- 1UWA Oceans Institute & School of Biological Sciences, University of Western Australia, Crawley, WA, Australia
- 2UMS 2006 Patrimoine Naturel, Muséum National d'Histoire Naturelle, Paris, France
- 3The Environment Institute and School of Earth and Environmental Sciences, University of Adelaide, Adelaide, SA, Australia
- 4Department of Environment, Water and Natural Resources, State Herbarium of South Australia, Adelaide, SA, Australia
- 5Departamento de Botânica, Centro de Ciências Biológicas, Universidade Federal de Santa Catarina, Florianópolis, Brazil
As plants on land, seaweeds are likely to be susceptible to temperature-mediated changes in phenology such as shifts in their reproductive timing. With this review, we aimed to investigate the importance of temperature on reproductive phenophase transitions (i.e., maturation and release of propagules) of temperate seaweeds while discussing how global warming might affect their reproductive phenology. A systematic literature search returned a total of 81 relevant papers, which were reviewed for evidence of environmental, factors (including temperature) driving reproductive phenology. Only a few of studies reported effects of temperature on propagule release (spores and gametes). In contrast, reproductive maturation (both sporogenesis and gametogenesis) was found predominantly to be controlled by temperature. Our findings highlight the potential for phenological shifts in seaweeds in response to ocean warming. In contrast to the consistent advancement of spring events observed for terrestrial plants, there was evidence that warming can both advance and delay the timing of reproductive events for temperate seaweeds, especially the maturation of propagules. Because temperature was often found to act in combination with either day length or spectral composition, ocean warming might result in a mismatch between light and temperature requirements that could lead to reduced reproductive performance.
Introduction
Shifts in phenology—the seasonal timing of species' life-cycle events—has been one of the most pervasive biological responses to global warming over the past decades (Walther et al., 2002; Parmesan and Yohe, 2003; Menzel et al., 2006; Parmesan, 2006; Poloczanska et al., 2013). The advancement of spring events like earlier flowering (Parmesan and Yohe, 2003; Menzel et al., 2006), has been particularly well-documented on land through long-term studies of plants, such as cherry blossom in Japan drawing on data as early as the nineth century (Menzel and Dose, 2005). Differences among species in phenological responses to climate change are likely to have important ecological consequences through asynchrony in interactions between trophic levels and mismatch between organism development and food availability (Edwards and Richardson, 2004; Visser and Both, 2005; Marshall et al., 2010; Thackeray et al., 2010).
Detecting impacts of climate change on species and ecosystems has been severely impeded by the lack of suitable baselines and time series against which to detect change, and these limitations have been particularly severe in marine ecosystems (Richardson and Poloczanska, 2008; Brown et al., 2011; Wernberg et al., 2012). However, globally, the seasonal timing of spring temperatures have been shifting 30–40% faster in the ocean than on land, seasonal isotherms moving forward at a rate of 1.5–2.5 days decade−1 (Burrows et al., 2011). Given that many marine species are in close equilibrium with their thermal niches (Sunday et al., 2012) and that thermal variation across varying time scales in the marine environment is narrower than those observed in most terrestrial ecosystems (Pearson and Brawley, 1996; Miller-Rushing and Primack, 2008), it is reasonable to expect that phenological shifts in marine species will be similar or even more pronounced in response to climate warming, to what has already been observed on land.
The evidence for more pronounced phenological changes in the sea is increasing, with marine studies reporting some of the highest rates of advancement in spring phenology. For example, gonad development of the limpet Patella depressa has advanced by 10.2 days decade−1 (Moore et al., 2011), blooms of dinoflagellates and development of meroplankton by 5.2 and 6.1 days decade−1, respectively (Edwards and Richardson, 2004), and the onset of spawning in the bivalve Macoma balthica by 4.4 days decade−1 (Philippart et al., 2003). A recent review of impacts of climate change in marine systems confirmed that average phenological shifts are greater in the ocean than land (Poloczanska et al., 2013).
A critical step in the phenology of any organism is the recurring and seasonal transitions between the different phases of the life cycle (vegetative or reproductive) or phenophases (such as breaking leaf buds, open flowers, pollen release for plants). For seaweeds, the reproductive phenophase transitions include the maturation and release of propagules (spores and/or gametes). Like plants on land, most temperate seaweeds (marine macroalgae) are finely tuned to changes in their environment and often have distinct seasonal growth and reproductive cycles (Dring, 1988; Brawley and Johnson, 1992; Lüning, 1993; Pearson et al., 1998; Mohring et al., 2013). Seaweeds comprise a group of phylogenetically unrelated, morphologically and physiologically diverse group of benthic autotrophic organisms that are found more diverse in temperate regions than in the tropics (mostly for the Rhodophyta and Phaeophyceae) (Kerswell, 2006; Keith, 2014). Forecasting how climate change and global temperature increases might affect the phenology of temperate seaweeds is difficult because many species have complex life cycles with an alternation between haploid and diploid phases (Figure 1) each of which could be controlled by an intricate interplay between endogenous cycles (e.g., circannual, circadian, or lunar) and a variety of environmental triggers. For example, in the kelp Saccharina latissima the growth cycle is under endogenous circannual regulation (Laminaria saccharina in Makarov et al., 1999), the formation of sori (clusters of sporangia which contain spores) is induced by short-day cycles within a specific temperature range (Lüning, 1988) while gametogenesis in female gametophytes is a blue-light mediated response (Lüning, 1980). Consequently, it remains a substantial challenge to identify where in the seaweed life cycle, a temperature increase is likely to have an effect, and in which direction (advancement or delay in the phenology) the outcome might change.
