- 1School of Zoology, The George S. Wise Faculty of Life Sciences, Tel-Aviv University, Tel-Aviv, Israel
- 2The Interuniversity Institute for Marine Sciences in Eilat, Eilat, Israel
Mesophotic coral-reef ecosystems (MCEs) are light-dependent communities occurring at 30 to ∼150 m depth in the clear waters of tropical and sub-tropical regions. The extent to which MCEs and shallow benthic populations are connected, and whether coral sexual reproduction is maintained under the mesophotic environmental conditions, are still unclear. Despite the trend of increasing studies concerning the MCEs, the reproductive traits of MCE Indo-Pacific octocorals have remained uninvestigated to date. The current study engages with two common zooxanthellate octocorals in the northern Red Sea: the internal-brooder Ovabunda spp. and the surface-brooder Rhytisma fulvum fulvum. It addresses the hypothesis that, similar to their shallow-zone conspecifics, the upper mesophotic populations also reproduce sexually. We analyze the reproductive traits of tropical, upper mesophotic octocoral populations and compare them for the first time to the traits of their conspecifics on shallow reefs. In addition, we discuss the timing of surface-brooding events in relation to lunar phase and daily seawater temperature. The populations of Ovabunda spp. in the upper MCE and shallow-water display several similar reproductive traits (e.g., maximal gonad diameter and fecundity). Consequently, this species seems to be a successful depth-generalist. In contrast, the reproductive features of R. f. fulvum differ between the reefs in the upper MCE and in those shallow-water, with a decreased abundance of female colonies, lower fecundity, and a lower percentage of colonies exhibiting surface-brooding in the former. It thus seems that the R. f. fulvum population encounters certain constraints in the MCE that may, in turn, lead to its reduced reproductive performance there. In addition, surface-brooding events for R. f. fulvum in the upper-mesophotic zone and in shallow-water were separated by two weeks. Since a distinctive seawater temperature rise preceded each surface-brooding event, temperature regimes at different depths may present extrinsic cues that influence the timing of reproduction. The findings from this study contribute to our understanding of the life-history of mesophotic benthic inhabitants in general and of octocorals in particular. Overall, the current findings reinforce the viewpoint that the refuge potential of MCEs may be reflective of species-specific reproductive traits.
Introduction
Mesophotic coral-reef ecosystems (MCEs) have been defined as warm or temperate water, light-dependent communities, comprising corals and other organisms that occur at depths below 30 m and above 150 m (Kahng et al., 2010). It has been suggested that MCEs can be divided into two distinct zones: an upper mesophotic one, between 30–60 m; and a lower one, below 60 m (Lesser et al., 2010; Brazeau et al., 2013; Bongaerts et al., 2015). It has also been broadly suggested that deeper reefs are less affected by direct climate-induced disturbances compared to shallow reefs (Glynn, 1996; Hughes and Tanner, 2000; Bak et al., 2005; Laverick and Rogers, 2017; Baird et al., 2018) and, therefore, the occurrence of thriving MCE communities has led to the “deep-reef refuge hypothesis” (DRRH: Bongaerts et al., 2010, 2019). It is hypothesized that MCEs may function as a refuge for certain organisms during periods of stress in the shallow waters and, in the aftermath, act as a potential source of propagules for recovery of the disturbed shallow communities (e.g., Kahng et al., 2014; Bongaerts et al., 2017).
The sexual reproduction of MCE stony corals has gained increasing interest recently (e.g., Holstein et al., 2015, 2016; Eyal-Shaham et al., 2016; Prasetia et al., 2016, 2017; Feldman et al., 2018; Shlesinger et al., 2018). To date, around 10 stony coral species have been reported as manifesting a decreased reproductive performance with depth (e.g., Shlesinger et al., 2018). The reproductive output of Stylophora pistillata in the Red Sea, for example, decreases with depth, shedding 5–20-fold fewer planulae in the MCE, and also displaying a shorter gametogenic cycle than its conspecific shallow population (Rinkevich and Loya, 1987). Similarly, Seriatopora hystrix in the Okinawan MCE (Japan) produces smaller planulae and features a shorter gametogenic cycle with increasing depth (Prasetia et al., 2017). The reproductive performance of eight MCE stony coral species from the Red Sea manifests a decrease with depth, as reflected in delayed or protracted spawning events, decreased fecundity and/or diameter of oocytes, and even an apparent absence of female gonads (e.g., Feldman et al., 2018; Shlesinger et al., 2018). In contrast, in the United States Virgin Islands, the polyp fecundity of the depth-generalist Orbicella faveolata increases with depth (e.g., Holstein et al., 2015) while Porites astreoides features equal planulae densities along the depth gradient there (e.g., Holstein et al., 2016). The above findings have moderated the optimism concerning the DRRH, suggesting its restriction to individual depth-generalist species during periods of high disturbance, rather than constituting a prevalent phenomenon (Bongaerts et al., 2017). The current knowledge therefore posits that Indo-Pacific stony corals display a decreased reproductive performance with increasing depth.
Octocorals are considered the second most common group of macrobenthic organisms on many Indo-Pacific reefs, after the reef-building stony corals (e.g., Fabricius and Alderslade, 2001). They are similarly common on mesophotic reefs (Benayahu et al., 2019). However, the ramification of depth-related effects on octocoral reproductive performance (e.g., decreased light intensity and seawater temperature regime across depth) has been largely ignored. The gametogenic cycles of only two sub-tropical mesophotic species of the north-western Mediterranean Sea have been studied to date and compared to their shallow-water conspecifics: the precious red coral, Corallium rubrum, which exhibits a similar gonad diameter and fecundity with depth down to 45 m (Santangelo et al., 2003; Tsounis et al., 2006). However, it also features a depth-related staggered spawning, which coincides with the differences in the seawater temperature regimes between the deep and shallow zones (40–45 and 16–18 m, respectively); and Eunicella singularis, which features a decreased gonad diameter and a shorter gametogenic cycle in the MCEs compared to its shallow-water conspecific (Gori et al., 2012). Strikingly, to date no reproductive features of mesophotic Indo-Pacific reef-dwelling octocorals have been studied (Benayahu et al., 2019).
The current study engages with two zooxanthellate octocorals Ovabunda spp. and Rhytisma fulvum fulvum (see Fabricius and Alderslade, 2001; Halász et al., 2014). Based on Barneah et al. (2004) and Goulet et al. (2008) both taxa harbor symbionts of the genus Cladocopium (LaJeunesse et al., 2018), with no data currently available on the MCE species. Interestingly, while Ovabunda spp. parental colonies vertically transmit their symbionts to the planulae, in R. f. fulvum the transmission is horizontal (Benayahu and Loya, 1983, 1984b). Ovabunda spp. have been recorded in the Red Sea and western Indian Ocean (Benayahu, 2002; Halász et al., 2014; McFadden et al., 2017), with one additional record from the Andaman Sea (Janes et al., 2014). R. f. fulvum has a rather wide distribution in the Red Sea, Indian Ocean, Indonesia, Ryukyu Archipelago, and Taiwan (e.g., Benayahu, 2002; Benayahu et al., 2004). Ovabunda spp. and R. f. fulvum are both highly abundant in the upper MCEs of the northern Gulf of Eilat/ Aqaba, (Shoham and Benayahu, 2017). Their sexual reproduction has been previously studied in the northern Red Sea to a maximal depth of 30 m (Benayahu and Loya, 1983, 1984a,b). Ovabunda species are gonochoric internal-brooders, demonstrating a rather uniform gonadal structure and annual development among the different species (Benayahu and Loya, 1984a,b; Benayahu, 1990, 1991). In the polyps of octocorals, the gonads develop from endodermal cells in the mesoglea of four lateral and two ventral mesenteries (Farrant, 1986) and are primarily directed toward the center of the gastrovascular cavity (e.g., Benayahu and Loya, 1983). The term gonads is used here to describe mesenteries with mature gametes. Upon maturation, the oocytes detach from the mesenteries and pass into temporary unique brooding-chambers where they undergo embryogenesis to planulae, which are released into the water annually between June–September (Benayahu and Loya, 1984a,b). Colonies of R. f. fulvum exhibit two distinct color morphs: yellow-brown colonies dominate mostly the shallow reef, while gray or purple-gray ones are mainly found at deeper depths (Benayahu and Loya, 1983). R. f. fulvum is a gonochoric surface-brooder. During spawning the ripe spermaries release gametes into the water and the oocytes are fertilized within the polyps shortly before initiation of the surface-brooding event (Benayahu and Loya, 1983). Developing planulae are brooded on the surface of the female colonies and within six days post-fertilization they mature, detach and sink to the bottom (Benayahu and Loya, 1983). The surface-brooding event corresponds to the lunar periodicity and occurs annually from a few days after a new or full moon during June–July. Surface-brooding commences first among the shallow population (3–5 m) and subsequently takes place among the deeper one (27–30 m: Benayahu and Loya, 1983). The coloration of the embryos and of the resulting planulae corresponds to the color morph of the parent colony (Figures 1A,B; yellow vs. gray-white). The current study examined the reproductive features of Ovabunda spp. and R. f. fulvum, hypothesizing that the upper MCE populations of both species would exhibit a viable sexual reproduction. Accordingly, the following questions were addressed: (1) what are the temporal aspects of their gonad development in terms of duration and timing of maturation; (2) what are their sex ratios and fecundities; and (3) how do their mode of reproduction and breeding pattern correlate to lunar phase and seawater temperature? The reproductive biology of the two upper MCE octocorals was compared to that of their respective shallow-reef conspecifics in order to provide insights into the reproductive features of the two taxa also in relation to the prevailing conditions at the lower edge of their depth occurrence. The findings would seem to challenge in part the prevailing hypotheses, including the DRRH (e.g., Bongaerts et al., 2010, 2017), as well as to negate a possible connectivity of the two studied octocoral species between the two reef zones, and the ability of the MCE populations to replenish degraded shallow-reefs (see also: Kahng et al., 2010, 2014; Van Oppen et al., 2011).
