- 1Department of Marine Sciences, University of Puerto Rico at Mayagüez, Mayagüez, PR, United States
- 2Department of Marine Biology, Texas A&M University at Galveston, Galveston, TX, United States
- 3Gehrmann Laboratories, School of Biological Sciences, University of Queensland, St Lucia, QLD, Australia
The benthic and fish communities of the central portion of the Guánica, Puerto Rico shelf edge were studied to determine species abundance, distributions and species overlap between two depth stratifications, 20 and 45 m, at eight sites. A total of 67 fish species belonging to 21 families were identified. Similar species richness estimates were observed between depths, though fish assemblage composition differed significantly, with observable changes in feeding guild contributions of herbivore and omnivore (20 m) to a deeper assemblage composed of piscivores and planktivores (45 m). Coral assemblages consisted of 31 species at 20 m and 11 species at 45 m, accounting for 17.0% (±1.76 SE) and 2.6% (±0.89 SE) benthic cover for the altiphotic and mesophotic surveys, respectively. The altiphotic and mesophotic coral reef communities support different scleractinian coral assemblages with minimal species overlap. Altiphotic surveys of sponges yielded a higher species richness than mesophotic, with 60 and 54 species respectively, and an overall total of 71 species identified from both depths, with 45 species overlapping (63.0%). The percent cover of sponges surveyed at altiphotic reefs accounted for 9.0% (±1.04 SE), while the percent cover of sponges surveyed at mesophotic reefs was 14.0% (±1.96 SE). Our data show fish, coral, and sponge assemblages are differentiated between 20 and 45 m along the Guánica shelf edge offshore of the Guánica Biosphere Reserve. This study represents the first observations for species distributions of adjacent altiphotic and mesophotic coral reef habitats along the Guánica shelf edge, as well as provides an annotated species list of the local sponge fauna. Combined, these results highlight the need for continued environmental stewardship and conservation in the area.
Introduction
Mesophotic coral ecosystems (MCEs) are found on insular and continental slopes between 30–40 and 150 m in the tropics (Hinderstein et al., 2010; Locker et al., 2010; Slattery et al., 2011). They are characterized by low light, decreasing water temperatures, upwelling of nutrients, as well as providing unique ecological niches for benthic and mobile fauna (Lesser et al., 2009; Sherman et al., 2010). Research on MCEs has increased substantially over the past two decades (Kahng et al., 2010, 2014; Loya et al., 2016). This is largely due to the combination of increased ease of in situ data observations and collections through underwater technological advancements (i.e., SCUBA, closed circuit rebreathers, remotely operated vehicles, and submersibles) and the appraisal of MCEs as potential refuges for adjacent altiphotic (i.e., shallow reef habitats adjacent to upper mesophotic reefs; see Baldwin et al., 2018) coral reefs (Glynn, 1996; Lesser et al., 2009; Bongaerts et al., 2010; Hinderstein et al., 2010). As deep-water refuges, MCEs may provide a haven for scleractinian corals and commercially important fish (Lindfield et al., 2016), thereby replenishing adjacent impacted shallow water reefs with both coral (Bongaerts et al., 2017; Kahng et al., 2017) and fish larvae (Bejarano et al., 2014; Vaz et al., 2016), leading to the formation of the deep reef refuge hypothesis (Bongaerts et al., 2010).
Despite their depth (~30–120 m), mesophotic reefs are not immune to stressors and can experience environmental degradation at levels comparable to nearby altiphotic coral reefs (Lesser and Slattery, 2011; Loya et al., 2016; Smith et al., 2016). For instance, mesophotic reefs in the Bahamas experienced drastic reductions in coral and sponge coverage associated with a lack of herbivorous fish, which was caused by predation from the invasive lionfish (Lesser and Slattery, 2011). The effect of this outbreak casts doubts on whether MCEs are more resilient than their altiphotic counterparts to the effects of invasive species (Lesser and Slattery, 2011). In addition, MCEs closer to shore, and especially near terrestrial outflows and coastal development, exhibit decreased coral coverage and smaller associated fish assemblages (Appeldoorn et al., 2016a; Baker et al., 2016; MacDonald et al., 2016). Thus, mesophotic reefs cannot be assumed to be buffered from natural and anthropogenic stressors solely because of their increased depth or distance from the coast (Smith et al., 2016; White et al., 2017).
Recently, more attention has been given to MCEs in the Atlantic and Caribbean region, including research off Puerto Rico (Galindo-Estronza et al., 2016; Veglia et al., 2018), the US Virgin Islands (Holstein et al., 2016; Weinstein et al., 2016), Curaçao (Hoeksema et al., 2016; Glasl et al., 2017), Bermuda (Goodbody-Gringley et al., 2015), Honduras (Laverick et al., 2017), Belize (Lesser and Slattery, 2013), the Bahamas (Slattery and Lesser, 2012; Slattery et al., 2016), Little Cayman (Brazeau et al., 2013), Colombia (Gonzalez-Zapata et al., 2018), and Mexico (Gress et al., 2017). In Puerto Rico, the primary focus of past (e.g., Starck and Colin, 1978; Nelson and Appeldoorn, 1985; Dennis et al., 2004) and more recent work (Sherman et al., 2010, 2013a,b) has been on MCEs located off the south coast of the island (see review by Appeldoorn et al., 2018). Along this coast, benthic coral coverage tends to increase east to west along the insular continental shelf edge; from Ponce, a major city and a maritime port, to La Parguera Natural Reserve (Appeldoorn et al., 2016b). In general, benthic coral coverage and tridimensional complexity are affected regionally by sediment transport levels and the slope degree of the insular shelf edge (Sherman et al., 2016), both of which change rapidly over small distances (Sherman et al., 2010, 2013a,b). The well documented nature of this area (García-Sais et al., 2017), combined with the influence of freshwater outflow from the nearby coastline (Whitall et al., 2013a), allows for comparisons between less affected MCEs to the west (La Parguera) and more affected MCEs to the east (Ponce) (Appeldoorn et al., 2016a).
Located centrally between Ponce and La Parguera, the Guánica Biosphere Reserve was created in 1981 to protect terrestrial, coastal, and marine (strictly within the insular shelf-break, < 30 m) ecosystems (García-Sais et al., 2001). Detailed studies regarding Guánica's marine ecosystem have only begun taking place within the last 20 years (Sherman et al., 2010, 2013b, 2016). Several concerns persist for the area, such as the introduction of pollutants and terrigenous sediments flowing in through the Rio Loco system (Morelock et al., 1994; Sherman et al., 2013a). Further coastal development in the city of Ponce (García-Sais et al., 2005) is of concern as well due to the westerly currents created by the northeast-trade winds, which has the potential to significantly decrease water quality, affecting shallow and mesophotic coral reefs west of Ponce (Figure 1).
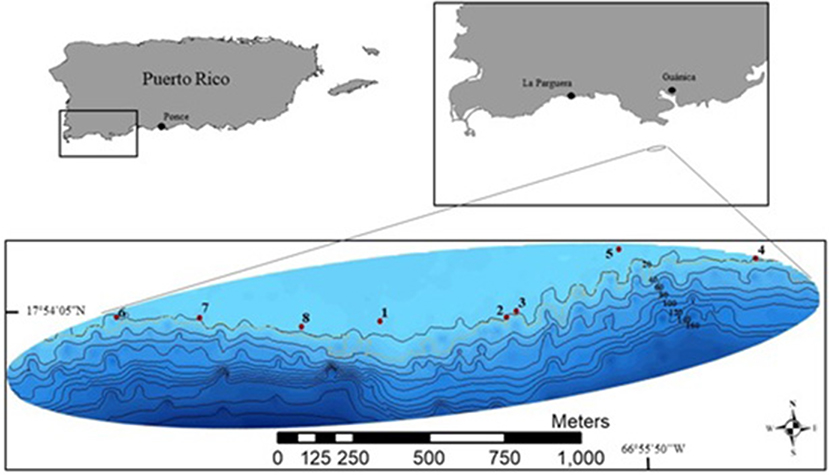
Figure 1. Site map for the central portion of the Guánica shelf edge, Puerto Rico. Numbers one to eight represent each specific transect area for the shallow (20 m) surveys, with the mesophotic (45 m) surveys being done directly adjacent to the shallow surveys.
Though many studies in Puerto Rico have investigated both the physical conditions (e.g., bathymetry, sediment transport, prevailing hydrodynamic forces, etc.) (Lumsden et al., 2007; Hubbard et al., 2008; Locker et al., 2010; Armstrong and Singh, 2012; Sherman et al., 2013a,b; Whitall et al., 2013a; Appeldoorn et al., 2016a,b) and biological components (Armstrong et al., 2009, 2011; Bauer et al., 2013; Ballantine et al., 2016) of these deep coral reef habitats, few have attempted to compare the ecology between altiphotic and mesophotic reefs (García-Sais, 2010; Pittman et al., 2010; Bejarano et al., 2014; Lucas et al., 2016; Hammerman et al., 2017). In Puerto Rico, even less studies have explored the diversity, abundance, disease and biomass of altiphotic and mesophotic reef sponges (e.g., Vicente, 1990; Weil, 2005; Rivero-Calle, 2010). Within the Caribbean region, sponges are considered one of the most visually dominant and diverse taxa inhabiting shallow and deep coral reef ecosystems (Rützler, 2004; Wulff, 2012; Slattery et al., 2013). In Puerto Rico, one of the first studies regarding marine sponges were carried out by Wilson (1902) and de Laubenfels (1934). Wilson (1902) reported a total of 41 new sponges from Puerto Rico and the remainder of the Greater Antilles ecoregion. Three decades later, de Laubenfels (1934) analyzed a large collection of Puerto Rican deep water sponges which were collected during the First Johnson-Smithsonian Deep-Sea Expedition, identifying 27 species of sponges. In the late twentieth century, Vicente (1990) produced the first list of sponge species (35) associated with photosynthetic symbionts from shallow surveys off La Parguera Natural Reserve. Followed by Weil (2005), who produced an updated systematic list of marine invertebrates from Puerto Rico, emphasizing that no major taxonomic revisions of sponges have been done in the last 60 years.
Thus, the aim of this study is to characterize, describe and compare the biological composition along the Guánica shelf edge to: (1) rest if there is a difference in the presence, overlap, and abundance of coral, fish, and sponge species between altiphotic (20 m) and mesophotic (45 m) coral reefs, and (2) to provide an annotated list of sponges encountered within our surveys, since the true diversity of Puerto Rican sponges is currently not known. This comparison will give insight into the dynamics of the Guánica MCE, which is adjacent to the less impacted and better studied MCEs of La Parguera, and whether Guánica could act as a refuge for important coral, fish, and sponge species.
Materials and Methods
Site Selection
Surveys were conducted in two depth stratifications, classified here as either altiphotic (20 m) or mesophotic (45 m) (Figures 2A,B). Eight altiphotic sites were chosen randomly along the central extension of the Guánica shelf edge using the program ARCGIS v. 10 (ESRI, 2011). The most direct downslope reef at 45 m respective to each altiphotic site was then selected for the mesophotic surveys (Figures 1, 2I–K). The maximum distance between randomly selected altiphotic survey sites was roughly 1.5 km, and the minimum was at least 50 m apart.
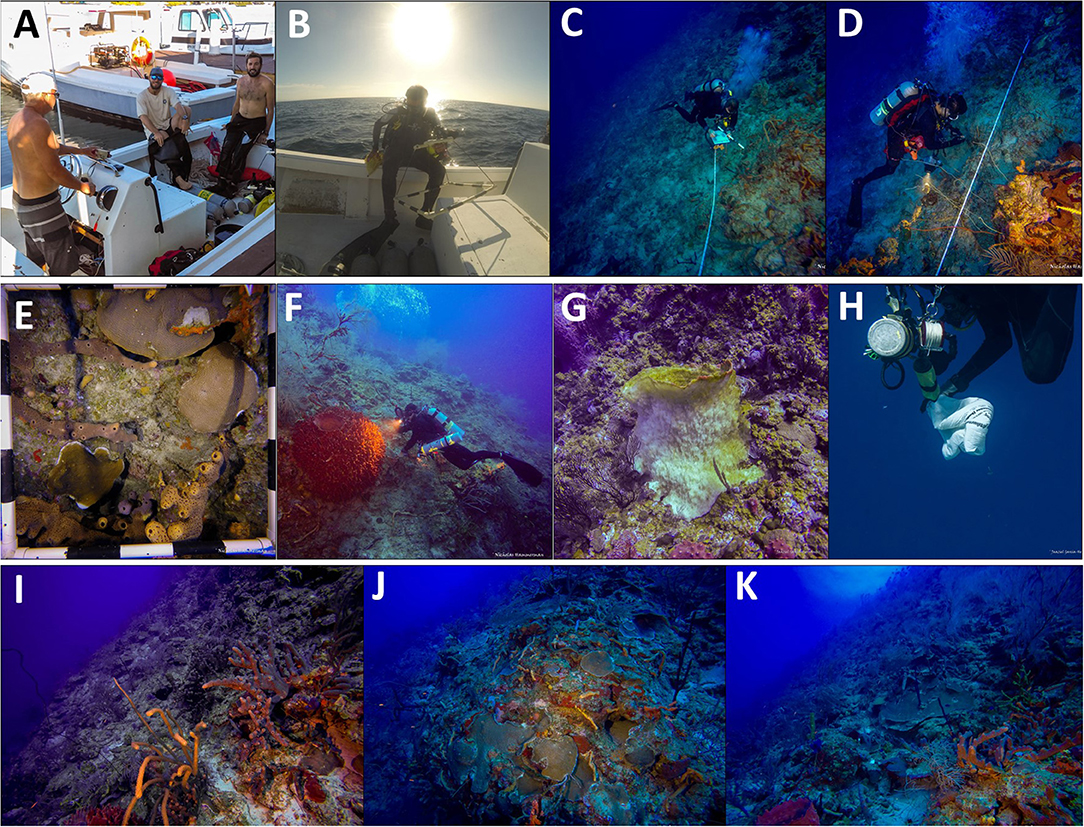
Figure 2. (A) Research team, Milton Carlo, Phillip Sanchez, Nicholas Hammerman. (B) NMH with photo-quadrat. (C) PJS performing fish surveys. (D) JEGH performing visual sponge surveys/collections. (E) Photoquadrat example. (F) Healthy X. muta at 40 m, PJS for size reference. (G) Specimen of X. muta affected by unknown disease or bleached. (H) Plastic bag recovered by NMH. (I–K) Upper mesophotic coral reefs along the Guánica continental shelf edge (45–50 m).
