- 1International Research Institute of Stavanger-Environment, Randaberg, Norway
- 2CNR- Istituto di Scienze Marine, Largo Fiera della Pesca, Ancona, Italy
- 3Coop. “Mare Ricerca”, Ancona, Italy
Plastic micro litter represents an emerging contaminant as well as a multiple stress agent in aquatic environments. Microplastics are found even in the remote areas of the world. Together with their occurrence in all environmental compartments, there is a growing concern about their potential to adsorb pollutants co-occurring in the environment. At present, little is known about this source of exposure for aquatic organisms in the benthic environment. Exposure conditions were set up to mimick the contribution of microplastics through different exposure routes. Potential biological effects resulting from these exposures were investigated in the model organism Hediste diversicolor, an annelid worm. Cellular effects including alterations of immunological responses, lysosomal compartment changes, mitochondrial activity, oxyradical production and onset of genotoxicity were assessed in coelomocytes while temporary and permanent effects of oxidative stress were also performed at tissue level. In this study polyvinylchloride (PVC) microparticles were shown to adsorb benzo(a)pyrene with a time and dose-dependent relationship. The elevated bioavailability of the model pollutant after ingestion induced a clear pattern of biological responses. Toxicity mainly targeted impairment of cellular functioning and genotoxicity in H. diversicolor coelomocytes, while permanent effects of oxidative stress were observed at tissue level. Coelomocytes responded fast and with a higher degree of sensitivity to the adverse stimuli. The results showed that microplastic particles in sediments may play a significant role as vectors for organic pollutants. The highest adverse responses were observed in those H. diversicolor exposed to sediments spiked with PVC particles pre-incubated with B[a]P when compared against sediments spiked with B[a]P and plastic microparticles separately.
Introduction
Along with environmental stressors such as global warming, ocean acidification, and habitat destruction, pollution causes undesirable changes in marine ecosystems. In particular, plastic particles accidentally or deliberately released as a result of anthropogenic activities represent a growing environmental concern for aquatic environments. Marine debris, particularly the fraction composed of plastic fragments at micrometric size, is considered a multiple stressor in aquatic habitats. Firstly it acts as physical stressor congesting digestive traits and ducts hampering the functioning of key organs i.e., gill, stomach, liver, and kidney in vertebrate and invertebrate marine species (Wright et al., 2013; Kühn et al., 2015; Clark et al., 2016; Pellini et al., 2018). Secondly, micrometric scaled plastic fragments can act as chemical reservoirs and potential vectors for the widespread dispersal of hydrophobic pollutants co-occurring in the aquatic environment. Polymers with densities higher than seawater (e.g., PVC) tend to sink and accumulate in seafloor sediments, making organisms within the benthic community more vulnerable to organic pollutants, as they may be adsorbed from both the water column and the sediment. Ingestion of particles has been documented in vertebrate and invertebrate marine organisms (Gregory, 2009; Davison and Asch, 2011; Carson, 2013; Hall et al., 2015). After the ingestion, the ingestion processes, dominated shifts in pH, temperature and redox conditions, microplastic particles can potentially release the adsorbed environmental pollutants therefore enhancing both their bioavailability and biological adverse effects to biota. This route of exposure for aquatic organisms, the so called “Trojan horse” effect, remains under-investigated with limited data concerning such phenomena (Syberg et al., 2015; Paul-Pont et al., 2016; Gaspar, 2017).
In our study the possible contribution of plastic particles toward increasing dispersion, bioavailability and associated adverse biological effects of an organic pollutant molecule model benzo(a)pyrene (B[a]P) was investigated in a zoobenthic model organism, the ragworm Hediste diversicolor. B[a]P was chosen as a representative of the polycyclic aromatic hydrocarbon (PAH) class of compounds. PAHs are widely distributed toxic compounds with, in some cases, carcinogenic, mutagenic, and teratogenic effects, as is the case for B[a]P (IARC, 1987). In aquatic environments, most PAHs primarily accumulate in the sediment as a result of their chemical and physical characteristics. Bottom-dwelling deposit-feeding organisms such as polychaetes are potentially exposed to PAHs, both directly through dermal contact with interstitial water or particulate matter and indirectly through the gut, from food and particle ingestion (Jørgensen et al., 2008). Furthermore, polychaetes can be also exposed by the water phase and during high tide, they can be exposed by the water compartment. For this reason, they are a good candidate species for toxicological studies addressing different exposure routes.