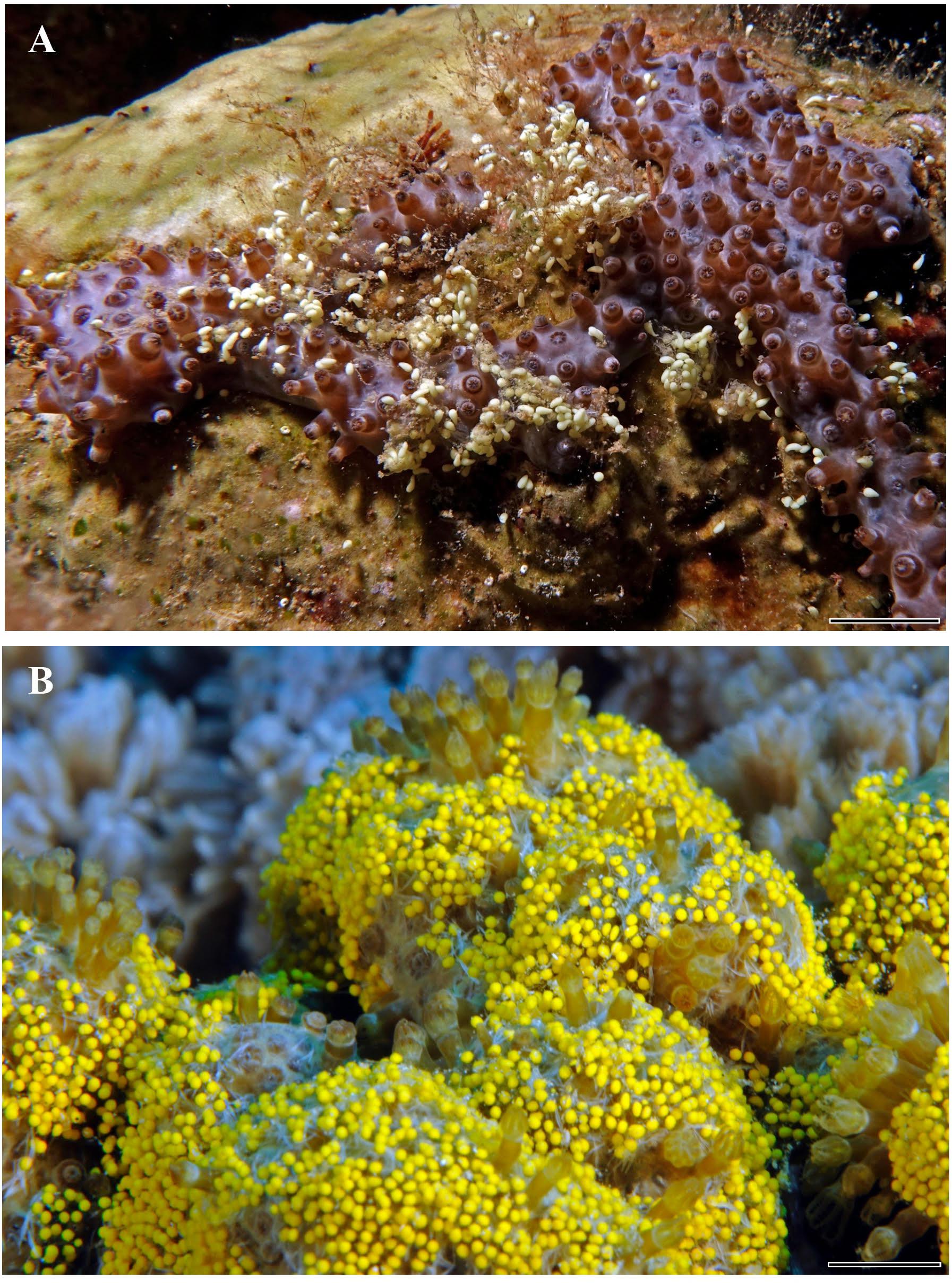
Figure 1. Surface-brooding of Rhytisma fulvum fulvum: (A) mesophotic gray morph and (B) shallow-water yellow color morph. Both images feature brooded planula-larvae entangled in a mucus layer during surface-brooding. Scale bars = 0.5 cm. (Photo (B) courtesy of Gil Koplovitz).
Materials and Methods
Study Site and Studied Species
Samples of Ovabunda spp. and R. f. fulvum were obtained from the reef across from the Interuniversity Institute for Marine Sciences in Eilat (IUI), northern Gulf of Aqaba, Israel (41 practical salinity units, 29°30′N, 034°55′E) during 2016–2017 (see details ahead). The reef there features a flat, mostly hard bottom, a moderate slope down to 12 m, and then a rather steep descent to 35–38 m, along a sandy slope. Deeper, it further descends along a moderate sloping sea-bed down to the lower edge of the MCE (150 m). In the upper MCE (38–45 m), colonies of Ovabunda spp. attain a height of only several cm and are usually found in aggregations. A recent study demonstrated the difficulty in distinguishing among Ovabunda species in the field due to the lack of morphological variation, overlap in habitat use, and other ecological characteristics of the colonies (McFadden et al., 2017). The current study therefore adopted a genus level designation, while keeping in mind that O. macrospiculata is the most common species at the study site (Halász et al., 2014). Colonies of R. f. fulvum of the two different color morphs had been previously identified as the same morphospecies (McFadden et al., 2011). The colonies feature an encrusting growth form, commonly growing on both natural and artificial substrates (Benayahu and Loya, 1983).
Reproductive Studies
To study the reproductive features of Ovabunda spp. and R. f. fulvum, samples comprising 6–12 random colonies of each species were collected monthly from the upper MCE (38–45 m, January 2016–August 2017), using technical diving. Additional monthly samples, of eight colonies each of Ovabunda spp. and R. f. fulvum from the shallow reef (< 12 m), were obtained by SCUBA-diving during their expected season of reproduction (Ovabunda spp.: June–September 2016, April–May 2017 and R. f. fulvum: June–September 2016, April–August 2017, see: Benayahu and Loya, 1983, 1984a). Samples were placed underwater in Ziploc bags, brought to the laboratory, and fixed overnight in 4% formaldehyde in seawater. They were then carefully rinsed three times in double-distilled water and preserved in 70% ethyl-alcohol for further examination (see below). The gastrovascular cavities of polyps of both species were examined under a dissecting microscope in order to study their gonads. Samples of R. f. fulvum were decalcified (see ahead) prior to this examination in order to dissolve their large spindle-shape sclerites that could have hampered the study of the gonads. Ovabunda’s minute platelets did not require this procedure. Next, gonads were removed from 3–5 polyps of each sample using fine forceps, and wet mounts were prepared for light microscopy examination (Nikon OPTIPHOT). The sex of the gonads was determined, and their maximal diameter was measured to a precision of 10 μm. Samples with no gonads were assigned as the indeterminate group. The sex of colonies of both species with gonads measuring < 50 μm in diameter could not be determined in the wet mounts and were therefore histologically sectioned. For this, samples of both species were decalcified for two successive periods of 30 min each, using a mixture comprising a 1:1 ratio of 50% formic acid in distilled water (DW) and 20% trisodium citrate in DW, washed three times with double-distilled water, and then dehydrated in a graded series of ethyl-alcohol (Rinkevich and Loya, 1979). Subsequently, the samples were placed in paraffin (56°C) and cross-sections, 6–7 μm, were obtained using a microtome (MIR, SHANDON). The serial sections from each sample were mounted on microscope slides, stained with Ehrlich’s hematoxylin and eosin (Carson, 1997), and examined under a light microscope for sex determination and gonad measurements. The gonad measurements were used to calculate average diameter of male and female gonads of both species and their monthly size-class frequency distribution.
Sex Ratio and Fecundity
Calculations of sex ratios were based on monthly samples that featured either male or female gonads, with specimens of Ovabunda spp. collected during April 2016–December 2017 (except for September 2016) and April 2017–May 2017, and R. f. fulvum collected during April-July in both years. Fecundity was considered as the number of mature oocytes per polyp (Kahng et al., 2011). This was calculated for Ovabunda spp. by examining samples obtained in January 2016 and June–November 2016 (see Benayahu and Loya, 1984a). For this purpose, three random polyps from each colony were selected, and the number of oocytes > 300 μm in diameter was determined (see above). The fecundity of R. f. fulvum was determined during its breeding season: June–July 2016 and 2017 (Benayahu and Loya, 1983) by examining seven polyps from each sample, and the number of oocytes per polyp was similarly determined. A comparison was conducted between sex ratio and fecundity for both the upper MCE and the shallow-reef zone (see below).
Monitoring of Surface-Brooding Events
Line transects (30 m long) were marked by buoys prior to the expected reproduction season of R. f. fulvum (June 2017) as follows: two transects at 42–45 m and one at each of the following depths: 38–42, 25, 13, and 7 m. Daily underwater examination of colonies along these transects was conducted from 24 June to the end of July 2017. Colonies with surface-brooded embryos were counted per transect and their percentage out of the total number of colonies per a given depth transect was determined and termed “surface-brooding intensity.”
Temperature Measurements
In order to obtain temperature data, two data loggers (HOBO Water Temp Pro v2, Onset Computer Corporation) were deployed at 5 and 45 m depth and set to take measurements every 15 min (for details see Shlesinger et al., 2018). Data were obtained during January 2016–August 2017. Average monthly seawater temperature at each depth was calculated in order to correlate to mean monthly oocyte diameter. Average daily seawater temperature values during June–July, for both years, were calculated in order to determine a possible correlation with the timing of surface-brooding events at each depth zone.
Data Analysis
Statistical analyses were performed on the results obtained for each octocoral species using R statistical software (R Core Development Team, 2016). Data were tested for the assumptions of parametric tests. Where assumptions were violated, randomization tests were introduced using the package lmPerm (Wheeler, 2013), followed by Tukey’s HSD post hoc test. To examine the differences in gametogenic cycle between the two depth populations, mean spermary/oocyte diameter was calculated for each colony for each month, followed by a permutational two-way ANOVA test. Considering the relatively large variation in the diameter of Ovabunda oocytes (Benayahu and Loya, 1984a), the average diameter of the largest 50 oocytes of each colony per a given month was calculated and compared between the two reef zones using a two-way ANOVA test. Possible sex ratio differences between depth zones (< 12 m vs. 38–45 m) were tested using a two-sided Fisher exact test. Possible deviations from a 1:1 sex ratio were tested using a χ2 test. The effect of depth on the fecundity of the two focal species was analyzed using a mixed-effects model (nested Anova), with the number of oocytes in a single polyp nested in a colony at each depth. Correlation between mean monthly temperature and mean monthly diameter of oocytes was tested using the Pearson correlation test. Descriptive statistics in the text are reported as means and standard error (± SE).
Results
Sexuality and Mode of Reproduction
The upper MCE Ovabunda spp. are gonochoric, and monthly examination of the colonies revealed that a colony had either male or female gonads during April 2016—January 2017. Gonad initiation takes place along the mesenteries in the upper part of the polyps, and each gonad is individually attached to the mesenteries by a short pedicel. The spermaries are round, relatively translucent, and upon maturation they become oval in shape (Figures 2A,B). The oocytes are spherical and become opaque cream-white as their development progresses. The nucleus is embedded in the granular ooplasm of the oocytes, which are enclosed by a thin mesogleal layer and an external follicular gastrodermis (Figures 2C,D). In several female colonies of both depth zones planulae were found inside the brooding pouches (see Benayahu and Loya, 1984b) during July and August 2016.
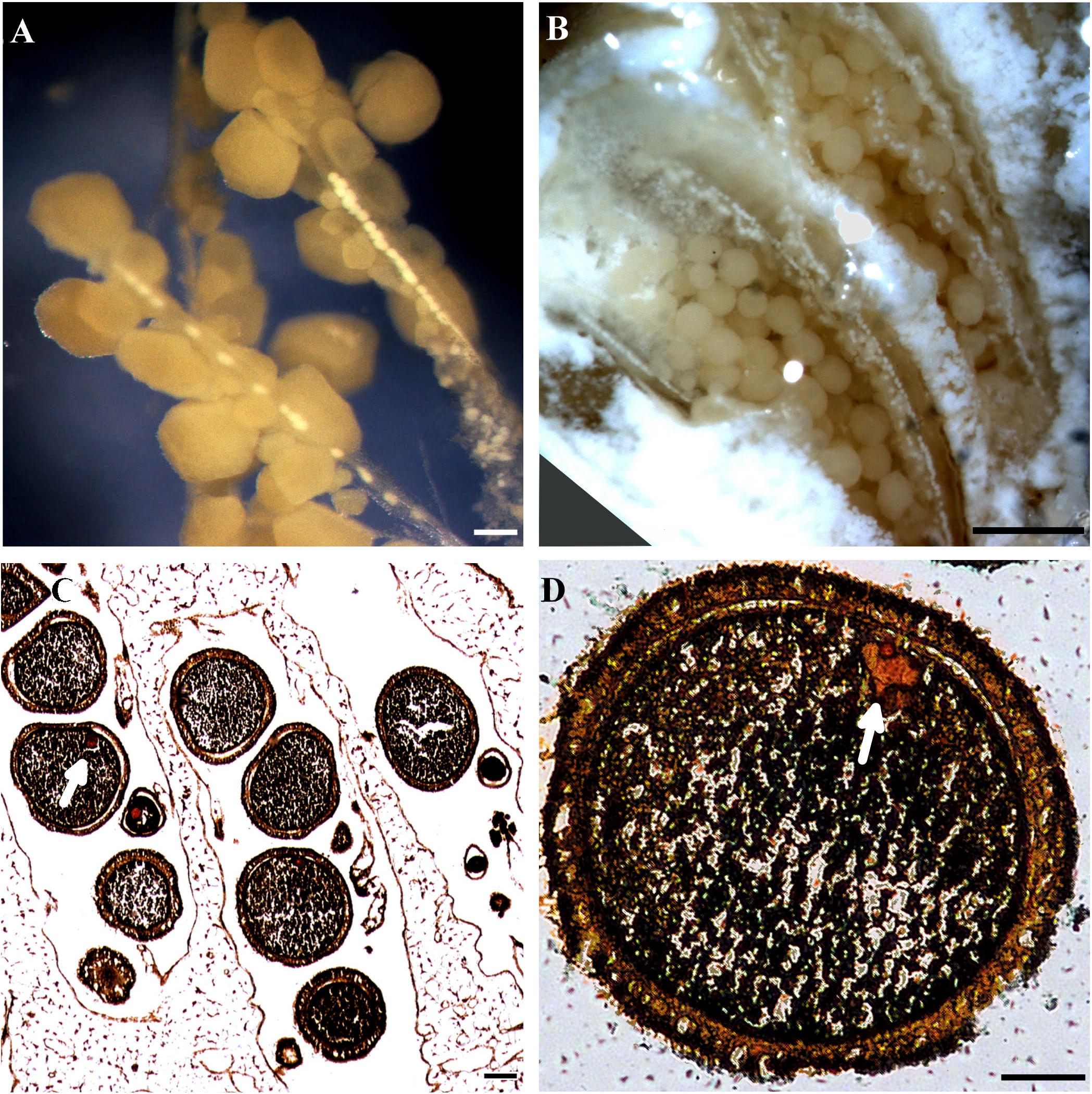
Figure 2. Gonads of upper MCE Ovabunda spp. (A) Spermaries on mesenteries (July 2017). (B) Male colony with spermaries filling polyp cavities (July 2016). (C) Sagittal section in female colony featuring ripe and immature oocytes (August 2016). (D) Cross-section of mature oocyte. White arrow points toward the nucleus (August 2016). Scale bars = 100 μm.