Fish Sampling
A 15 × 3 m belt transect survey, over a standardized 10-min duration, was conducted at each site on SCUBA (Figure 2C). Altiphotic transects (20 m) were run on top of the benthos, while mesophotic transects (45 m) were run alongside the slope. Skittish fish were documented at the onset of the survey due to their likelihood of diver avoidance; common and cryptic species were targeted for the remainder of the survey along the transect. Fish were identified to species and lengths estimated within five cm bins up to 30 cm, after which they were classified as greater than 30 cm. While retrieving the transect tape, the point of highest rugosity (area of largest uninterrupted relief perpendicular to the surface of the benthos) was measured to the nearest cm using a 1 m long measuring stick, for each 1 m-length of the transect, extending 1.5 m to each side (1 × 3 m bin), resulting in 15 rugosity measurements per transect. A roving fish survey was conducted simultaneously with the transect survey and rugosity measurement collection to document the presence of meso-predators unlikely to be encountered within the limited spatial scale of the belt transect. In the roving survey, all meso-predators and lionfish observed were identified to species with lengths estimated to the nearest cm. No distance of fish to diver restrictions were placed on the roving surveys. The belt-transects and roving survey datasets were then combined into a single dataset for assemblage analysis. No length data was ultimately used in the analyses.
Fish Data Analysis
Species percent frequency (frequency of occurrence seen in transects) and density (ind./45 m2) were calculated for the 20 and 45 m depth transects. Differences in fish abundance and richness between depths were analyzed using Welch's t-tests. Species were categorized into the following feeding guilds: herbivores, mobile invertivores, omnivores, piscivores, planktivores, and sessile invertivores. Classifications were based on a combination of Randall (1967), Bejarano et al. (2014), and fishbase.org.
A nonmetric multidimensional scaling (NMDS) ordination analysis was used to inspect the relationship between the species assemblages across sites coupled with a vector fit of the environmental variables depth, mean rugosity (per transect), and maximum rugosity (per transect). ANOSIMS were ran to analyze the effects of the environmental variables on fish assemblages and a multi-response permutation procedure (MRPP) was used to statistically analyze the effects of depth on differences of fish assemblages in R package “VEGAN” (Oksanen et al., 2017). Distances were calculated using the Bray-Curtis dissimilarity. The NMDS was inspected using a stress plot and goodness-of-fit test. Individual species contributions to assemblage differences between depths were analyzed using an indicator species analysis (ISA) in R-package “INDICSPECIES” (De Cáceres and Legendre, 2009).
Coral and Sponge Photographic Documentation
A total of 320 photo-transect images using a 0.50 m2 quadrat, across 16 surveys (altiphotic and mesophotic), were acquired and analyzed (Figure 2B). Quadrate photos were taken at one-meter increments along the first 10 m of the fish belt transect, one photo on each side of the tape. This resulted in 20 photos per transect, totaling a 10 m2 image field per transect (Figure 2E). All photographs were then exported to CPCe for visualization and quantification (Kohler and Gill, 2006). The average percent cover and abundance of benthic groups, including corals and sponges, were calculated accordingly for further analysis. Within CPCe every coral's parameter was outlined to get a total percent cover per photoquadrat. Sponges were identified to lowest taxonomic identity using relevant identification guides (Zea et al., 2014) and morphological analysis to species that were uncertain.
Benthic Composition Analysis
Custom bash shell scripts were used to quantify average coral and sponge percent cover and abundance (Supplementary Table 1). Percent cover was calculated by averaging coral or sponge cover from all eight sites per depth and variance was shown with standard error (Figures 6–8). Order was depicted in Figure 8, since family level delineation created values too small to accurately or easily portray on a graph. Diversity indices were calculated per coral and sponge species respective to either solely the altiphotic or mesophotic transects (e.g., Species Richness, Simpson's Index, Simpson's Index of Diversity, Simpson's Reciprocal Index, Shannon-Wiener Index and Evenness) (Tables 4, 5). Sorting of coral and sponge specimens resulted in a data matrix for numerical abundance of different taxa that were used to construct similarity matrices among transects using the Bray-Curtis index. Dissimilarity matrices were used to perform non-metric multivariate ordinations (NMDS) to illustrate patterns of spatial distribution of coral and sponge assemblages associated with altiphotic and mesophotic transects. All analyses were done in RStudio (R version3.2.4) running the Vegan, MASS, and DPLYR Packages (R Core Team, 2016).
Results
Fish Abundance and Diversity
A total of 67 species belonging to 21 families were recorded. Forty-eight species (19 families) were recorded at 20 m depth and 47 species (18 families) were recorded at 45 m depth. A total species list with both frequency of occurrence and density (ind./45 m2) are given in Table 1. Though overall richness is comparable at 20 and 45 m, mean abundance and diversity per transect at the two depths are different (Figure 3). Fifteen of the twenty-one families have higher abundances at 20 m. Six families (Epinephelidae, Gobiidae, Gramatidae, Pomacanthidae, Scorpaenidae, and Serranidae) however, have higher abundances at 45 m. Pomacentridae (9 species) and Serranidae (7) were the most speciose families represented, but displayed opposite trends in abundance between the two depths. Pomacentridae abundance at 20 m is greater than at 45 m depth, while Serranidae abundance is higher at 45 m than 20 m. These fish families were followed in decreasing order by Haemulidae (6), Pomacanthidae (5), Chaetodontidae (4), Holocentridae (4), Labridae (4), Lutjanidae (4), and Scaridae (4).
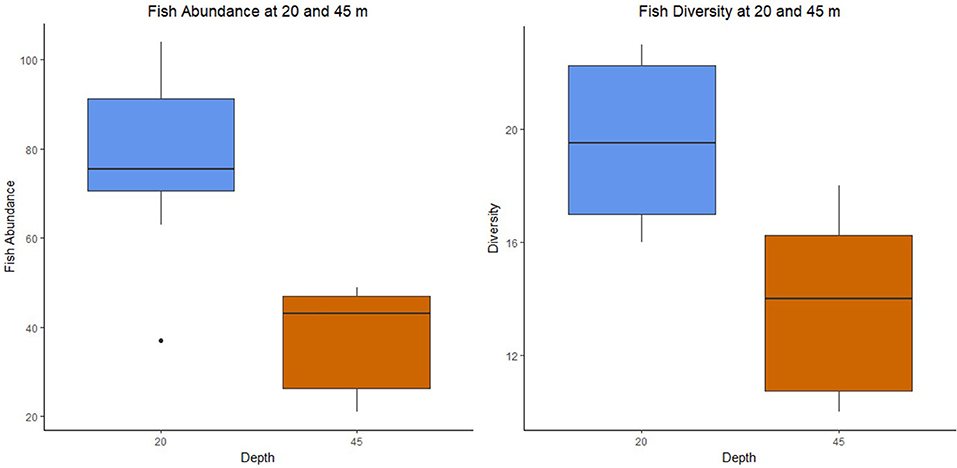
Figure 3. Differences in fish abundance (Welch's T-test, p = 0.001) and diversity (Welch's T-test, p = 0.002) at 20 and 45 m. An average of 59.9 fish and 16.6 species were recorded in all transects, with a mean of 77.4 individuals and 19.6 species at 20 m depth, and 37.6 individuals and 13.8 species at 45 m depth.
Maximum and average rugosity were collinear (Pearson's, p-value < 0.01), and neither were found individually to be significant as a covariable with depth and so were removed from species assemblage comparisons (ANOSIM, p-values = 0.351 and 0.191, respectively). However, assemblages are significantly different between 20 and 45 m (MRPP, p = 0.002) (Figure 5). Ten species are significantly associated with the depth-related assemblage differences; eight species correlated with 20 m and an additional two species at 45 m (Multilevel pattern analysis, p < 0.05). Five of the eight species (Ocyurus chrysurus, Scarus taeniopterus, Scarus iseri, Sparisoma virides, and Stegastes partitus) identified at 20 m are classified as herbivorous or omnivorous. On the other hand, Chromis insolata and Neoniphon marianus, identified at 45 m, are a planktivore and mobile invertivore, respectively (Table 2). Lionfish (Pterois volitans) were seen at both depths with regular frequency of occurrence (Tables 1, 2).
Effects of depth on feeding guild composition changes were not statistically analyzed due to data limitations. The following description therefore does not reflect statistics results, but the trends found in the data play a potential role in assemblage divergences and are supported in the current body of literature. This is further addressed in the Discussion. Herbivores, planktivores, and mobile invertivores composed greater than 80% fish abundance at 20 m, accounting for 28.3, 27.0, and 25.7% of all individuals recorded, respectively (Figure 4; Table 3). However, at 45 m, the relative abundance of herbivores was half of that at 20 m, while the relative abundances of planktivores and mobile invertivores were higher at 45 m. The only guild with a higher overall abundance at the deeper sites was the piscivores (12.9%), compared to 4.5% at 20 m (Figure 4; Table 3).
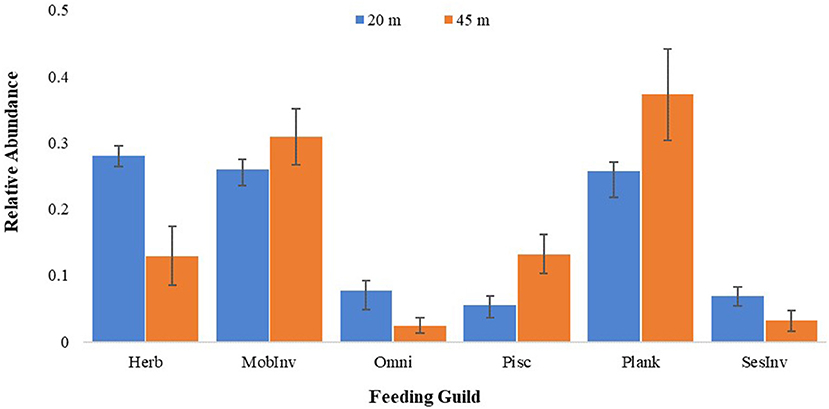
Figure 4. Mean relative abundance of fish feeding guilds in 20 and 45 m surveys. Bars represent standard errors.
Scleractinian Corals Abundance, Percent Cover, and Diversity
A total of 33 coral species were recorded in the 16 transect surveys. Thirty-one species (16 genera) of corals were recorded at 20 m, while eleven species (6 genera) were recorded at 45 m (Table 4). Of the 33 species of coral observed, nine species had overlapping distribution between altiphotic and mesophotic surveys. The most abundant genera within the altiphotic surveys were Orbicella and Porites. The most abundant species in the shallow surveys were Orbicella franksi (N = 185) and Porites astreoides (N = 117). In contrast, the most abundant genera within the mesophotic surveys were Madracis (N = 49) and Agaricia (N = 58), with the corals Madracis senaria and Agaricia grahamae solely encountered on the 45 m surveys.
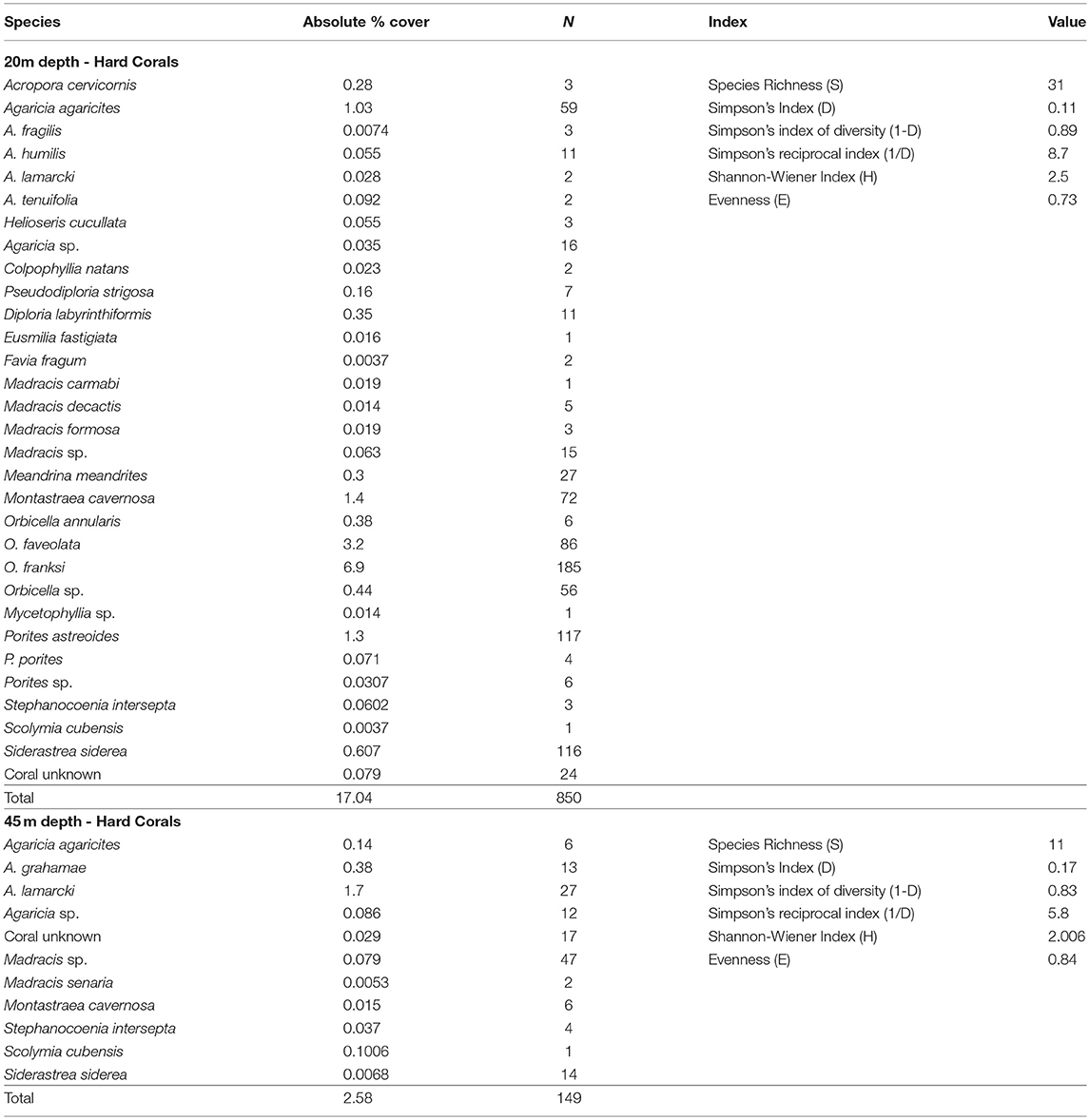
Table 4. Scleractinian species identified in deep (45 m) and altiphotic (20 m) transect surveys from the central portion of Guánica shelf edge, southwest Puerto Rico; ~ 40 m2 was surveyed per depth designation.
