 10.1016/j.seares.2018.02.001
Bomber, J. W., Guillard, R. R. L., and Nelson, W. G. (1988). Roles of temperature, salinity, and light in seasonality, growth, and toxicity of ciguatera causing Gambierdiscus toxicus Adachi et Fukuyo (Dinophyceae). J. Exp. Mar. Biol. Ecol. 115, 53–65. doi: 10.1016/0022-0981(88)90189-X
Bomber, J. W., Rubio, M. G., and Norris, D. R. (1989). Epiphytism of dinoflagellates associated with the disease ciguatera: substrate specificity and nutrition. Phycologia 28, 360–368. doi: 10.2216/i0031-8884-28-3-360.1
Chateau-Degat, M. L., Chinain, M., Cerf, N., Gingras, S., Hubert, B., and Dewailly, E. (2005). Seawater temperature, Gambierdiscus spp. variability and incidence of ciguatera poisoning in French Polynesia. Harmful Algae 4, 1053–1062. doi: 10.1016/j.hal.2005.03.003
Chinain, M., Germain, M., Paulliac, S., and Legrand, A.-M. (1999). Seasonal abundance and toxicity of the dinoflagellate Gambierdiscus spp. (Dinophyceae), the causative agent of ciguatera in Tahiti French Polynesia. Mar. Biol. 135, 259–267. doi: 10.1007/s002270050623
Cohu, S., Mangialajo, L., Thibaut, T., Blanfuné, A., Marro, S., and Lemée, R. (2013). Proliferation of the toxic dinoflagellate Ostreopsis cf. ovata in relation to depth, biotic substrate and environmental factors in the North West Mediterranean Sea. Harmful Algae 24, 32–44. doi: 10.1016/j.hal.2013.01.002
Cruz-Rivera, E., and Villareal, T. A. (2006). Macroalgal palatibility and the flux of ciguatera toxins through marine food webs. Harmful Algae 5, 497–525. doi: 10.1016/j.hal.2005.09.003
Delgado, G., Lechuga-Devéze, C. H., Popowski, G., Troccoli, L., and Salinas, C. A. (2006). Epiphytic dinoflagellates associated with ciguatera in the northwestern coast of Cuba. Rev. Biol. Trop. 54, 299–310. doi: 10.15517/rbt.v54i2.13870
Delgado-Pech, B. (2016). Comunidades de Macroalgas en Arrecifes Coralinos de Quintana Roo: Composición Taxonómica y Patrones de Colonización. Master's thesis, Centro de Investigación Científica de Yucatán, Cancún.
Faimali, M., Giussani, V., Piazza, V., Garaventa, F., Corrà, C., Asnaghi, V., et al. (2012). Toxic effects of harmful benthic dinoflagellate Ostreopsis ovata on invertebrate and vertebrate marine organisms. Mar. Environ. Res. 76, 97–107. doi: 10.1016/j.marenvres.2011.09.010
Faust, M. A. (1990). Morphologic details of six benthic species of Prorocentrum (Pyrrophyta) from a mangrove island, Twin Cays, Belize, including two new species. J. Phycol. 26, 548–558. doi: 10.1111/j.0022-3646.1990.00548.x
Faust, M. A. (1993). Three new benthic species of Prorocentrum (Dinophyceae) from Twin Cays, Belize: P. maculosum sp. nov., P. foraminosum sp. nov., and P. formosum sp. nov. Phycologia 32, 410–418. doi: 10.2216/i0031-8884-32-6-410.1
Faust, M. A. (1994). Three new benthic species of Prorocentrum (dinophyceae) from Carrie Bow Cay, Belize: P. sabulosum sp. nov., P. sculptile sp. nov., and P. arenarium sp. nov. J. Phycol. 30, 755–763. doi: 10.1111/j.0022-3646.1994.00755.x
Faust, M. A. (1995). Observation of sand-dwelling toxic dinoflagellates (Dinophyceae) from widely differing sites, including two new species. J. Phycol. 31, 996–1003. doi: 10.1111/j.0022-3646.1995.00996.x
Faust, M. A. (1997). Three new benthic species of Prorocentrum (dinophyceae) from Belize: P. norrisianum sp. nov., P. tropicalis sp. nov., and P. reticulatum sp. nov. J. Phycol. 33, 851–858. doi: 10.1111/j.0022-3646.1997.00851.x
Faust, M. A. (1999). Three new Ostreopsis species (Dinophyceae): O. marina sp. nov., O. belizeanus sp. nov., and O. caribbeanus sp. nov. Phycologia 38, 92–99. doi: 10.2216/i0031-8884-38-2-92.1
Faust, M. A., Morton, S. L., and Quod, J. P. (1996). Further SEM study of marine dinoflagellates: the genus Ostreopsis (Dinophyceae). J. Phycol. 32, 1053–1065 doi: 10.1111/j.0022-3646.1996.01053.x
Fukuyo, Y. (1981). Taxonomical study on benthic dinoflagellates collected in coral reefs. Bull. Jpn. Soc. Sci. Fish. 47, 967–978. doi: 10.2331/suisan.47.967
Gingold, B. D., Strickland, M. J., and Hess, J. J. (2014). Ciguatera fish poisoning and climate change: analysis of national poison data in the United States, 2001-2011. Environ. Health Perspect. 122, 580–586. doi: 10.1289/ehp.1307196
Grasshoff, K., Ehrhardt, M., and Kremling, K. (1983). Methods of Seawater Analysis, 2nd Edn. Weinheim: Verlag Chemie.