Colonies of the upper MCE R. f. fulvum are gonochoric and reproduce by surface-brooding of their planula-larvae, as do the shallow-water colonies. Similarly, in Ovabunda spp., the gonads of both sexes develop along the four lateral and two ventral mesenteries. The gonads are located in the middle part of each mesentery and directed toward the center of the polyp gastrovascular cavity (Figure 3C). During the first 2–3 months of gametogenesis, increasing numbers of spermaries and oocytes develop in clusters of 2–5 each, and upon achieving their full size they fill the polyp cavity (Figures 3A–D). The average diameter of male and female gonads of both species did not significantly differ between the upper MCE and the shallow-water colonies (permutation two-way ANOVA, p > 0.05). The percentage of reproductive colonies in the month preceding the breeding season was calculated for each species in the two reef zones (Figure 4). Gonads, either male or female, were recorded in 67% of upper MCE Ovabunda spp. colonies and in all of the shallow-water ones during June 2016. Similarly, gonads were recorded in all and 65% of the upper MCE R. f. fulvum colonies during June 2016 and 2017, respectively, and in all the shallow-water colonies in May 2016 and 2017.
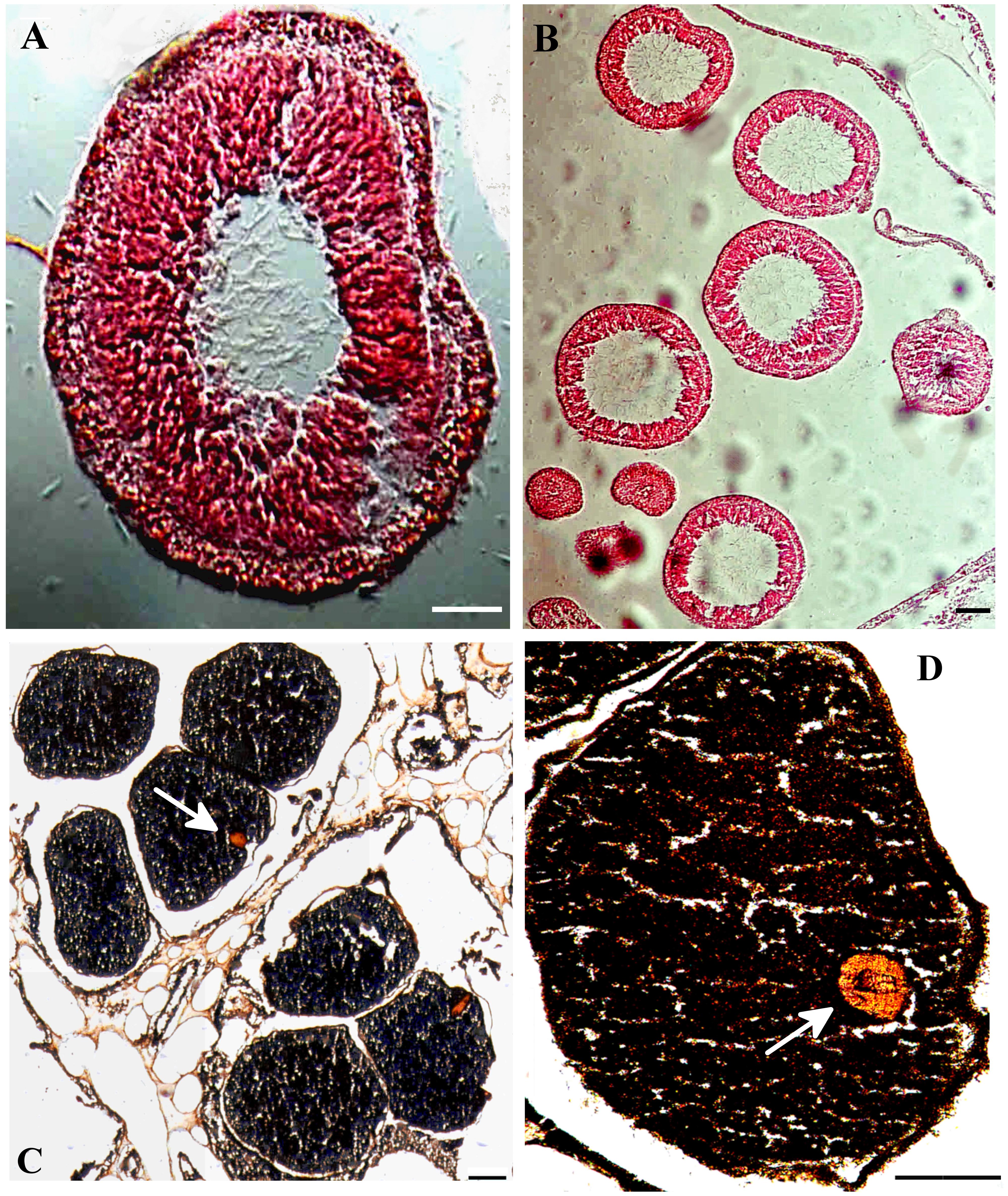
Figure 3. Gonads of upper MCE Rhytisma fulvum fulvum (A) Sagittal section in colony with a mature spermary featuring spermatids in its periphery (June 2016) (B) Cross-section of a male colony showing mature and immature spermaries, featuring spermatids oriented to the center of the spermaries (June 2016) (C, D) Sagittal sections in colony, with mature oocytes featuring a nucleus in their periphery. White arrow points toward the nucleus (July 2016). Scale bars = 100 μm.
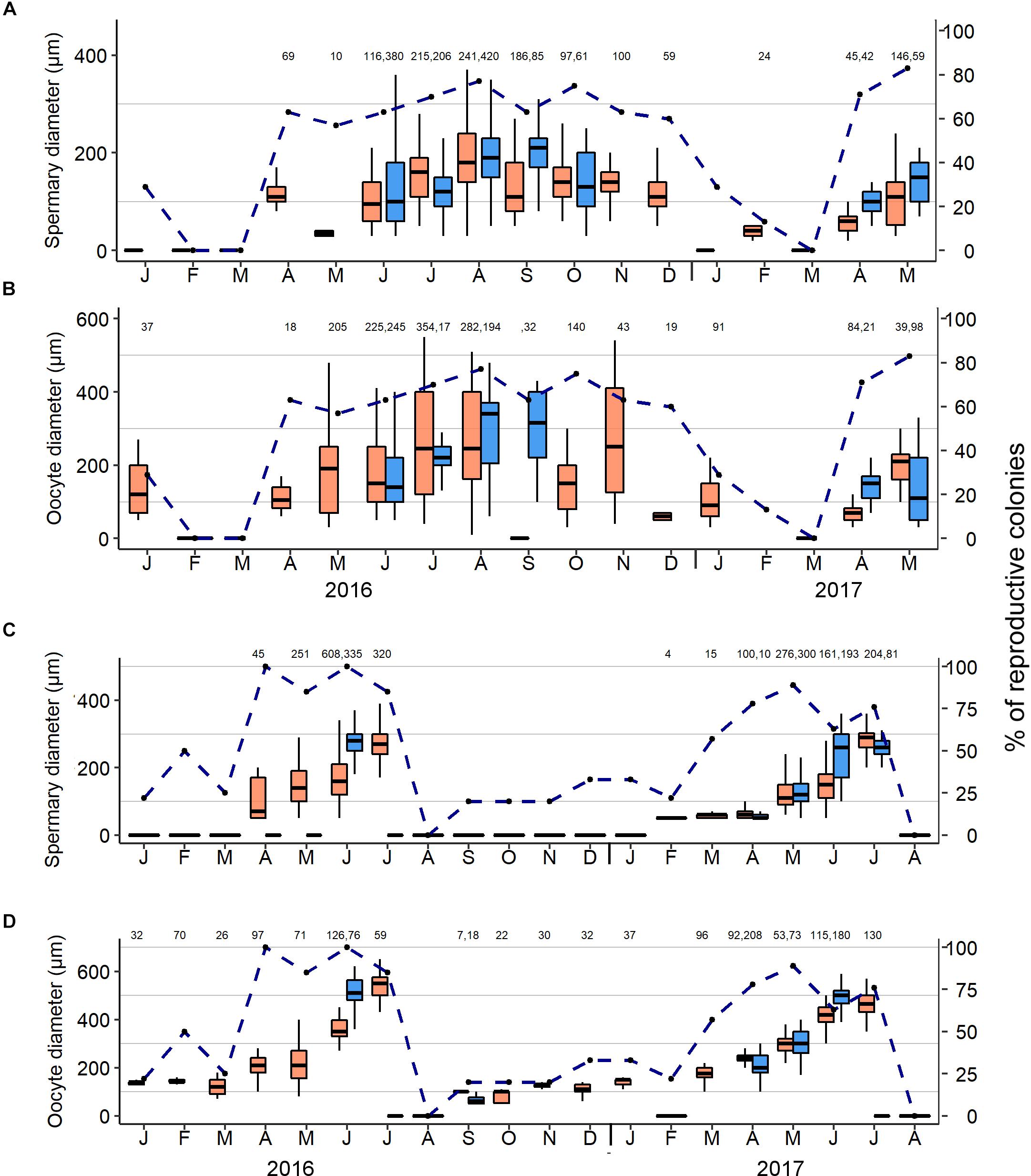
Figure 4. Monthly average diameter of gonads of upper mesophotic (in orange) and shallow-water (in blue) octocorals during January 2016–August 2017: Ovabunda spp. (A) Spermaries and (B) Oocytes, and Rhytisma fulvum fulvum (C) Spermaries and (D) Oocytes. Box limits represent 25th and 75th percentiles, whiskers span upper and lower limits of data, horizontal lines within boxplots represent medians, and above x-axis number of gonads measured each month. Dashed line represents percentage of reproductive colonies of each species.