The coral species with the greatest percent coverage for the 20 m surveys were O. franksi (6.9%) and O. faveolata (3.2%) followed by Montastraea cavernosa and P. astreoides (1.3%, for both). Agaricia lamarcki constituted the largest percent cover for the 45 m surveys at 1.6% (Table 4). Hard corals accounted for 17.0% (±1.76 SE) and 2.6% (±0.89 SE) benthic cover for the altiphotic and mesophotic surveys, respectively (Figure 6). The altiphotic and mesophotic coral communities support vastly different scleractinian assemblages with minimal species overlap, as represented with the nonmetric multidimensional scaling (NMDS) using Bray-Curtis similarities (Figure 5). This result was supported by PERMANOVA analysis, with a significant division between the depth stratifications (PERMANOVA: df = 1, r2 = 0.560, P = 0.001). However, among sites per depth, coral assemblages were not significantly different (PERMANOVA: df = 7, r2 = 0.239, P = 0.897).
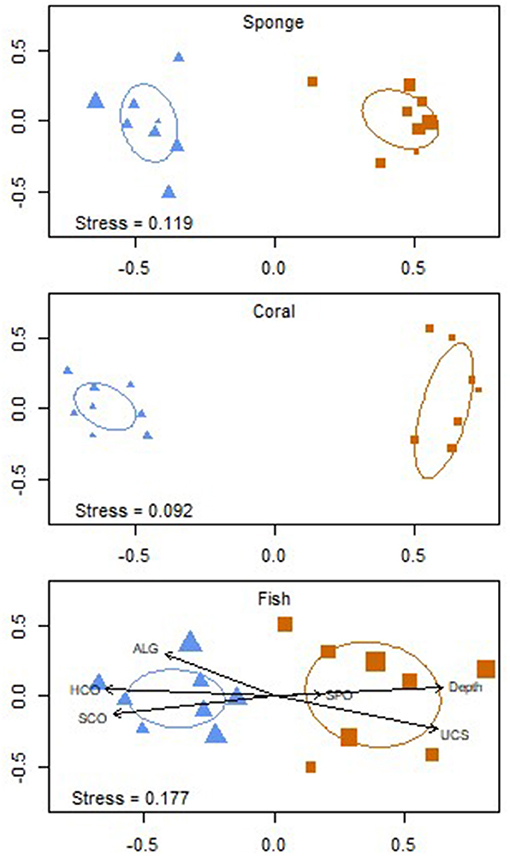
Figure 5. Non-metric multidimensional scaling (NMDS) plots of the sponge, coral, and fish assemblages surveyed at 16 sites (8 at 20 m and 8 at 45 m
depths) at the Guánica shelf edge along the southern coast of Puerto Rico. Distances calculated using Bray-Curtis similarities. Ellipses represent 1 standard deviation. Site characters scaled to goodness of fit (GOF). GOF ranges: Sponge [20 m (0.015–0.040), 45 m (0.024–0.04)], Coral [20 m (0.016–0.023), 45 m (0.016–0.034)], Fish [20 m (0.028–0.051), 45 m (0.035–0.059)].
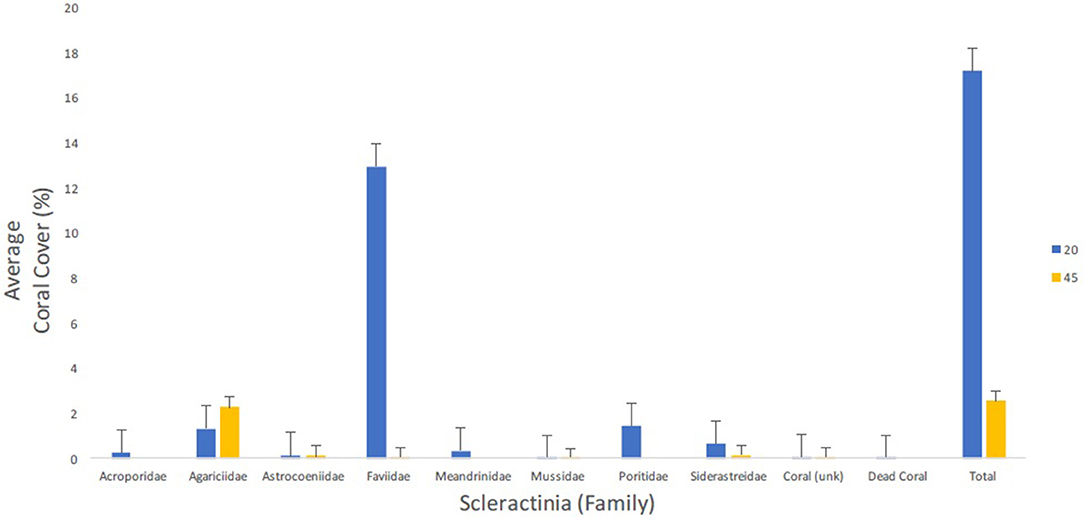
Figure 6. Average percent cover (+SE) per coral family from both the altiphotic and deep transect surveys along the central portion of the Guánica shelf edge. A total of 40 m2 were surveyed per depth stratification and percent cover was calculated in CPCe and tabulated using custom Linux scripts.
Additional functional groups analyzed were algae and unconsolidated sediment, though were not the central theme of this investigation. Algae accounted for 19.0% (±2.20 SE) cover at 20 m, and 7.7% (±1.92 SE) cover at 45 m. Further identification of algal species was not performed (see Ballantine et al., 2016; van der Loos et al., 2017). Lastly, accumulation of hardground/unconsolidated sediments accounted for 52.0% (±1.76 SE) at 20 m and 75.0% (±3.41 SE) at 45 m (Figure 7).
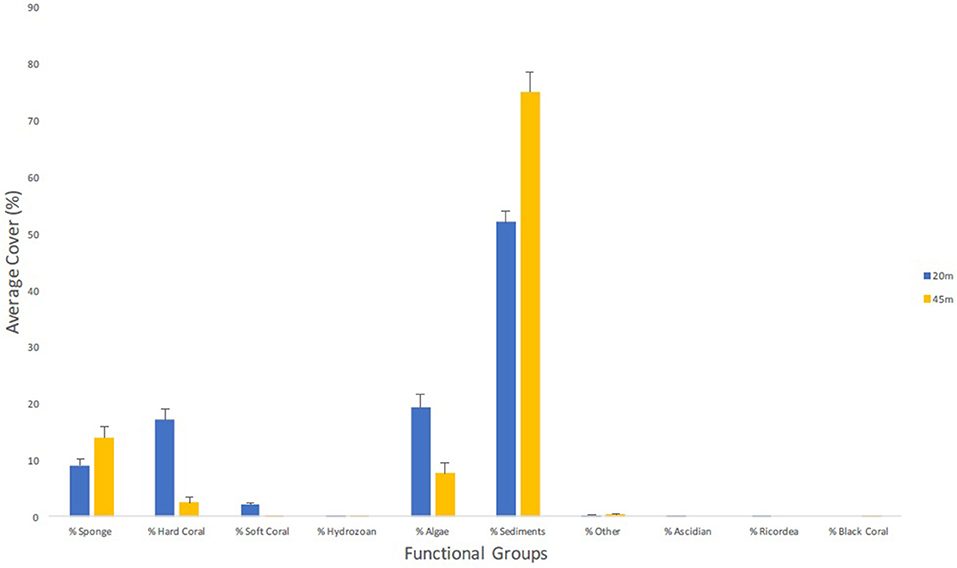
Figure 7. Average percent cover (+SE) of major functional groups from both the altiphotic and deep transect surveys along the central portion of the Guánica shelf edge. A total of 40 m2 were surveyed per depth stratification and percent cover was calculated in CPCe and tabulated using custom Linux scripts.
Sponge Abundance, Percent Cover and Diversity
A total of 3,464 individual sponges were recorded, 1,163 throughout altiphotic reefs (20 m), and 2,301 for mesophotic reefs (45 m). Comparing both depth ranges, altiphotic surveys revealed a higher species richness than mesophotic, 60 and 54 species respectively (Table 5), with a total of 71 species found at both depths (63.0% species overlap). As for depth specific species, altiphotic surveys revealed a higher number of species (N = 17) compared to those found in mesophotic surveys (N = 11). The percent cover of sponges surveyed at altiphotic reefs was 9.0% (±1.04 SE), while percent cover of sponges surveyed at mesophotic reefs was 14.0% (±1.96 SE) (Figure 8; Table 5).
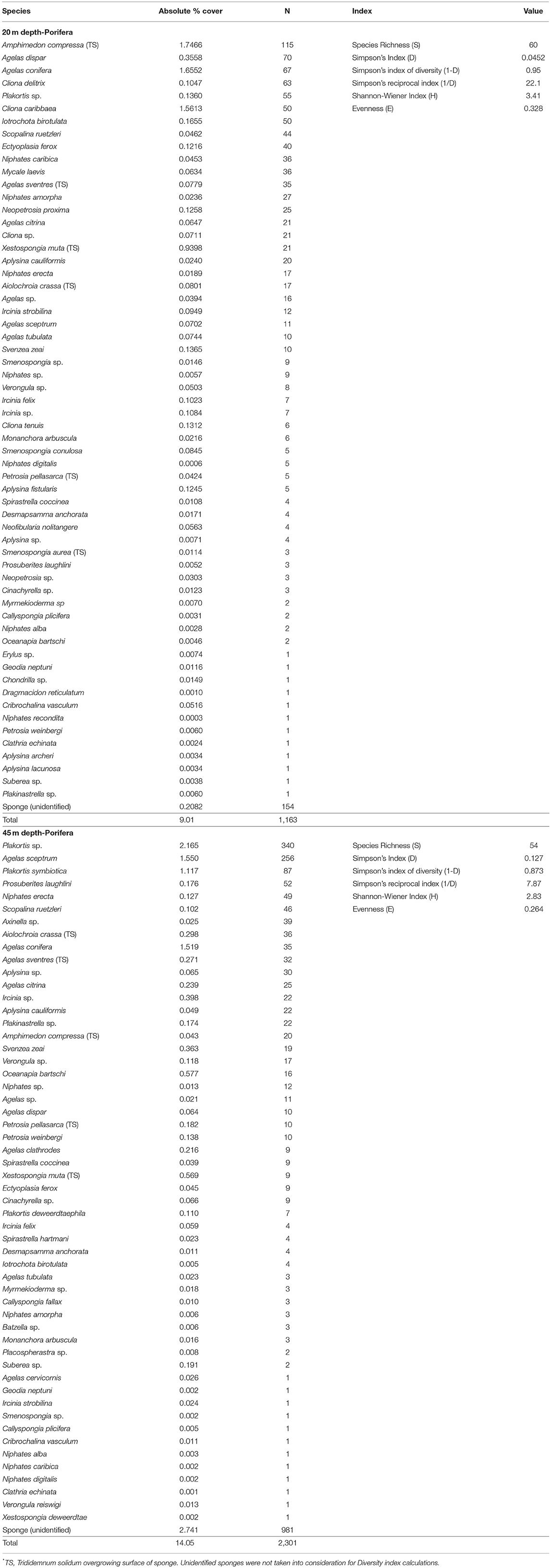
Table 5. Marine species identified in altiphotic (20 m) and deep (45 m) transect surveys from the central portion of the Guánica shelf edge, ~ 40 m2 was surveyed per depth designation.
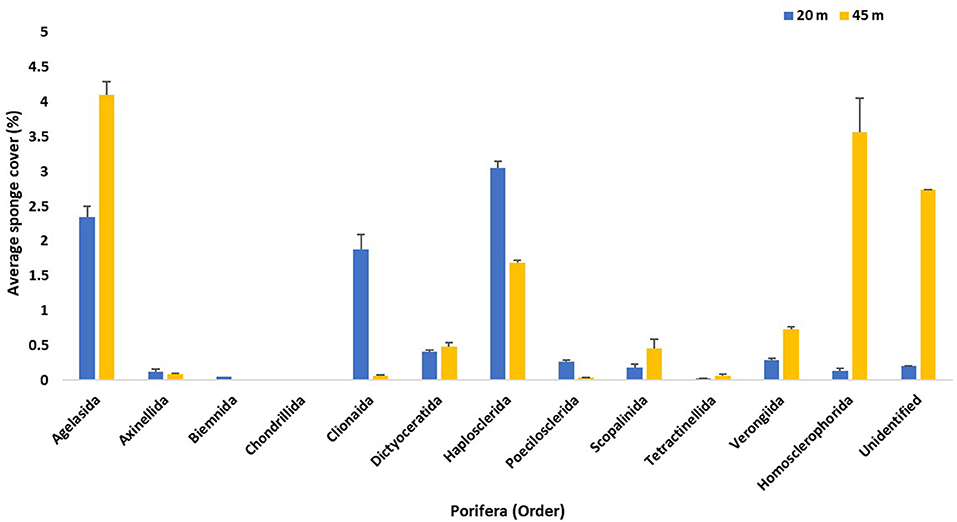
Figure 8. Average percent cover (+SE) per sponge Orders from both the altiphotic and deep transect surveys along the central portion of the Guánica shelf edge. A total of 40 m2 were surveyed per depth stratification and percent cover was calculated in CPCe and tabulated using custom Linux scripts.