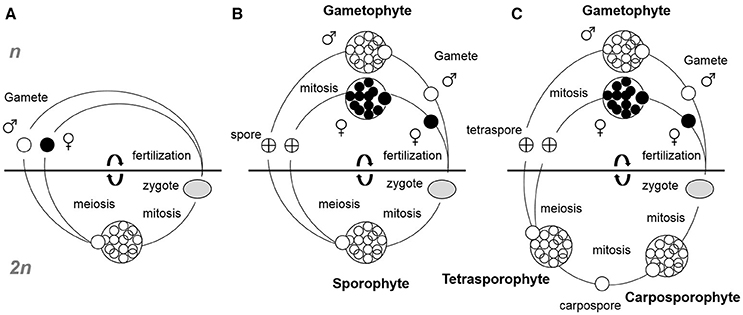
Figure 1. Simplified seaweed life cycles. (A) Diplontic (monophasic), (B) Diplohaplontic (biphasic), and (C) Diplohaplontic (triphasic). Haplontic monophasic life cycles are not shown as they mostly represent unicellular algae and not seaweeds. The horizontal lines separate the haploid (n) from diploid (2n) phases.
Seaweed life cycles can take one of three principal forms: haplontic monophasic (haploid thalli with zygotic meiosis), diplontic monophasic (diploid thalli with gametic meiosis, Figure 1A) or diplohaplontic biphasic or triphasic (different mode of alternation of generations between haploid and diploid generations, Figures 1B,C). The haplontic monophasic life cycle is most common for unicellular planktonic algae and less representative in seaweeds. The diplontic monophasic life cycle is characteristic of most fucoids brown algae (e.g., Fucus, Sargassum, Cystoseira), including the genus Durvillea and the green algal genus Codium. Diplohaplontic biphasic or triphasic life cycles are most common in seaweeds. Biphasic life cycles are well represented in the Laminariales and correspond to the alternation between macroscopic diploid sporophytes and microscopic haploid gametophytes. The more complex triphasic life cycles are common and restricted to the Rhodophyta and, in the majority of cases, encompass one haploid gametophyte phase and two diploid sporophyte phases. In this review, we deliberately discriminated propagule (here defined as spores and gametes) maturation from propagule release because it has been demonstrated that each process can be controlled by very different environmental factors, and therefore potentially exhibit different timing responses. For instance, Fucus vesiculosus in the northeast Atlantic initiates gametogenesis in response to short days (Bäck et al., 1991; Berger et al., 2001) whereas gamete release is a function of water movements and lunar or tidal cycles (Andersson et al., 1994; Serrão et al., 1996).
Despite the complexity and diversity of reproductive phenophase transitions found in temperate seaweeds (Figure 1) their need to be in tune with their environment to ensure evolutionary and ecological success implies a strong potential for temperature control over the phenophase transitions and for temperature-mediated phenological shifts. Here we review studies of the controls of phenophase transitions in temperate seaweeds. In doing so, we aimed to identify where temperature has been shown to affect different aspects of seaweed reproductive phenology to propose hypotheses about how global warming might affect seaweed reproductive phenology. Finally, we discuss the potential use of seaweed herbarium collections for detecting recent changes in seaweed phenology.
Methods
Search Criteria
We searched for peer-reviewed studies of factors driving reproductive phenology in temperate seaweeds (25–65° latitude). Given the vast literature available on the controls of life cycle transitions in temperate seaweeds, we deliberately focused our review only on temperate seaweeds to consider seaweeds thriving in relatively similar environmental conditions (see Wiencke and Clayton Margaret, 2009, for vegetative and reproductive phenology in polar seaweeds). Studies included both controlled laboratory experiments and detailed field observations. We searched available on-line data bases including the Web of Science, Current Contents, the UWA library collection, One Search and Google Scholar using different combinations of key words pertaining to seaweeds and their reproduction: “seaweed,” “macroalgae,” “temperate,” “reproduction,” “reproductive ecology,” “spore,” “zoospore,” “carpospore,” “tetraspore,” “gamete,” “phenology,” “seasonality,” “gametogenesis,” “sporogenesis,” “sporulation,” “tetrasporogenesis,” “control,” “trigger,” and “cue.” Additional papers were located by backtracking from the reference lists in the initial search publications. Papers were included in our review only if they presented field and/or laboratory data to infer induction of reproduction by exogenous or endogenous factors. We excluded all published works that referred to sporadic field observations and conclusions made without clear demonstration or reporting (Appendix 1 in Supplementary Material).
For each seaweed (sometimes the same species at different latitudes), we extracted information on species identity, location, which factors were considered and the corresponding phenological event(s) (Figure 2). We considered tetrasporogenesis and sporogenesis as one event (sporogenesis), but we did not pool this with carposporogenesis because this maturation is very likely the direct consequence of fertilization of the carpogonium and the subsequent development of gonimoblast tissue. We then quantified the frequency of seaweed identifying temperature as a trigger or control for four reproductive events (gametogenesis, sporogenesis, gamete release, spore release), alone or in combination with other factors.
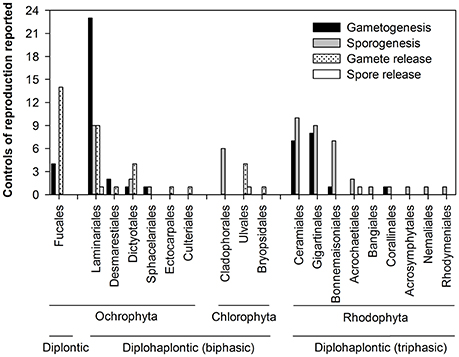
Figure 2. Distribution of reproductive events (gametogenesis, sporogenesis, gamete release, and spore release) controlled by environmental/endogenous factors reported in this review (N = 136 reproductive events) within seaweed order, phylum, and life cycle.