Gametogenic Cycle
The monthly sampling of Ovabunda spp. and R. f. fulvum colonies revealed their annual gonadal development (Figure 4 and Supplementary Data Sheets S1, S2) along with their monthly size-class frequency distribution (Figure 5). Male and female gonads of the upper MCE Ovabunda spp. colonies developed concomitantly during April-December 2016. In addition, oocytes were observed in January 2016 and 2017. Large numbers of gonads, filling 70–80% of the length of the mesenteries, were found in low occurrence during July–November 2016, and not at all in February and March of both years, except for one colony found with spermaries in February 2017. The largest spermaries among the upper MCE colonies were recorded in August 2016 (Figure 4A, 187 ± 82 μm in diameter; n = 241); while among the shallow-water colonies a maximum diameter was recorded in September 2016 (205 ± 50 μm; n = 85). Correspondingly, the largest oocytes among the MCE colonies were found during July, August, and November 2016, with no significant difference among these months (Figure 4B, 266 ± 143, 268 ± 138 and 273 ± 150 μm, respectively; two-way permutation ANOVA test, n = 679, p > 0.05). Additionally, the largest oocyte diameter of the shallow-water colonies was recorded in August and September 2016 (300 ± 97 and 298 ± 110 μm, n = 194 and 32, respectively). The average diameter of the 50 largest oocytes of each colony was calculated and compared between the two reef zones, and similarly revealed no significant difference (two-way ANOVA, p > 0.05). The largest oocytes of the upper MCE colonies were found in July and August 2016 (456 ± 34 and 454 ± 27 μm, respectively); while the largest oocytes of the shallow-water colonies were recorded in August 2016 (410 ± 27 μm). The monthly oocyte size-class frequency distribution of upper MCE colonies revealed small oocytes < 100 μm for most months, with only a few being recorded during June–November 2016 (Figure 5A). Oocytes > 400 μm in diameter were common in July, August, and November 2016, but only sporadically in January 2016.
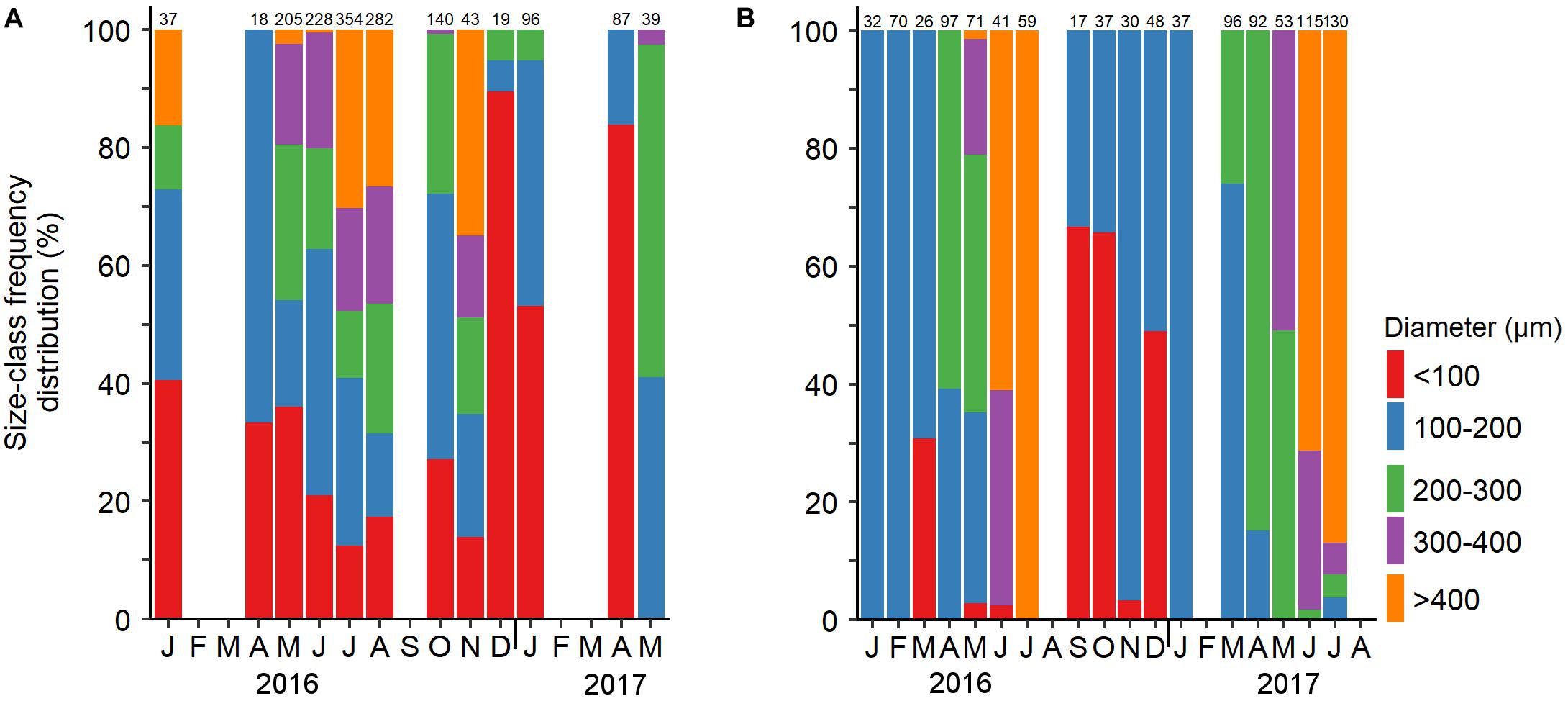
Figure 5. Size frequency distribution of monthly diameters of oocytes in upper MCE (A) Ovabunda spp. and (B) Rhytisma fulvum fulvum in January 2016–August 2017. Above columns, number of oocytes measured each month derived from 1–5 colonies per month, three polyps per Ovabunda spp. colony and five per R. f. fulvum colony.
Gonad development and maximal oocyte diameter of R. f. fulvum in the upper MCE lagged behind those of their shallow-reef conspecifics in both 2016 and 2017 (Figure 4D), also corresponding to the lag in the increase in water temperature recorded between 5 and 45 m depth (Figure 6). Spermatogenesis in both reef zones succeeded oogenesis and occurred for 4–6 months each year (February–July), while oogenesis lasted for 9–10 months until maturation (September–July). No gonads were found in August for both years. The diameter of spermaries steadily increased between April–July 2016 and February–July 2017, reaching the largest diameter in July of both years (267 ± 50 and 279 ± 46 μm; n = 45 and 204, respectively). In correspondence to the upper MCE colonies, the shallow-water ones also exhibited a gradual increase in spermary diameter from April 2017 to early July 2017, with the largest spermaries being recorded in June 2016 (Figure 4C, 277 ± 37 μm; n = 335). Oocyte development in both depth zones commenced in September and the average oocyte diameter of the deeper colonies did not significantly differ from each other during the first four months of oogenesis (September-December 2016, two-way permutation ANOVA test, p > 0.05). A steady increase in their diameter then took place during January-July, for both years, reaching the largest diameter in July, of both years (Figure 4D, 541 ± 46 μm in July 2016 and 442 ± 89 μm in July 2017; n = 59 and 180, respectively). Oogenesis among the shallow-reef colonies was shorter, and their surface-brooding event took place prior to that in the upper MCE (see below). Correspondingly, the largest shallow-reef oocytes were recorded in June of both years (509 ± 85 μm in 2016 and 492 ± 50 μm in 2017; n = 76 and 180, respectively). The monthly oocyte size-class frequency distribution revealed small oocytes of < 100 μm during the first four months of oogenesis, followed by a subsequent development and expanded range of gonad size groups, yielding ripe oocytes > 400 μm in June–July of both years (Figure 5B).
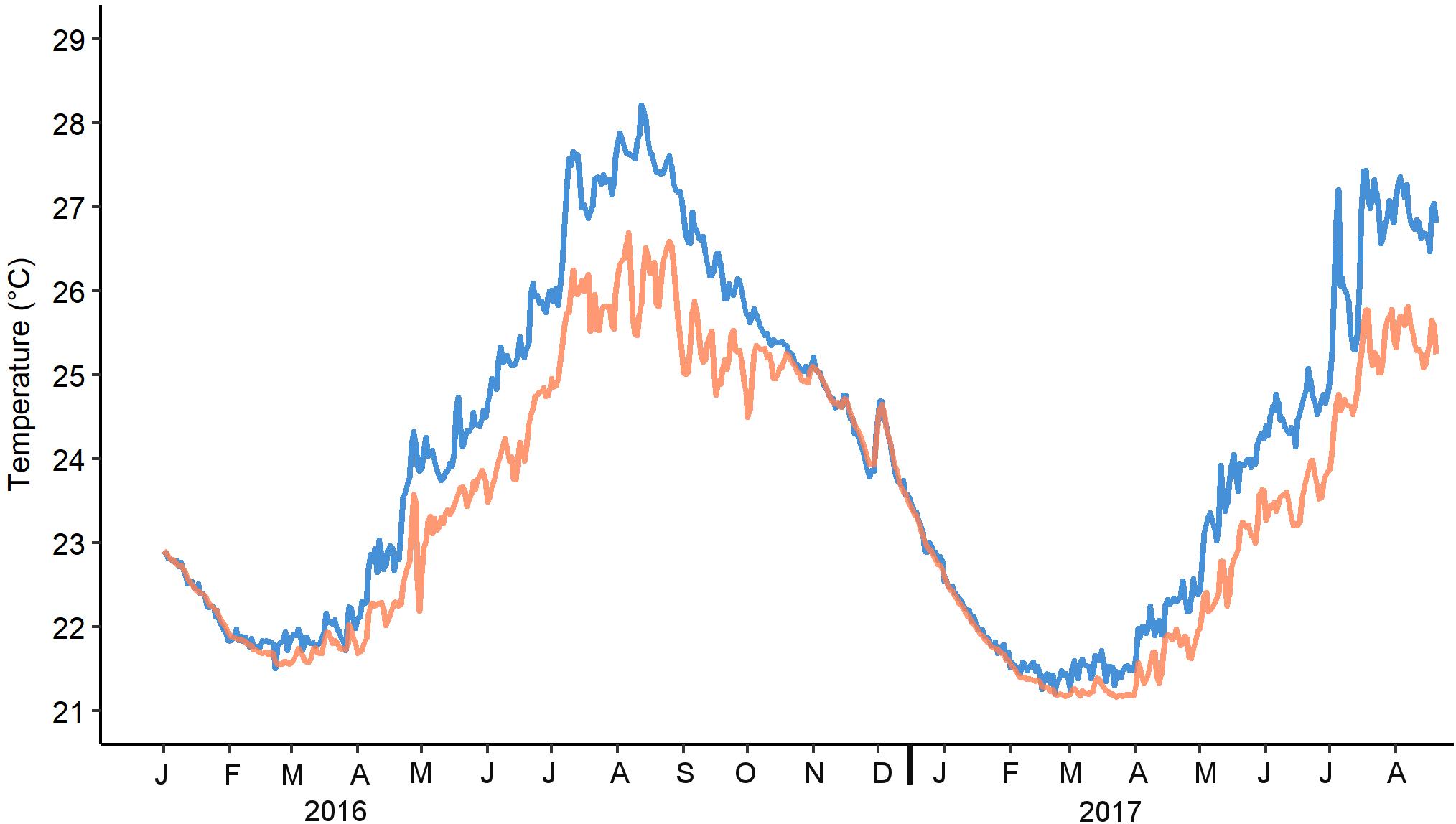
Figure 6. Continuous average daily temperature measurements from shallow-water (blue) and upper mesophotic (orange) zones.