Hales, S., Weinstein, P., and Woodward, A. (1999). Ciguatera (fish poisoning), El Niño, and Pacific sea surface temperatures. Ecosyst. Health 5, 20–25. doi: 10.1046/j.1526-0992.1999.09903.x
Henry, W. K. (1979). Some aspects of the fate of cold fronts in the Gulf of Mexico. Mon. Weather Rev. 107, 1078–1082.
Hernandez-Becerril, D. U., and Almazan-Becerril, A. (2004). El género Gambierdiscus (Dinophyceae) en el Caribe Mexicano. Rev. Biol. Trop. 52, 77–87. doi: 10.15517/RBT.V52I1.26521
Hoegh-Guldberg, O., Mumby, P. J., Hooten, A. J., Steneck, R. S., Greenfield, P., Gomez, E., et al. (2007). Coral reefs under rapid climate change and ocean acidification. Science 318, 1737–1742. doi: 10.1126/science.1152509
Karafas, S., York, R., and Tomas, C. (2015). Morphological and genetic analysis of the Coolia monotis species complex with the introduction of two new species, Coolia santacroce sp. nov. and Coolia palmyrensis sp. nov. (Dinophyceae). Harmful Algae 46, 18–33. doi: 10.1016/j.hal.2015.05.002
Kibler, S. R., Tester, P. A., Moore, S. K., and Litaker, R. W. (2015). Effects of ocean warming on growth and distribution of dinoflagellates associated with ciguatera fish poisoning in the Caribbean. Ecol. Model. 316, 194–2010. doi: 10.1016/j.ecolmodel.2015.08.020
Kohler, S. T., and Kohler, C. C. (1992). Dead bleached coral provides new surfaces for dinoflagellates implicated in ciguatera fish poisonings. Environ. Biol. Fish. 35, 413–416 doi: 10.1007/BF00004993
Lartigue, J., Jester, E. L., Dickey, R. W., and Villareal, T. A. (2009). Nitrogen source effects on the growth and toxicity of two strains of the ciguatera-causing dinoflagellate Gambierdiscus toxicus. Harmful Algae 8, 781–791. doi: 10.1016/j.hal.2008.05.006
Litaker, R. W., Vandersea, M. W., Faust, M. A., Kibler, S. R., Chinain, M., Holmes, M. J., et al. (2009). Taxonomy of Gambierdiscus including four new species, Gambierdiscus caribaeus, Gambierdiscus carolinianus, Gambierdiscus carpenteri and Gambierdiscus ruetzleri (Gonyaulacales, Dinophyceae). Phycologia 48, 344–390. doi: 10.2216/07-15.1
Littler, M. M., Littler, D. S., and Taylor, P. R. (1983). Evolutionary strategies in a tropical barrier reef system: functional-form groups of marine macroalgae. J. Phycol. 19, 229–237. doi: 10.1111/j.0022-3646.1983.00229.x
Lobel, P. S., Anderson, D. M., and Durand-Clement, M. (1988). Assessment of ciguatera dinoflagellate populations: sample variability and algal substrate selection. Biol. Bull. 175, 94–101. doi: 10.2307/1541896
McCook, L. J. (1999). Macroalgae, nutrients and phase shifts on coral reefs: scientific issues and management consequences for the Great Barrier Reef. Coral Reefs 18, 357–367. doi: 10.1007/s003380050213
Moreira, A., Fernandes, L., Escarrá, R., Díaz, L., Rodríguez, F., Riobo, P., et al. (2017). “Notes on morphology, phylogeny and toxicity of a dominant community of toxic benthic dinoflagellates from southern-central coast of Cuba,” in Marine and Freshwater Harmful Algae. Proceedings of the 17th International Conference on Harmful Algae, eds L. O. A. Proença and M. Hallegraeff (Florianópolis: International Society for the Study of Harmful Algae 2017), 88–91.
