- 1Division of Biological and Environmental Science and Engineering, Red Sea Research Center, King Abdullah University of Science and Technology, Thuwal, Saudi Arabia
- 2Coral Reef Laboratory, Ocean and Earth Science, National Oceanography Centre, University of Southampton, Southampton, United Kingdom
- 3Institute for Life Sciences, University of Southampton, Southampton, United Kingdom
- 4Marine Biology Laboratory, Centre for Genomics and Systems Biology, New York University Abu Dhabi, Abu Dhabi, United Arab Emirates
The adaptation of tropical coral communities to the world's hottest sea, the Persian/Arabian Gulf (PAG), has recently been associated with ecological selection acting on a group of coral-associated algal symbionts, the Symbiodinium thermophilum group. Previous studies have shown that considerable genetic diversity exists within the group and that group members found within the PAG are significantly differentiated from those found externally, in the Gulf of Oman and wider waters. However, little is known about this genetic diversity. As an initial step towards understanding whether this diversity could represent niche adapted, selectable populations within the S. thermophilum group that may act as natural sources of stress tolerant associations to Indo-Pacific reefs, we investigate whether the diversity is structured between populations and where the location of the internal-external genetic partition lies. We use regions of the nuclear ribosomal DNA (ITS1-5.8S-ITS2) and chloroplastic psbA gene (non-coding region) from >100 S. thermophilum group-harbouring Porites spp. (P. lobata, P. lutea, and P. harrisoni) sampled across steep temperature and salinity gradients to conduct analyses of variance and create maximum parsimony networks to assess genetic structure and (dis)similarity within and between populations of S. thermophilum found within the PAG and externally in the Gulf of Oman. Our analyses resolve a sharp genetic boundary between Symbiodinium populations in the western Strait of Hormuz and identify significant genetic structure between populations with as little as 20 km between them demonstrating that differentiation between populations is likely due to factors other than limited connectivity. Further, we hypothesize that genotypes identified outside of the PAG in the Gulf of Oman existing in near-oceanic salinities, yet thermally challenging waters, putatively represent candidates for stress-tolerant symbionts that could act as natural seed populations of stress tolerant genotypes to the wider indo-Pacific.
Introduction
The Persian/Arabian Gulf (PAG) is a thermally extreme and hypersaline body of water with temperatures regularly exceeding 34°C during summer in its southern and hottest part (Hume et al., 2013; Shuail et al., 2016; Figure 1, Figure S1), temperatures that prove fatal to most tropical corals (Coles and Riegl, 2013; Hume et al., 2013). Yet, thriving coral communities are found along its coastline (Coles, 2003). These exceptional coral communities are gaining attention as model systems to inform on how corals globally might adapt to increasing sea surface temperatures (SSTs; Hume et al., 2013; Burt et al., 2014; D'Angelo et al., 2015). Such increases are causing more frequent and severe coral bleaching events (Logan et al., 2014) during which the obligate symbiotic relationship between coral and algal symbiont (genus Symbiodinium) breaks down leading to symbiont loss, and if recovery is not timely, death of the host (Hughes et al., 2003). Temperature thresholds at which these bleaching events occur are dependent on host and symbiont genotypes but typically lie 1°C above the average maximum summer temperature for a reef (Goreau and Hayes, 1994; Shuail et al., 2016).
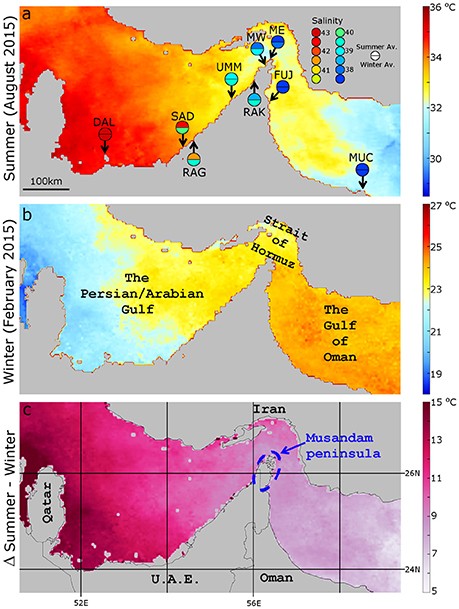
Figure 1. Sampling sites across temperature and salinity gradients of the Persian/Arabian Gulf, Strait of Hormuz, and Gulf of Oman. (a) Monthly average (August 2015; warmest month) sea surface temperatures (SSTs) representative of a summer thermal profile. Sampling locations are denoted according to three or two letter codes: DAL, Dalma Island; SAD, Saadiyat reef; RAG, Ras Ghanada; UMM, Umm Al-Quwain; RAK, Ras Al-Khaimah; MW, Musandam peninsula West; ME, Musandam peninsula East; FUJ, Fujairah; & MUC, Muscat. Modelled seasonal (summer, J-A-S; winter, J-F-M) salinities from Yao and Johns (2010) for sampling sites are denoted. (b) Monthly average (February 2015; coolest month) SSTs representative of a winter thermal profile. (c) Difference in monthly average SSTs between (a and b) representative of a regular seasonal temperature range. Remotely sensed SST data of (a,b) are Aqua MODIS Sea Surface Temperature (11 μ daytime), monthly, 4 km resolution accessed through the level 3 data browser of OceanColor Web (oceancolor.gsfc.nasa.gov/cgi/I3). netCDF libraries were viewed and images exported using Panoply 4.3 (gis.nasa.gov/tools/panoply). Panel (c) was created using Panoply's combine plot function subtracting the winter profile from the summer.
Given that the maximum mean temperature of the majority of coral reefs is ≤30°C (Hume et al., 2013), the ability of corals from the southern PAG to withstand temperatures in excess of 35°C without bleaching is truly exceptional (Coles and Riegl, 2013; Hume et al., 2013; Shuail et al., 2016). This exceptional thermal tolerance may be in part due to their algal associations given that hosting certain Symbiodinium types may confer an increased thermal tolerance to the coral host (LaJeunesse et al., 2010; Silverstein et al., 2015). Specifically, corals of the southern PAG predominantly form year-round associations with the recently described Symbiodinium thermophilum (Hume et al., 2015). The prevalence of this symbiont within PAG corals suggests it is at least partially responsible for their thermotolerance (D'Angelo et al., 2015; Hume et al., 2015, 2016).