Within the total sponge percent cover calculated from altiphotic surveys, the sponges Amphimedon compressa (1.8%), Agelas conifera (1.7%), Cliona caribbaea (1.6%), Xestospongia muta (0.9%), and Agelas dispar (0.4%) comprised the largest percent cover at this depth. With respect to total abundance, the sponges A. compressa (N = 115), Agelas dispar (N = 70), A. conifera (N = 67), Cliona delitrix (N = 63), and Plakortis sp. (N = 55) dominated the altiphotic reef substratum. In contrast, within the total percent sponge cover calculated from mesophotic reef sites, the sponges Plakortis sp. (2.2%), Agelas sceptrum (1.6%), A. conifera (1.5%), Plakortis symbiotica (1.0%), and Oceanapia bartschi (0.6%) comprised the largest percent cover at 45 m. With respect to total abundance, the sponges Plakortis sp. (N = 340), A. sceptrum (N = 256), P. symbiotica (N = 87), Prosuberites laughlini (N = 52), and Niphates erecta (N = 49), dominated the mesophotic reef substratum (Table 5). Overall, demosponges dominated numerically and visually both our altiphotic and mesophotic coral reef benthic surveys.
NMDS analysis showed two major clusters of sponges, suggesting that depth significantly influenced abundance and composition of sponge species (Figure 5) (PERMANOVA: df = 1, r2 = 0.591, P = 0.001), however no significant difference was observed between sites per depth (PERMANOVA: df = 7, r2 = 0.027, P = 0.866).
Discussion
Fish Assemblage
The fish abundance and species richness per survey in Guánica decreased with increased depth, including a 51.4% decrease in mean fish abundance (Figure 3). The two depths, however, exhibit similar levels of overall richness (48 and 47 total number of species) and depth specialists, species unique to a single depth (18 and 17 species). Fifty-two percent of the recorded fish species are unique to either the 20 or 45 m surveys, suggesting a divergence in fish assemblages evident in Figure 5. In nearby La Parguera, a distinct MCE fish assemblage was found at similar depths, between 40 and 70 m (Bejarano et al., 2014). Similar trends with depth were found in fish assemblages from eastern Australia, Hawaii, and Brazil (Pearson and Stevens, 2015; Pyle et al., 2016; Rosa et al., 2016), though the depth that a distinct MCE assemblage develops can vary with geographical location (Pinheiro et al., 2016).
Our data shows that fish assemblage differences include a potential beginning transition at shallow from herbivore and omnivore to a deeper assemblage composed of more piscivores and planktivores (Figure 4). Decreased light availability at mesophotic depths limits primary productivity potential for benthic autotrophs, and therefore food availability for grazers (Brokovich et al., 2010; Sherman et al., 2010). In our benthic surveys, coral percent cover decreased while sponge percent cover increased, supporting the likelihood of this light availability transition.
Terrigenous sediment outflow has also been shown to effect benthic community structure both locally (Torres, 2001; Sherman et al., 2010; Appeldoorn et al., 2016a) and along other coastal zones (MacDonald et al., 2016). However, neither light availability nor bay outflow data were collected in this study, therefore the observed changes in benthos and associated fish assemblages cannot be directly attributed to these physical parameters.
The increase in fish planktivore and mobile invertivore relative percentages at 45 m, combined with decreased herbivore concentration, is indicative of a transition toward a plankton-based trophic web between the two depths (Brokovich et al., 2008). The vertical geomorphology of the shelf slope provides access to deeper water through potential upwelling, and therefore higher concentrations of zooplankton, which support previous results from Puerto Rico (García-Sais et al., 2010a). However, schooling planktivorous fishes (e.g., Ocyurus chrysurus, Clepticus parrae, etc.) congregated in the water column just seaward from the top of the Guánica shelf edge (PJS pers. obs.). Therefore, the adjacent nature of the altiphotic survey sites to the vertical escarpment of the shelf edge could have minimized the magnitude of change for the observed feeding guild transition, which may become more evident with increased cross-shelf surveys.
The addition of an insular shelf sampling band (closer to shore) could clarify if assemblage changes increase even more moving along the shelf perpendicular to the shelf edge (e.g., MacDonald et al., 2016). Conversely, additional transects further down the shelf edge (>50 m) would allow us to determine if the fish assemblages continue to diverge from its shallow water counterpart (e.g., altiphotic vs. deep mesophotic) as is shown in the adjacent La Parguera (Bejarano et al., 2014).
Coral Assemblage
The central portion of the Guánica shelf edge had 31 and 11 coral species at 20 and 45 m, respectively, nine of which have distributional overlaps (Figures 5, 6; Table 4). The geomorphology of MCEs in the area, as originally described by Sherman et al. (2010), could be a key factor in the lack of corals in the 45 m surveys, indicative of the predominate southeast facing walls surveyed. Here, sediment transport is amplified across open and low relief slopes, which may hinder coral recruitment by smothering newly settled juvenile corals (Sherman et al., 2013b). However, where sediments, light attenuation, and slope are not key factors in coral propagation, mesophotic reefs to the east at El Seco (Vieques, PR) had an astonishing 41.0% hard coral coverage (García-Sais et al., 2014, 2016), as well as MCEs in St. Thomas (USVI), which had a maximum coral cover of 70.0% between depths of 38–40 m (Armstrong et al., 2006).
In contrast, the spur and groove bathymetry which typified the 20 m surveys, afforded corals ample substrate to thrive and accounted for 17.0% (±1.76 SE) overall benthic coverage. A similar percentage of hard coral coverage was recorded in Guánica at 10–30 m (García-Sais et al., 2017). In our phototransects, O. franksi (N = 185) and P. astreoides (N = 117) were the most common species, while O. franksi and O. faveolata accounted for the highest individual coral coverage, 6.9 and 3.2%, respectively (Figure 6; Table 4). Orbicella and Porites are thought to be the most abundant genera in the Caribbean due to their variable genomic responses to thermal anomalies (Wegley et al., 2007; Dziedzic et al., 2017), advantageous morphologies for sediment removal and ability to broadcast spawn to increase dispersal potential (Miller and Mundy, 2003; Cowen and Sponaugle, 2009). Combined, these traits might predisposition these genera to recover in areas where coral mortality due to bleaching and disease is prevalent. For instance, in several deep reefs off the US Virgin Islands (~40 m depth), the O. annularis complex dominated benthic composition accounting for roughly 16.0% of total coral coverage (Armstrong et al., 2006; Smith et al., 2010). This species complex is also the most important contributor to percent cover of reefs across the Puerto Rican basin, though since 2005, bleaching events have drastically diminished the presence of the species complex (García-Sais et al., 2017). The other most abundant coral species P. astreoides, is a highly adaptable species which can quickly alter zooxanthellae composition with changing environmental conditions and has thermo-tolerant bio-physiology (Wegley et al., 2007; Serrano et al., 2016). Porites astreoides dominated the coral composition at both 15 and 20 m depth within study sites at Isla Desecheo (west coast of Puerto Rico) and has increased its relative abundance in relation to other corals since the 2005 bleaching event in many reefs around Puerto Rico (García-Sais et al., 2017).
The Agaricia genus was found in both depths but often was difficult to differentiate species due to observed morphological convergence and phenotypic plasticity (Todd, 2008). Agaricia did account for the largest number of individuals and percent cover for the mesophotic surveys, with A. lamarcki being the most prevalent species (N = 27). A similar trend in prevalence of Agaricia spp. was observed for mesophotic reefs at Desecheo, being the predominate coral to a depth of at least 60 m (García-Sais, 2010) and at 70–85 m in Curaçao (Hoeksema et al., 2016). The Agaricia genus has a wide morphological plasticity, including encrusting sheets, thick leaves, and flat plates, which allows them to attach to a wide range of substratum, including sponges (García-Hernández et al., 2017). However, the plate-like morphology of Agaricia makes them particularly susceptible to sedimentation, as was noticed on several colonies on the 45 m surveys (NMH per. obs.).
The Madracis genus is also moderately abundant across both depths, though identification to species was difficult for most individuals in the mesophotic surveys. Both the Agaricia and Madracis genera are thought to be primarily brooders. The release of competent planula larvae throughout the year gives these corals a higher reproductive potential in more hostile habitats such as sediment laden insular slopes (Bongaerts et al., 2017; Prasetia et al., 2017). However, their re-seeding capabilities are limited since brooded larvae typically settle within 50–100 m of the adult colony, making both horizontal and vertical dispersion more difficult (Bongaerts et al., 2010).
The altiphotic and mesophotic surveys showed nine species with distributional overlaps; the NMDS analysis showed clear divisions between altiphotic and mesophotic surveys (Figure 5). Compared to the 20 m surveys, most of the 45 m surveys had low scleractinian coral abundance, possibly due to the geomorphological constraints. The extent to which the shared species are interconnected is dependent upon vertical connectivity patterns and local adaptation of all components of the coral holobiont. For example, six of the nine shared corals are brooders and would be expected to have limited vertical dispersal, however caution should be used in asserting such claims without the inclusion of genetic connectivity estimates, which often are species-specific (Foster et al., 2012; Hammerman et al., 2017; Rippe et al., 2017).
Several threatened coral species were observed on these surveys, these included O. franksi, O. faveolata, O. annularis, M. cavernosa, Acropora cervicornis, and Mycetophyllia ferox. The Orbicella genus was particularly abundant in the 20 m surveys, which is supported by previous studies in the Caribbean (Carpenter et al., 2008; García-Sais et al., 2014, 2016, 2017). The presence of many rare and threatened species makes this area particularly valuable for coral conservation and management (García-Sais et al., 2017). Conservation is especially important in Guánica, given regions to the east are affected by local dredging and continual watershed activities that have significantly smothered shelf edge reefs in both terrigenous and re-suspended sediments (Appeldoorn et al., 2016a). The prevailing northeast trade winds create a westward driven current, therefore sediment and watershed activities to the east of Guánica have the potential to negatively affect water clarity and quality (Capella and Laboy, 2003; Sherman et al., 2013b). Coupled with recent reports of organic and metal contamination in Guánica Bay, only three km north of the Guánica shelf edge, there exist many land-based threats (e.g., contamination, nutrient input, sedimentation, diseases, trash, plastics) that are negatively contributing to the health of Guánica coral ecosystems (Pait et al., 2008, 2009; Sherman et al., 2013b; Whitall et al., 2013b, 2014). The cumulative effect could hinder coral recovery after bleaching and disease events, which given anthropogenic perturbations, the periodicity of such events are expected to strengthen and increase with time (Hughes et al., 2018; Figure 2H).
Sponge Assemblage
Comparison of overall species richness along Guánica's altiphotic and mesophotic reefs remained relatively similar across both depths, with 60 species at 20 m, and 54 species at 45 m, accounting for 63.0% species overlap (Figure 8; Table 5). The total number of species (71) found across Guánica's altiphotic and mesophotic reefs reflects the general trend of sponge diversity within other regions in the Caribbean (e.g., van Soest, 1978; Hajdu and Rützler, 1998; Alcolado, 2002; Diaz and Rützler, 2009; Thacker et al., 2010; Valderrama and Zea, 2013; Villamizar et al., 2013; Loh and Pawlik, 2014; Pérez et al., 2017; Cóndor-Luján et al., 2018). However, additional detailed surveys and exploratory dives targeting specific groups, such as calcareans (Fontana et al., 2018; JEGH unpubl. data) and homoscleromorphs (Ruiz et al., 2017) are necessary, and will surely yield new species, especially those inhabiting cryptic and sciophilous environments (Rützler et al., 2014; Ribeiro et al., 2016). Within our surveys, Agelas spp., A. compressa, Iotrochota birotulata, N. erecta, and X. muta were the most abundant sponges and major component of the benthic substratum across both depths.
In contrast to species richness, our data shows an increase of percent sponge cover between altiphotic to mesophotic depths, 9.0% (±1.04 SE) and 14.0% (±1.96 SE), respectively (Figure 8). These data reflect similar results to what previous studies have reported along the southwest and west coasts of Puerto Rico (Armstrong et al., 2009; García-Sais, 2010; Rivero-Calle, 2010; Sherman et al., 2010; Appeldoorn et al., 2016a). Interestingly, sites surveyed to the east of the main island of Puerto Rico (i.e., Culebra, Fajardo, Vieques and the US Virgin Islands) show extremely low percent sponge coverage (1.0–4.0%) which can be attributed to the dominance of the scleractinian corals O. annularis (16.0%; Smith et al., 2010) and O. franksi (38.0%; García-Sais et al., 2016), leaving little substrate for other benthic invertebrates to colonize. Comparing our results to what has been reported from the La Parguera Natural Reserve shelf edge. Armstrong et al. (2009) reported an average sponge cover of 13.0% at 25–35 m, while Appeldoorn et al. (2016a) reported sponge cover from two sites, Hole-in-the-Wall (17% and 16% from 50 m and 70 m, respectively), and El Hoyo (15% and 18% from 50 m and 70 m, respectively). Isolated and offshore sites to the west of Puerto Rico like Abrir La Sierra (García-Sais et al., 2010b), Isla Desecheo (García-Sais, 2010), Bajo de Sico (García-Sais et al., 2007), and Isla Mona (García-Sais et al., 2010a) have also reported high percent sponge cover at depths surveyed from 30–60 m.
Within Guánica's coral reef ecosystems, our total sponge cover data from 20 m (9.0% ± 1.04 SE) and 45 m (14.0% ± 1.96 SE) resemble those made by previous studies. García-Sais et al. (2014, 2015, 2017) reported an average sponge cover of 7.0% at 20 m from the Guánica insular shelf edge. Rivero-Calle (2010) analyzed phototransect surveys and compared benthic cover from two separate sampling years within Guánica's shelf edge. Rivero-Calle (2010) results show an apparent decrease of sponge cover across time and depth, with sponge cover at 30–40 m in 2004 (15.0%) decreasing to 10.0% in 2008, while sponge cover at 40–50 m in 2004 (11.0%) decreased to 7.7% in 2008, with similar decreases in sponge cover across 50–100 m depth intervals. Armstrong et al. (2009) also analyzed phototransects collected in 2004 across two depth stratifications, reporting overall similar results, with 25.0% cover at 25–35 m, and 38.0% cover at 36–50 m. Sponge cover, and to some extend diversity and abundance (154 unidentified specimens at 20 m, compared to 981 unidentified specimens at 45 m), seems to follow a similar trend at specific locations within the Caribbean, increasing with depth (García-Sais, 2010; Lesser and Slattery, 2013). However, care should be taken not generalize this trend until more studies (with a standardized method) take place across across multiple sites in the Caribbean (see Scott and Pawlik, 2018).