Reproductive Features in Relation to Seawater Temperature and Moon Phase
A significant correlation was found between seawater temperature in the upper MCE (45 m) and monthly mean diameter of oocytes of both Ovabunda spp. (Pearson Correlation test, R = 0.75, p = 0.017) and R. f. fulvum (Pearson correlation test, R = 0.96, p < 0.001 and R = 0.89, p < 0.01 in 2016 and 2017, respectively). The largest oocytes of Ovabunda spp. were recorded in July and August 2016, coinciding with the prevailing highest seawater temperature (Figure 6 and Supplementary Data Sheet S3). A surface-brooding event of the upper MCE R. f. fulvum colonies took place from July 19–25, 2017, between the third lunar quarter and new moon, 2 weeks after that of the shallow-water colonies, which took place from 4–9 July, between the first lunar quarter and the full moon (Figure 7). Interestingly, the timing of surface-brooding events coincided with the delay in seawater warming with depth, as indicated by the difference in the average daily seawater temperature between the two depth zones (Figure 7). It would thus seem that a temperature threshold governs surface-brooding induction in both depth zones. A substantial increase of 1.2°C in seawater temperature occurred at 45 m during 12–18 July, achieving a daily maximum of 25.7°C and then stabilized between 25–26°C during the surface-brooding event. Similarly, a distinct increase of 2.5°C in seawater temperature occurred at 5 m between 29 June and 5 July 2017, achieving a maximum of 27.2°C and then stabilizing above 26°C during the shallow reef surface-brooding event. Similarly, the latter was associated with seawater warming in June–July 2016 (Supplementary Figure S1). Notably, the intensity of the surface-brooding event in the upper MCE was lower than that in the shallow-reef, with only 8–29% of colonies in the MCE surface-brooding planulae, compared to 45–50% of the colonies in the shallow reef (Figure 7).
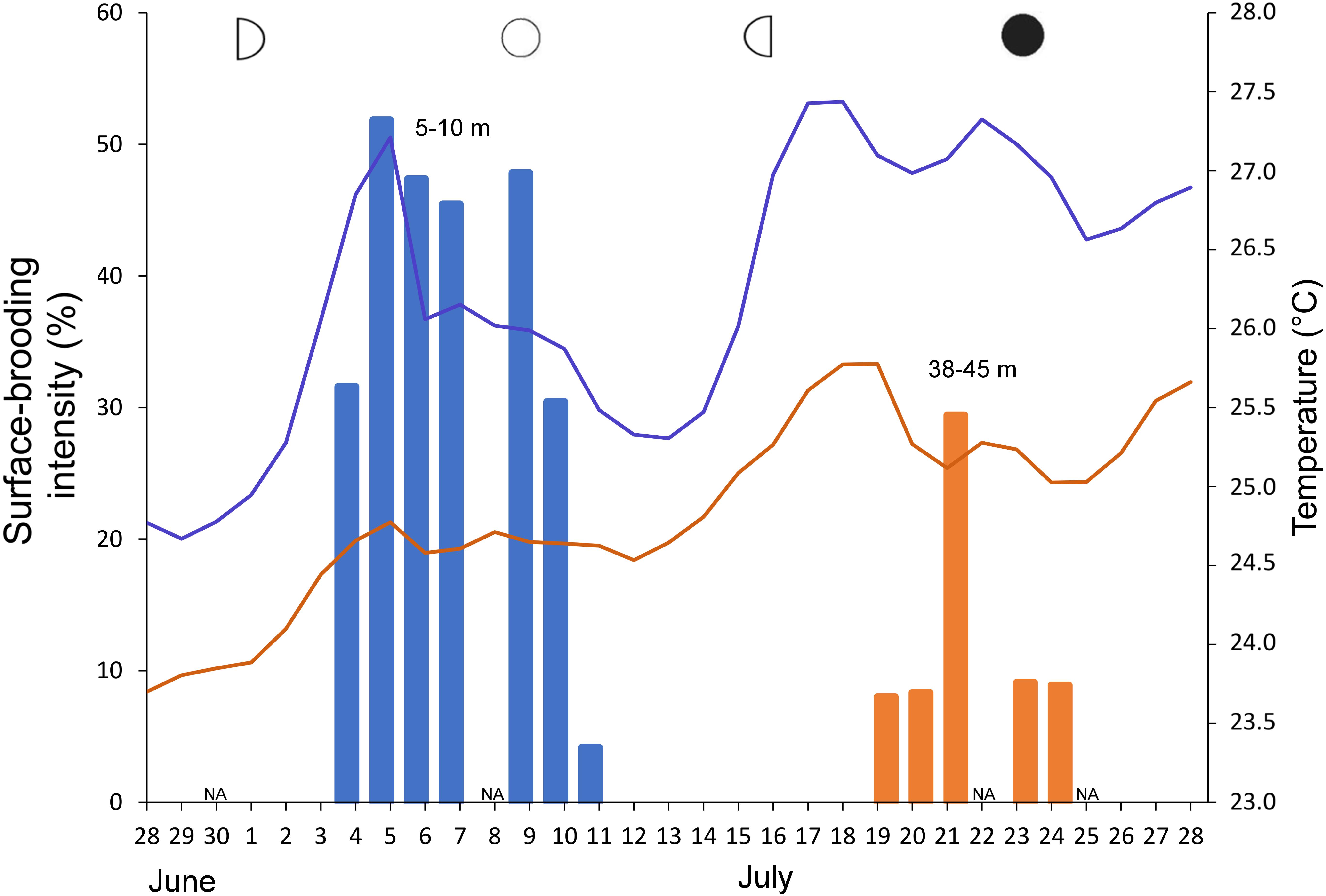
Figure 7. Daily percentage of surface-brooded colonies of Rhytisma fulvum fulvum colonies in upper MCE (orange) and shallow reef (blue) during June–July 2017, with indication of lunar phase. Respective curves present daily mean temperature in both reef zones. NA represents days on which no dive was conducted.
Sex Ratio
The sex ratio of Ovabunda spp. and R. f. fulvum was calculated for each reef zone when both female and male gonads were present (Table 1). The upper MCE Ovabunda spp. colonies revealed a 1:1 sex ratio and the shallow-water ones 1:1.6, indicating no significant difference between them (two-sided Fisher exact test, p > 0.05). Similarly, a χ2 test did not reveal a significant deviation from a 1:1 ratio for both zones (p > 0.05). The upper MCE population of Ovabunda spp. comprised 26 female, 26 male, and 20 colonies with no gonads (∼36, 36, and 28% of the population, respectively). The shallow-reef population comprised 12 female, 20 male, and 8 colonies with no gonads (∼30, 50, and 20% of the population, respectively).
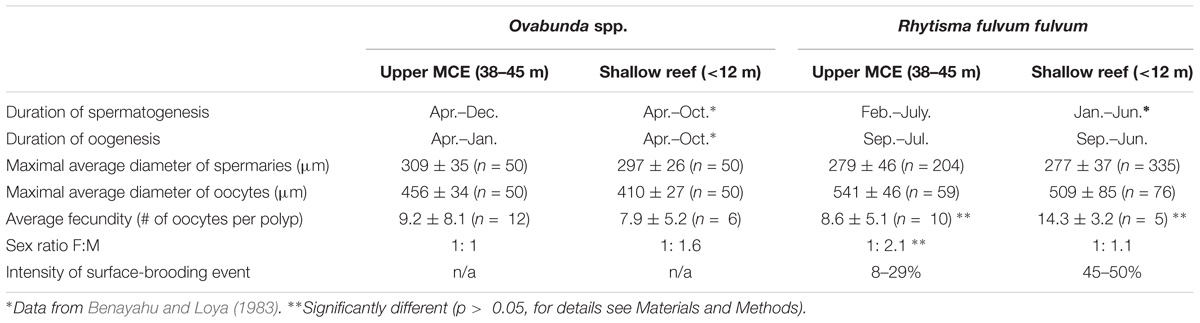
Table 1. Ovabunda spp. and Rhytisma fulvum fulvum: Comparison of reproductive features between the upper MCE and shallow reef (n indicates sample size).
The sex ratio of R. f. fulvum colonies in the upper MCE was 1:2.1 female-to-male and in the shallow reef 1:1.1 female-to-male, indicating no significant difference between the two zones (two-sided Fisher exact test, p > 0.05). A χ2 test revealed a deviation from the 1:1 sex ratio only in the upper MCE colonies (n = 62, df = 1, p = 0.003), but not in the shallow-reef ones (n = 32, df = 1, p > 0.05). The upper MCE colonies comprised 20 female, 42 male, and 7 colonies with no gonads (∼29, 61, and 10% of the population, respectively), and the shallow-water colonies comprised 14 female, 18 male, and 1 colony with no gonads (∼42, 55, and 3% of the population, respectively).
Fecundity
The polyp fecundity of Ovabunda spp. and R. f. fulvum was calculated for both reef zones during their respective breeding seasons (Figure 8 and Supplementary Data Sheet S4 see Section “Materials and Methods” for the exact months). There was no significant difference between the fecundity of the Ovabunda spp. colonies of the upper MCE and those of the shallow-reef, averaging 9.2 ± 8.1 and 7.9 ± 5.2 oocytes per polyp (n = 12 and 6, respectively, Figure 5A, mixed- effects model, p > 0.05). All colonies featured a large variation in polyp fecundity, ranging from 2–44 ripe oocytes per polyp in the upper MCE colonies and 1–23 in the shallow-water ones. The fecundity of upper MCE R. f. fulvum colonies was significantly lower compared to the shallow-reef colonies during June-July of both 2016 and 2017, averaging 8.6 ± 5.1 and 14.3 ± 3.2 oocytes per polyp (n = 10 and 5, respectively, Figure 8B, mixed-effects model, p < 0.05).
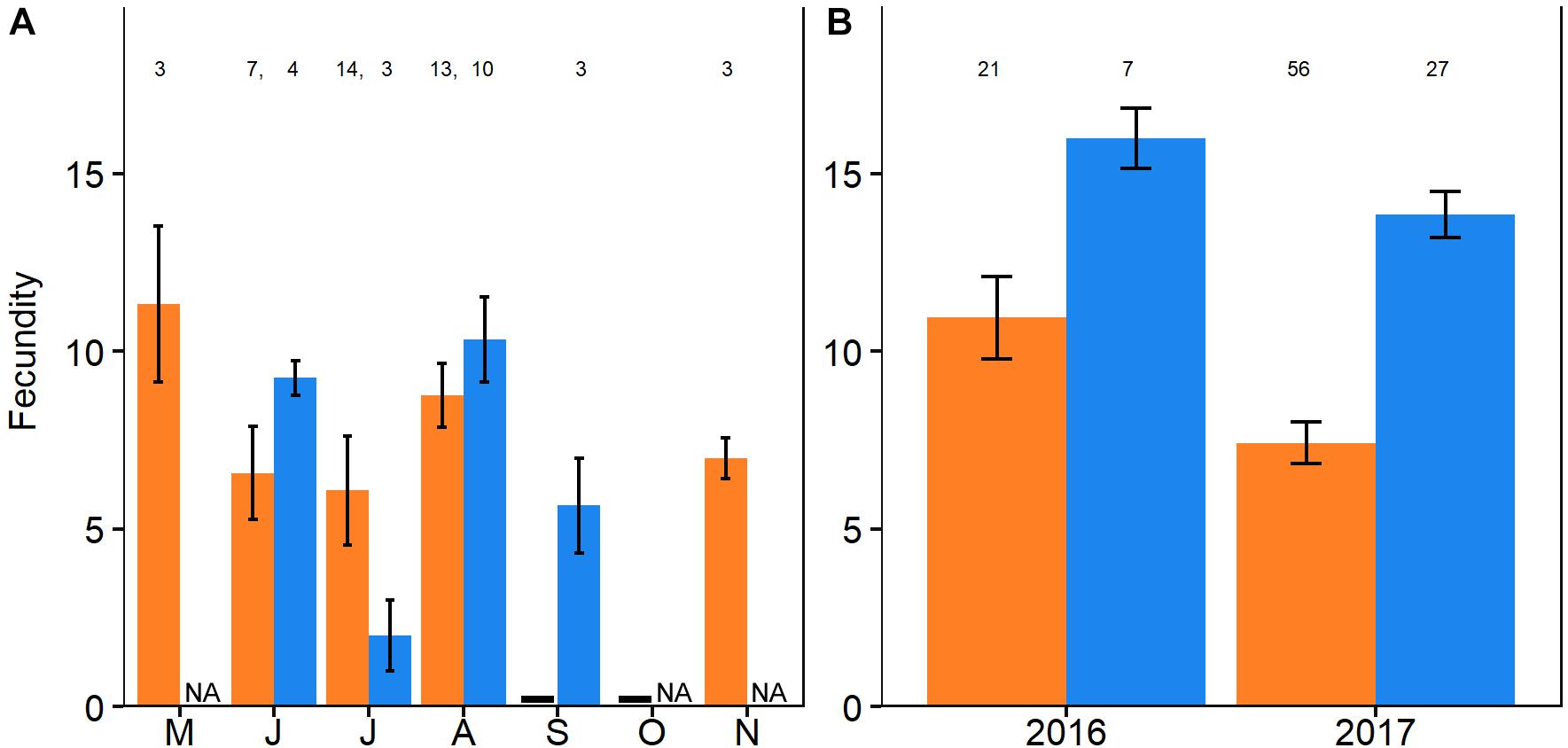
Figure 8. Number of mature oocytes per polyp of upper MCE (orange) and shallow-water (blue) (A) Ovabunda spp. and (B) Rhytisma fulvum fulvum during peak breeding season of 2016 and 2017. Number of examined polyps per season above x-axis. NA represent months in which no sample was analyzed.