S. thermophilum was defined using four genetic markers including specific sequences of the mitochondrial cytochrome b (cob; K234522) and chloroplastic ribosomal 23S (cp23S; KP234523) (Hume et al., 2015). In addition, genetic characteristics of the nrDNA second internal transcribed spacer regions (ITS2) were used. This non-coding region is multicopy in character with multiple genetic variants of different but closely related sequences present in an individual Symbiodinium genome (Thornhill et al., 2007; Arif et al., 2014). Within S. thermophilum, an 8bp duplication insert (called S. thermo.-indel) in a minority proportion of intragenomic ITS2 variants (~15%) in an otherwise C3 type sequence (KP234524 & KM487748) was described and its presence has been used as an effective means of screening for this symbiont species (D'Angelo et al., 2015; Hume et al., 2016). The last genetic marker used in the description, the highly variable chloroplastic psbA non-coding region (psbAncr), resolves at a subspecies level in the Symbiodinium genus (Thornhill et al., 2014). As such, multiple haplotypes were recovered from the S. thermophilum symbionts (Hume et al., 2015). Critically, all sequences resolved within a monophyletic grouping separated from other ITS2 C3 type symbionts by considerable genetic distance (Hume et al., 2015). Since the formal description of S. thermophilum, additional samples containing the characteristic ITS2 S. thermo.-indel have been genotyped using the psbAncr (D'Angelo et al., 2015; Hume et al., 2016). These recovered psbAncr sequences showed a considerably larger diversity than the diversity seen in the original sensu strictu S. thermophilum definition. Hence, these symbionts are identified as members of the “S. thermophilum group” (D'Angelo et al., 2015).
The extreme conditions and exceptional coral-algal associations found in the PAG are a product of the regions unique geography. The PAG is connected to the adjacent Gulf of Oman by the ~40 km wide Strait of Hormuz that navigates the Musandam Peninsula and is fed by a dominant surface inflow of near-oceanic salinity water (Figure 1). Due to high evaporation rates and minimal freshwater input, the PAG is characterised by a net inflow with only a minor deep water outflow of heavier, more saline water through the Strait (Yao and Johns, 2010). This surface inflow is strongest in summer month with the cooler, lower salinity surface water moving northwest towards Qatar or south into the main basin forming an overall cyclonic circulation in the southern Gulf (Yao and Johns, 2010). Despite this lower salinity inflow, salinities in the southern PAG remain high year round (>41.5) resulting in a gradual decrease in salinity from the southern PAG towards the tip of the Musandam Peninsula into the Gulf of Oman (D'Angelo et al., 2015; Figure 1a). Temperature gradients along the same coast are seasonally dependent. In summer, the inflow has a cooling effect and the resultant temperature gradient decreases from the southern PAG to the Gulf of Oman whereas in winter month the southern PAG is considerably cooler (winter averages ~21°C) than the inflowing water (~24°C; Figures 1a,b).
Although cooler than the PAG, the coral-harbouring waters immediately external to it, in the Gulf of Oman, represent an environment with salinities closer to those of the wider Indo-Pacific but with thermal regimes still greater than are found in the majority of coral-containing waters globally. In addition to the relatively large annual thermal ranges of the region (max ~31°C, min ~23°C), coral communities in these waters may experience acute hypothermal shocks due to local upwelling events (Coles, 2003). As such the coral communities of these waters should also be considered extraordinarily stress tolerant.
Recently, the abundance of S. thermophilum group symbionts was investigated at a range of sites spanning from within the PAG to Muscat, in the neighbouring Gulf of Oman. Along the southern coast of the PAG from Dalma to Ras Al-Khaimah, the association of Porties spp. corals with members of the S. thermophilum group was complete (100% of associations) despite considerable changes in salinity and temperature (D'Angelo et al., 2015; Hume et al., 2015, 2016). However, immediately external to the PAG off the coast of the Musandam Peninsula in the Strait of Hormuz an increasing number of the Porites spp. corals were found in association with symbiont types other than S. thermophilum, namely ITS2 type C15 and S. trenchii (ITS2 type D1a or D1-4). This decrease in S. thermophilum group associations continues with increasing distance from the PAG until associations are almost undetectable in reefs off Muscat. Interestingly, this low level abundance of S. thermophilum group associations has been documented as widely distributed as the northern Red Sea (Hume et al., 2016).
Phylogenetic analyses conducted as part of these studies using the psbAncr revealed a greater genetic diversity of S. thermophilum group symbionts external to the PAG than within (Hume et al., 2016). Investigation into the age of this diversity (>several million years), and the group members themselves (~13 Mya), demonstrated them to be considerably older than the age of the PAG (~12.5 kya; Purkis et al., 2010; D'Angelo et al., 2015; Hume et al., 2016). These findings led to the hypothesis that positive selection for thermal and salinity tolerance acting on the diversity of group members found external to the PAG, facilitated the colonisation of the physically extreme PAG by a subset of Indo-Pacific corals. Other ecological mechanisms including founder or bottleneck effects were also considered in the explanation of the group's prevalence and relative genetic homogeneity in the PAG. However, the PAG's dominant inflow (Yao and Johns, 2010), the presence of several other thermally tolerant symbiont types within the PAG (Baker et al., 2004; LaJeunesse et al., 2014; Hume et al., 2015) and the fact that Symbiodinium can disperse rapidly over large distances (Baird et al., 2009; Pettay et al., 2015) make these mechanisms unlikely.
In the same way that members of the S. thermophilum group underwent ecological selection to proliferate in the extreme conditions of the PAG, these members may be selected to enrich reefs in the Gulf of Oman and wider Indo-Pacific with tolerant symbionts. Such an enrichment may afford these reefs greater resilience to perturbations that may arise from predicted increases in SSTs. However, to better understand the capacity for such ecological selection to occur we must first better understand the genetic diversity that exists within the S. thermophilum group to know which populations are available for selection. Here, we specifically investigate whether the genetic diversity of the S. thermophilum group is structured between populations as a first indication towards whether niche adapted, selectable populations exist. To assess for genetic structure within the group, we use a novel approach analysing the diversity of intragenomic variants in the ITS1-5.8S-ITS2 region of the nrDNA as well as the psbAncr to compute ϕst indices and create maximum parsimony networks to identify population groupings and assess genetic (dis)similarity within and between individual populations. Our findings identify significant genetic differentiation between S. thermophilum group populations and further resolve the coral-symbiont biogeographical boundary in the Strait of Hormuz.