Results and Discussion
Limitations in Seaweed Phenology Research
We found 81 papers totalling 136 reproductive events from 71 temperate seaweed species. Most studies on the influence of environmental/endogenous factors on reproductive phenology (hereafter “reproductive events”) were controlled laboratory experiments (Appendix 1 in Supplementary Material). A large part of the reproductive events studied (>40%) originated from northwestern Europe where such research has been particularly abundant (Germany, France, Ireland, and UK, Appendix 1 in Supplementary Material) and from North America (~28%, USA, Canada). This geographical bias limits our understanding of temperature as a driver of reproductive phenology to a narrow range of seaweed taxa and latitudes. In general, the research on seaweed reproductive phenology identified by our selection criteria was most intensive between 1980 and 1995 then decreased for the most recent period (Appendix 1 in Supplementary Material). The strong geographical bias and the declining numbers of publications for phenological research in temperate seaweeds are worrying given the growing importance of phenological shifts in response to climate warming.
There was also a strong disparity in the seaweed taxa and reproductive events studied (Figure 2). Most research focused on five seaweed orders (~75%: Laminariales, Fucales, Ceramiales, Gigartinales, and Bonnemaisoniales) with ~30% of reproductive events (42 out 136) studied on Laminariales (Figure 2, Appendix 1 in Supplementary Material). Limited information was found on spore release (3/136 studies) compared to the other phenophase transitions, which were studied at higher frequency: 51, 47, and 35 out of 136 studies for sporogenesis, gametogenesis and gamete release (Figure 2, Appendix 1 in Supplementary Material). Data reported from the Fucales were mostly on gamete release (>75%) whereas gametogenesis, sporogenesis, and gamete release were the focus for Laminariales (>95%) (Figure 2, Appendix 1 in Supplementary Material). Most reproductive events in Rhodophyta were spore (~66%) and gamete maturation (~32%). We found limited information for Chlorophyta with only 6 studies on sporogenesis and 5 and 1 on gamete and spore release, respectively (12/136; Figure 2, Appendix 1 in Supplementary Material).
Role of Temperature in Phenophase Transitions
Propagule Release
Only a few studies demonstrated exogenous control of spore release such as wave motion in Alaria esculenta and Ulva lactuca (Gordon and Brawley, 2004) or bacteria in Acrochaetium sp. (Weinberger et al., 2007) (Appendix 1 in Supplementary Material). Many papers mentioned periodicity of spore release linked with tidal movement, specific day time (light intensity) and wave action to both facilitate the mechanical scouring of reproductive tissue and spore dispersal, and synchronize spore release once matured (Hoffmann, 1987; Amsler and Neushul, 1989; de Bettignies et al., 2013). A certain level of seasonal synchrony in spore release might be required to deliver propagules under conditions favorable for survival and/or growth (e.g., substrate availability, optimum temperature, etc.) and to maximize subsequent fertilization success when needed and sporophyte recruitment. Such synchrony might depend on the timing and rate of spore maturation (sporogenesis). Seaweeds like Undaria pinnatifida (Laminariales) in California show pulses in recruitment coinciding with temperature drops, indicating that temperature might play a role in some species for spore maturation/release when variation in seawater temperature is severe and/or sudden (Thornber et al., 2004). Alternatively, these recruitment pulses could have been driven by nutrients, which are highly correlated with temperature in California (Edwards and Estes, 2006).
Gamete release in the studied seaweeds was found to be triggered by various combinations of exogenous and endogenous factors such as lunar or tidal cycles (endogenous and exogenous), irradiance threshold (day light), water movement (release during calm periods) and female pheromones (Page and Kingsbury, 1968; Müller et al., 1979; Brawley and Johnson, 1992; Andersson et al., 1994; Serrão et al., 1996; Berndt et al., 2002; Gordon and Brawley, 2004; Engelen et al., 2008; Lüning et al., 2008) (Appendix 1 in Supplementary Material). Temperature was only found to be a good predictor of gamete release for two seaweeds, Ascophyllum nodosum in Maine (USA) (Bacon and Vadas, 1991) and Sargassum muticum in California (USA) (Norton, 1981), both in combination with tidal parameters.
Propagule Maturation
Many studies reported propagule maturation to be a temperature-mediated process with both gametogenesis and sporogenesis under the influence of temperature in 70–75% of the seaweeds studied (Appendix 1 in Supplementary Material, Figure 3). Most studies on propagule maturations were found for seaweeds with an alternation of generations such as the Laminariales and only a few orders of Rhodophyta represented (mainly Ceramiales, Bonnemaisoniales and Gigartinales; see Figure 2, Appendix 1 in Supplementary Material). Interestingly, temperature was observed to have opposite effects on reproductive maturation rate and timing depending on the species considered (Appendix 1 in Supplementary Material, Table 1), presumably because the effects can be triggered either by cold or warm temperature.
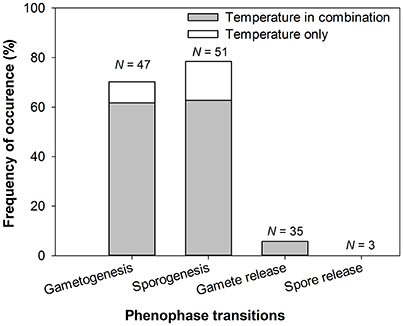
Figure 3. Occurrence of temperature as driver of reproductive events for each phenophase transition (gametogenesis, sporogenesis, gamete release, spore release), alone or in combination with other factors. N, number of reproductive events (136 reproductive events in total controlled by environmental/endogenous factors from 81 papers, totalling 71 seaweed species).
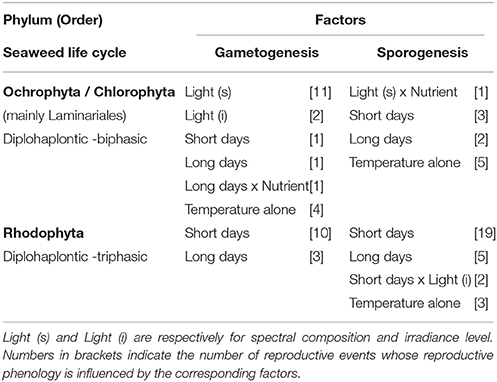
Table 1. Factors associated with reproductive maturation (gametogenesis and sporogenesis) of temperate seaweeds studied found in combination with temperature for the three main phyla/life cycles studied (Chlorophyta, Ochrophyta, and Rhodophyta).