Moreira, A., Rodríguez, F., Riobó, P., Franco, J. M., Martínez, N., Chamero, D., et al. (2012). Notes on Ostreopsis sp. from southern-central coast of Cuba. Cryptol. Algol. 33, 217–224. doi: 10.7872/crya.v33.iss2.2011.217
Morton, S. L., and Faust, M. A. (1997). Survey of toxic epiphytic dinoflagellates from the Belizean barrier reef ecosystem. Bull. Mar. Sci. 61, 899–906.
Nakahara, H., Sakami, T., Chinain, M., and Ishida, Y. (1996). The role of macroalgae in epiphytism of the toxic dinoflagellate Gambierdiscus toxicus (Dinophyceae). Phycol. Res. 44, 113–117. doi: 10.1111/j.1440-1835.1996.tb00385.x
Norris, D. R., Bomber, J. W., and Balech, E. (1985). “Benthic dinoflagellates associated with ciguatera from the Florida Keys. I. Ostreopsis heptagona sp. nov.,” in Toxic Dinoflagellates, eds D. M. Anderson, A. W. White, and D. G. Baden (New York, NY: Elsevier Scientific), 39–44.
Okolodkov, Y. B., Campos-Bautista, G., Gárate-Lizárraga, I., González-González, J. A. G., Hoppenrath, M., and Arenas, V. (2007). Seasonal changes of benthic and epiphytic dinoflagellates in the Veracruz reef zone, Gulf of Mexico. Aquat. Microb. Ecol. 47, 223–237. doi: 10.3354/ame047223
Okolodkov, Y. B., Virgilio, F. D. C. M., Castillo, J. A. A., Trujillo, A. C. A., Espinosa-Matías, S., and Silveira, A. H. (2014). Seasonal changes in epiphytic dinoflagellate assemblages near the northern coast of the Yucatan Peninsula, Gulf of Mexico. Acta Bot. Mex. 107, 121–151. doi: 10.21829/abm107.2014.204
Parsons, M. L., Aligizaki, K., Bottein, M. Y. D., Fraga, S., Morton, S. L., Penna, A., et al. (2012). Gambierdiscus and Ostreopsis: Reassessment of the state of knowledge of their taxonomy, geography, ecophysiology and toxicology. Harmful Algae 14, 107–129. doi: 10.1016/j.hal.2011.10.017
Parsons, M. L., and Preskitt, L. B. (2007). A survey of epiphytic dinoflagellates from the coastal waters of the island of Hawaii. Harmful Algae 6, 658–669. doi: 10.1016/j.hal.2007.01.001
Parsons, M. L., Settlemier, C. J., and Ballauer, J. M. (2011). An examination of the epiphytic nature of Gambierdiscus toxicus, a dinoflagellate involved in ciguatera fish poisoning. Harmful Algae 10, 598–605. doi: 10.1016/j.hal.2011.04.011
Patocka, J., Nepovimova, E., Wu, Q., and Kuka, K. (2017). Palytoxin congeners. Arch. Toxicol. 92, 143–156. doi: 10.1007/s00204-017-2105-8
Penna, A., Vila, M., Fraga, S., Giacobbe, M. G., Andreoni, F., Riobó, P., et al. (2005). Characterization of Ostreopsis and Coolia (dinophyceae) isolates in the western Mediterranean Sea based on morphology, toxicity and internal transcribed spacer 5.8 s rDNA sequences. J. Phycol. 41, 212–225. doi: 10.1111/j.1529-8817.2005.04011.x
Rains, L. K., and Parsons, M. L. (2015). Gambierdiscus species exhibit different epiphytic behaviors toward a variety of macroalgal hosts. Harmful Algae 49, 29–39. doi: 10.1016/j.hal.2015.08.005
Ramos, V., and Vasconcelos, V. (2010). Review: palytoxin and analogs: biological and ecological effects. Mar. Drugs 8, 2021–2037 doi: 10.3390/md8072021
Reguera, B., Alonso, R., Moreira, A., Méndez, S., and Dechraoui-Bottein, M. Y. (2016). Guide for Designing and Implementing a Plan to Monitor Toxin-Producing Microalgae, 2nd Edn. IOC Manuals and Guides, No. 59. Paris; Vienna: Intergovernmental Oceanographic Commission (IOC) of United Nations Educational Scientific and Cultural Organization (UNESCO) and International Atomic Energy Agency (IAEA).