Materials and Methods
Sample Collection and Genotyping by nrDNA and psbAncr
S. thermophilum group-containing coral samples of P. lobata, P. lutea, and P. harrisoni were collected and underwent nrDNA (ITS1-5.8S-ITS2) and psbAncr genotyping as part of D'Angelo et al. (2015). Field identification relied on local experts to differentiate between Porites spp. The relatively low diversity of Porites spp. found in the PAG and Gulf of Oman simplified the identification (Bauman et al., 2013). Fragments of P. lobata and P. lutea were bleached in sodium hypochlorite solution and inspected under a microscope (MZ10, Leica). Analysis of the corallite structure (Veron, 2000) confirmed the field identification of these samples. The P. lobata samples were distinguishable from P. lutea by the lack of a protruding ring of five tall pali, and from P. solida by the lack of a flattened columella. However, it should be noted that Porites spp. corals are notoriously difficult to identify from skeletal morphology alone (Forsman et al., 2009). P. harrisoni was differentiated from P. lobata and P. lutea by its gross morphology being more columnar in form. Unfortunately for samples collected at Ras Al Khaimah (RAK) and the one sample collected in Muscat, field identification by an expert was not available. Rather than omit these samples from the study they were identified conservatively as Porites spp.
In summary, corals were sampled at between 2 and 7 m in September 2012 and March 2013 from sites within the PAG, the Strait of Hormuz and the Gulf of Oman (Figure 1; Figure S2). Throughout this study two or three character length codes (e.g., DAL—Dalma) will be used to refer to sampling sites as denoted in Figure 1a. Genomic DNA extractions were performed using a CTAB protocol and the PCR amplification of the nrDNA and psbAncr markers was achieved using the SYM_VAR_FWD/SYM_VAR_REV and psbAFor_1/psbA_Rev_1 primer pairs. nrDNA amplicons were cloned using a StrataClone PCR cloning kit before sequencing (universal primer T7) whilst psbAncr amplicons were directly sequenced using the internal primer psbA_int_Fwd. Sequences were aligned in MEGA 6 (Tamura et al., 2013) with nrDNA sequences' unsupported nucleotides (nucleotides divergent from the alignment consensus that were not found in more than one PCR amplicon library) reverted to the consensus to minimise incorporation of genetic diversity generated through PCR error. A full list of psbAncr sequences used in this study may be found in Table S1, Supplementary Material (106 sequences). The number of colonies nrDNA sequenced as well as the average sequencing depth can be found in Table S2, Supplementary Material. A multiple sequence alignment of the ITS1-5.8S-ITS2 nrDNA sequences used in this study has been submitted to the Dryad Repository (675 sequences).
Assessment of Host-Effect on Symbiodinium Sequence
Porites spp. abundance between sites was heterogeneous and uneven sampling across sites was unavoidable (Figure S2). To assess whether any signature of genetic structure found in this study might be an artefact caused by host species distribution due to different species of Porites hosting different Symbiodinium types, rather than other factors (e.g., environmental conditions), cluster-based correlation analysis between Symbiodinium sequence recovered and host species was conducted. For both markers, genetic distances between all unique sequences were calculated in MOTHUR 1.36.1 (Schloss et al., 2009) using the “pairwise.seqs” function. Column-based distances were converted to matrices using a custom PYTHON script before being plotted in two dimensional space through multidimensional scaling using the R package bios2mds. K-means clustering was conducted in R (3 clusters; one per species) using the function “kmeans” with the initial seed set to 20 and with an nstart equalling 50.
If host species had no effect on sequence recovered, sequences should be randomly distributed between the three groups irrespective of host. A two-way table (species, cluster) and chi-square test were employed to test if there was a significant difference between the distribution of sequences amongst the three k-means determined clusters according to either: observed distribution by species, or expected random distribution disregarding species. The counts of sequences from each of the three identified species (unidentified Porites sp. were discounted from the analysis) associated within each of the determined clusters were calculated alongside frequencies representative of the null hypothesis of no association between species and cluster. A chi-squared test was performed and results were compared to critical values at 4 degrees of freedom (3 species, 3 clusters).
Inclusion of ITS1 and 5.8s nrDNA Regions in Genetic Analyses
Biogeographical and phylogenetic analyses of Symbiodinium populations often use only one region of the nrDNA, commonly the ITS2. To maximise possible inferences on genetic structure and investigate possible improvements in phylogenetic resolution within the S. thermophilum group the ITS1 and 5.8S regions of the nrDNA were included in all analyses in this study.
Population Genetic Structure Inference by Analysis of Variance
To offer an improved resolution of the previously described biogeographic boundary (located in the Strait of Hormuz) to S. thermophilum group-containing coral populations (D'Angelo et al., 2015; Hume et al., 2016) three group configurations were tested for variance. Specifically, two groups of populations were defined as “internal” and “external” (approximate to PAG and Gulf of Oman populations). Within these groupings the sites, Dalma, Saadiyat, Ras Ghanada, Umm Al-Quwain, and Ras Al-Khaimah were always placed in the internal groups whilst Fujairah and Muscat were always in the external group. The three permutations of these groups with the addition or lack of one or both of the Musandam West or Musandam East sites were then tested for among group variance in WinArl 3.5.1.3 (Excoffier and Lischer, 2010) using the complete ITS1-5.8S-ITS2 region of the nrDNA (611 bp) and the partial psbAncr (495 bp). By this means, the two populations in proximity to the Strait with the greatest haplotypic difference may be identified. These two populations will be the most easterly and westerly population in the internal and external groupings that have largest among group variance.
In addition to the calculation of among groups variance (Va), among populations within groups (Vb), and within populations variances (Vc) were calculated along with their associated fixation indices (φCT, φSC, and φST, respectively). In this analytical scenario, the among group variance (Va) represents the variance between individual gene sequences that are grouped as belonging to either an internal or external site. When calculating the among group variance, the population to which a sequence belongs is not taken into account. That is, individual gene comparisons are only constrained by which group they belong to. The among populations within groups variance (Vb) represents the average variance found amongst populations that belong to the same group. Here, population comparisons are nested hierarchically within the groups so that only gene sequences found within populations that are found within the same groups will be compared. Within population variance (Vc) represents the variance found between sequences for each population. Here, which group the population belongs to is not taken into account and one Vc value is calculated for each population (i.e., sequences from different populations are not compared). For each of the comparison types listed above, the respective fixation indices are calculated using the same grouping constraints. These fixation indices represent a measure of population differentiation due to genetic structure (i.e., gene flow isolation) with a value closer to 1 representing greater isolation and therefore structure.