Discussion
This study is the first to examine the sexual reproduction of Indo-Pacific zooxanthellate octocorals in the upper MCE and compare it to that of their shallow-reef conspecifics. The findings confirm the hypothesis that the two studied upper MCE octocorals, Ovabunda spp. and R. f. fulvum, reproduce sexually and features a similar mode of reproduction in the two reef zones: Ovabunda spp. is a gonochoric internal-brooder (Benayahu and Loya, 1984a), and R. f. fulvum is a gonochoric surface-brooder (Benayahu and Loya, 1983).
Each octocoral species displays several distinct features in the upper MCE that differ from those in the shallow-reef zone. Both species are gonochoric, similar to most other studied octocorals (Kahng et al., 2011) and both exhibit a longer annual oocyte development in the upper MCE than in the shallow reef (Table 1). Protracted spawning or brooding periods have been similarly recorded for several Red Sea stony corals in MCEs (Shlesinger et al., 2018). It was suggested that such a pattern may result from a reduced seasonal range of seawater temperature in the MCE (e.g., Shlesinger et al., 2018). It is interesting to note that, based on a latitudinal scale, a decrease in the annual range of seawater temperature has been posited to be associated with a prolonged reproductive period in both octocorals (De Putron and Ryland, 2009) and stony corals (Richmond and Hunter, 1990; Glynn et al., 2017). For example, it has been suggested that a decrease in the annual temperature range may result in a 2-month longer reproductive period in Pseudoplexaura porosa in Panama than in Bermuda (e.g., ∼4°C in Panama and 10–15°C in Bermuda) (De Putron and Ryland, 2009).
The current findings indicate that the gonads of both studied octocoral species reach a similar maximal size in the two studied reef zones (Figure 1 and Table 1). Likewise, the oocyte diameter of the north-west Mediterranean octocorals C. rubrum and E. singularis do not vary with depth (Tsounis et al., 2006; Gori et al., 2012). In contrast, Briareum asbestinum at 35 m in the Caribbean features smaller oocytes compared to those at 5 m (West et al., 1993). Among several Red Sea stony corals, a reduced oocyte diameter with depth has also been observed (e.g., Paramontastraea peresi: Feldman et al., 2018, Acropora squarrosa, A. valida, Montipora verrucosa, and Galaxea fascicularis: Shlesinger et al., 2018). Similarly, among brooders, a decreased planula size with depth was reported for the upper MCE stony coral S. hystrix in Japan (Prasetia et al., 2017) and in the Gulf of Aqaba (Shlesinger et al., 2018). It has been suggested that among marine invertebrates larger eggs or larvae are associated with a higher survivorship due to the provision of larger energetic reserves (Marshall and Keough, 2007). Consequently, the lower temperature and reduced solar irradiance with depth (Lesser et al., 2009; Kahng et al., 2014) could result in smaller oocytes and planula-larvae. Such environmental constraints could in turn limit the nutritional reservoirs of the propagules and thus also their dispersal range (i.e., Prasetia et al., 2017; Shlesinger et al., 2018). However, the current findings regarding Ovabunda spp. and R. f. fulvum indicate that oocyte dimensions remain stable with depth, suggesting that the planula-larvae of both studied species benefit from similar energetic reserves.
Lunar phase and seawater temperature have been suggested to be among the foremost environmental cues for determining the timing of coral reproduction (Alino and Coll, 1989; Richmond and Hunter, 1990; Baird et al., 2009; Harrison, 2011). Lunar phase has been established as a predictor for spawning of many marine organisms, including octocorals (e.g., Benayahu and Loya, 1983; Pakes and Woollacott, 2008; Mandelberg-Aharon and Benayahu, 2015) and stony corals (Shlesinger and Loya, 1985; Guest et al., 2005; Villanueva et al., 2008). The surface-brooding event of R. f. fulvum in both depth zones is entrained to a distinct lunar phase, although the exact lunar day would appear to shift between years (2016 vs. 2017), as similarly noted by Benayahu and Loya (1983). The current study revealed a distinct seawater temperature increase preceding the surface-brooding event of R. f. fulvum in both depth zones (Figure 4 and Supplementary Figure S1), thus suggesting that a necessary temperature threshold be reached prior to this coral initiating reproduction. Such an increase in seawater temperature has been suggested to induce spawning in several marine invertebrates (Battaglene et al., 2002: Holothuroidea; Caballes and Pratchett, 2017: crown of thorns starfish, Acantaster planci). Furthermore, over geographical scales, a rapid increase in seawater temperature has been recently suggested as being the most prominent environmental cue for the timing of coral spawning events (Keith et al., 2016). The timing of surface-brooding events in the two studied reef zones demonstrated temporal differences between 2016 and 2017. In 2016 two surface-brooding events took place for the shallow-reef population, with a 2-week interval between them; while the deep population displayed only one event (Supplementary Figure S1); and in 2017 a temporal reproductive isolation of surface-brooding events was demonstrated between the upper MCE and the shallow reef (Figure 7). Interestingly, in those 2 years the timing of surface-brooding events corresponded to the timing of a distinct seawater temperature rise. It thus seems that the seawater temperature regime along depth plays an intrinsic role in determining the timing of the surface-brooding events along depth. The possibility of gamete exchange between depth zones might thus be hampered by differential peak temperatures along depth, and thereby reduce connectivity between the different depth populations.
Sex Ratio
An equal female-to-male sex ratio is considered ideal for a population featuring random mating (Maynard Smith, 1978). However, only 46% of the gonochoric octocoral species studied to date have revealed a balanced ratio, while a female-skewed one was reported in 44% of the studies (e.g., Kahng et al., 2011). In the present study a balanced sex ratio was found for Ovabunda spp. in both depth zones. In contrast, a male-skewed ratio was previously recorded in Ovabunda macrospiculata, at both 3–5 and 27–30 m depth (1:1.6 and 1:2.1, respectively, Benayahu and Loya, 1984a). Although the results of the present study contrast with the Benayahu and Loya, 1984a findings, it is reasonable to assume that the small sample size prevented identification of a statistically significant deviation from a 1:1 ratio. The male-skewed ratio in the upper MCE R. f. fulvum population, and the balanced one found in the shallow-reef zone, offer an intriguing finding. Furthermore, the current findings correspond to previous findings indicating an increased occurrence of male R. f. fulvum colonies between 5 and 30 m depth (Benayahu and Loya, 1983). A male-skewed sex ratio is rare among octocorals and to date has been found in only 7% of gonochoric shallow-water octocorals (Kahng et al., 2011). For example, Brazeau and Lasker (1990) noted a male-skewed sex ratio in B. asbestinum from almost twenty locations across the Caribbean; while the temperate octocoral Capnella gaboensis (Australia) exhibited a male-skewed sex ratio in its shallow-water population (< 10 m; Farrant, 1986). However, the finding of a male-biased ratio exclusively in a deeper population is yet to be reported. Two possible explanations for the increase in male occurrence with depth should be considered. Fertilization success among various marine invertebrates has been suggested to be highly affected by sperm limitation (Levitan, 1991, 2004; Lasker, 2006) and, therefore, an increased number of male colonies in the immediate vicinity of female colonies may enhance fertilization success among gonochoric brooders (Benayahu and Loya, 1984a; Brazeau and Lasker, 1990). Hence, the male-skewed R. f. fulvum population recorded in the upper MCE may compensate for its decreased abundance with depth, as recorded by Shoham and Benayahu (2017). Alternatively, as oocyte production is energetically more costly than that of spermary (Charnov, 1982; Loya and Sakai, 2008), the decreasing light with increasing depth (Lesser et al., 2009) may limit the carbon fixation available in the MCE, and thus lead to a decrease in female abundance and concomitantly reduce fecundity (see also below). Determining the factors that contribute to sex ratio is important for a better understanding of the energetic budgets and reproductive plasticity in octocorals, as well as of gonochorism, which is prevalent among octocorals and whose significance awaits further study.
Fecundity and Intensity of Surface-Brooding Events
Polyp fecundity in terms of the number of mature oocytes per polyp is frequently used as a criterion for the reproductive output in marine invertebrates, including corals (Harrison, 2011; Kahng et al., 2011). The current results demonstrate a similar fecundity for Ovabunda spp. colonies in the two reef zones (see Figure 8 and Table 1). For R. f. fulvum, however, a lower fecundity was found in upper MCE colonies compared to those in the shallow reef (Figure 8) and, additionally, a lower intensity of surface-brooding events was found in the deeper population (Figure 4). Similarly, a reduced fecundity has been noted for temperate upper MCE octocorals (Tsounis et al., 2006; Gori et al., 2012) and for most of the studied MCE stony corals (e.g., Rinkevich and Loya, 1987; Prasetia et al., 2016; Feldman et al., 2018; Shlesinger et al., 2018). For example, Gori et al. (2012) reported a lower reproductive output in the upper MCE E. singularis population in comparison to the shallow-water one. However, the latter colonies feature photosymbionts in their tissues, whereas the deeper colonies are azooxanthellate, which probably leads to a decrease in energetic resources with depth. In addition, a lower reproductive effort has been reported for several Red Sea upper MCE stony corals. Shlesinger et al. (2018) noted a reduction in fecundity with depth in S. hystrix, G. fascicularis, Turbinaria reniformis, Montipora verrucosa, and Acropora pharaonis. In contrast, in the United States Virgin Islands, a higher fecundity was recorded for the 40 m depth population of the stony coral O. faveolata in comparison to that of its 8 m depth conspecific; while, equal reproductive effort values were recorded for 35 and 10 m depth P. astreoides colonies at the same location (Holstein et al., 2015, 2016). It thus seems that the substantial decrease in light intensity with depth (Lesser et al., 2009; Eyal-Shaham et al., 2016) might limit the available energy for corals, impacting their energetic-investment in reproduction. These limitations would nonetheless appear to be species-specific, indicating that the upper MCE Ovabunda spp. colonies have undergone adaptations that enable them to reproduce as successfully as in the shallow-reef (Figures 1, 5 and Table 1). However, caution should be taken in asserting the nature of the suggested adaptations, keeping in mind that in the current work the reproductive traits of Ovabunda spp. were studied for only one gametogenic cycle in both reef-zones. Consequently, it is suggested that Ovabunda spp. colonies, for example, are well adapted to the environmental conditions of the upper MCE, and might possess an alternative pathway that contributes to their energy budget, such as enhanced heterotrophy (Anthony and Fabricius, 2000) or higher photosynthetic efficiency (e.g., Mass et al., 2007). The lower occurrence of female colonies and lower fecundity along with the lower intensity of the surface-brooding events in the upper MCE R. f. fulvum, seem to indicate the constraints this species may encounter with increasing depth (Table 1). We therefore suggest that future studies should examine the reproductive intensity of other MCE octocoral species in order to better understand how depth-related constraints affect their population dynamics.