The incidence of unidentified sponge disease and bleaching affecting several species (A. conifera, A. sceptrum, A. compressa, C. vaginalis, S. zeai, V. gigantea, and X. muta) was opportunistically observed across both depths (i.e., Rützler, 1988; Olson et al., 2006, 2014; Angermeier et al., 2011, 2012; Deignan and Pawlik, 2016). However, since these observations occurred outside of our phototransect surveys, they were not considered for quantitative analysis. A potential disease of great concern (most likely orange band disease) was observed affecting the giant barrel sponge (X. muta) at both depths (Figure 2G) (JEGH pers. obs.), a species which acts as a bioengineer and provides habitat and structure for an array or organisms (McMurray et al., 2008; Hammerman and García-Hernández, 2017; Skinner, 2018; Swierts et al., 2018). Monitoring, recording and reporting sponge disease/bleaching outbreaks, subsequent die-offs and/or recovery events of large and morphologically diverse species (and their associates) is crucial to assess the overall health of the ecosystem in an ever-changing environment (Wulff, 2013; Deignan et al., 2018; Pita et al., 2018). For example, at Bajo de Sico seamount, an important Nassau and Black grouper aggregation site in Puerto Rico (Tuohy et al., 2015; Sanchez et al., 2017), occurrence of disease and bleaching was observed affecting several large specimens of X. muta at depths between 35 and 50 m (JEGH and Tuohy pers. Obs.). In one occasion, the complete disintegration of a large specimen of X. muta occurred 3–5 days after initial monitoring (Cowart et al., 2006; McMurray et al., 2011).
Duffy et al. (2013) highlighted a devastating example of how the disappearance of important host sponge species from reefs in Belize and Jamaica served as the primary cause for the dramatic decline of two species of eusocial shrimps. Large sponges may also be serving as zoogeographical “stepping-stones” as suggested by Collette and Rützler (1977), as well as recruitment habitat for several species of fish and benthic invertebrates (Tyler and Böhlke, 1972; Colin and Clavijo, 1988; Rocha et al., 2000; Rocha, 2003; García-Sais et al., 2007, 2010b). Future benthic surveys of Puerto Rican altiphotic and mesophotic coral reefs should include sponges in their ecology assessments, especially the most common and large species (Wulff, 2001; Bell et al., 2017). These large sponges play a key role in the overall health of the coral reef ecosystem by providing ecological services (Diaz and Rützler, 2001; de Goeij et al., 2013), and in some instances, contribute to the preservation of associated fauna biodiversity (Thomas and Klebba, 2006; Hultgren et al., 2010; Padua et al., 2013; Rix et al., 2018). A collapse of large species of sponges in Guánica and nearby reefs, which provide structure and habitat, may be devastating to some species of fish, especially at mesophotic depths (Figure 2F).
Unconsolidated sediments (Figure 7) accounted for a large portion of percent cover and influence both sponge and hard coral cover (Lesser and Slattery, 2011; Slattery and Lesser, 2014; Appeldoorn et al., 2016a), by limiting availability of substrate for larvae to settle upon (Duckworth, 2016). Algal cover of mesophotic sites in the adjacent La Parguera (50–70 m depth) averaged 52% of the habitat followed by sponges and corals, highlighting the importance of these taxa in shaping the communities at those depths (Appeldoorn et al., 2016b). This contrasts with what we observed, where algae benthic cover at 20 m (19.0 ± 2.20 SE) was greater than that at 45 m (7.6 ± 1.92 SE) (Figure 7), thus making sponges the most abundant, dominant, and important taxa at upper mesophotic reefs in Guánica. Additional attention should be given to any local increase of biological threats to these deeper reefs, such as cyanobacterial mats, known to disrupt benthic community assemblages (de Bakker et al., 2017), opportunistic benthic algae blooms (Langston and Spalding, 2017; Sherman and Tuohy pers. obs.), and the aggressive overgrowing colonial ascidian (Trididemnum solidum) known to overgrow corals (e.g., Rodríguez-Martínez et al., 2012; JEGH pers. obs.) and sponges (Table 4; JEGH pers. obs.).
Conclusion
Our dataset increases the knowledge of southwest Puerto Rico's altiphotic and mesophotic fish, coral, and sponge assemblages. We highlight the need to continue monitoring this dynamic ecosystem and emphasize that it is crucial to perform long-term time series assessments of the general area to fully assess any fluctuations in community structure (i.e., abundance, dominant species shift, disease, bleaching) (Wulff, 2001, 2006; Easson et al., 2015) and preservation of the overall marine biodiversity (Miloslavich et al., 2010; Jenkins and van Houtan, 2016; Hoeksema et al., 2017). This study demonstrates that altiphotic and mesophotic reefs across the Guánica continental shelf edge support different assemblages of corals, sponges and fish. These results provide preliminary data for the region supporting the arising hypothesis that mesophotic coral reefs should not be seen as refuge for shallower reefs, instead, they are actually different and unique ecosystems (i.e., Semmler et al., 2017; Rocha et al., 2018). Of the species which were present across both depths, only the inclusion of genetics can decisively determine gene flow and connectivity. Given the prevalence of anthropogenic vectors both directly adjacent to Guánica (e.g., Rio Loco System and Guánica Bay) and further east in Ponce (e.g., Ponce mega port and watershed activities), and the presence of a wide array of unique and ecologically important species, we propose increased monitoring from local, state, and federal agencies to protect and include mesophotic coral reef ecosystems within the Guánica Biosphere Reserve.
Author Contributions
JG-H, PS, and NH contributed equally to the manuscript. JG-H, PS, NH, and NS conceived, designed, and drafted the article. JG-H served as the sponge specialist. PS served as the fish specialist. NH served as the coral specialist. NS served as the PI of the first three authors. All authors developed, implemented, and analyzed data, and contributed to the theory and interpretation of the data. All authors critically revised and approved the final version of the article.
Conflict of Interest Statement
The authors declare that the research was conducted in the absence of any commercial or financial relationships that could be construed as a potential conflict of interest.
Acknowledgments
JG-H thanks Marta Wayne, Stuart McDaniel, Gustav Paulay, Amanda Bemis, John Slapcinsky, and Robert Lasley for hosting him at the University of Florida and at the Florida Museum of Natural History following the aftermath of Hurricane Maria (January–May, 2018), allowing him to complete this manuscript. We thank Milton Carlo and Orlando Espinosa, who were an integral component of the diving team and provided extensive logistical support throughout field operations. JG-H would like to thank The Explorers Club Exploration Fund–Mamont Scholars Program for providing funds to conduct field work, as well as Idea Wild for providing two Light & Motion SOLA 1200 lumen lights. Additionally, JG-H thanks Professor Jorge Corredor for supporting The Explorers Club student member application to join this prestigious organization. Lastly, JG-H thanks Sea Grant Puerto Rico and Dr. Chaparro for providing funds to purchase a DNA extraction kit. Authors thank the Department of Marine Sciences at UPRM for their access to medium size boats, as well as boat captains Anibal Santiago and Eduardo Mercado. The authors would like to dedicate this manuscript to Angel “negrito” Camacho for his service and commitment to graduate students at Isla Magueyes Research Station. Authors also thank the reviewers, who through their suggestions and comments, helped to improve our manuscript. Sponge collecting permit was provided to JG-H by the Department of Natural and Environmental Resources of Puerto Rico (#O-VS-PVS15-MA-00021-22122015).
Supplementary Material
The Supplementary Material for this article can be found online at: https://www.frontiersin.org/articles/10.3389/fmars.2018.00303/full#supplementary-material
References
Alcolado, P. M. (2002). Catalogo de las esponjas de Cuba. Avicennia: Revis. de Ecol. Oceano. Biodiv. Trop. 15, 53–72. Available online at: https://biodiversitylibrary.org/page/44343440
Angermeier, H., Glöckner, V., Pawlik, J. R., Lindquist, N. L., and Hentschel, U. (2012). Sponge white patch disease affecting the Caribbean sponge Amphimedon compressa. Dis. Aquat. Org. 99, 95–102. doi: 10.3354/dao02460
Angermeier, H., Kamke, J., Abdelmohsen, U. R., Krohne, G., Pawlik, J. R., Lindquist, N. L., et al. (2011). The pathology of sponge orange band disease affecting the Caribbean barrel sponge Xestospongia muta. FEMS. Microbiol. Ecol. 75, 218–230. doi: 10.1111/j.1574-6941.2010.01001.x
Appeldoorn, R., Ballantine, D., Bejarano, I., Carlo, M., Nemeth, M., Otero, E., et al. (2016b). Mesophotic coral ecosystems under anthropogenic stress: a case study at Ponce, Puerto Rico. Coral Reefs. 35, 63–75. doi: 10.1007/s00338-015-1360-5
Appeldoorn, R., Ballantine, D., Bejarano, I., Ruiz, H., Schizas, N. V., Schmidt, W., et al. (2016a). “Mesophotic coral ecosystems examined: La Parguera, Puerto Rico, USA,” in Mesophotic Coral Ecosystems:A lifeboat for coral reefs? The United Nations Environment Programme and GRID-Arendal, Nairobi and Arendal, Vol. 5, eds E. K. Baker, K. A. Puglise, and P. T. Harris 45–49. Available online at: www.unep.org,www.grida.no
Appeldoorn, R. S., Alfaro, M., Ballantine, D. L., Bejarano, I., Ruiz, H. J., Schizas, N. V., et al. (2018). “Puerto Rico,” in Mesophotic Coral Ecosystems of the World, eds Y. Loya, K. A. Puglise, and T. C. L. Bridge (New York, NY: Springer). Available online at: https://www.springer.com/us/book/9783319927343
Armstrong, R. A., and Singh, H. (2012). “Mesophotic coral reefs of the Puerto Rico shelf,” in Seafloor Geomorphology as Benthic Habitat: GeoHab Atlas of Seafloor Geomorphic Features and Benthic Habitats. eds P. Harris, and E. Baker (London: Elsevier), 365–374.
Armstrong, R. A., Singh, H., and Kunz, C. (2011). “Large-scale mapping and characterization of deep reef habitats in the US Caribbean,” in Proceedings of the 63rd Gulf and Caribbean Fisheries Institute, Vol. (San Juan), 134–138.
Armstrong, R. A., Singh, H., Rivero, S., and Gilbes, F. (2009). “Monitoring coral reef in optically-deep waters,” in Proceedings of the 11th International Coral Reef Symposium (Ft. Lauderdale, FL), 593–597.
Armstrong, R. A., Singh, H., Torres, J., Nemeth, R., Can, A., Roman, C., et al. (2006). Characterizing the deep insular shelf coral reef habitat of the Hind Bank Marine Conservation District (US Virgin Islands) using the Seabed Autonomous Underwater Vehicle. Cont. Shelf Res. 26, 194–205. doi: 10.1016/j.csr.2005.10.004
Baker, E. K., Puglise, K. A., and Harris, P. T. (2016). Mesophotic Coral Ecosystems - A Lifeboat for Coral Reefs? The United Nations Environment Program and GRID-Arendal, Nairobi and Arendal, 98.
Baldwin, C. C., Tornabene, L., and Robertson, D. R. (2018). Below the Mesophotic. Sci. Reports, 8:4920. doi: 10.1038/s41598-018-23067-1
Ballantine, D. L., Ruiz, H. T., and Aponte, N. E. (2016). The mesophotic, coral reef-associated, marine algal flora of Puerto Rico, Caribbean Sea. Smithsonian Contrib. Bot. 105, 1–41. doi: 10.5479/si.1938-2812.105
Bauer, L. J., Edwards, K., and Caldow, C. (2013). “Baseline characterization of Fish communities and associated benthic habitats in the Guanica Bay Region of Southwest Puerto Rico,” in Baseline assessment of Guanica Bay, Puerto Rico in support of watershed restoration. NOAA Technical memorandum NOS NCCOS 176. Prepared by the NCCOS Center for Coastal Monitoring and Assessment Biogeography Branch, eds D. Whitall, L. J. Bauer, C. Sherman, K. Edwards, A. Mason, T. Pait, and C. Caldow (Silver Spring, MD), 5–62.
Bejarano, I., Appeldoorn, R. S., and Nemeth, M. (2014). Fishes associated with mesophotic coral ecosystems in La Parguera, Puerto Rico. Coral Reefs 33, 313–328. doi: 10.1007/s00338-014-1125-6
Bell, J. J., Biggerstaff, A., Bates, T., Bennett, H., Marlow, J., McGrath, E., et al. (2017). Sponge monitoring: moving beyond diversity and abundance measures. Ecol. Indic. 78, 470–488. doi: 10.1016/j.ecolind.2017.03.001
Bongaerts, P., Ridgway, T., Sampayo, E. M., and Hoegh-Guldberg, O. (2010). Assessing the ‘deep reef refugia' hypothesis: focus on Caribbean reefs. Coral Reefs 29, 309–327. doi: 10.1007/s00338-009-0581-x
Bongaerts, P., Riginos, C., Brunner, R., Englebert, N., Smith, S. R., and Hoegh-Guldberg, O. (2017). Deep reefs are not universal refuges: Reseeding potential varies among coral species. Mar. Ecol. 3:e1602373. doi: 10.1126/sciadv.1602373
Brazeau, D. A., Lesser, M. P., and Slattery, M. (2013). Genetic structure in the coral, Montastraea cavernosa: assessing genetic differentiation among and within mesophotic reefs. PLoS ONE 8:e65845. doi: 10.1371/journal.pone.0065845
Brokovich, E., Ayalon, I., Einbinder, S., Segev, N., Shaked, Y., Genin, A., et al. (2010). Grazing pressure on coral reefs decreases across a wide depth gradient in the Gulf of Aqaba, Red Sea. Mar. Ecol. Prog. Ser. 399, 69–80. doi: 10.3354/meps08354
Brokovich, E., Einbinder, S., Shashar, N., Kiflawi, M., and Kark, S. (2008). Descending to the twilight-zone: changes in coral reef fish assemblages along a depth gradient down to 65 m. Mar. Ecol. Prog. Ser. 371, 253–262. doi: 10.3354/meps07591
Capella, J., and Laboy, E. (2003). “Chapter 2: environmental settings,” in Jobos Bay Estuarine Profile, ed R. Field 14–39.