Population Genetic Structure Inference by Population Pairwise Distance
To test for genetic dissimilarity between populations, population pairwise genetic distances were also calculated in WINARL in the form of pairwise ϕst values. A null distribution of pairwise ϕst under the hypothesis of no difference between the populations is obtained by permuting haplotypes between populations as part of WINARL's “compute pairwise difference” function. The outputted probability values represent the proportion of permutations leading to a ϕst value larger or equal to the observed one (Excoffier and Lischer, 2010). Whilst one psbAncr sequence per sample was used in the pairwise analyses, further considerations were taken in the appropriate use of the nrDNA sequences. Whilst not a traditional genetic marker used in population biology, the nrDNA's heritability and complex character states (in the form of multiple intagenomic variants due to the nrDNA's multicopy character) enable its use as such a marker (Sunnucks, 2000). In fact, given that approximations to ecologically relevant species level descriptions in Symbiodinium are commonly made according to the sequence and predominance of intragenomic variants of the nrDNA (LaJeunesse et al., 2010; Arif et al., 2014), quantifying dissimilarity between individuals and populations according to these intragenomic abundances is likely an effective means of identifying ecologically significant units. Thus, to ensure the consideration of all available nrDNA intragenomic variants and their frequencies within individual corals and populations each sequence was treated as an individual rather than creating a single “inclusivity consensus sequence” (Barshis et al., 2010) by incorporating IUPAC ambiguity nucleotides representative of polymorphisms detected within clone libraries into the consensus sequence from that individual; a method that cannot take into consideration the frequency of polymorphism-containing intragenomic variants as previously discussed by Barshis et al. (2010). Additionally, the treatment of intragenomic variants as individuals enables the incorporation of samples that are represented by a considerable number of clones but from a low number of individuals (e.g., Muscat site; only one sample with 14 nrDNA clones). Whilst such a low sampling is not ideal, further sampling is limited by the rarity of S. thermophilum group external to the PAG. The single Muscat sample is potentially highly informative given its rarity and the considerable distance to its next nearest sampling site (Fujairah 363 km). As such Muscat was included in the pairwise ϕst analyses using the nrDNA marker but excluded using the psbAncr marker due to the direct sequencing approach used (i.e., returning only a single sequence). Pairwise differences were considered significant at P < 0.05.
To test for significant correlation between distances and pairwise genetic dissimilarities between populations a Mantel test (test of correlation between two matrices) was additionally calculated using WINARL (the distance matrix may be found in Table S3).
Creation of Maximum Parsimony Networks
Maximum parsimony networks were created using the ITS2 and the complete ITS1-5.8S-ITS2 region of the nrDNA and the partial psbAncr using TCS (Clement et al., 2000). For the nrDNA networks, sequences with frequency of 1 were not plotted. TCSbu (Múrias dos Santos et al., 2015) was used to aesthetically arrange the network before further manual manipulation for aesthetic purposes only. To account for extreme variability in the psbAncr, sequences were clustered to a distance of 0.04 (empirically determined to cluster groups of sequences separated by large mutational distances) using the cluster command and average neighbour algorithm in MOTHUR 1.36.1 (Schloss et al., 2009).
Script Access
Access to R and PYTHON scripts used in this study may be found in Code S1 in Supplementary Material.
Results
Assessment of Host-Effect on Symbiodinium Sequence
To test for an effect of host species on Symbiodinium sequence recovered, a χ2 analysis was conducted to assess whether sequences were distributed independent of host species amongst three k-means clustered groupings on a 2D plot of sequence pairwise distances. A non-significant interaction was found between species and cluster [ = 5.94, p > 0.05] for the nrDNA regions. In contrast, a significant interaction between species and cluster was identified [ = 21.99, p < 0.05] for the psbAncr marker. However, 80.4% of the divergence from expected was attributable to a single species, P. harrisoni, with a single cluster representing 55.9% of this variance.
Population Genetic Structure Inference
To determine into which population groupings (internal or external) the Musandam sites were best allocated to maximise between group variance, multiple combinations were assessed. Allocation of the Musandam West and Musandam East populations to the internal and external groups, respectively, were supported by the highest “among group” (Va and ϕct) and lowest “among population within group” (Vb and ϕsc) variances and fixation indices (Va = 11%, 29%, ϕct = 0.108, 0.294; Vb = 3%, 7%, ϕsc = 0.039, 0.097; nrDNA and psbAncr, respectively). Pairwise ϕst analysis of the 9 populations corroborated the strong genetic dissimilarity between the external (ME, FUJ, and MUC; Table 1) and internal (all other populations) populations in both genetic markers.
For both markers, “among individuals within populations” (population = site) accounted for the majority of variance (86 and 63% for the nrDNA and psbAncr, respectively) whilst relatively low “among populations/within groups” (3 and 7%) and relatively high “among group” (11 and 29%; group = internal or external) variances supported the groupings of internal and external populations as characterised by the associated high and low fixation indices (ϕct = 0.108, 0.294; ϕsc = 0.039, 0.097). Inclusion of MW within the external grouping did not increase “among group” variance (5 vs. 11 and 9 vs. 29% for the nrDNA and psbAncr markers, respectively).
Despite the well-supported genetic disparity between internal and external populations and relatively low ϕsc indices, some populations within each group were found to have significant genetic disparity (Table 1) from each other. Within the external grouping, disparity was seen amongst the majority of sites. Within the internal grouping, MW showed disparity to all populations with the psbAncr marker but to only two in the nrDNA marker whilst DAL showed complete disparity to all sites with the psbAncr and SAD demonstrated dissimilarity to neighbouring DAL, RAG, and UMM with the nrDNA marker.
Mantel tests for both genetic markers recovered insignificant P (rand. > = obs.) values >> than 0.05; 0.222 and 0.219, nrDNA and psbAncr, respectively.
The maximum parsimony networks for both markers resolved phylogenetic groupings that corresponded well to the pairwise ϕst matrices and population groupings (Figures 2, 3). Within the nrDNA network the external sequences shared no haplotypes with internal sequences except for sequences containing the 8bp S. thermo.-indel mutation (Figure 3A). In addition, the strong genetic disparity between the DAL population and all other populations is exemplified by a monophyletic grouping of approximately half of the DAL sequences in the psbAncr phylogeny. MW sequences grouped exclusively with high frequency haplotypes comprising internal and external sequences within the nrDNA phylogeny and yet with the psbAncr sequences resolved at considerable distance from the main internal grouping, although some haplotypes were shared.
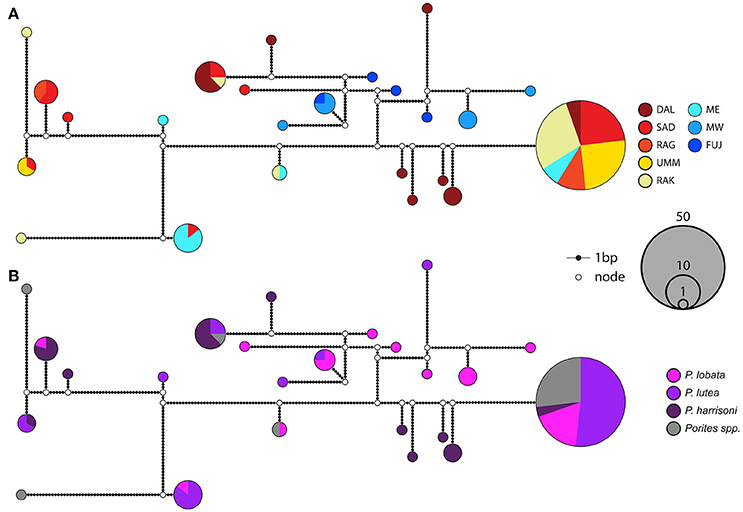
Figure 2. Maximum parsimony network using the psbAncr genetic marker. Populated nodes are representative sequences of operational taxonomic units (OTUs) created by clustering at a distance of 0.04. Nodes are colored according to both site (A) and host species (B).