For the majority of Laminariales studied, gametogenesis required low to medium temperature, relative to normal growth conditions, in combination with a specific spectral composition (Table 1, blue-light for most species of Laminaria) (Lüning and Neushul, 1978; Lüning, 1980). This suggests that an increase in seawater temperature might delay the formation and ultimately the release of gametes for many species of the Laminariales. Furthermore, reproduction (mostly sporogenesis) was often resumed as soon as conditions returned to favorable for growth (longer days and increasing irradiance) (Lüning and tom Dieck, 1989), and therefore warming might also result in a narrowing of the reproductive window for many species.
For most of the Rhodophyta species (77%), both gametogenesis and sporogenesis (usually tetrasporogenesis) were controlled by a unique combination of temperature and day length resulting in a strict reproductive window (Dring, 1984; Molenaar et al., 1996; Molenaar and Breeman, 1997) (Table 1). This mechanism provides an accurate timing of the different reproductive phases and ensures the three modes of reproduction are well ordered through different temperature-day length combinations (van den Hoek, 1982; Kain, 1987). Temperate red seaweeds fail to reproduce, or their reproductive response is much lower outside the specific photoperiodic and temperature requirements (Molenaar et al., 1996) and this temperature-day length regulation of reproduction has been invoked to explain phytogeographic “reproductive boundaries” (Colijn and van den Hoek, 1971; Rietema and Breeman, 1982; van den Hoek, 1982; Yarish et al., 1984). This highlights that seaweed distribution is not only a function of temperature range for growth and survival, but also reproduction. Often the persistence of species outside of these reproductive boundaries is only possible through vegetative propagation or sporadic environmental conditions favorable for sexual reproduction such as Asparagopsis armata in northern Europe (Guiry and Dawes, 1992). If temperature increase is non-lethal and photoperiod requirements are met (day length and number of cycles), then it can either delay the timing of reproductive events for “short-day” species (induction under lower-than-average temperature) or advance it for “long-day” species (induction under higher-than-average temperature). This effect is likely to be amplified in seaweed species where critical day lengths are modified by temperature, indicating that temperature can overcome photoperiod thresholds as the main driver of reproduction (Cunningham et al., 1993; Molenaar et al., 1996). Indeed shortening of the critical day length at lower temperatures for “short-day” species (Cunningham et al., 1993) and lengthening at higher temperature for “long-day” species (Molenaar et al., 1996) have been demonstrated. Collectively these findings (Table 1, Figure 3) stress the high potential for phenological shifts in temperate Rhodophyta under global warming, but the direction of shifts will depend on whether the seaweed is a “short-day” or “long-day” species.
In contrast to species from the Laminariales and some Rhodophyta species, we found insufficient information on temperature effects on gametogenesis in the Fucales (Appendix 1 in Supplementary Material). However, the commencement of receptacle development in spring for many Fucales, when light and seawater temperature start to increase (Terry and Moss, 1980; Bäck et al., 1991; Berger et al., 2001), suggest that temperature might play a role in this process. Recent findings (Kraufvelin et al., 2012) and previous work on gamete release (Norton, 1981; Bacon and Vadas, 1991) support this hypothesis, but more work is needed to understand the possible link between temperature, receptacle initiation and related factors such as day length. If the timing of reproduction is determined by temperature, it implies that warming could trigger earlier onset of receptacle initiation and subsequently earlier gamete release, which itself might not be under temperature-control.
Maturation of seaweed propagules requires time to complete, and consequently, cues indicative of seasonal changes are critical to deliver propagules when resources are optimal for their development. Both temperature and light parameters (particularly photoperiod) are reliable cues of seasonal change for the temperate seaweed species found in most studies (40–55° N, Appendix 1 in Supplementary Material) as indicated by their maximum seasonal variation across these latitudes (Appendix 2 in Supplementary Material). Temperature was often found in combination with different light characteristics such as day length, irradiance and spectral composition (Table 1, Figure 3). However, temperature and light changes indicative of season vary with latitude (Appendix 2 adapted from Wilczek et al., 2010) and their seasonal variations do not follow the same pattern with latitude (i.e., bell-shape curve of temperature vs. linear increase of seasonal variation in day length with increasing latitude, Appendix 2 in Supplementary Material). Consequently, their relative roles as seasonal cues for seaweed development might also vary with latitude. Temperate seaweeds of lower latitude (30–40° latitude) and tropical species might respond more to temperature variations than day length whereas seaweed reproductive development at higher latitude (50–65° latitude) and polar regions (65–90° latitude) might be more sensitive to light given their respective variations with latitude (Appendix 2 in Supplementary Material). More work at different latitudes is therefore needed to determine if the role of light and temperature variation (min/max, mean and or standard deviation of sea surface temperature) as triggers of reproductive phenology are consistent across latitudes.
Use of Herbarium Collections to Detect Phenological Shifts in Seaweeds
Detection of shifts in seaweed phenology in response to warming requires historical observations of timing of life cycle events such as reproduction, yet this information has rarely been collected systematically, particularly in the marine environment. However, like terrestrial botanists, phycologists have been preserving seaweeds in herbaria for at least two centuries (Coleman and Brawley, 2005; Wernberg et al., 2011). Because the reproductive organs are preserved in most herbarium specimens these collections are a promising source of historical information for detecting climate-related shifts in seaweed phenology and/or reduction of reproductively mature specimens (Primack et al., 2004; Miller-Rushing et al., 2006). The exploration of data contained in herbarium collections becomes highly relevant when and where no other source of baseline information exists and where sufficient numbers of exsiccatae for a same species have been preserved.