Carpenter, K. E., Abrar, M., Aeby, G., Aronson, R. B., Banks, S., Bruckner, A., et al. (2008). One-third of reef-building corals face elevated extinction risk from climate change and local impacts. Science 25, 560–563. doi: 10.1126/science.1159196
Colin, P. L., and Clavijo, I. E. (1988). Spawning activity of fishes producing pelagic eggs on a shelf edge coral reef, southwestern Puerto Rico. Bull. Marine Sci. 43, 249–279.
Collette, B. B., and Rützler, K. (1977). “Reef fishes over sponge bottoms off the mouth of the Amazon River,” in Third International Coral Reef Symposium (Miami, FL), 305–310.
Cóndor-Luján, B., Louzada, T., Hajdu, E., and Klautau, M. (2018). Morphological and molecular taxonomy of calcareous sponges (Porifera: Calcarea) from Curaçao, Caribbean Sea. Zool. J. Linn. Soc. 183, 459–525. doi: 10.1093/zoolinnean/zlx082
Cowart, J. D., Henkel, T. P., McMurray, S. E., and Pawlik, J. R. (2006). Sponge orange band (SOB): a photogenic-like condition of the giant barrel sponge, Xestospongia muta. Coral Reefs. 25:513. doi: 10.1007/s00338-006-0149-y
Cowen, R. K., and Sponaugle, S. (2009). Larval dispersal and marine population connectivity. Ann. Rev. Mar. Sci. 1, 443–466. doi: 10.1146/annurev.marine.010908.163757
de Bakker, D. M., van Duyl, F. C., Bak, R. P. M., Nugues, M. M., Nieuwland, G., and Meesters, E. H. (2017). 40 Years of benthic community change on the Caribbean reefs of Curaçao and Bonaire: the rise of slimy cyanobacterial mats. Coral Reefs. 36, 355–367. doi: 10.1007/s00338-016-1534-9
De Cáceres, M., and Legendre, P. (2009). Associations between species and groups of sites: indices and statistical inference. Ecology 90, 3566–3574. doi: 10.1890/08-1823.1
de Goeij, J. M., van Oevelen, D., Vermeij, M. J. A., Osinga, R., Middelburg, J. J., de Goeij, A. F. P. M., et al. (2013). Surviving in a marine desert: the sponge loop retains resources within coral reefs. Science 342, 108–110. doi: 10.1126/science.1241981
de Laubenfels, M. (1934). Reports on the collections obtained by the first Johnson-Smithsonian Deep-Sea Expedition to the Puerto Rican deep. New sponges from the Puerto Rican Deep. Smith. Misc. Collect. 91, 1–28.
Deignan, L. K., and Pawlik, J. R. (2016). “Demographics of the Caribbean brown tube sponge Agelas tubulata on Conch Reef, Florida Keys, and a description of Agelas Wasting Syndrome (AWS),” in Proceedings of the 13th International Coral Reef Symposium (Honolulu, HI), 72–84.
Deignan, L. K., Pawlik, J. R., and López-Legentil, S. (2018). Evidence for shifting genetic structure among Caribbean giant barrel sponges in the Florida Keys. Marine Biol. 165:106. doi: 10.1007/s00227-018-3355-6
Dennis, G. D., Hensley, D., Colin, P. L., and Kimmel, J. J. (2004). New records of marine fishes from the Puerto Rican plateau. Caribb. J. Sci. 40, 70–87. Available online at: http://repositorio.upr.edu:8080/jspui/bitstream/10586%20/351/1/New%20record%20of%20Marine%20Fishes%20.pdf
Diaz, M. C., and Rützler, K. (2001). Sponges: an essential component of Caribbean coral reefs. B. Mar. Sci. 69, 535–546. Available online at: https://repository.si.edu/bitstream/handle/10088/7877/iz_Diaz_Ruetzler_2001.pdf
Diaz, M. C., and Rützler, K. (2009). Biodiversity and abundance of sponges in caribbean mangrove: indicators of environmental quality. Smithsonian Contr. Mar. Sci. 38, 151–172. doi: 10.5479/si.01960768.38.151
Duckworth, A. R. (2016). Substrate type affects the abundance and size of a coral-reef sponge between depths. Mar. Freshw. Res. 67, 246–255. doi: 10.1071/MF14308
Duffy, J. E., Macdonald, K. S. III., Hultgren, K. M., Chak, T. C. S., and Rubenstein, D. R. (2013). Decline and local extinction of Caribbean eusocial shrimp. PLoS ONE 8:e54637. doi: 10.1371/journal.pone.0054637
Dziedzic, K., Elder, H., and Meyer, E. (2017). Heritable variation in bleaching responses and its functional genomic basis in reef-building corals (Orbicella faveolata). bioRxiv. doi: 10.1101/185595
Easson, C. G., Matterson, K. O., Freeman, C. J., Archer, S. K., and Thacker, R. W. (2015). Variation in species diversity and functional traits of sponge communities near human populations in Bocas del Toro, Panama. PeerJ 3:e1385. doi: 10.7717/peerj.1385
Fontana, T., Cóndor-LujÁn, B., Azevedo, F., Pérez, T., and Klautau, M. (2018). Diversity and distribution patterns of Calcareous sponges (subclass Calcinea) from Martinique. Zootaxa 4410, 331–369. doi: 10.11646/zootaxa.4410.2.5
Foster, N. L., Paris, C. B., Kool, J. T., Baums, I. B., Stevens, J. R., Sanchez, J. A., et al. (2012). Connectivity of Caribbean coral populations: complementary insights from empirical and modelled gene flow. Mol. Ecol. 21, 1143–1157. doi: 10.1111/j.1365-294X.2012.05455.x
Galindo-Estronza, A. M., Alfaro, M., and Schizas, N. V. (2016). Morphological and genetic species diversity in ostracods (Crustacea: Oligostraca) from Caribbean Reefs. Mar. Bio. 47, 37–53. doi: 10.1007/s12526-016-0525-y
García-Hernández, J. E., Van Moorsel, G. W. N. M., and Hoeksema, B. W. (2017). Lettuce corals overgrowing tube sponges at St. Eustatius, Dutch Caribbean. Mar. Bio. 47, 55–56. doi: 10.1007/s12526-016-0467-4
García-Sais, J. R. (2010). Reef habitats and associated sessile-benthic and fish assemblages across a euphotic–mesophotic depth gradient in Isla Desecheo, Puerto Rico. Coral Reefs 29, 277–288. doi: 10.1007/s00338-009-0582-9
García-Sais, J. R., Appeldoorn, R., Bruckner, A., Caldow, C., Christensen, J. D., Lilyestrom, C., et al. (2005). “The state of coral reef ecosystems of the commonwealth of Puerto Rico,” in The States: NOAA Technical Memorandum NOS NCCOS 11, ed J. Waddell (Silver Spring, MD: NOAA/NCCOS Center for Coastal Monitoring and Assessment's Biogeography Team), 91–134.
García-Sais, J. R., Castro, R., Sabater, J., and Carlo, M. (2007). Characterization of Benthic Habitats and Associated Reef Communities at Bajo de Sico Seamount. Final Report Submitted To Caribbean Fishery Management Council (CFMC/NOAA), Mona Passage.
García-Sais, J. R., Castro, R., Sabater-Clavell, J., Carlo, M., Esteves, R., and Williams, S. (2010a). Monitoring of coral reef communities from Natural Reserves in Puerto Rico: Isla Desecheo, Isla de Mona, Rincon, Guanica, Ponce, Caja de Muerto and Mayaguez. 2009-10. Final Report submitted to the Department of Natural Resources (DNER), U.S. Coral Reef National Monitoring Program, NOAA, 221.
García-Sais, J. R., Castro, R. L., and Sabater-Clavell, J. (2001). Coral Reef Communities From Natural Reserves in Puerto Rico: A Quantitative Baseline Assessment for Prospective Monitoring Programs, Vol. 1, Cordillera de Fajardo, Isla Caja de Muertos, Bosque Seco de Guanica, Bahia de Mayaguez. Final Report, U.S. Coral Reef Initiative (CRI-NOAA) and DNER.
García-Sais, J. R., Castro-Gomez, R. L., Sabater-Clavell, J., Esteves, R., Williams, S., and Carlo, M. (2010b). Mesophotic benthic habitats and associated marine communities at Abrir La Sierra, Puerto Rico. NOAA coral grant. 1–117.
García-Sais, J. R., Esteves, R., Williams, S., Sabater-Clavell, J., and Carlo, M. (2014). Monitoring of Coral Reef Communities From Natural Reserves in Puerto Rico: 2012-13. Final Report submitted to the Department of Natural and Environmental Resources (DNER), U.S. Coral Reef National Monitoring Program, NOAA.
García-Sais, J. R., Esteves, R., Williams, S., Sabater-Clavell, J., and Carlo, M. (2015). Monitoring of Coral Reef Communities From Natural Reserves in Puerto Rico: 2015. Final Report submitted to the Department of Natural and Environmental Resources (DNER), U.S. Coral Reef National Monitoring Program, NOAA.
García-Sais, J. R., Esteves, R., Williams, S., Sabater-Clavell, J., and Carlo, M. (2016). Monitoring of Coral Reef Communities From Natural Reserves in Puerto Rico: 2016. Final Report submitted to the Department of Natural and Environmental Resources (DNER), U.S. Coral Reef National Monitoring Program, NOAA.
García-Sais, J. R., Williams, S., Esteves, R., Sabater-Clavell, J., and Carlo, M. (2017). Monitoring of Coral Reef Communities From Natural Reserves in Puerto Rico: 2017. Final Report submitted to the Department of Natural and Environmental Resources (DNER), U.S. Coral Reef National Monitoring Program, NOAA.
Glasl, B., Bongaerts, P., Elisabeth, N. H., Hoegh-Guldberg, O., Herndl, G. J., and Frade, P. R. (2017). Microbiome variation in corals with distinct depth distribution ranges across a shallow-mesophotic gradient (15-85 m). Coral Reefs 36, 447–452 doi: 10.1007/s00338-016-1517-x
Glynn, P. W. (1996). Coral reef bleaching: facts, hypotheses and implications. Glob. Chang. Biol. 2, 495–509. doi: 10.1111/j.1365-2486.1996.tb00063.x
Gonzalez-Zapata, F. L., Bongaerts, P., Ramirez-Portilla, C., Adu-Oppong, B., Walljasper, G., Reyes, A., et al. (2018). Holobiont diversity in a reef-building coral over its entire depth range in the mesophotic zone. Front. Mar. Sci. 5:29. doi: 10.3389/fmars.2018.00029
Goodbody-Gringley, G., Marchini, C., Chequer, A. D., and Goffredo, S. (2015). Population structure of Montastraea cavernosa on Shallow versus Mesophotic reefs in Bermuda. PLoS ONE 10:e0142427. doi: 10.1371/journal.pone.0142427
Gress, E., Arroyo-Gerez, M. J., Wright, G., and Andradi-Brown, D. A. (2017). Mesophotic coral ecosystems inside and outside a Caribbean Marine Protected Area. bioRxiv [Preprint]. bioRxiv 241562. doi: 10.1101/241562
Hajdu, E., and Rützler, K. (1998). Sponges, genus Mycale (Poecilosclerida, Demospongiae, Porifera), from a Caribbean mangrove and comments on subgeneric classification. Proc. Biol. Soc. Wash. 111, 737–773.
Hammerman, N. M., and García-Hernández, J. E. (2017). The sponge Xestospongia muta offers shelter to the stony coral Madracis auretenra (Northwest Puerto Rico). Mar. Biodiv. 47, 57–58. doi: 10.1007/s12526-016-0574-2
Hammerman, N. M., Rivera-Vicens, R. E., Galaska, M. P., Weil, E., Appeldoorn, R. S., Alfaro, M., et al. (2017). Population connectivity of the plating coral Agaricia lamarcki from southwest Puerto Rico. Coral Reefs. 37, 183–191. doi: 10.1007/s00338-017-1646-x
Hinderstein, L. M., Marr, J. C. A., Martinez, F. A., Dowgiallo, M. J., Puglise, K. A., Pyle, R. L., et al. (2010). Theme section on “Mesophotic Coral Ecosystems: Characterization, Ecology, and Management”. Coral Reefs. 29, 247–251. doi: 10.1007/s00338-010-0614-5
Hoeksema, B. W., Bongaerts, P., and Baldwin, C. C. (2016). High coral cover at lower mesophotic depths: a dense Agaricia community at the leeward side of Curaçao, Dutch Caribbean. Mar. Bio. 47, 67–70. doi: 10.1007/s12526-015-0431-8
Hoeksema, B. W., Reimer, J. D., and Vonk, R. (2017). Editorial: biodiversity of Caribbean coral reefs (with a focus on the Dutch Caribbean). Marine Biodiv. 47, 1–10. doi: 10.1007/s12526-017-0641-3
Holstein, D. M., Smith, T. B., and Paris, C. B. (2016). Depth-independent reproduction in the reef coral Porites astreoides from shallow to mesophotic zones. PLoS ONE 11:e0146068 doi: 10.1371/journal.pone.0146068
Hubbard, D. K., Burke, R. B., Gill, I. P., Ramirez, W. R., and Sherman, C. E. (2008). “Coral-reef geology: Puerto Rico and the US Virgin Islands,” in Coral Reefs of the USA, eds B. M. Riegl and R. E. Dodge (Dania Beach, FL: Springer; Nova Southeastern University), 263–302.