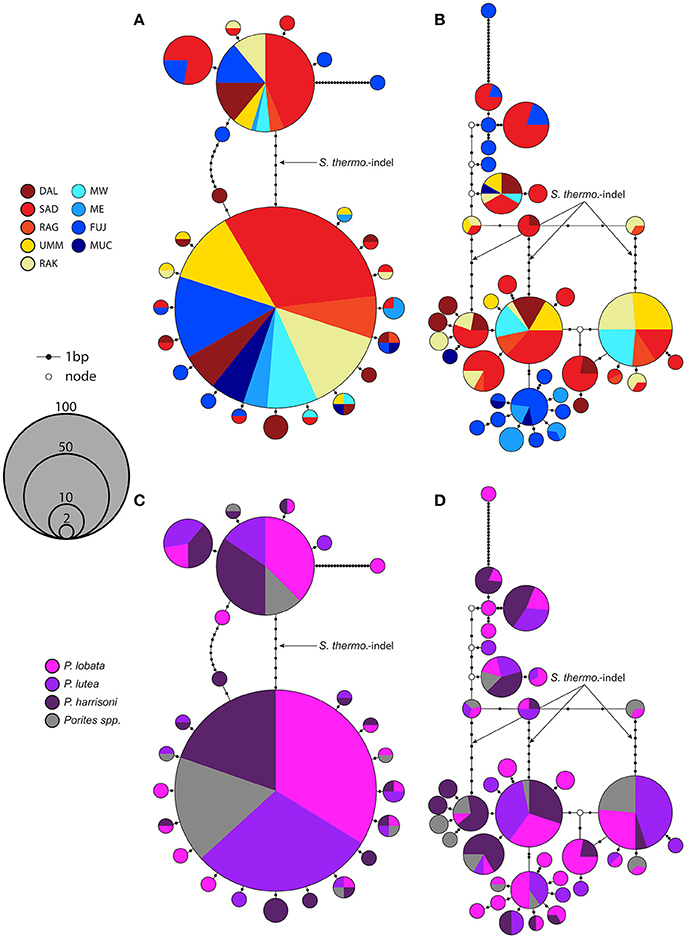
Figure 3. Maximum parsimony networks using the nrDNA. Populated nodes are representative of nrDNA sequences of either the ITS2 region only (A + C) or the complete ITS1-5.8S-ITS2 region (B + D). Nodes supported by only a single sequence are not displayed. Nodes are colored by site (A + B) and host-species (C + D). The link representing the 8 bp S. thermo.-indel is annotated.
Maximum parsimony networks of the nrDNA regions were clearly divided into two groupings; those with and those without the 8bp S. thermo.-indel insert (Figure 3). The inclusion of the ITS1 and 5.8S regions of the nrDNA greatly improved phylogenetic resolution within the S. thermophilum group samples evidenced by the further separation of a single large haplotype in the ITS2 only network into 6 haplotypes with further minor satellite haplotypes in the combined ITS1-5.8S-ITS2 analysis (Figure 3).
Discussion
Host-Effect on Symbiodinium Sequence
To assess whether any signature of genetic structure found in this study might be an artefact of host species distribution due to different species of Porites hosting different Symbiodinium types, cluster-based correlation analysis between Symbiodinium sequence recovered and host species was conducted. Whilst a significant result of the psbAncr chi-squared test suggests a significant interaction between species and cluster for this marker it should be noted that the majority of the divergence was driven by the lower than expected abundance of P. harrisoni in the most populace cluster (55.9% of divergence). The large difference between the expected and observed values in this cluster is likely an artefact caused by the high sampling frequency of P. lutea (raising the expected value in the cluster in question) and the smaller number of more western sites at which P. harrisoni was sampled (lowering the recovered genetic diversity for P. harrisoni; P. harrisoni sampled at 3 vs., 6 and 5 sites for P. lutea and P. lobata, respectively; Figure S2) rather than a true species effect of P. harrisoni on sequence recovered. Given the likely artefactual cause of this significant result for the psbAncr marker and the lack of significant correlation between coral host and sequence for the nrDNA marker, Symbiodinium sequences and any associated genetic structure identified in this study will be discussed independent of host-species.
Genetic Diversity of the S. thermophilum Group
S. thermophilum sensu stricto was originally described from Porites spp. samples collected from the DAL, SAD and UMM regions of the southern PAG (Hume et al., 2015). Since this initial description considerable additional genetic diversity has been uncovered from samples collected outside of Southern PAG. This increase in genetic diversity has led to the use of the term S. thermophilum group due to the fact that such diversity may be indicative of additional species.
Whilst all of the samples used in the initial S. thermophilum description resolved with a single dominant ITS2 type C3 sequence, a range of psbAncr haplotypes were recovered (Hume et al., 2015). The majority of these psbAncr sequences (17/22) cluster within the largest OTU of the psbAncr maximum parsimony network created in this study with the remaining 5 clustering amongst the monophyletic DAL grouping also from this study (Figure 2). The majority of internal samples (from DAL, SAD, RAG, UMM, RAK, and MW) analysed in this study also cluster within the same psbAncr OTU and display a limited diversity of closely related intragenomic ITS1-5.8S-ITS2 variants that share only 3 out of 38 haplotypes with external samples (ME, FUJ, MUC; Figures 2, 3). Taken together, these findings suggest that the majority of S. thermophilum group symbionts found within the southern PAG are S. thermophilum sensu stricto but that additional S. thermophilum group diversity is also present, such as the PAG Acropora spp.—specific symbiont (C3V1; KM487747) identified in Hume et al. (2015). In contrast, whilst this study's external S. thermophilum group populations also return ITS2 type C3 and S. thermo-indel containing sequences characteristic of the S. thermophilum group, their lack of shared haplotypes with internal PAG symbionts (both non-S. thermo-indel-containing nrDNA and psbAncr sequences) suggest that they are either a genetically distinct subpopulation of S. thermophilum or represent a separate species. Indeed, the quantification of genetic distances between members within the group by Hume et al. (2016) as comparable to those observed between other Symbiodinium types estimated to have diverged more than 10 Mya would also support the likely existence of multiple subpopulations within the group.