A number of different exposure scenarios were set up mimicking the rising occurrence of plastic micro litter in an environment already subjected to organic pollution contamination. An extended suite of biomarkers was selected to compare the biological responses observed both in cells extracted from the coelomic fluid and at tissue level and to unveil microplastics driven toxicity mechanisms at organism, cellular and sub-cellular level.On coelomic fluid cells the phagocytosis assay (PhC) and the lysozyme activity responses (Lz) were measured to investigate possible impairments of the immune system. On the other hand, the mitochondrial activity (MtO) and the lysosomal membrane stability (LMS) were estimated to assess general information about cellular functioning; while the oxyradical production assay (ROx) was estimated as index of oxidative stress. Finally DNA fragmentation and micronuclei frequency (Mn) were assessed to investigate genotoxic effects in exposed organisms. In the meantime, lipid peroxidation (LPO) and the activity of catalase (CAT) were investigated at tissue level as marker of oxidative stress in treated organisms. Obtained biological responses were compared with as the body burden accumulation of B[a]P.
Materials and Methods
Chemicals and Reagents
All chemicals were of analytical grade and were used as received without any further purification. Methanol (purity: < 99.5%), B[a]P (purity: < 99.5%) and Hank's artificial seawater salts mixture was procured from Sigma-Aldrich. Polyvinyl chloride (PVC) powder of 250.0 ± 2.5 μm size was obtained from Goodfellow Ltd (Cambridge, UK). Reagents for artificial sea water (ASW) preparation were of purity > 99.5% from Sigma Aldrich. Bi-distilled water used in this study was obtained from a MilliQ apparatus (Millipore, Milan, Italy) according to the manufacturer's guidelines.
Test Design
Laboratory assays followed the ASTM guidelines (ASTM, 2013) for conducting sediment tests with annelids with some minor adaptations. A set of five replicate test aquaria (10 l glass beakers) each containing eight organisms were used per treatment. Ragworms were first put individually in beakersto avoid possible cannibalism and then transferred on aquaria for the exposure test,., Each aquaria contained some glass tubes with 1 cm diameter, placed in the sediment surface as possible temporary shelter for the organisms. Approximately 3 kg of sediments (≈ 8-cm layer of sediment) were carefully placed, at the bottom of the beakers. Artificial seawater medium (ASW) of 34 g l−1 salinity (ASTM, 2013) was added yielding a sediment: overlying water depth ratio of 1:3 (w:v). Organisms were exposed at 20.0 ± 1.0°C and under a 16 h light: 8 h dark cycle. Food was added during the assays at 1% of body weight and 2/3 of the water was carefully siphoned out from the water surface and replaced every week. Water parameters (salinity, pH, dissolved oxygen) were monitored throughout the exposure period.
A series a chronic 28 days experiments were carried out by exposing H. diversicolor to uncontaminated sediments (C), sediments spiked with B[a]P (S-B[a]P), sediments spiked only with virgin PVC at 200 particles/kg of sediment (LC-MPS) and 2,000 particles/kg (HC-MPS) as well as similar concentrations for B[a]P spiked microplastic particles (B[a]P-LC; B[a]P-HC). The lower bead concentration was set to match with environmentally realistic levels (Claessens et al., 2013; Hanvey et al., 2017), the 10 times higher level was chosen to address potential acute exposure effects and does not represent environmental conditions.
Ragworm Collection
Specimens of H. diversicolor were purchased from a bait dealer (Lescachepesca.eu, Italy). Only individuals in good condition and similar size-class length (12.0 ± 2.0 cm) were selected for testing. Organisms were left for 7 days in ASW and in the dark at 20.0 ± 2.0°C to acclimate and depurate. During the acclimatization period individuals were fed ad libitum every 2–3 days with commercial fish food (48.6% protein and 7.7% fat). 5 gr of food was extracted in triplicate and analyzed for microplastic content. No plastic particles were detected in the analyzed samples.