Some caution is however warranted when using herbarium collections because specimens were often primarily collected for taxonomic and/or biodiversity inventory purposes where reproductive organs have been important characteristics for identification. Collectors might thus have been biased to keep only reproductive specimens or species for which no reproductive structures are needed for accurate taxonomic identifications, such that the herbarium population could be non-representative of the overall population (Coleman and Brawley, 2005). Also, additional caution needs to be taken with herbarium data because temporal patterns might be confounded by sampling bias, spatial patterns, (e.g., more records and taxa are collected closer to major research centers than at any other location, and haphazard collections tend to occur closer to the summer during periods of good weather), and/or decadal variation of local temperatures. An analysis of the Dinard Herbarium in France demonstrated such bias with its algal collection reflecting changes in laboratory locations and differences in sampling strategies (exhaustive approach = few specimens for many taxa as possible vs. biocenotic approach = many specimen from different habitat for common taxa only) (Robuchon et al., 2016).
Conclusion
This review has highlighted the importance of temperature for reproductive maturation (both sporogenesis and gametogenesis) in temperate seaweeds. Although the literature is heavily biased toward mid to high latitudes in the northern Hemisphere and species from a limited number of seaweed orders, it is clear that different aspects of the reproductive phenology of temperate seaweeds are strongly linked to variation in temperature.
Contrary to the highly consistent unidirectional effect of warming, with the advance of spring events (e.g., earlier flowering), seen in most terrestrial plants (Primack et al., 2004; Menzel and Dose, 2005; Miller-Rushing and Primack, 2008), there was evidence to suggest that warming can both advance and delay reproductive phenology of temperate seaweeds, depending on a diversity of temperature requirements for reproductive maturation and interactions with additional cues such as day length. In many temperate seaweeds, the reproductive maturation (~60%) was also associated with specific light characteristics (e.g., day length or spectral composition) in addition to favorable temperatures. Warming might then result in a mismatch between temperature and light requirements exacerbating negative effects on reproduction and potentially lead to reproductive failure. There is a clear need for a broader understanding of the interplay between temperature, other environmental drivers (e.g., day length) and seaweed reproduction. While this line of research is picking up again (Oppliger et al., 2012; Bogaert et al., 2016; Martins et al., 2017) further effort should be made to develop more integrative approach with interaction experiments in ecophysiological research (Oppliger et al., 2012; Martins et al., 2017) in combination with modeling if possible. Such results should be put then in perspective with underlying molecular mechanisms (Liu et al., 2017). So far, phenological shifts in response to ocean warming have not been detected for seaweeds. This is, however, likely to reflect a lack of studies based on appropriate baseline data. Detailed investigations of historical herbarium collections could reveal shifts that are either analogous or opposite to observations from terrestrial plants, or occurring in multiple more complex directions such as spring advancement, followed by a pronounced summer interruption (due to excessive warming), followed by a new reproductive spike in late summer and early autumn. Regardless, it is clear that our mechanistic understanding of how warming might influence the reproductive phenology of temperate seaweeds is limited by strong geographic and taxonomical biases, and that this is a research area ready for renewed effort.
Author Contributions
TW and TdB conceived the study. TdB, TW, and CFDG designed the methodology. TdB collected and analyzed the data. TdB led the writing of the manuscript. All authors contributed critically to the drafts and gave final approval for publication.
Conflict of Interest Statement
The authors declare that the research was conducted in the absence of any commercial or financial relationships that could be construed as a potential conflict of interest.
Acknowledgments
This study was funded by the Australian Research Council through Linkage grant LP120100023 to TW and CFDG. We thank Gary Kendrick, Line Le Gall and the two reviewers for their constructive criticisms.
Supplementary Material
The Supplementary Material for this article can be found online at: https://www.frontiersin.org/articles/10.3389/fmars.2018.00218/full#supplementary-material
References
Amsler, C. D., and Neushul, M. (1989). Diel periodicity of spore release from the kelp Nereocystis luetkeana (Mertens) Postels et Ruprecht. J. Exp. Mar. Biol. Ecol. 134, 117–127. doi: 10.1016/0022-0981(90)90104-K
Andersson, S., Kautsky, L., and Kalvas, A. (1994). Circadian and lunar gamete release in Fucus vesiculosus in the atidal Baltic Sea. Mar. Ecol. Prog. Ser. 110, 195–201. doi: 10.3354/meps110195
Bäck, S., Collins, J. C., and Russell, G. (1991). Aspects of the reproductive biology of Fucus vesiculosus from the coast of SW Finland. Ophelia 34, 129–141. doi: 10.1080/00785326.1991.10429701
Bacon, L. C., and Vadas, R. L. (1991). A model for gamete release in Ascophyllum nodosum (Phaeophyta). J. Phycol. 27, 166–173. doi: 10.1111/j.0022-3646.1991.00166.x
Berger, R., Malm, T., and Kautsky, L. (2001). Two reproductive strategies in Baltic Fucus vesiculosus (Phaeophyceae). Eur. J. Phycol. 36, 265–273. doi: 10.1080/09670260110001735418
Berndt, M.-L., Callow, J. A., and Brawley, S. H. (2002). Gamete concentrations and timing and success of fertilization in a rocky shore seaweed. Mar. Ecol. Prog. Ser. 226, 273–285. doi: 10.3354/meps226273
Bogaert, K., Beeckman, T., and De Clerck, O. (2016). Abiotic regulation of growth and fertility in the sporophyte of Dictyota dichotoma (Hudson) J.V. Lamouroux (Dictyotales, Phaeophyceae). J. Appl. Phycol. 28, 2915–2924. doi: 10.1007/s10811-016-0801-z
Brawley, S. H., and Johnson, L. E. (1992). Gametogenesis, gametes and zygotes: an ecological perspective on sexual reproduction in the algae. Br. Phycol. J. 27, 233–252. doi: 10.1080/00071619200650241
Brown, C. J., Schoeman, D. S., Sydeman, W. J., Brander, K., Buckley, L. B., Burrows, M., et al. (2011). Quantitative approaches in climate change ecology. Glob. Chang. Biol. 17, 3697–3713. doi: 10.1111/j.1365-2486.2011.02531.x
Burrows, M. T., Schoeman, D. S., Buckley, L. B., Moore, P., Poloczanska, E. S., Brander, K. M., et al. (2011). The pace of shifting climate in marine and terrestrial ecosystems. Science 334, 652–655. doi: 10.1126/science.1210288
Coleman, M. A., and Brawley, S. H. (2005). Variability in temperature and historical patterns in reproduction in the Fucus distichus complex (Heterokontophyta; Phaeophyceae): implications for speciation and the collection of herbarium specimens. J. Phycol. 41, 1110–1119. doi: 10.1111/j.1529-8817.2005.00147.x
Colijn, F., and van den Hoek, C. (1971). The life history of Sphacelaria furcigera Kiitz.(Phaeophyceae) II. the influence of daylength and temperature on sexual and vegetative reproduction. Nova Hedwigia 21, 899–922.