Rhodes, L. (2011). World-wide occurrence of the toxic dinoflagellates genus Ostreopsis Schmidt. Toxicon 3, 400–407. doi: 10.1016/j.toxicon.2010.05.010
Richlen, M. L., and Lobel, P. S. (2011). Effects of depth, habitat, and water motion on the abundance and distribution of ciguatera dinoflagellates at Johnston Atoll, Pacific Ocean. Mar. Ecol. Prog. Ser. 421, 51–66. doi: 10.3354/meps08854
Shears, N. T., and Ross, P. M. (2009). Blooms of benthic dinoflagellates of the genus Ostreopsis; an increasing and ecologically important phenomenon on temperate reefs in New Zealand and worldwide. Harmful Algae 8, 916–925. doi: 10.1016/j.hal.2009.05.003
Steneck, R. S., and Deither, M. N. (1994). A functional group approach to the structure of algal-dominated communities. Oikos 69, 476–498. doi: 10.2307/3545860
Strickland, J. D., and Parsons, T. R. (1972). A Practical Handbook of Seawater Analysis. Ottawa, ON: Fisheries Research Board of Canada.
Tester, P. A., Feldman, R. L., Nau, A. W., Kibler, S. R., and Litaker, W. R. (2010). Ciguatera fish poisoning and sea surface temperatures in the Caribbean Sea and the West Indies. Toxicon 56, 698–710. doi: 10.1016/j.toxicon.2010.02.026
Tosteson, T. R. (2004). Caribbean ciguatera: a changing paradigm. Rev. Biol. Trop. 52, 109–113. doi: 10.15517/RBT.V52I1.26524
Turquet, J., Quod, J. P., Ten-Hage, L., Dahalani, Y., and Wendling, B. (2001). “Example of a Gambierdiscus toxicus flare-up following the 1998 coral bleaching event in Mayotte Island (Comoros, southwest Indian Ocean),” in Harmful Algal Blooms 2000, eds G. M. Hallegraeff, S. I. Blackburn, C. J. Bolch, and R. J. Lewis (Hobart, TAS: Intergovernmental Oceanographic Commission of UNESCO), 50–53
Vila, M., Garcés, E., and Masó, M. (2001). Potentially toxic epiphytic dinoflagellate assemblages on macroalgae in the NW Mediterranean. Aquat. Microb. Ecol. 26, 51–60. doi: 10.3354/ame026051
Yasumoto, T., Inoue, A., Ochi, T., Fujimoto, K., Oshima, Y., Fukuyo, Y., et al. (1980). Environmental studies on a toxic dinoflagellate responsible for ciguatera. Bull. Jpn. Soc. Sci. Fish. 46, 1397–1404. doi: 10.2331/suisan.46.1397
Keywords: Ostreopsis, Dictyota, ciguatera, Caribbean Sea, coral reef
Citation: Irola-Sansores ED, Delgado-Pech B, García-Mendoza E, Núñez-Vázquez EJ, Olivos-Ortiz A and Almazán-Becerril A (2018) Population Dynamics of Benthic-Epiphytic Dinoflagellates on Two Macroalgae From Coral Reef Systems of the Northern Mexican Caribbean. Front. Mar. Sci. 5:487. doi: 10.3389/fmars.2018.00487
Received: 03 September 2018; Accepted: 30 November 2018;
Published: 18 December 2018.
Edited by:
Jorge I. Mardones, Instituto de Fomento Pesquero (IFOP), ChileReviewed by:
Sai Elangovan S, National Institute of Oceanography (CSIR), IndiaConrad Sparks, Cape Peninsula University of Technology, South Africa
Copyright © 2018 Irola-Sansores, Delgado-Pech, García-Mendoza, Núñez-Vázquez, Olivos-Ortiz and Almazán-Becerril. This is an open-access article distributed under the terms of the Creative Commons Attribution License (CC BY). The use, distribution or reproduction in other forums is permitted, provided the original author(s) and the copyright owner(s) are credited and that the original publication in this journal is cited, in accordance with accepted academic practice. No use, distribution or reproduction is permitted which does not comply with these terms.
*Correspondence: Antonio Almazán-Becerril, YWxtYXphbkBjaWN5Lm14