Sediment Collection and Characterization
A total of 100 kg of natural sediments were collected using a stainless-steel box corer in October 2013 from an off-shore site (coordinates) considered distant from major anthropogenic sources of hydrocarbons and micro plastic particles. Collected material was used to prepare the control and spiked sediments. Sediments were homogenized and native animals removed before taking a subsample to determine: salinity and organic matter content (OM, Schumacher, 2002). Particle size distribution was determined using a LS 13-320 laser particle-sizing instrument (Beckmann Coulter, Milan, Italy) and the total content of polyaromatic hydrocarbons assessed according to the methods of Durou et al. (2007). Furthermore, microplastic content and characterization, based on a density separation method according to Nuelle et al. (2014) and a detection method according to Vianello et al. (2013), was carried out. A negligible (2.0 ± 0.9 particles/kg) amount of polyethylene fragments in a range of 30–40 μm were detected ([2]). This sandy-mud sediment contained 1.5% of organic carbon, 82.4% of sand, 13.9% of clay and 3.7% of silt.
Spiking Preparation
The adsorption of B[a]P to PVC particles was performed by mixing solutions of microplastics (~10 g/L in ASW) with B[a]P at an environmentally realistic concentration of 5 μg/L (Bihari et al., 2007; Maria and Bebianno, 2011; Avio et al., 2015). The incubating solutions were kept in 50 ml glass tubes under continuous rotation within a Gallenkamp chamber for 7 days at room temperature in darkness. PVC adsorption efficiency was checked at the end of the incubation process by spinning down spiked plastic beads (5,000 g, 10 min at RT), collecting the pellet, sub sampling it three times and placing sub samples in a GF/A fiberglass filter. Samples were flushed with 5 ml of ASW and adsorbed B[a]P extracted by 10 ml of methanol (purity < 99,9%) using a microwave assisted technique. Desorbed B[a]P in methanol was preconcentrated by a Rotavapor and quantified by an Agilent 1100 series HPLC-DAD system (Figure 1—SEM). B[a]P was subsequently identified by the retention time of standard solution of CRM48743 Supelco mix.
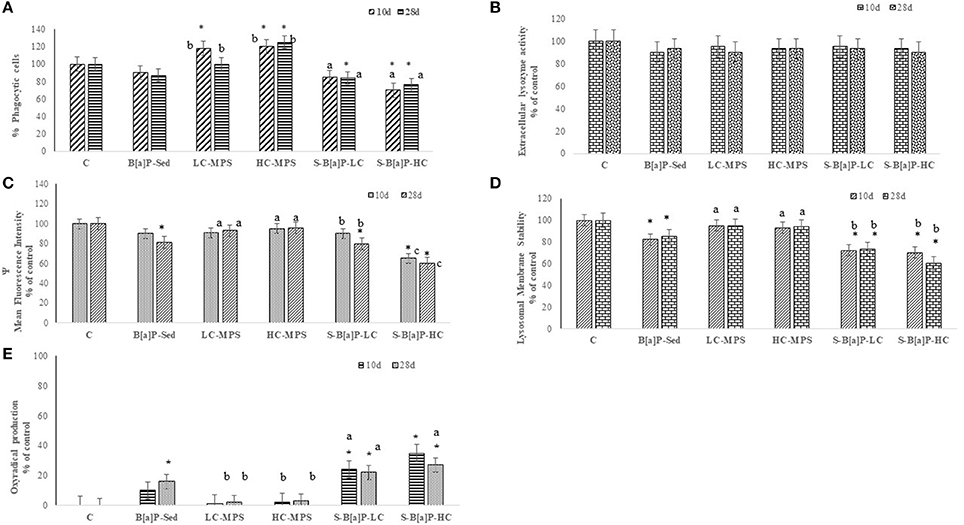
Figure 1. Biological responses in coelomocytes of H. diversicolor exposed to uncontaminated sediments (C), D sediments spiked with 1 mg/L B[a]P (S-B[a]P), sediments spiked with 200 and 2000 particles/kg virgin PVC (LC-MPS, HC-MPS) and sediments with B[a]P spiked microplastic particles (B[a]P-LC: 200 particles/kg.; B[a]P-HC: 2000 particles/kg). (A) Response of the phagocytic activity, (B) Extracellular lysozyme release, (C) mitochondrial activity, (D) Lysosomal membrane stability, (E) Oxyradical production. *p < 0.05. Data are expressed as mean values ± standard deviation, n = 6. Different letters indicate significant differences between groups of means (post-hoc comparison).