Hughes, T. P., Anderson, K. D., Connolly, S. R., Heron, S. F., Kerry, J. T., Lough, J. M., et al. (2018). Spatial and temporal patterns of mass bleaching of corals in the Anthropocene. Science 359, 80–83. doi: 10.1126/science.aan8048
Hultgren, K. M., MacDonald, K. S. III., and Duffy, J. E. (2010). Sponge-dwelling snapping shrimps of Curaçao, with descriptions of three new species. Zootaxa 2372, 221–262. Available online at: http://www.mapress.com/j/zt/article/view/7773
Jenkins, C. N., and van Houtan, K. S. (2016). Global and regional priorities for marine biodiversity protection. Biol. Conserv. 204, 333–339. doi: 10.1016/j.biocon.2016.10.005
Kahng, S. E., Copus, J., and Wagner, D. (2014). Recent advances in the ecology of mesophotic coral ecosystems (MCEs). Curr. Opin. Env. Sust. 7, 72–81. doi: 10.1016/j.cosust.2013.11.019
Kahng, S. E., Copus, J. M., and Wagner, D. (2017). “Mesophotic coral ecosystems,” in Marine Animal Forests: The Ecology of Benthic Biodiversity Hotspots (Cham: Springer International Publishing), 185–206.
Kahng, S. E., Garcia-Sais, J. R., Spalding, H. L., Brokovich, E., Wagner, D., Weil, E., et al. (2010). Community ecology of mesophotic coral reef ecosystems. Coral Reefs 29, 255–275. doi: 10.1007/s00338-010-0593-6
Kohler, K. E., and Gill, S. M. (2006). Coral Point Count with Excel extensions (CPCe): A Visual Basic program for the determination of coral and substrate coverage using random point count methodology. Comput. Geosci. 32, 1259–1269. doi: 10.1016/j.cageo.2005.11.009
Langston, R. C., and Spalding, H. L. (2017). A survey of fishes associated with Hawaiian deep-water Halimeda kanaloana (Bryopsidales: Halimedacea) and Avrainvillea sp. (Bryopsidales: Udoteaceae) meadows. PeerJ 5:e3307. doi: 10.7717/peerj.3307
Laverick, J. H., Andradi-Brown, D. A., and Rogers, A. D. (2017). Using light-dependent scleractinia to define the upper boundary of mesophotic coral ecosystems on the reefs of Utila, Honduras. PLoS ONE 12:e0183075. doi: 10.1371/journal.pone.0183075
Lesser, M. P., and Slattery, M. (2011). Phase shift to algal dominated communities at mesophotic depths associated with lionfish (Pterois volitans) invasion on a Bahamian coral reef. Biol. Inv. 13, 1855–1868. doi: 10.1007/s10530-011-0005-z
Lesser, M. P., and Slattery, M. (2013). Ecology of Caribbean sponges: are top-down or bottom-up processes more important. PLoS ONE. 8:e79799. doi: 10.1371/journal.pone.0079799
Lesser, M. P., Slattery, M., and Leichter, J. J. (2009). Ecology of mesophotic coral reefs. J. Exp. Mar. Biol. Ecol. 375, 1–8. doi: 10.1016/j.jembe.2009.05.009
Lindfield, S. J., Harvey, E. S., Halford, A. R., and McIlwain, J. L. (2016). Mesophotic depths as refuge areas for fishery-targeted species on coral reefs. Coral Reefs 35, 125–137. doi: 10.1007/s00338-015-1386-8
Locker, S. D., Armstrong, R. A., Battista, T. A., Rooney, J. J., Sherman, C., and Zawada, D. G. (2010). Geomorphology of mesophotic coral ecosystems: current perspective on morphology, distribution, and mapping strategies. Coral Reefs 29, 329–345. doi: 10.1007/s00338-010-0613-6
Loh, T. L., and Pawlik, J. R. (2014). Chemical defenses and resource trade-offs structure sponge communities on Caribbean coral reefs. Proc. Natl. Acad. Sci. U.S.A. 111, 4151–4156. doi: 10.1073/pnas.1321626111
Loya, Y., Eyal, G., Treibitz, T., Lesser, M. P., and Appeldoorn, R. (2016). Theme section on mesophotic coral ecosystems: advances in knowledge and future perspective. Coral Reefs 35, 1–9. doi: 10.1007/s00338-016-1410-7
Lucas, M. Q., Stat, M., Smith, M. C., Weil, E., and Schizas, N. V. (2016). Symbiodinium (internal transcribed spacer 2) diversity in the coral host Agaricia lamarcki (Cnidaria: Scleractinia) between shallow and mesophotic reefs in the Northern Caribbean (20-70 m). Mar. Ecol. 37, 1079–1087. doi: 10.1111/maec.12367
Lumsden, S. E., Hourigan, T. F., Bruckner, A. W., and Dorr, G. (2007). The State of Deep Coral Ecosystems of the United States. U.S. Department of Commerce, NOAA Technical Memorandum CRCP.
MacDonald, C., Bridge, T. C. L., and Jones, G. P. (2016). Depth, bay position and habitat structure as determinants of coral reef fish distributions: are deep reefs a potential refuge? Mar. Ecol. Prog. Ser. 561, 217–231. doi: 10.3354/meps11953
McMurray, S. E., Blum, J. E., Leichter, J. J., and Pawlik, J. R. (2011). Bleaching of the giant barrel sponge Xestospongia muta in the Florida Keys. Limnol. Oceanogr. 56, 2243–2250. doi: 10.4319/lo.2011.56.6.2243
McMurray, S. E., Blum, J. E., and Pawlik, J. R. (2008). Redwood of the reef: growth and age of the giant barrel sponge Xestospongia muta in the Florida Keys. Mar. Biol. 155, 159–171 doi: 10.1007/s00227-008-1014-z
Miller, K., and Mundy, C. (2003). Rapid settlement in broadcast spawning corals: implications for larval dispersal. Coral Reefs. 22, 99–106. doi: 10.1007/s00338-003-0290-9
Miloslavich, P., Díaz, J. M., Klein, E., Alvarado, J. J., Díaz, C., Gobin, J., et al. (2010). Marine Biodiversity in the Caribbean: regional estimates and distribution patterns. PLoS ONE. 5:e11916. doi: 10.1371/journal.pone.0011916
Morelock, J., Winget, E., and Goenaga, C. (1994). Geological Maps of the Southwestern Puerto Rico Parguera to Guanica Insular Shelf. USGS Miscellaneous Map Series. Washington, DC: U.S. Geological survey.
Nelson, W. R., and Appeldoorn, R. S. (1985). Cruise Report: R/V Seward Johnson. A submersible Survey of the Continental Slope of Puerto Rico and the U.S. Virgin Islands October 1-23, 1985. NOAA/NMFS/SEFC Mississippi Laboratory and University of Puerto Rico Sea Grant Program.
Oksanen, J., Blanchet, F. G., Friendly, M., Kindt, R., Legendre, P., McGlinn, D., et al. (2017). Vegan: Community Ecology Package. R Package Version 2.4–4. Available online at: https://cran.r-project.org/web/packages/vegan/index.html
Olson, J. B., Gochfeld, D. J., and Slattery, M. (2006). Aplysina red band syndrome: a new threat to Caribbean sponges. Dis. Aquat. Org. 71, 163–168. doi: 10.3354/dao071163
Olson, J. B., Thacker, R. W., and Gochfeld, D. J. (2014). Molecular community profiling reveals impacts of time, space, and disease status on the bacterial community associated with the Caribbean sponge Aplysina cauliformis. FEMS. Microbiol. Ecol. 87, 268–279. doi: 10.1111/1574-6941.12222
Padua, A., Lanna, E., and Klautau, M. (2013). Macrofauna inhabiting the sponge Paraleucilla magna (Porifera: Calcarea) in Rio de Janeiro, Brazil. J. Mar. Biol. Assoc. UK. 93, 889–898. doi: 10.1017/S0025315412001804
Pait, A. S., Jeffrey, C. F. G., Caldow, C., Whitall, D. R., Hartwell, S. I., Mason, A. L., et al. (2009). Chemical Contaminants in the Coral Porites Astreoides From Southwest Puerto Rico. NOS NCCOS 91. Silver Spring, MD: NOAA/NOS for Coastal Monitoring and Assessment.
Pait, A. S., Whitall, D. R., Jeffrey, C. F. G., Caldow, C., Mason, A. L., Lauenstein, G. G., et al. (2008). Chemical contamination in southwest Puerto Rico: an assessment of organic contaminants in near shore sediments. Mar. Poll. Bull. 56, 580–606. doi: 10.1016/j.marpolbul.2007.11.007
Pearson, R., and Stevens, T. (2015). Distinct cross-shelf gradient in mesophotic reef fish assemblages in subtropical eastern Australia. Mar. Ecol. Prog. Ser. 532, 185–196. doi: 10.3354/meps11351
Pérez, T., Díaz, M. C., Ruiz, C., Cóndor-Luján, B., Klautau, M., Hajdu, E., et al. (2017). How a collaborative integrated taxonomic effort has trained new spongiologists and improved knowledge of Martinique Island (French Antilles, eastern Caribbean Sea) marine biodiversity. PLoS ONE 12:e0173859. doi: 10.1371/journal.pone.0173859
Pinheiro, H. T., Goodbody-Gringley, G., Jessup, M. E., Shepherd, B., Chequer, A. D., and Rocha, L. A. (2016). Upper and lower mesophotic coral reef fish communities evaluated by underwater visual censuses in two Caribbean locations. Coral Reefs. 35, 139–151. doi: 10.1007/s00338-015-1381-0
Pita, L., Rix, L., Slaby, B. M., Franke, A., and Hentschel, U. (2018). The sponge holobiont in a changing ocean: from microbes to ecosystems. Microbiome 6:46. doi: 10.1186/s40168-018-0428-1
Pittman, S. J., Hile, S. D., Jeffrey, C. F. G., Clark, R., Woody, K., Herlach, B. D., et al. (2010). Coral Reef Ecosystems of Reserva Natural La Parguera (Puerto Rico): Spatial and Temporal Patterns in Fish and Benthic Communities (2001-2007).. Silver Spring, MD: NOAA Technical Memorandum NOS NCCOS 107.
Prasetia, R., Sinniger, F., Hashizume, K., and Harii, S. (2017). Reproductive biology of the deep brooding coral Seriatopora hystrix: implications for shallow reef recovery. 12:e0177034. PLoS ONE. doi: 10.1371/journal.pone.0177034
Pyle, R. L., Boland, R., Bolick, H., Bowen, B. W., Bradley, C. J., Kane, C., et al. (2016). A comprehensive investigation of mesophotic coral ecosystems in the Hawaiian Archipelago. PeerJ. 4:e2475. doi: 10.7717/peerj.2475
R Core Team (2016). R: A Language and Environment for Statistical Computing. Vienna: R Foundation for Statistical Computing. Available online at: https://www.R-project.org/
Randall, J. E. (1967). Food habits of reef fishes of the West Indies. Stud. Trop. Oceanogr. 5, 665–847.
Ribeiro, B., Padua, A., Paiva, P. C., and Klautau, M. (2016). Exploitation of micro refuges and epibiosis: survival strategies of a calcareous sponge. J. Mar. Biol. Assoc. U. K. 1–9. doi: 10.1017/S002531541600151X
Rippe, J. P., Matz, M. V., Green, E. A., Medina, M., Khawaja, N. Z., Pongwarin, T., et al. (2017). Population structure and connectivity of the mountainous star coral, Orbicella faveolata, throughout the wider Caribbean region. Ecol. Evol. 1-13. doi: 10.1002/ece3.3448
Rivero-Calle, S. (2010). Ecological Aspects of Sponges in Mesophotic Coral Ecosystems. M.S. Thesis, University of Puerto Rico, Mayagüez, PR.
Rix, L., de Goeij, J. M., van Oevelen, D., Struck, U., Al-Horani, F. A., Wild, C., et al. (2018). Reef sponges facilitate the transfer of coral-derived organic matter to their associated fauna via the sponge loop. Mar. Ecol. Prog. Ser. 589, 85–96. doi: 10.3354/meps12443
Rocha, L. A. (2003). Patterns of distribution and processes of speciation in Brazilian reef fishes. J. Biogeogr. 30, 1161–1171. doi: 10.1046/j.1365-2699.2003.00900.x
Rocha, L. A., Pinheiro, H. T., Shepherd, B., Papastamatiou, Y. P., Luiz, O. J., Pyle, R. L., et al. (2018). Mesophotic coral ecosystems are threatened and ecologically distinct from shallow water reefs. Science 361, 281–284. doi: 10.1126/science.aaq1614
Rocha, L. A., Rosa, I. L., and Feitoza, B. M. (2000). Sponge dwelling fishes of northeastern Brazil. Environ. Biol. Fishes. 59, 453–458. doi: 10.1023/A:1026584708092
Rodríguez-Martínez, R. E., Jordán-Garza, A. G., Baker, D. M., and Jordán-Dahlgren, E. (2012). Competitive interactions between corals and Trididemnum solidum on Mexican Caribbean reefs. Coral Reefs. 31, 571–577. doi: 10.1007/s00338-011-0871-y
Rosa, M. R., Alves, A. C., Medeiros, D. V., Coni, E. O. C., Ferreira, C. M., Ferreira, B. P., et al. (2016). Mesophotic reef fish assemblages of the remote St. Peter and St. Paul's Archipelago, Mid-Atlantic Ridge, Brazil. Coral Reefs 35, 113–123. doi: 10.1007/s00338-015-1368-x
Ruiz, C., Muricy, G., Lage, A., Domingos, C., Chenesseau, S., and Pérez, T. (2017). Descriptions of new sponge species and genus, including aspiculate Plakinidae, overturn the Homoscleromorpha classification. Zool. J. Linnean Soc. 179, 707–724. doi: 10.1111/zoj.12480
Rützler, K. (1988). Mangrove sponge disease induced by cyanobacterial symbionts: failure of a primitive immune system? Dis. Aquat. Org. 5, 143–149. doi: 10.3354/dao005143
Rützler, K. (2004). Sponges on coral reefs: a community shaped by competitive cooperation. Boll. Mus. Ist. Biol. Univ. Genova. 68, 85–148. Available online at: https://repository.si.edu/handle/10088/163
Rützler, K., Piantoni, C., van Soest, R. W. M., and Diaz, M. C. (2014). Diversity of sponges (Porifera) from cryptic habitats on the Belize barrier reef near Carrie Bow Cay. Zootaxa 3805, 1–129. doi: 10.11646/zootaxa.3805.1.1
Sanchez, P. J., Appeldoorn, R. S., Schärer-Umpierre, M. T., and Locascio, J. V. (2017). Patterns of courtship acoustics and geophysical features at spawning sites of black grouper (Mycteroperca bonaci). Fish. Bull. 115, 186–195. doi: 10.7755/FB.115.2.5
Scott, A. R., and Pawlik, J. R. (2018). A review of the sponge increase hypothesis for Caribbean mesophotic reefs. Marine Biodiv. 1–10. doi: 10.1007/s12526-018-0904-7
Semmler, R. F., Hoot, W. C., and Reaka, M. L. (2017). Are mesophotic coral ecosystems distinct communities and can they serve as refugia for shallow reefs? Coral Reefs. 36, 433–444. doi: 10.1007/s00338-016-1530-0
Serrano, X. M., Baums, I. B., Smith, T. B., Jones, R. J., Shearer, T. L., and Baker, A. C. (2016). Long distance dispersal and vertical gene flow in the Caribbean brooding coral Porites astreoides. Sci. Rep. 6:21619. doi: 10.1038/srep21619
Sherman, C., Appeldoorn, R., Ballantine, D., Bejarano, I., Carlo, M., Kesling, D., et al. (2013a). “Exploring the mesophotic zone: diving operations and scientific highlights of three research cruises across Puerto Rico and US Virgin Islands,” in Proceedings of the 2013 AAUS/ESDP Curaçao Joint International Scientific Diving Symposium, October 24–27, 2013, eds M. A. Lang and M. D. J. Sayer (Dauphin Island, AL: American Academy of Underwater Sciences), 297–312.