Conclusion
In conclusion, we have shown that the Indo-Pacific octocorals Ovabunda spp. and R. f. fulvum sexually reproduce down to 45 m depth, featuring a similar mode of reproduction to that in the shallow-reef zone. However, each species would appear to possess several life-history traits in the upper-MCE that are distinct from those in the shallow waters. We have demonstrated that Ovabunda spp. is a successful “depth-generalist” while R. f. fulvum’s reproductive performance diminishes in the upper MCE and, consequently, its potential contribution to the replenishment of degraded shallow-reef is questionable. Our findings thus support other recent indications that the deep-reef refuge hypothesis may be relevant only to certain coral species that are considered to be “depth-generalists” (Smith et al., 2016; Bongaerts et al., 2017; Shlesinger et al., 2018). Furthermore, our findings may indicate that a temperature threshold constitutes a prominent external cue for determining the timing of R. f. fulvum surface-brooding events and, as such, its regime along depth may have certain implications regarding the potential connectivity between the two depth-zone populations.
Author Contributions
RL designed and conducted the field and laboratory work, analyzed the data, and did the scientific writing. TS assisted in field work, contributed to data analyses, and provided valuable comments to the manuscript. YL provided helpful comments to the manuscript. YB led the research topic, contributed to data interpretation and understanding their significance.
Funding
The research was in part supported by the TASCMAR project to YB (Tools and strategies to access original bioactive compounds from cultivated marine invertebrates and associated symbionts), who received funding from the European Union’s Horizon 2020 research and innovation program under grant agreement No 634674, and from the Israel Cohen Chair in Environmental Zoology. Collection of animals complied with official permits issued by the respective authorities.
Conflict of Interest Statement
The authors declare that the research was conducted in the absence of any commercial or financial relationships that could be construed as a potential conflict of interest.
Acknowledgments
We thank the Interuniversity Institute for Marine Sciences in Eilat (IUI) for its hospitality and help. We acknowledge V. Wexler for digital editing, G. Koplovitz for underwater photography, E. Shoham, O. Hameiri and G. Zalzman for technical diving assistance, M. Weis for technical assistance, I. Brickner for histology, and N. Paz for editorial assistance. We would also like to thank the reviewers of the manuscript for their constructive comments.
Supplementary Material
The Supplementary Material for this article can be found online at: https://www.frontiersin.org/articles/10.3389/fmars.2018.00445/full#supplementary-material
FIGURE S1 | Mean daily temperature curves during June-July 2016 representing both depth-zones: upper MCE (orange) and shallow-reef zone (blue) with indication of lunar phase. Timing of Rhytisma fulvum fulvum surface-brooding events are indicated in gray: upper MCE (gray-orange) and shallow-reef (gray-blue).
DATA SHEET S1 | Diameter of gonads of upper mesophotic and shallow-water Ovabunda spp. during January 2016–May 2017.
DATA SHEET S2 | Diameter of gonads of upper mesophotic and shallow-water Rhytisma fulvum fulvum during January 2016–August 2017.
DATA SHEET S3 | Continuous daily temperature measurements from shallow-water and upper mesophotic zones in the reef across the interuniversity institute in Eilat during January 2016–August 2017.
DATA SHEET S4 | Number of mature oocytes per polyp of upper MCE and shallow-water Ovabunda spp. and R. f. fulvum during peak breeding season of 2016 and 2017.
References
Alino, P. M., and Coll, J. C. (1989). Observations of the synchronized mass spawning and postsettlement activity of octocorals on the Great Barrier Reef, Australia: biological aspects. Bull. Mar. Sci. 45, 697–707.
Anthony, K. R. N., and Fabricius, K. E. (2000). Shifting roles of heterotrophy and autotrophy in coral energetics under varying turbidity. J. Exp. Mar. Biol. Ecol. 252, 221–253. doi: 10.1016/S0022-0981(00)00237-9
Baird, A. H., Guest, J. R., and Willis, B. L. (2009). Systematic and biogeographical patterns in the reproductive biology of scleractinian corals. Ann. Rev. Ecol. Evol. Systemat. 40, 551–571. doi: 10.1146/annurev.ecolsys.110308.120220
Baird, A. H, Madin, J. S., Álvarez-Noriega, M., Fontoura, L., Kerry, J. T., Kuo, C., et al. (2018). A decline in bleaching suggests that depth can provide a refuge from global warming in most coral taxa. Mar. Ecol. Prog. Ser. 603, 257–264. doi: 10.3354/meps12732
Bak, R. P. M., Nieuwland, G., and Meesters, E. H. (2005). Coral reef crisis in deep and shallow reefs: 30 years of constancy and change in reefs of Curacao and Bonaire. Coral Reefs 24, 475–479. doi: 10.1007/s00338-005-0009-1
Barneah, O., Weis, V. M., Perez, S., and Benayahu, Y. (2004). Diversity of dinoflagellate symbionts in Red Sea soft corals: mode of symbiont acquisition matters. Mar. Ecol. Prog. Ser. 275, 89–95. doi: 10.3354/meps275089
Battaglene, S. C., Seymour, J. E., Ramofafia, C., and Lane, I. (2002). Spawning induction of three tropical sea cucumbers, Holothuria scabra, H. fuscogilva and Actinopyga mauritiana. Aquaculture 207, 29–47. doi: 10.1016/S0044-8486(01)00725-6
Benayahu, Y. (1990). Xeniidae (Cnidaria: Octocorallia) from the Red Sea, with the description of a new species. Zool. Meded. 64,113–120.
Benayahu, Y. (1991). Reproduction and developmental pathways of Red Sea Xeniidae (Octocorallia, Alcyonacea). Hydrobiologia 21, 125–130. doi: 10.1007/BF00026452
Benayahu, Y. (2002). Soft corals (Octocorallia: Alcyonacea) of the southern Ryukyu Archipelago: the families Tubiporidae, Clavulariidae, Alcyoniidae and Briareidae Y. Galaxea 4, 11–32. doi: 10.3755/jcrs.2002.11
Benayahu, Y., Bridge, T., Colin, P. L., Liberman, R., McFadden, C., Pizarro, O., et al. (2019). Mesophotic Coral Ecosystems, eds Y. Loya, K. A. Puglise, and T. Bridge (New York, NY: Springer).
Benayahu, Y., Jeng, M. S., Perkol-Finkel, S., and Dai, C. F. (2004). Soft corals (octocorallia: alcyonacea) from southern Taiwan, II. Species Diversity and Distributional Patterns. Zool. Stud. 43, 548–560.
Benayahu, Y., and Loya, Y. (1983). Surface brooding in the Red Sea soft coral Parerythropodjum fulvum fulvum (Forskål, 1775). Biol. Bull. 165, 353–369. doi: 10.2307/1541201
Benayahu, Y., and Loya, Y. (1984a). Life history studies on the Red Sea soft coral Xenia macrospiculata Gohar, 1940. I. Annual dynamics of gonadal development. Biol. Bull. 166, 32–43. doi: 10.2307/1541428
Benayahu, Y., and Loya, Y. (1984b). Life history studies on the Red Sea soft coral Xenia macrospiculata Gohar, 1940. II. Planulae shedding and post larval development. Biol. Bull. 166, 44–53.
Bongaerts, P., Frade, P. R., Hay, K. B., Englebert, N., Latijnhouwers, K. R., Bak, R. P., et al. (2015). Deep down on a Caribbean reef: lower mesophotic depths harbor a specialized coral-endosymbiont community. Sci. Rep. 5:7652. doi: 10.1038/srep07652
Bongaerts, P., Ridgway, T., Sampayo, E. M., and Hoegh-Guldberg, O. (2010). Assessing the ‘deep reef refugia’ hypothesis: focus on Caribbean reefs. Coral Reefs 29, 309–327. doi: 10.1007/s00338-009-0581-x
Bongaerts, P., Riginos, C., Brunner, R., Englebert, N., Smith, S. R., and Hoegh-Guldberg, O. (2017). Deep reefs are not universal refuges: reseeding potential varies among coral species. Sci. Adv. 3:e1602373. doi: 10.1126/sciadv.1602373
Bongaerts, P., Tyler, B., and Smith, T. B. (2019). “Beyond the ‘deep reef refuge’ hypothesis: a conceptual framework to characterize persistence at depth,” in Mesophotic Coral Ecosystems, eds Y. Loya, K. A. Puglise, and T. Bridge (New York, NY: Springer).
Brazeau, D. A., and Lasker, H. R. (1990). Sexual reproduction and external brooding by the Caribbean gorgonian Briareum asbestinum. Mar. Biol. 104, 465–474. doi: 10.1007/BF01314351
Brazeau, D. A., Lesser, M. P., and Slattery, M. (2013). Genetic structure in the coral, Montastraea cavernosa: assessing genetic differentiation among and within mesophotic reefs. PLoS One 8:e65845. doi: 10.1371/journal.pone.0065845
Caballes, C. F., and Pratchett, M. S. (2017). Environmental and biological cues for spawning in the crown-of-thorns starfish. PLoS One 12:e0173964. doi: 10.1371/journal.pone.0173964
Charnov, E. L. (1982). The Theory of Sex Allocation, Vol. 18. Princeton, NJ: Princeton University Press.
De Putron, S. J., and Ryland, J. S. (2009). Effect of seawater temperature on reproductive seasonality and fecundity of Pseudoplexaura porosa (Cnidaria: Octocorallia): latitudinal variation in Caribbean gorgonian reproduction. Invertebr. Biol. 128, 213–222. doi: 10.1111/j.1744-7410.2009.00170.x
Eyal-Shaham, L., Eyal, G., Tamir, R., and Loya, Y. (2016). Reproduction, abundance and survivorship of two Alveopora spp. in the mesophotic reefs of Eilat, Red Sea. Sci. Rep. 6:20964. doi: 10.1038/srep20964
Fabricius, K. E., and Alderslade, P. (2001). Soft Corals and Sea Fans: A Comprehensive Guide to the Tropical Shallow Water Genera of the Central-West Pacific, the Indian Ocean and the Red Sea. Crawley WA: Australian Institute of Marine Science.