The stock of the spiked microplastic beads were stored at−20 C prior to exposure tests.
To realize S-B[a]P testing conditions, sediments were contaminated by spiking with B[a]P (>99% dissolved in acetone, HPLC-grade, Sigma-Aldrich, Italy). To insure a comparable exposing dose among all tested exposure routes, ~16 kg of fresh sediments were spiked with 80 μg of B[a]P considering a concentration of 5 μg/L previously adopted for PVC particle incubation as well as a standard rate of sediment: overlying water of 1:3 (w/v) set within the exposure tests. Spiked sediments were gently mixed with a stainless-steel spoon for 20 min and allowed to equilibrate for a week at RT in dark conditions in a well-ventilated fume hood. For the uncontaminated treatment, fresh sand was only spiked with acetone. Final B[a]P concentrations obtained with this procedure were 4.58 ± 0.51 μg kg−1 (ww) while sediments analyzed before the spiking exercise did not contain detectable levels of B[a]P (< 1,0 μg kg−1).
To realize S-B[a]P testing conditions, previously spiked sediments were equilibrated with filtered seawater for 48 h prior to the addition of microplastic beads and test organisms. In the meantime, to realize LC-MPS, HC-MPS, B[a]P-LC, and B[a]P-HC testing conditions, B[a]P spiked particles and virgin particles at the two different concentrations were added on top of each of the aquaria used for the different experimental conditions were re-equilibrated natural sediments were previously placed.
The number of particles to be added to the aquaria was calculated using the concentration of a mixture of either virgin or B[a]P spiked beads mixed with 5 ml of ASW. The working mixtures were sub-sampled 10 times, collecting 100 μl working solution, diluting it 1:1,000 (v:v) in Isoton II Diluent (Beckmann, Milan, Italy) and finally analyzing each of the subsamples by a Multisizer II Coulter Counter coupled with a 1,000 μm aperture size tube (Beckmann, Milan, Italy). Spiking solutions with concentration of 50.0 ± 6.0 beads / ml were then prepared. Beads were allowed to sediment for 3 days, mimicking the naturally occurring deposition of microplastic from the water column, prior to turning on the aeration and placement of test organisms. Aeration was provided using a 1 ml glass pipette suspended ~5 cm above the sediment surface, with test organisms added to the vessel 2 h later. While adding the organisms, aeration was stopped for 10 min to allow them to settle. A set of randomly placed glass tubes and pipettes were added to the aquaria as shelters for the organisms.
Chemical Analyses
The body burden of B[a]P in exposed organisms was estimated according to Durou et al. (2007) on three individuals collected from each replicate of each testing condition, while the accumulation of MPS was estimated on an individual per replicate of each testing condition according to a combined enzymatic and alkali driven extraction followed by a μFTIR analysis. Briefly, single individuals were incubated with 50 ml protease from Bacillus sp (P3111, Sigma, Italy) and PBS-buffer at pH 9 mixture (1:5). The sample was sonicated for 5 min and incubated for 24 h at 45°C and then filtered through a 20 μm stainless steel sieve (Setacci Giuliani Spa, Italy). The residue was washed off with MilliQ water pre-filtered through a 0,45 μm filter, collected in a clean 100 ml beaker and further treated with 50 ml Chitinase from Trichoderma (C8241, Sigma, Italy) and PBS-buffer at pH 7 mixture (1:10). The sample was sonicated for 5 min, incubated 24 h at 30°C and finally filtered through a 20 μm stainless steel sieve. The residue was transferred to a 50 ml beaker and incubated with KOH 10% 12 h at 50°C. The alkali degraded sample was then diluted 1:1 with MilliQ water and filtered through a 47 mm Anodisc filter with pore size of 0.2 μm (Whatman, Germany) and submitted to μFTIR according to Vianello et al. (2013). Four individuals collected from each testing condition involving PVC beads were analyzed.
Biological Analyses
Endpoints were measured after 10 and 28 days of exposure to the tested conditions, being the measurements after 10 days of exposure a good intermediate sampling point useful to follow up the development of the stress syndrome in the investigated organisms. Ragworms were removed from exposure and individually maintained in ASW to void gut content in preparation for MPS accumulation assessment as well as biological and chemical analyses. After ~10 h, ragworms were submitted to biological analyses or snap-frozen and stored at −40°C until chemical analyses.