Genetic Resolution of S. thermophilum Sub-taxa
The consideration of nrDNA intragenomic sequence variants in Symbiodinium ITS2 phylogenetics has enabled the further resolution of types originally designated only according to their predominant ITS2 sequence, for example the distinction between D1, D1-4 (S. trenchii) and D1-4-6 (LaJeunesse et al., 2010). Whilst the consideration of such intragenomic variants has enabled the distinction of S. thermophilum group symbionts from other ITS2 type C3 symbionts, neither the presence nor frequency of specific intragenomic variants has yet been able to resolve different populations of S. thermophilum group symbionts to the same extent as the inclusion of additional regions of the nrDNA has in this study (Figure 3A vs. Figure 3B). This inclusion of the 5.8S and ITS1 region has effectively brought the resolution of the rDNA marker closer to that of the psbAncr marker, a marker generally considered hypervariable (Thornhill et al., 2014). However, by sequencing nrDNA ITS2 PCR amplicons to a greater depth using NGS technologies (sensu (Arif et al., 2014), Smith et al., 2017) fine scale assessment of differences in the presence and proportion of intragenomic sequence variants will likely result in a significant increase in the resolving power of this marker.
Identification of S. thermophilum Sub-populations
Whilst S. thermophilum group symbionts have been documented throughout reefs on the south coast of the PAG and to a lesser extent externally in the Strait of Hormuz and Gulf of Oman (D'Angelo et al., 2015) it is unclear whether the diversity of physical environments over which they are found has led to a range of genetically disparate sub-populations. Here we further resolve the previously described biogeographical boundary to coral holobiont distribution found in the Strait of Hormuz and identify genetically disparate populations either side of it.
D'Angelo et al. (2015) first documented the host-symbiont biogeographical boundary at the Strait of Hormuz through noting a gradual decrease in the predominance of S. thermophilum group associations moving from the western Strait of Hormuz out into the Gulf of Oman. The significant effect of this boundary upon a large number of taxa is qualified by the limited diversity found within the PAG compared to the wider Indo-Pacific (Coles, 2003; D'Angelo et al., 2015). Given the large among group variance between internal and external populations, location either side of the boundary, rather than distance between study sites, would be expected to explain genetic disparity. As such, insignificant results from the mantel test also corroborates the presence of this boundary.
The genetic structure identified in this study would suggest that the western edge of the Strait of Hormuz (i.e., site MW) may represent a specific area where the decrease in genetic diversity from the Gulf of Oman to the PAG is greatest. This is demonstrated through the significant genetic difference of MW to all outer sites in concert with an only mixed differentiation from internal sites (Table 1). In addition, the fact that the two significantly differentiated Musandam sites (i.e., MW, ME) have only ~20 km between them may point to an sudden spatial divide between the internal and external symbiont biomes in this region. Such a small distance between genetically differentiated sites could suggest that local adaptation to specific local conditions, rather than allopatric diversification due to limited connectivity, is responsible for the genetic heterogeneity observed.
Given that the surface inflow into the PAG effectively acts to buffer thermal extremes by cooling in the summer and warming in the winter, a gradient of seasonal thermal range exists that correlates with the penetrative distance of this inflow (Figure 1c). As such, coral communities within the southern and more westerly PAG experience the coolest winters and warmest summers in conjunction with the highest year round salinities (~42; Figure 1). Remotely sensed estimated upper bleaching thresholds of reefs in this southern region reflect this with a difference of more than 0.5°C between DAL and SAD (35.05 and 34.53°C, respectively; Shuail et al., 2016). The genetic disparity seen between DAL and to a lesser extent SAD with other internal sites may therefore be due to local adaptation to different thermal histories. However, further population genetic data would be required to verify this.
S. thermophilum Group Symbionts as Stress Tolerant Genetic Resources
As coral reef ecosystems become increasingly under threat so do the natural systems and human populations that rely upon them (Hughes et al., 2003). Coupled with the advanced state of reef degradation these ecosystems are therefore increasingly the focus of conservation efforts (Burt et al., 2014). With their extraordinary stress tolerance, coral associations of the PAG have been suggested as potential candidates to seed thermally degraded reefs in order to accelerate reef recovery and increase future stress tolerance through the integration of genetic resources (Coles and Riegl, 2013). However, mesocosm-based studies by D'Angelo et al. (2015) demonstrated that whilst some S. thermophilum-associated corals from the PAG were able to survive in waters of normal oceanic salinity, their growth was reduced and their exceptional thermal tolerance lost. As such, due to the trade-offs associated with the local adaptations to the higher salinity of the PAG, corals from this waterbody appear to have a small potential to colonise reefs elsewhere that are characterised by a more oceanic salinity.
However, the presence of genetically distinct sub populations of the S. thermophilum group identified in this study would suggest that a considerable pool of group member symbionts upon which natural selection could act exist. Specifically, group members in the Gulf of Oman surviving in waters characterised as thermally challenging yet with oceanic-like salinities, would appear to be model candidates to act as natural sources of stress tolerant associations to Indo-Pacific reefs. The modification of symbiont associations by corals to those of a more stress tolerant type has been demonstrated (Ortiz et al., 2013; Silverstein et al., 2015) and such mechanisms could allow for the ecological proliferation and integration of S. thermophilum group symbionts amongst reefs, especially considering the broad range of cnidarians S. thermophilum group symbionts have been identified in association with (more than 40 genera; Hume et al., 2016). Indeed, the spread of a stress-tolerant coral generalist Symbiodinium trenchii throughout Caribbean reefs in a period of possibly only several decades has recently been documented and would imply that such an ecological proliferation could occur in a time frame relevant to the slowing of further reef degradation due to future warming (Pettay et al., 2015). Nevertheless, the almost undetectable abundance of S. thermophilum group symbionts external to the PAG (found in ~4% of corals in the Red Sea and Gulf of Oman, often at low within coral abundances; Hume et al., 2016) would suggest that S. thermophilum group associations are not competitive given current environmental conditions and extant symbiont populations.
As coral reef ecosystems continue to be degraded by increasingly stressful conditions, and typical associations begin to breakdown, stress tolerant S. thermophilum group associations may become more competitive conferring increased tolerances to reefs in future conditions (Pettay et al., 2015). However, many other biotic and abiotic factors that will influence whether these symbionts will be able to acquire a niche and thus proliferate must be taken into account. In particular, trade-offs such as reduced calcification rates that can be associated with thermal tolerance must also be considered (Jones and Berkelmans, 2010). The ability of S. thermophilum group associations to be able to confer increased tolerances will be dependent on the maximal retention of genotypes within the group from which better adapted and resilient symbionts may gain predominance and enable reef adaptation to warming waters. As such, reduction of any reef stressors other than rising SSTs that may negatively affect this biodiversity must be prioritised in conservation strategies (D'Angelo and Wiedenmann, 2014).