Cunningham, E. M., Guiry, M. D., and Breeman, A. M. (1993). Environmental regulation of development, life history and biogeography of Helminthora stackhousei (Rhodophyta) by daylength and temperature. J. Exp. Mar. Biol. Ecol. 171, 1–21. doi: 10.1016/0022-0981(93)90136-C
de Bettignies, T., Wernberg, T., Lavery, P. S., Vanderklift, M. A., and Mohring, M. (2013). Contrasting mechanisms of dislodgement and erosion contribute to production of kelp detritus. Limnol. Oceanogr. 58, 1680–1688. doi: 10.4319/lo.2013.58.5.1680
Dring, M. J. (1988). Photocontrol of development in algae. Annu. Rev. Plant Physiol. Plant Mol. Biol. 39, 157–174. doi: 10.1146/annurev.pp.39.060188.001105
Edwards, M., and Richardson, A. J. (2004). Impact of climate change on marine pelagic phenology and trophic mismatch. Nature 430, 881–884. doi: 10.1038/nature02808
Edwards, M. S., and Estes, J. A. (2006). Catastrophe, recovery and range limitation in NE Pacific kelp forests: a large-scale perspective. Mar. Ecol. Prog. Ser. 320, 79–87. doi: 10.3354/meps320079
Engelen, A. H., Espirito-Santo, C., Simões, T., Monteiro, C., Serrão, E. A., Pearson, G. A., et al. (2008). Periodicity of propagule expulsion and settlement in the competing native and invasive brown seaweeds, Cystoseira humilis and Sargassum muticum (Phaeophyta). Eur. J. Phycol. 43, 275–282. doi: 10.1080/09670260801979279
Gordon, R., and Brawley, S. H. (2004). Effects of water motion on propagule release from algae with complex life histories. Mar. Biol. 145, 21–29. doi: 10.1007/s00227-004-1305-y
Guiry, M. D., and Dawes, C. J. (1992). Daylength, temperature and nutrient control of tetrasporogenesis in Asparagopsis armata (Rhodophyta). J. Exp. Mar. Biol. Ecol. 158, 197–217. doi: 10.1016/0022-0981(92)90227-2
Hoffmann, A. J. (1987). The arrival of seaweed propagules at the shore: a review. Bot. Mar. 30, 151–166. doi: 10.1515/botm.1987.30.2.151
Kain, J. M. (1987). Photoperiod and temperature as triggers in the seasonality of Delesseria sanguinea. Helgoländer Meeresuntersuchungen 41, 355–370. doi: 10.1007/BF02366198
Keith, S. A. (2014). Global diversity of marine macroalgae: environmental conditions explain less variation in the tropics. Global Ecol. Biogeogr. 23, 517–529. doi: 10.1111/geb.12132
Kerswell, A. P. (2006). Global biodiversity patterns of benthic marine algae. Ecology 87, 2479–2488. doi: 10.1890/0012-9658(2006)87[2479:GBPOBM]2.0.CO;2
Kraufvelin, P., Ruuskanen, A., Bäck, S., and Russell, G. (2012). Increased seawater temperature and light during early springs accelerate receptacle growth of Fucus vesiculosus in the northern Baltic proper. Mar. Biol. 159, 1795–1807. doi: 10.1007/s00227-012-1970-1
Liu, X., Bogaert, K., Engelen Aschwin, H., Leliaert, F., Roleda Michael, Y., and De Clerck, O. (2017). Seaweed reproductive biology: environmental and genetic controls. Bot. Mar. 60:89. doi: 10.1515/bot-2016-0091
Lüning, K. (1980). Critical levels of light and temperature regulating the gametogenesis of three Laminaria species (Phaeophyceae). J. Phycol. 16, 1–15. doi: 10.1111/j.1529-8817.1980.tb02992.x
Lüning, K. (1988). Photoperiodic control of sorus formation in the brown alga Laminaria saccharina. Mar. Ecol. Prog. Ser. 45, 137–144. doi: 10.3354/meps045137
Lüning, K. (1993). Environmental and internal control of seasonal growth in seaweeds. Hydrobiologia 260–261, 1–14. doi: 10.1007/978-94-011-1998-6_1
Lüning, K., and tom Dieck, I. (1989). Environmental triggers in algal seasonality. Bot. Mar. 32, 389–397. doi: 10.1515/botm.1989.32.5.389
Lüning, K., Kadel, P., and Pang, S. (2008). Control of reproduction rhytmicity by environmental and endogenous signals in Ulva pseudocurvata (Chlorophyta). J. Phycol. 44, 866–873. doi: 10.1111/j.1529-8817.2008.00535.x
Lüning, K., and Neushul, M. (1978). Light and temperature demands for growth and reproduction of laminarian gametophytes in southern and central California. Mar. Biol. 45, 297–309. doi: 10.1007/BF00391816
Makarov, V. N., Makarov, M. V., and Schoschina, E. V. (1999). Seasonal dynamics of growth in the Barents Sea seaweeds: endogenous and exogenous regulation. Bot. Mar. 42:43. doi: 10.1515/BOT.1999.007
Marshall, D. J., Monro, K., Bode, M., Keough, M. J., and Swearer, S. (2010). Phenotype–environment mismatches reduce connectivity in the sea. Ecol. Lett. 13, 128–140. doi: 10.1111/j.1461-0248.2009.01408.x