Mortality was recorded as a high-level endpoint test following the exposure period. All sub lethal biological responses were conducted on four individuals per replicate in each of the treatment and sampling time.
Phagocytosis, lysozyme activity, mitochondrial activity, LMS, oxyradical production, Comet assay, and micronuclei frequency were assessed on the coelomic fluid cells. Coelomic cells were obtained using a non-invasive extrusion method according to Eyambe et al. (1991) with some modifications. Polychaetes were individually placed in 15 ml glass tubes containing 10 ml of 3% ethanol and 1,0 mg/ml EDTA in Hanks' balanced salt solution (H6648, Sigma Italy). The obtained cell suspension was centrifuged at 200 g at 4°C for 5 min to recover the cells; water was discarded and the pellet gentle resuspended in 2 ml ASW. In detail, phagocytosis analysis was assessed by quantifying cellular intake of Neutral Red-stained Zymosan according to Ciacci et al. (2012). 100 uL of cell suspension was incubated with stained Zymosan with 20 to 40 cells from each exposure condition then examined using image analysis by ImageJ release 1.51 K shareware software (NiH, USA).
Lysosomal enzyme release was evaluated by measuring lysozyme activity in the extracellular medium according to Chu and La Peyre (1989). Lysozyme activity in coelomic cell suspensions obtained from organisms exposed to the different conditions was determined spectrophotometrically at 450 nm utilizing M. lysodeikticus and referenced against results of organisms exposed to natural sediment (Ciacci et al., 2012).
Mitochondrial activity in coelomocytes was assessed by the mitochondrial membrane potential estimation thorough the tetramethylrhodamine ethyl ester perchlorate (TMRE, ex/em: 488/580 nm) selective fluorescent dye (Scaduto and Grotyohann, 1999). A commercially available kit (AB113852, Abcam, Italy) was used. Coelomic cells were incubated with 50 nM TMRE for 15 min in a 2 ml Eppendorf tube, centrifuged 500 g at RT °C for 2 min and placed on a glass slide. Fluorescent emission was captured using an inverted microscope coupled with fluorescent emission (Axiovert 135, Carl Zeiss, Italy). From 30 to 60 cells were image analyzed from each exposure condition.
To assess oxyradical production (ROx) the cell-permeant 6-carboxy-2′,7′-dichlorodihydrofluorescein diacetate (H2DCFDA, C400, Thermofisher, Italy) was used as an indicator for reactive oxygen species formation in coelomocytes. 100 uL of cell suspension were incubated in humid chambers with 1 μM H2DCFDA in ASW for 15 min at RT in dark conditions following the general recommendation supplied with the manufacturer's protocol. LMS was evaluated by the Neutral Red Retention time assay as described by Catalano et al. (2012) with minor modifications. Briefly, 50 μl of cell suspension was placed on a polylysinated glass slide in a dark humidity chamber. 10 μl of neutral red working solution (50 μg/mL) was added to the slide. Cells were observed under a light inverted microscope every 10 min and pictures from several fields taken to visualize a minimum of 80–100 cells. Observation was stopped when the number of cells showing leakage of neutral red in the cell's cytosol exceeded 50% of the total. This time was taken as the neutral red retention time.
DNA fragmentation (Comet assay) was estimated according to Buffett et al. (2014). Analyses were performed on 50 cells per where 2 slides per individual were prepared, 4 individuals per treatment. After staining with DAPI (50 ng/ mL), nuclei were individually observed under a fluorescence microscope (500x). The level of DNA damage was expressed as % Tail DNA.
Micronuclei frequency (Mn), lipid peroxidation (LPO), and enzymatic antioxidant catalase (CAT) activity were assessed according to Catalano et al. (2012).
Briefly, for Mn assessment aliquots of ~1,000 coelomocytes were collected from each individual and fixed in Carnoy's solution with 4 individuals per treatment evaluated. Fixed cells were distributed on glass slides and stained with DAPI. For each specimen, 200 coelomocytes were scored to determine micronuclei formation.
LPO was assayed on intestinal cross sections (7 μm) of individual ragworm, obtained using a Leica cryostat. Cryo-sections were stained by the Schorml's reaction according to Moore (1988) and lipid peroxidation end-products quantified by image analysis (ImageJ 1.51 K, NiH, USA).