Farrant, P. A. (1986). Gonad development and the planulae of the temperate Australian soft coral Capnella gaboensis. Mar. Biol. Int. J. Life Oceans Coastal Waters 92, 381–392. doi: 10.1007/BF00392679
Feldman, B., Shlesinger, T., and Loya, Y. (2018). Mesophotic coral-reef environments depress the reproduction of the coral Paramontastraea peresi in the Red Sea. Coral Reefs 37, 201–214. doi: 10.1007/s00338-017-1648-8
Glynn, P. W. (1996). Coral reef bleaching: facts, hypotheses and implications. Global Change Biol. 2, 495–509. doi: 10.1111/j.1365-2486.1996.tb00063.x
Glynn, P. W., Colley, S., Carpizo-Ituarte, E., and Richmond, R. (2017). Coral reproduction in the Eastern Pacific. Mar. Biol. 8, 435–476. doi: 10.1007/978-94-017-7499-4_15
Gori, A., Viladrich, N., Gili, J., Kotta, M., Cucio, C., Magni, L., et al. (2012). Reproductive cycle and trophic ecology in deep versus shallow populations of the Mediterranean gorgonian Eunicella singularis (Cap de Creus, northwestern Mediterranean Sea). Coral Reefs 31, 823–837. doi: 10.1007/s00338-012-0904-1
Goulet, T. L., Simmons, C., and Goulet, D. (2008). Worldwide biogeography of Symbiodinium in Tropical Octocorals. Mar. Ecol. Prog. Ser. 355, 45–58. doi: 10.3354/meps07214
Guest, J. R., Baird, A. H., Goh, B. P., and Chou, L. M. (2005). Seasonal reproduction in equatorial reef corals. Invertebrate Reproduct. Dev. 48, 207–218. doi: 10.1080/07924259.2005.9652186
Halász, A., McFadden, C. S., Aharonovich, D., Toonen, R., and Benayahu, Y. (2014). A revision of the octocoral genus Ovabunda (Alderslade, 2001) (Anthozoa, Octocorallia, Xeniidae). ZooKeys 373, 1–41. doi: 10.3897/zookeys.373.6511
Harrison, P. L. (2011). “Sexual reproduction of scleractinian corals,” in Coral Reefs: An Ecosystem in Transition, eds Z Dubinsky and N Stambler (Dordrecht: Springer), 59–85. doi: 10.1007/978-94-007-0114-4_6
Holstein, D. M., Smith, T. B., Gyory, J., and Paris, C. B. (2015). Fertile fathoms: deep reproductive refugia for threatened shallow corals. Sci. Rep. 5:12407. doi: 10.1038/srep12407
Holstein, D. M., Smith, T. B., and Paris, C. B. (2016). Depth-independent reproduction in the reef coral Porites astreoides from shallow to mesophotic zones. PLoS One 11:e0146068. doi: 10.1371/journal.pone.0146068
Hughes, T. P., and Tanner, J. E. (2000). Recruitment failure, life histories, and long-term decline of Caribbean corals. Ecology 81, 2250–2263. doi: 10.1890/0012-9658(2000)081[2250:RFLHAL]2.0.CO;2
Janes, M. P., McFadden, C. S., and Chanmethakul, T. (2014). A new species of Ovabunda (Octocorallia, Xeniidae) from the Andaman Sea, Thailand with notes on the biogeography of this genus. ZooKeys 431, 1–17. doi: 10.3897/zookeys.431.7751
Kahng, S. E., Benayahu, Y., and Lasker, H. R. (2011). Sexual reproduction in octocorals. Mar. Ecol. Progr. Ser. 443, 265–283. doi: 10.3354/meps09414
Kahng, S. E., Copus, J. M., and Wagner, D. (2014). Recent advances in the ecology of mesophotic coral ecosystems (MCEs). Curr. Opin. Environ. Sustain. 7, 72–81. doi: 10.1016/j.cosust.2013.11.019
Kahng, S. E., Garcia-Sais, J. R., Spalding, H. L., Brokovich, E., Wagner, D., Weil, E., et al. (2010). Community ecology of mesophotic coral reef ecosystems. Coral Reefs 29, 255–275. doi: 10.1007/s00338-010-0593-6
Keith, S. A., Maynard, J. A., Edwards, A. J., Guest, J. R., Bauman, A. G., van Hooidonk, R., et al. (2016). Coral mass spawning predicted by rapid seasonal rise in ocean temperature. Proc. R. Soc. B Biol. Sci. 283:20160011. doi: 10.1098/rspb.2016.0011
LaJeunesse, T. C., Parkinson, J. E., Gabrielson, P. W., Jeong, H. J., Reimer, J. D., Voolstra, C. R., et al. (2018). Systematic revision of Symbiodiniaceae highlights the antiquity and diversity of coral endosymbionts. Curr. Biol. 28, 2570.e6–2580.e6. doi: 10.1016/j.cub.2018.07.008
Lasker, H. R. (2006). High fertilization success in a surface-brooding Caribbean gorgonian. Biol. Bull. 210, 10–17. doi: 10.2307/4134532
Laverick, J. H., and Rogers, A. D. (2017). Experimental evidence for reduced mortality of Agaricia lamarcki on a mesophotic reef. Mar. Environ. Res. 134, 37–43. doi: 10.1016/j.marenvres.2017.12.013
Lesser, M. P., Marc, S., Michael, S., Michiko, O., Gates, R. D., and Andrea, G. (2010). Photoacclimatization by the coral Montastraea cavernosa in the mesophotic zone: light, food, and genetics. Ecology 91, 990–1003. doi: 10.1890/09-0313.1
Lesser, M. P, Slattery, M., and Leichter, J. J. (2009). Ecology of mesophotic coral reefs. J. Exp. Mar. Biol. Ecol. 375, 1–8. doi: 10.1016/j.jembe.2009.05.009
Levitan, D. R. (1991). Influence of body size and population density on fertilization success and reproductive output in a free-spawning invertebrate. Biol. Bull. 181, 261–268. doi: 10.2307/1542097
Levitan, D. R. (2004). Density-dependent sexual selection in external fertilizers: variances in male and female fertilization success along the continuum from sperm limitation to sexual conflict in the sea urchin Strongylocentrotus franciscanus. Am. Natural. 164, 298–309.
Loya, Y., and Sakai, K. (2008). Bidirectional sex change in mushroom stony corals. Proc. R. Soc. B Biol. Sci. 275, 2335–2343. doi: 10.1098/rspb.2008.0675
Mandelberg-Aharon, Y., and Benayahu, Y. (2015). Reproductive features of the Red Sea octocoral Sarcophyton auritum Verseveldt & Benayahu, 1978 are uniform within generic boundaries across wide biogeographical regions. Hydrobiologia 759, 119–132. doi: 10.1007/s10750-015-2225-1
Marshall, D. J., and Keough, M. J. (2007). The evolutionary ecology of offspring size in marine invertebrates. Adv. Mar. Biol. 53, 1–60. doi: 10.1016/S0065-2881(07)53001-4
Mass, T., Einbinder, S., Brokovich, E., Shashar, N., Vago, R., Erez, J., et al. (2007). Photoacclimation of Stylophora pistillata to light extremes: metabolism and calcification. Mar. Ecol. Progr. Ser. 334, 93–102. doi: 10.3354/meps334093
Mcfadden, C. S., Benayahu, Y., Pante, E., Thoma, J. N., Nevarez, P. A., and France, S. C. (2011). Limitations of mitochondrial gene barcoding in Octocorallia. Mol. Ecol. Res. 11, 19–31. doi: 10.1111/j.1755-0998.2010.02875.x
McFadden, C. S., Haverkort-Yeh, R., Reynolds, A. M., Halàsz, A., Quattrini, A. M., Forsman, Z. H., et al. (2017). Species boundaries in the absence of morphological, ecological or geographical differentiation in the Red Sea octocoral genus Ovabunda (Alcyonacea: Xeniidae). Mol. Phylogenet. Evol. 112, 174–184. doi: 10.1016/j.ympev.2017.04.025
Pakes, M. J., and Woollacott, R. M. (2008). Reproduction of the gorgonian Plexaura flexuosa in Bermuda. J. Exp. Mar. Biol. Ecol. 357, 121–127. doi: 10.1016/j.jembe.2008.01.003
Prasetia, R., Sinniger, F., and Harii, S. (2016). Gametogenesis and fecundity of Acropora tenella (Brook 1892) in a mesophotic coral ecosystem in Okinawa, Japan. Coral Reefs 35, 53–62. doi: 10.1007/s00338-015-1348-1
Prasetia, R., Sinniger, F., Hashizume, K., and Harii, S. (2017). Reproductive biology of the deep brooding coral Seriatopora hystrix: implications for shallow reef recovery. PLoS One 12:e0177034. doi: 10.1371/journal.pone.0177034
R Core Development Team (2016). R: A Language and Environment for Statistical Computing. Vienna: R Foundation for Statistical Computing. Available at: http://www.r-project.org/
Richmond, R., and Hunter, C. (1990). Reproduction and recruitment of corals: comparisons among the Caribbean, the Tropical Pacific, and the Red Sea. Mar. Ecol. Progr. Ser. 60, 185–203. doi: 10.3354/meps060185
Rinkevich, B., and Loya, Y. (1979). The reproduction of the Red Sea coral Stylophora pistilata I. Gonads and Planulae. Mar. Ecol. Prog. Ser. 1, 133–144. doi: 10.3354/meps001133
Rinkevich, B., and Loya, Y. (1987). Variability in the pattern of sexual reproduction of the coral Stylophora pistillata at Eliat, Red Sea: a long-term study. Biol. Bull. 173, 335–344. doi: 10.2307/1541546
Santangelo, G., Carletti, E., Maggi, E., and Bramanti, L. (2003). Reproduction and population sexual structure of the overexploited Mediterranean red coral Corallium rubrum. Mar. Ecol. Progr. Ser. 248, 99–108. doi: 10.3354/meps248099
Shlesinger, T., Grinblat, M., Rapuano, H., Amit, T., and Loya, Y. (2018). Can mesophotic reefs replenish shallow reefs? Reduced coral reproductive performance casts a doubt. Ecology 99, 421–437. doi: 10.1002/ecy.2098
Shlesinger, Y., and Loya, Y. (1985). Coral community reproductive patterns: Red Sea versus the Great Barrier Reef. Science 228, 1333–1335. doi: 10.1126/science.228.4705.1333
Shoham, E., and Benayahu, Y. (2017). Higher species richness of octocorals in the upper mesophotic zone in Eilat (Gulf of Aqaba) compared to shallower reef zones. Coral Reefs 36, 71–81. doi: 10.1007/s00338-016-1528-7
Smith, T. B., Gyory, J., Brandt, M. E., Miller, W. J., Jossart, J., and Nemeth, R. S. (2016). Caribbean mesophotic coral ecosystems are unlikely climate change refugia. Glob. Change Biol. 22, 2756–2765. doi: 10.1111/gcb.x13175
Tsounis, G., Rossi, S., Aranguren, M., Gili, J. M., and Arntz, W. (2006). Effects of spatial variability and colony size on the reproductive output and gonadal development cycle of the Mediterranean red coral (Corallium rubrum L.). Mar. Biol. 148, 513–527. doi: 10.1007/s00227-005-0100-8
Van Oppen, M. J. H., Bongaerts, P., Underwood, J. N., Peplow, L. M., and Cooper, T. F. (2011). The role of deep reefs in shallow reef recovery: an assessment of vertical connectivity in a brooding coral from west and east Australia. Mol. Ecol. 20, 1647–1660. doi: 10.1111/j.1365-294X.2011.05050.x
Villanueva, R. D., Yap, H. T., and Montaño, M. N. E. (2008). Timing of planulation by pocilloporid corals in the northwestern Philippines. Mar. Ecol. Progr. Ser. 370, 111–119. doi: 10.3354/meps07659
West, J. M., Harvell, C. D., and Walls, A. M. (1993). Morphological plasticity in a gorgonian coral (Briareum asbestinum) over a depth cline. Mar. Ecol. Progr. Ser. 94, 61–69. doi: 10.3354/meps094061
Wheeler, R. E. (2013). R Documentation Permutation Tests for Linear Models in R. Available at: https://cran.r-project.org/web/packages/lmPerm/vignettes/lmPerm.pdf
Keywords: mesophotic coral ecosystem, Red Sea, gametogenesis, surface-brooding, lunar phase, planula-larvae
Citation: Liberman R, Shlesinger T, Loya Y and Benayahu Y (2018) Octocoral Sexual Reproduction: Temporal Disparity Between Mesophotic and Shallow-Reef Populations. Front. Mar. Sci. 5:445. doi: 10.3389/fmars.2018.00445
Received: 12 May 2018; Accepted: 06 November 2018;
Published: 10 December 2018.
Edited by:
Noga Stambler, Bar-Ilan University, IsraelReviewed by:
Ernesto Weil, University of Puerto Rico at Mayagüez, Puerto RicoCharles Alan Jacoby, St. Johns River Water Management District, United States
Copyright © 2018 Liberman, Shlesinger, Loya and Benayahu. This is an open-access article distributed under the terms of the Creative Commons Attribution License (CC BY). The use, distribution or reproduction in other forums is permitted, provided the original author(s) and the copyright owner(s) are credited and that the original publication in this journal is cited, in accordance with accepted academic practice. No use, distribution or reproduction is permitted which does not comply with these terms.
*Correspondence: Yehuda Benayahu, eWVodWRhYkB0YXVleC50YXUuYWMuaWw=