Data Accessibility
Genbank accession numbers for psbAncr sequences analysed as part of this study are listed in Table S1. A multiple sequence alignment of the ITS1-5.8S-ITS2 nrDNA sequences used in this study has been submitted to the Dryad Repository. MOTHUR, R, and PYTHON scripts used in this study are available at https://github.com/didillysquat/PAG-Code.
Author Contributions
BH: Conceived and conducted the analysis; BH, CD, and JW: Planned and conceived the project; BH: Wrote the initial report; BH, CD, and JW: Revised final versions of the report. JB: Sponsored fieldwork to collect samples and along with JW contributed resources to the analysis.
Funding
The study was funded by NERC (NE/K00641X/1 to JW) and by the European Research Council under the European Union's Seventh Framework Programme (FP/2007-2013)/ERC Grant Agreement no. 311179 to JW.
Conflict of Interest Statement
The authors declare that the research was conducted in the absence of any commercial or financial relationships that could be construed as a potential conflict of interest.
Acknowledgments
We thank the NYU Abu Dhabi Institute for supporting the 2012/2013 field workshops during which samples for this study were collected; Dawood Shuail and Matthew Cobain (University of Southampton) for assistance in obtaining remote sensing data and assistance with statistical analyses, respectively; and Ed Smith (NYUAD) for initial collection of Muscat samples. Our appreciation is extended to A Al-Hemeri of the UAE Federal Environment Agency and A Al-Cibahy of the Environment Agency of Abu Dhabi for provision of CITES export permits (no. 09FEA555). We also thank Tropical Marine Centre (London) and Tropic Marin (Wartenberg) for sponsoring the NOCS Coral Reef Laboratory.
Supplementary Material
The Supplementary Material for this article can be found online at: https://www.frontiersin.org/articles/10.3389/fmars.2018.00138/full#supplementary-material
References
Arif, C., Daniels, C., Bayer, T., Banguera-Hinestroza, E., Barbrook, A., Howe, C. J., et al. (2014). Assessing Symbiodinium diversity in scleractinian corals via next-generation sequencing-based genotyping of the ITS2 rDNA region. Mol. Ecol. 23, 4418–4433. doi: 10.1111/mec.12869
Baird, A. H., Guest, J. R., and Willis, B. L. (2009). Systematic and biogeographical patterns in the reproductive biology of scleractinian corals. Annu. Rev. Ecol. Evol. Syst. 40, 551–571. doi: 10.1146/annurev.ecolsys.110308.120220
Baker, A. C., Starger, C. J., McClanahan, T. R., and Glynn, P. W. (2004). Coral reefs: corals' adaptive response to climate change. Nature 430, 741–741. doi: 10.1038/430741a
Barshis, D. J., Stillman, J. H., Gates, R. D., Toonen, R. J., Smith, L. W., and Birkeland, C. (2010). Protein expression and genetic structure of the coral Porites lobata in an environmentally extreme Samoan back reef: does host genotype limit phenotypic plasticity? Mol. Ecol. 19, 1705–1720. doi: 10.1111/j.1365-294XX.2010.04574.x
Bauman, A. G., Feary, D. A., Heron, S. F., Pratchett, M. S., and Burt, J. A. (2013). Multiple environmental factors influence the spatial distribution and structure of reef communities in the northeastern Arabian Peninsula. Mar. Pollut. Bull. 72, 302–312. doi: 10.1016/j.marpolbul.2012.10.013
Burt, J., Van Lavieren, H., and Feary, D. A. (2014). Persian Gulf reefs an important asset for climate science in urgent need of protection. Ocean Challenge 20, 49–56. Available online at: http://www.challenger-society.org.uk/oceanchallenge/OC_Vol20.pdf
Clement, M., Posada, D., and Crandall, K. A. (2000). TCS: a computer program to estimate gene genealogies. Mol. Ecol. 9, 1657–1659. doi: 10.1046/j.1365-294xx.2000.01020.x
Coles, S. L. (2003). Coral species diversity and environmental factors in the Arabian Gulf and the Gulf of Oman: a comparison to the Indo-Pacific region. Atoll Res. Bull. 507, 1–19. doi: 10.5479/si.00775630.507.1
Coles, S. L., and Riegl, B. M. (2013). Thermal tolerances of reef corals in the Gulf: a review of the potential for increasing coral survival and adaptation to climate change through assisted translocation. Mar. Pollut. Bull. 72, 323–332. doi: 10.1016/j.marpolbul.2012.09.006
D'Angelo, C., Hume, B. C., Burt, J., Smith, E. G., Achterberg, E. P., and Wiedenmann, J. (2015). Local adaptation constrains the distribution potential of heat-tolerant Symbiodinium from the Persian/Arabian Gulf. ISME J. 9, 2551–2560. doi: 10.1038/ismej.2015.80
D'Angelo, C., and Wiedenmann, J. (2014). Impacts of nutrient enrichment on coral reefs: new perspectives and implications for coastal management and reef survival. Curr. Opin. Environ. Sustainabil. 7, 82–93. doi: 10.1016/j.cosust.2013.11.029
Excoffier, L., and Lischer, H. E. (2010). Arlequin suite ver 3.5: a new series of programs to perform population genetics analyses under Linux and Windows. Mol. Ecol. Resour. 10, 564–567. doi: 10.1111/j.1755-0998.2010.02847.x
Forsman, Z. H., Barshis, D. J., Hunter, C. L., and Toonen, R. J. (2009). Shape-shifting corals: molecular markers show morphology is evolutionarily plastic in Porites. BMC Evol. Biol. 9:45. doi: 10.1186/1471-2148-9-45
Hughes, T. P., Baird, A. H., Bellwood, D. R., Card, M., Connolly, S. R., Folke, C., et al. (2003). Climate change, human impacts, and the resilience of coral reefs. Science 301, 929–933. doi: 10.1126/science.1085046
Hume, B. C., D'Angelo, C., Smith, E. G., Stevens, J. R., Burt, J., and Wiedenmann, J. (2015). Symbiodinium thermophilum sp nov., a thermotolerant symbiotic alga prevalent in corals of the world's hottest sea, the Persian/Arabian Gulf. Sci. Rep. 5:8562. doi: 10.1038/srep08562