CAT was measured using enzyme-specific spectrophotometric assays at constant temperature. The enzymatic activity was assayed by absorbance at 240 nm, using 10 mM hydrogen peroxide as substrate. For enzymatic assays, protein quantification was performed according to Lowry et al. (1951) using the single enzyme homogenates.
Data Analysis
Data were preliminary checked to meet the assumptions of normality and homogeneity of variances prior to analysis. Changes in the biochemical responses as well as in the accumulation pattern of microplastics and B[a]P were evaluated by analysis of variance using Newman–Keuls test for post-hoc comparisons (α: 0.05; p below to 0.05 expressed as < 0.05). When not normally distributed, data were analyzed using the Kruskal–Wallis non-parametric test. Statistical analyses were performed by SPSS 11.0 for Windows. To facilitate the visualization of correlations among all performed treatments as well as to rank samples into groups of homogeneous observations, a principal component analysis (PCA) of all chemical and biological results, was combined to a hierarchical clustering (HCA, Avio et al., 2015). Factorial analysis and hierarchical clustering are complementary tools for exploring data. However, HCA presents some advantages as removing the last factors of a factorial analysis it also removes noise and makes the clustering more robust (Ivosev et al., 2008). Hierarchical clustering analysis was performed in R (https://cran.r-project.org/). The degree of similarity of each pair of patterns was computed using Euclidean distance on normalized sub lethal stress index responses.
Results
No significant increments of mortality rate were observed among the treatments indicating that all observed biological responses were at the sub lethal level.
Among all investigated sub-lethal responses, the efficiency of the immune system was tested thorough Zymosan-stimulated phagocytic activity on ragworm's coelomic cells (Figure 1A). Results are expressed as % of response referred to the control (assumed as 100%). Coelomocytes of both organisms exposed to sediments spiked with virgin microplastics beads (LC-MPS; HC-MPS), and sediments spiked with B[a]P pre-incubated plastic beads (B[a]P-LC; B[a]P-HC) showed significantly different immune system responses with respect to control cells (p < 0.05). However, a different pattern of responses was observed among tested exposure conditions. Polychaetes exposed to sediments spiked with virgin microplastics showed an increased induction of immune system activity (LC-MPS: + 20%; HC-MPS: + 20–25% respect to control). On the contrary, coelomocytes of annelids exposed to sediments spiked with different concentrations of B[a]P pre-incubated plastic beads (B[a]P-LC; B[a]P-HC) showed a suppression of immune system activity (− 13–30%). No clear sampling time and microbeads concentrations correlations were observed among the reported biological responses. On the other hand, none of the tested exposure conditions stimulated lysozyme release with respect to controls (Figure 1B).
The effects on mitochondrial activity in haemocytes extracted from polychaetes submitted to the different exposure conditions were evaluated utilizing specific fluorescent dyes for mitochondrial membrane potential (TMRE) and the results are reported in Figure 1C. The results indicate that sediments spiked with B[a]P (−19% respect to control) as well as sediments spiked with plastic beads pre-incubated with B[a]P (B[a]P-LC; B[a]P-HC) induced a clear suppression of mitochondrial activity (− 10–30%; p < 0.05). Furthermore, a significant correlation among the biological responses and microplastic concentration was observed (p < 0.05) thus suggesting the potential role of the plastic beads to extend the toxicity of B[a]P. On the other hand, where statistically significant, the extent of the observed biological responses was directly correlated to both the concentration of the plastic particles and the exposure time (p < 0.05).
S-B[a]P, B[a]P-LC, and B[a]P-HC exposure conditions also induced a negative effect on the LMS of polychaete coelomocytes (Figure 1D). Among all treatments, significantly higher disruption levels were reported for treatments dealing with plastic beads pre-spiked with B[a]P when compared to those where the organic pollutant molecule was administrated directly to the sediments and the control (p < 0.05). Furthermore, no significant LMS alterations were observed in treatments containing only virgin microbeads. as well as no clear correlation among the observed biological responses, the concentration of administrated B[a]P spiked microbeads and the sampling time.