Sherman, C., Hernandez, R., Hutchinson, Y., and Whitall, D. (2013b). “Terrigenous sedimentation patterns at reefs adjacent to the Guánica Bay Watershed,” in Baseline Assessment of Guánica Bay, Puerto Rico in Support of Watershed Restoration. Prepared by the NCCOS Center for Coastal Monitoring and Assessment Biogeography Branch, eds D. Whitall, L.J. Bauer, C. Sherman, K. Edwards, A. Mason, T. Pait and C. Caldow (Silver Spring, MD), 103–112.
Sherman, C., Nemeth, M., Ruíz, H., Bejarano, I., Appeldoorn, R., Pagán, F., et al. (2010). Geomorphology and benthic cover of mesophotic coral ecosystems of the upper insular slope of southwest Puerto Rico. Coral Reefs. 29, 347–360. doi: 10.1007/s00338-010-0607-4
Sherman, C., Schmidt, W., Appeldoorn, R., Hutchinson, Y., Ruiz, H., Nemeth, M., et al. (2016). Sediment dynamics and their potential influence on insular-slope mesophotic coral ecosystems. Cont. Shelf Res. 129, 1–9. doi: 10.1016/j.csr.2016.09.012
Skinner, L. F. (2018). Record of Tubastraea coccinea on Xestospongia barrel sponge: a new threat to Caribbean and Gulf of Mexico reefs? Coral Reefs. 37, 809. doi: 10.1007/s00338-018-1706-x
Slattery, M., Gochfeld, D. J., Diaz, M. C., Thacker, R. W., and Lesser, M. P. (2016). Variability in chemical defense across a shallow to mesophotic depth gradient in the Caribbean sponge Plakortis angulospiculatus. Coral Reefs. 35, 11–22. doi: 10.1007/s00338-015-1324-9
Slattery, M., Gochfeld, D. J., Easson, C. G., and O'Donahue, L. R. K. (2013). Facilitation of coral reef biodiversity and health by cave sponge communities. Mar. Ecol. Prog. Ser. 476, 71–86. doi: 10.3354/meps10139
Slattery, M., and Lesser, M. P. (2012). “Mesophotic coral reefs: a global model of community structure and function,” in Proceedings of the 12th International Coral Reef Symposium, (Cairns, QLD), 9-13.
Slattery, M., and Lesser, M. P. (2014). Allelopathy in the tropical alga Lobophora variegate (Phaeophyceae): mechanistic basis for a phase shift on mesophotic coral reefs? J. Phycol. 50, 493–505. doi: 10.1111/jpy.12160
Slattery, M., Lesser, M. P., Brazeau, D., Stokes, M. D., and Leichter, J. J. (2011). Connectivity and stability of mesophotic reefs. J. Exp. Mar. Biol. Ecol. 408, 32–41. doi: 10.1016/j.jembe.2011.07.024
Smith, T. B., Blondeau, J., Nemeth, R. S., Pittman, S. J., Calnan, J. M., Kadison, E., et al. (2010). Benthic structure and cryptic mortality in a Caribbean mesophotic coral reef bank system, the Hind Bank Marine Conservation District, U.S. Virgin Islands. Coral Reefs. 29, 289–308. doi: 10.1007/s00338-009-0575-8
Smith, T. B., Gyory, J., Brandt, M. E., Miller, W. J., Jossart, J., and Nemeth, R. S. (2016). Caribbean mesophotic coral ecosystems are unlikely climate change refugia. Global Change Biol. 22, 2756–2765. doi: 10.1111/gcb.13175
Starck, W. A. II., and Colin, P. L. (1978). Gramma linki: a new species of grammid fish from the tropical western Atlantic. Bull. Mar. Sci. 28, 146–152.
Swierts, T., de Voogd, N. J., and Huang, Y. M. (2018). The giant barrel sponge facilitates the recovery of coral fragments after a tropical storm in Taiwan. Coral Reefs. 37, 675–675. doi: 10.1007/s00338-018-1691-0
Thacker, R. W., Díaz, M. C., de Voogd, N. J., van Soest, R. W. M., Freeman, C. J., Mobley, A. S., et al. (2010). Preliminary assessment of sponge biodiversity on Saba Bank, Netherlands Antilles. PLoS ONE 5:e9622. doi: 10.1371/journal.pone.0009622
Thomas, J. D., and Klebba, K. N. (2006). Studies of commensal Leucothoid amphipods: two new sponge-inhabiting species from south Florida and the Western Caribbean. J. Crustacean Biol. 26, 13–22. doi: 10.1651/C-2624.1
Todd, P. A. (2008). Morphological plasticity in scleractinian corals. Biol. Rev. 83, 315–337. doi: 10.1111/j.1469-185X.2008.00045.x
Torres, J. L. (2001). Impacts of sedimentation on the growth rates of Montastraea annularis in southwest Puerto Rico. Bull. Mar. Sci. 69, 631–637. Available online at: http://www.ingentaconnect.com/content/umrsmas/bullmar/2001/00000069/00000002/art00034?crawler=true
Tuohy, E., Nemeth, M. I., Bejarano, I., Schärer, M. T., and Appeldoorn, R. S. (2015). In situ tagging of Nassau Grouper Epinephelus striatus using closed-circuit rebreathers at a spawning aggregation in Puerto Rico. Mar. Technol. Soc. J. 49, 115–123. doi: 10.4031/MTSJ.49.1.9
Tyler, J. C., and Böhlke, J. E. (1972). Records of sponge-dwelling fishes, primarily of the Caribbean. Bull. Mar. Sci. 22, 601–642.
Valderrama, D., and Zea, S. (2013). Annotated checklist of sponges (Porifera) from the southernmost Caribbean reefs (North-West Gulf of Uraba), with description of new records for the Colombian Caribbean. Rev. Acad. Colomb. Cienc. 37, 353–378. Available online at: http://www.scielo.org.co/pdf/racefn/v37n144/v37n144a07.pdf
van der Loos, L. M., Prud'homme van Reine, W. F., Stokvis, F. R., Speksnijder, A. G. C. L., and Hoeksema, B. W. (2017). Beta diversity of macroalgal communities around St. Eustatius, Dutch Caribbean. Marine Biodiv. 47, 123–138. doi: 10.1007/s12526-016-0608-9
van Soest, R. W. M. (1978). “Marine sponges from Curaçao and other Caribbean localities Part I. Keratosa,” in Studies on the Fauna of Curaçao and other Caribbean islands, Amsterdam: Foundation for Scientific Research in Surinam and the Netherlands Antilles, eds P. Wagenaar Hummelinck and L. J. Van der Steen (Utrecht), 1–94.
Vaz, A. C., Paris, C. B., Olascoaga, M. J., Kourafalou, V. H., Kang, H., and Reed, J. K. (2016). The perfect storm: match-mismatch of bio-physical event drives larval reef fish connectivity between Pulley Ridge mesophotic reef and the Florida Keys. Continental Shelf Research 125, 136–146. doi: 10.1016/j.csr.2016.06.012
Veglia, J. A., Hammerman, N. M., Rosaly-Rivera, C. R., Lucas, M. Q., Galindo-Estronza, G., Corgosinho, P. H., et al. (2018). Characterizing population structure of coral-associated fauna from mesophotic and shallow habitats in the Caribbean. J. Marine Biol. Assoc. U.K. 1–11. doi: 10.1017/S0025315418000413
Vicente, V. P. (1990). Response of sponges with autotrophic endosymbionts during the coral-bleaching episode in Puerto Rico. Coral Reefs. 8, 199–202. doi: 10.1007/BF00265011
Villamizar, E., Diaz, M. C., Rützler, K., and De Nobrega, R. (2013). Biodiversity, ecological structure, and change in the sponge community of different geomorphological zones of the Barrier Fore-Reef at Carrie Bow Cay, Belize. Mar. Ecol. 35, 1–11. doi: 10.1111/maec.12099
Wegley, L., Edwards, R., Rodriguez-Briot, B., Liu, H., and Rohwer, F. (2007). Metagenomic analysis of the microbial community associated with the coral Porites astreoides. Environ. Microbiol. 11, 2707–2719. doi: 10.1111/j.1462-2920.2007.01383.x
Weil, E. (2005). “Puerto Rico,” in Caribbean Marine Biodiversity: The Known and the Unknown, eds P. Miloslavich and E. Klein 1–25.
Weinstein, D. K., Sharifi, A., Klaus, J. S., Smith, T. B., Giri, S. J., and Helmle, K. P. (2016). Coral growth, bioerosion, and secondary accretion of living orbicellid corals from mesophotic reefs in the US Virgin Islands. Mar. Ecol. Prog. Ser. 559, 45–63. doi: 10.3354/meps11883
Whitall, D., Bauer, L. J., Sherman, C., Edwards, K., Mason, A., Pait, T., et al. (2013a). Baseline Assessment of Guanica Bay, Puerto Rico in Support of Watershed Restoration. NOAA Technical Memorandum NOS NCCOS 176. Prepared by the NCCOS Center for Coastal Monitoring and assessment biogeography branch. Silver Spring, MD, 169.
Whitall, D., Mason, A., Brune, L., Pait, A., Fulton, M., Wirth, E., et al. (2013b). “Contaminants in surficial sediments and coral tissues of Guánica Bay,” in Baseline assessment of Guanica Bay, Puerto Rico in support of Watershed Restoration. NOAA Technical Memorandum NOS NCCOS 176, eds Whitall, D., Bauer, L. J., Sherman, C., Edwards, K., Mason, A., Pait, T., Caldow, C. “Silver Spring, MD: Prepared by the NCCOS Center for Coastal Monitoring and assessment biogeography branch), 63–10.
Whitall, D., Mason, A., Pait, A., Brune, L., Fulton, M., Wirth, E., et al. (2014). Organic and metal contamination in marine surface conch tissues of Guánica Bay, Puerto Rico. Mar. Pollut. Bull. 80, 293–301. doi: 10.1016/j.marpolbul.2013.12.053
White, K. N., Weinstein, D. K., Ohara, T., Denis, V., Montenegro, J., and Reimer, J. D. (2017). Shifting communities after typhoon damage on an upper mesophotic reef in Okinawa, Japan. PeerJ 5:e3573. doi: 10.7717/peerj.3573
Wilson, H. V. (1902). The sponges collected in Porto Rico in 1899 by the U.S. Fish Commission Steamer Fish Hawk. Bull. US Fish Commission (Kessinger Publishing, LLC.), 375–411.
Wulff, J. (2012). “Ecological interactions and the distribution, abundance, and diversity of sponges,” in Advances in Sponge Science: Phylogeny, Systematics, Ecology, Advances in Marine Biology, Vol. 61, eds M. A. Becerro, M. J. Uriz, M. Maldonado, and X. Turon (London: Elsevier Ltd.), 273–344.
Wulff, J. (2013). Recovery of sponges after extreme mortality events: morphological and taxonomic patterns in regeneration versus recruitment. Integr. Comp. Biol. 53, 512–523. doi: 10.1093/icb/ict059
Wulff, J. L. (2001). Assessing and monitoring coral reef sponges: Why and how? Bull. Mar. Sci. 69, 831–846. Available online at: http://www.ingentaconnect.com/contentone/umrsmas/bullmar/2001/00000069/00000002/art00046?crawler=true
Wulff, J. L. (2006). Rapid diversity and abundance decline in a Caribbean coral reef sponge community. Biol. Conserv. 127, 167–176. doi: 10.1016/j.biocon.2005.08.007
Zea, S., Henkel, T. P., and Pawlik, J. R. (2014). The Sponge Guide: A Picture to Caribbean Sponges, 3rd Edn. Available online at: www.spongeguide.org (Accessed on 22 02, 2017).
Keywords: MCE, biodiversity, conservation, ecology, management, Caribbean, coral reefs
Citation: García-Hernández JE, Sanchez PJ, Hammerman NM and Schizas NV (2018) Fish, Coral, and Sponge Assemblages Associated With Altiphotic and Mesophotic Reefs Along the Guánica Biosphere Reserve Continental Shelf Edge, Southwest Puerto Rico. Front. Mar. Sci. 5:303. doi: 10.3389/fmars.2018.00303
Received: 27 April 2018; Accepted: 08 August 2018;
Published: 10 September 2018.
Edited by:
Yehuda Benayahu, Tel Aviv University, IsraelReviewed by:
Darren James Coker, King Abdullah University of Science and Technology, Saudi ArabiaJacob L. Johansen, New York University Abu Dhabi, United Arab Emirates
Copyright © 2018 García-Hernández, Sanchez, Hammerman and Schizas. This is an open-access article distributed under the terms of the Creative Commons Attribution License (CC BY). The use, distribution or reproduction in other forums is permitted, provided the original author(s) and the copyright owner(s) are credited and that the original publication in this journal is cited, in accordance with accepted academic practice. No use, distribution or reproduction is permitted which does not comply with these terms.
*Correspondence: Nikolaos V. Schizas, nschizas@gmail.com
† These authors have contributed equally to this work