Martins, N., Tanttu, H., Pearson Gareth, A., Serrão Ester, A., and Bartsch, I. (2017). Interactions of daylength, temperature and nutrients affect thresholds for life stage transitions in the kelp Laminaria digitata (Phaeophyceae). Bot. Mar. 60, 109–121. doi: 10.1515/bot-2016-0094
Menzel, A., and Dose, V. (2005). Analysis of long-term time series of the beginning of flowering by Bayesian function estimation. Meteorol. Zeitschr. 14, 429–434. doi: 10.1127/0941-2948/2005/0040
Menzel, A., Sparks, T. H., Estrella, N., Koch, E., Aasa, A., Ahas, R., et al. (2006). European phenological response to climate change matches the warming pattern. Glob. Chang. Biol. 12, 1969–1976. doi: 10.1111/j.1365-2486.2006.01193.x
Miller-Rushing, A. J., and Primack, R. B. (2008). Global warming and flowering times in Thoreau's Concord: a community perspective. Ecology 89, 332–341. doi: 10.1890/07-0068.1
Miller-Rushing, A. J., Primack, R. B., Primack, D., and Mukunda, S. (2006). Photographs and herbarium specimens as tools to document phenological changes in response to global warming. Am. J. Bot. 93, 1667–1674. doi: 10.3732/ajb.93.11.1667
Mohring, M., Wernberg, T., Kendrick, G., and Rule, M. (2013). Reproductive synchrony in a habitat-forming kelp and its relationship with environmental conditions. Mar. Biol. 160, 119–126. doi: 10.1007/s00227-012-2068-5
Molenaar, F. J., and Breeman, A. M. (1997). Latitudinal trends in the growth and reproductive seasonality of Delesseria sanguinea, Membranoptera alata, and Phycodrys rubens (Rhodophyta). J. Phycol. 33, 330–343. doi: 10.1111/j.0022-3646.1997.00330.x
Molenaar, F. J., Breeman, A. M., and Venekamp, L. A. H. (1996). Ecotypic variation in Cystoclonium purpureum (Rhodophyta) synchronizes life history events in different regions. J. Phycol. 32, 516–525. doi: 10.1111/j.0022-3646.1996.00516.x
Moore, P. J., Thompson, R. C., and Hawkins, S. J. (2011). Phenological changes in intertidal con-specific gastropods in response to climate warming. Glob. Chang. Biol. 17, 709–719. doi: 10.1111/j.1365-2486.2010.02270.x
Müller, D. G., Gassmann, G., and Lüning, K. (1979). Isolation of a spermatozoid-releasing and -attracting substance from female gametophytes of Laminaria digitata. Nature 279, 430–431. doi: 10.1038/279430a0
Norton, T. A. (1981). Gamete expulsion and release in Sargassum muticum. Bot. Mar. 24, 465–470. doi: 10.1515/botm.1981.24.8.465
Oppliger, L. V., Correa, J. A., Engelen, A. H., Tellier, F., Vieira, V., Faugeron, S., et al. (2012). Temperature effects on gametophyte life-history traits and geographic distribution of two cryptic kelp species. PLoS ONE 7:e39289. doi: 10.1371/journal.pone.0039289
Page, J. Z., and Kingsbury, J. M. (1968). Culture studies on the marine green alga Halicystis parvula-Derbesia tenuissima. II. Synchrony and periodicity in gamete formation and release. Am. J. Bot. 55, 1–11. doi: 10.1002/j.1537-2197.1968.tb06937.x
Parmesan, C. (2006). Ecological and evolutionary responses to recent climate change. Annu. Rev. Ecol. Evol. Syst. 37, 637–669. doi: 10.1146/annurev.ecolsys.37.091305.110100
Parmesan, C., and Yohe, G. (2003). A globally coherent fingerprint of climate change impacts across natural systems. Nature 421, 37–42. doi: 10.1038/nature01286
Pearson, G. A., and Brawley, S. H. (1996). Reproductive ecology of Fucus distichus (Phaeophyceae): an intertidal alga with successful external fertilization. Mar. Ecol. Prog. Ser. 143, 211–223. doi: 10.3354/meps143211
Pearson, G. A., Serrão, E. A., and Brawley, S. H. (1998). Control of gamete release in fucoid algae: sensing hydrodynamic conditions via carbon acquisition. Ecology 79, 1725–1739. doi: 10.1890/0012-9658(1998)079[1725:COGRIF]2.0.CO;2
Philippart, C. J. M., Aken, H. M. V., Beukema, J. J., Bos, O. G., Cadée, G. C., and Dekker, R. (2003). Climate-related changes in recruitment of the bivalve Macoma balthica. Limnol. Oceanogr. 48, 2171–2185. doi: 10.4319/lo.2003.48.6.2171
Poloczanska, E. S., Brown, C. J., Sydeman, W. J., Kiessling, W., Schoeman, D. S., Moore, P. J., et al. (2013). Global imprint of climate change on marine life. Nat. Clim. Chang. 3, 919–925. doi: 10.1038/nclimate1958
Primack, D., Imbres, C., Primack, R. B., Miller-Rushing, A. J., and Del Tredici, P. (2004). Herbarium specimens demonstrate earlier flowering times in response to warming in Boston. Am. J. Bot. 91, 1260–1264. doi: 10.3732/ajb.91.8.1260
Richardson, A. J., and Poloczanska, E. S. (2008). Ocean science. under-resourced, under threat. Science 320, 1294–1295. doi: 10.1126/science.1156129