Hume, B. C., Voolstra, C. R., Arif, C., D'Angelo, C., Burt, J. A., Eyal, G., et al. (2016). Ancestral genetic diversity associated with the rapid spread of stress-tolerant coral symbionts in response to Holocene climate change. Proc. Natl. Acad. Sci. U.S.A. 113, 4416–4421. doi: 10.1073/pnas.1601910113
Hume, B., D'Angelo, C., Burt, J., Baker, A. C., Riegl, B., and Wiedenmann, J. (2013). Corals from the Persian/Arabian Gulf as models for thermotolerant reef-builders: prevalence of clade C3 Symbiodinium, host fluorescence and ex situ temperature tolerance. Mar. Pollut. Bull. 72, 313–322. doi: 10.1016/j.marpolbul.2012.11.032
Jones, A., and Berkelmans, R. (2010). Potential costs of acclimatization to a warmer climate: growth of a reef coral with heat tolerant vs. sensitive symbiont types. PLoS ONE 5:e10437. doi: 10.1371/journal.pone.0010437
LaJeunesse, T. C., Pettay, D. T., Sampayo, E. M., Phongsuwan, N., Brown, B., Obura, D. O., et al. (2010). Long-standing environmental conditions, geographic isolation and host-symbiont specificity influence the relative ecological dominance and genetic diversification of coral endosymbionts in the genus Symbiodinium. J. Biogeogr. 37, 785–800. doi: 10.1111/j.1365-2699.2010.02273.x
LaJeunesse, T. C., Wham, D. C., Pettay, D. T., Parkinson, J. E., Keshavmurthy, S., and Chen, C. A. (2014). Ecologically differentiated stress-tolerant endosymbionts in the dinoflagellate genus Symbiodinium (Dinophyceae) Clade D are different species. Phycologia 53, 305–319. doi: 10.2216/13-186.1
Logan, C. A., Dunne, J. P., Eakin, C. M., and Donner, S. D. (2014). Incorporating adaptive responses into future projections of coral bleaching. Glob. Chang. Biol. 20, 125–139. doi: 10.1111/gcb.12390
Múrias dos Santos, A., Cabezas, M. P., Tavares, A. I., Xavier, R., and Branco, M. (2015). tcsBU: a tool to extend TCS network layout and visualization. Bioinformatics 32, 627–628. doi: 10.1093/bioinformatics/btv636
Ortiz, J. C., Gonzalez-Rivero, M., and Mumby, P. J. (2013). Can a thermally tolerant symbiont improve the future of Caribbean coral reefs? Glob. Chang. Biol. 19, 273–281. doi: 10.1111/gcb.12027
Pettay, D. T., Wham, D. C., Smith, R. T., Iglesias-Prieto, R., and LaJeunesse, T. C. (2015). Microbial invasion of the Caribbean by an Indo-Pacific coral zooxanthella. Proc. Natl. Acad. Sci. U.S.A. 112, 7513–7518. doi: 10.1073/pnas.1502283112
Purkis, S. J., Rowlands, G., Riegl, B., and Renaud, P. (2010). The paradox of tropical karst morphology in the coral reefs of the arid Middle East. Geology 38, 227–230. doi: 10.1130/G30710.1
Schloss, P. D., Westcott, S. L., Ryabin, T., Hall, J. R., Hartmann, M., Hollister, E. B., et al. (2009). Introducing mothur: open-source, platform-independent, community-supported software for describing and comparing microbial communities. Appl. Environ. Microbiol. 75, 7537–7541. doi: 10.1128/AEM.01541-09
Shuail, D., Wiedenmann, J., D'Angelo, C., Baird, A. H., Pratchett, M. S., Riegl, B., et al. (2016). Local bleaching thresholds established by remote sensing techniques vary among reefs with deviating bleaching patterns during the 2012 event in the Arabian/Persian Gulf. Mar. Pollut. Bull. 105, 654–659. doi: 10.1016/j.marpolbul.2016.03.001
Silverstein, R. N., Cunning, R., and Baker, A. C. (2015). Change in algal symbiont communities after bleaching, not prior heat exposure, increases heat tolerance of reef corals. Glob. Chang. Biol. 21, 236–249. doi: 10.1111/gcb.12706
Smith, E. G., Ketchum, R. N., and Burt, J. A. (2017). Host specificity of Symbiodinium variants revealed by an ITS2 metahaplotype approach. ISME J. 11, 1500–1503. doi: 10.1038/ismej.2016.206
Sunnucks, P. (2000). Efficient genetic markers for population biology. Trends Ecol. Evol. 15, 199–203. doi: 10.1016/S0169-5347(00)01825-5
Tamura, K., Stecher, G., Peterson, D., Filipski, A., and Kumar, S. (2013). MEGA6: Molecular Evolutionary Genetics Analysis Version 6.0. Mol. Biol. Evol. 30, 2725–2729. doi: 10.1093/molbev/mst197
Thornhill, D. J., Lajeunesse, T. C., and Santos, S. R. (2007). Measuring rDNA diversity in eukaryotic microbial systems: how intragenomic variation, pseudogenes, and PCR artifacts confound biodiversity estimates. Mol. Ecol. 16, 5326–5340. doi: 10.1111/j.1365-294XX.2007.03576.x
Thornhill, D. J., Lewis, A. M., Wham, D. C., and LaJeunesse, T. C. (2014). Host-specialist lineages dominate the adaptive radiation of reef coral endosymbionts. Evolution 68, 352–367. doi: 10.1111/evo.12270
Veron, J. E. (2000). Corals of the World, Vol. 1–3. Townsville, QLD: Australian Institute of Marine Science.
Keywords: adaptation, biogeography, climate change, coral reefs, ITS2, Persian/Arabian Gulf, salinity, thermal tolerance
Citation: Hume BCC, D'Angelo C, Burt JA and Wiedenmann J (2018) Fine-Scale Biogeographical Boundary Delineation and Sub-population Resolution in the Symbiodinium thermophilum Coral Symbiont Group From the Persian/Arabian Gulf and Gulf of Oman. Front. Mar. Sci. 5:138. doi: 10.3389/fmars.2018.00138
Received: 19 December 2017; Accepted: 06 April 2018;
Published: 24 April 2018.
Edited by:
Emma Camp, University of Technology Sydney, AustraliaReviewed by:
Zac H. Forsman, University of Hawaii at Manoa, United StatesAldo Cróquer, Simón Bolívar University, Venezuela
Copyright © 2018 Hume, D'Angelo, Burt and Wiedenmann. This is an open-access article distributed under the terms of the Creative Commons Attribution License (CC BY). The use, distribution or reproduction in other forums is permitted, provided the original author(s) and the copyright owner are credited and that the original publication in this journal is cited, in accordance with accepted academic practice. No use, distribution or reproduction is permitted which does not comply with these terms.
*Correspondence: Benjamin C. C. Hume, benjamin.hume@kaust.edu.sa