Rietema, H., and Breeman, A. M. (1982). The regulation of the life history of Dumontia contorta in comparison to that of several other Dumontiaceae (Rhodophyta). Bot. Mar. 25, 569–576. doi: 10.1515/botm.1982.25.12.569
Robuchon, M., Lamy, D., Kervran, L., Dennetiere, B., Julliard, R., and Le Gall, L. (2016). Dinard herbarium: a source of information to infer temporal changes in seaweed communities? Crypt. Algol. 37, 47–60. doi: 10.7872/crya/v37.iss1.2016.47
Serrão, E. A., Pearson, G., Kautsky, L., and Brawley, S. H. (1996). Successful external fertilization in turbulent environments. Proc. Natl. Acad. Sci. U.S.A. 93, 5286–5290. doi: 10.1073/pnas.93.11.5286
Sunday, J. M., Bates, A. E., and Dulvy, N. K. (2012). Thermal tolerance and the global redistribution of animals. Nat. Clim. Chang. 2, 686–690. doi: 10.1038/nclimate1539
Terry, L. A., and Moss, B. L. (1980). The effect of photoperiod on receptacle initiation in Ascophyllum nodosum (L.) Le Jol. Br. Phycol. J. 15, 291–301. doi: 10.1080/00071618000650301
Thackeray, S. J., Sparks, T. H., Frederiksen, M., Burthe, S., Bacon, P. J., Bell, J. R., et al. (2010). Trophic level asynchrony in rates of phenological change for marine, freshwater and terrestrial environments. Glob. Chang. Biol. 16, 3304–3313. doi: 10.1111/j.1365-2486.2010.02165.x
Thornber, C. S., Kinlan, B. P., Graham, M. H., and Stachowicz, J. J. (2004). Population ecology of the invasive kelp Undaria pinnatifida in California: environmental and biological controls on demography. Mar. Ecol. Prog. Ser. 268, 69–80. doi: 10.3354/meps268069
van den Hoek, C. (1982). The distribution of benthic marine algae in relation to the temperature regulation of their life histories. Biol. J. Linnean Soc. 18, 81–144. doi: 10.1111/j.1095-8312.1982.tb02035.x
Visser, M. E., and Both, C. (2005). Shifts in phenology due to global climate change: the need for a yardstick. Proc. R. Soc. B Biol. Sci. 272, 2561–2569. doi: 10.1098/rspb.2005.3356
Walther, G.-R., Post, E., Convey, P., Menzel, A., Parmesan, C., Beebee, T. J. C., et al. (2002). Ecological responses to recent climate change. Nature 416, 389–395. doi: 10.1038/416389a
Weinberger, F., Beltran, J., Correa, J. A., Lion, U., Pohnert, G., Kumar, N., et al. (2007). Spore release in Acrochaetium sp. (Rhodophyta) is bacterially controlled. J. phycol. 43, 235–241. doi: 10.1111/j.1529-8817.2007.00329.x
Wernberg, T., Russell, B. D., Thomsen, M. S., Gurgel, C. F. D., Bradshaw, C. J. A., Poloczanska, E. S., et al. (2011). Seaweed communities in retreat from ocean warming. Curr. Biol. 21, 1828–1832. doi: 10.1016/j.cub.2011.09.028
Wernberg, T., Smale, D. A., and Thomsen, M. S. (2012). A decade of climate change experiments on marine organisms: procedures, patterns and problems. Glob. Chang. Biol. 18, 1491–1498. doi: 10.1111/j.1365-2486.2012.02656.x
Wiencke, C., and Clayton Margaret, N. (2009). Biology of polar benthic algae. Bot. Mar. 52, 479–481. doi: 10.1515/BOT.2009.083
Wilczek, A. M., Burghardt, L. T., Cobb, A. R., Cooper, M. D., Welch, S. M., and Schmitt, J. (2010). Genetic and physiological bases for phenological responses to current and predicted climates. Philos. Trans. R. Soc. B Biol. Sci. 365, 3129–3147. doi: 10.1098/rstb.2010.0128
Keywords: temperate macroalgae, life cycle, phenophase, sporogenesis, gametogenesis, reproduction, warming, herbarium data
Citation: de Bettignies T, Wernberg T and Gurgel CFD (2018) Exploring the Influence of Temperature on Aspects of the Reproductive Phenology of Temperate Seaweeds. Front. Mar. Sci. 5:218. doi: 10.3389/fmars.2018.00218
Received: 08 November 2017; Accepted: 04 June 2018;
Published: 20 June 2018.
Edited by:
Nuria Marba, Consejo Superior de Investigaciones Científicas (CSIC), SpainReviewed by:
Aschwin Hillebrand Engelen, University of Algarve, PortugalEmma Cebrian, University of Girona, Spain
Copyright © 2018 de Bettignies, Wernberg and Gurgel. This is an open-access article distributed under the terms of the Creative Commons Attribution License (CC BY). The use, distribution or reproduction in other forums is permitted, provided the original author(s) and the copyright owner are credited and that the original publication in this journal is cited, in accordance with accepted academic practice. No use, distribution or reproduction is permitted which does not comply with these terms.
*Correspondence: Thibaut de Bettignies, dGhpYmF1dC5kZS1iZXR0aWduaWVzQG1uaG4uZnI=
Thomas Wernberg, dGhvbWFzLndlcm5iZXJnQHV3YS5lZHUuYXU=