The effects of oxidative stress in coelomocytes were estimated as a function of oxyradical production (Figure 1E). Highest levels were observed in B[a]P-HC (+ 27–35% respect to control, p < 0.05) treatments followed by B[a]P-LC (+ 22–24%, p < 0.05) while coelomocytes exposed to sediments spiked with B[a]P showed a significant increase in oxyradical production only after 28 days of exposure. Organisms incubated with virgin microplastics did not show any significant difference in oxyradical production in respect to control organisms. Further statistical analysis identified a significant correlation between effects and the concentration of B[a]P-spiked plastic particles, though there was no connection among the observed biological responses and sampling time.
The potential of plastic particles to increase dispersion and effects of genotoxic pollutants such as B[a]P was investigated by both scoring the increments of micronuclei formation and DNA strand breaks in coelomocytes. As expected, all treatments involving the polyaromatic molecule induced a significant increase of micronuclei frequency with respect to control organisms (Figure 2A; p < 0.05). Among all exposure conditions, the highest Mn values were scored in organisms submitted to B[a]P-HC treatments (4–5%0). These values did not differ to those recorded in organisms exposed to B[a]P-LC (3–4%0) but they were significantly higher than those observed in organisms exposed to S-B[a]P conditions (2–3%0, p < 0,05). A similar pattern of response was observed while analyzing the results of DNA fragmentation (Figure 2B). B[a]P-HC exposure conditions induced the strongest effects on DNA fragmentation with 6% tail in scored cells; followed by B[a]P-LC (5%) and S-B[a]P (3–4%). No significant biological effects were observed in treatments where sediments were spiked with virgin microplastic alone. No clear correlation between the distribution pattern of the biological responses and the sampling time was observed; while on the contrary, a significant direct correlation between the DNA strand breaks analysis and the B[a]P spiked microbeads levels was observed (p < 0.05).
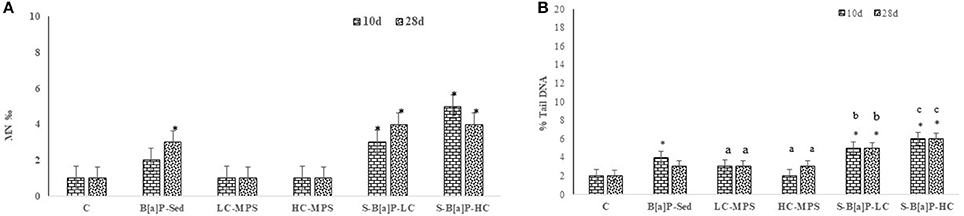
Figure 2. Genotoxicity assessment in coelomocytes of H. diversicolor exposed to uncontaminated sediments (C), D sediments spiked with 1 mg/L B[a]P (S-B[a]P), sediments spiked with 200 and 2000 particles/kg virgin PVC (LC-MPS, HC-MPS) and sediments with B[a]P spiked microplastic particles (B[a]P-LC: 200 particles/kg.; B[a]P-HC: 2000 particles/kg). (A) Microunuceli frequency, (B) DNA strand breaks. *p < 0.05. Data are expressed as mean values ± standard deviation, n = 4. Different letters indicate significant differences between groups of means (post-hoc comparison).
The effects of lipid peroxidation as well as the efficiency of the antioxidant system against the pro-oxidant effect of B[a]P activity were investigated at tissue level in H. diversicolor. Results of lipofuscin content and the activities of catalase are shown in Figure 3.
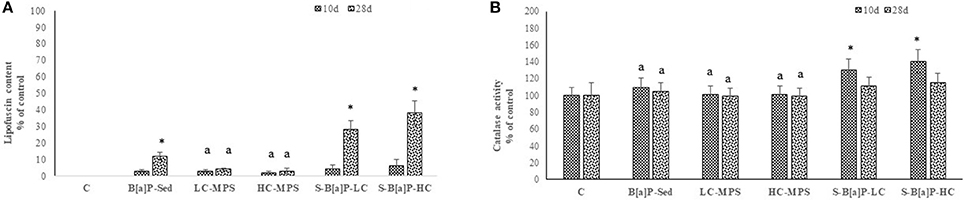
Figure 3. Biological responses in tissues of H. diversicolor exposed to uncontaminated sediments (C), D sediments spiked with 1 mg/L B[a]P (S-B[a]P), sediments spiked with 200 and 2000 particles/kg virgin PVC (LC-MPS, HC-MPS) and sediments with B[a]P spiked microplastic particles (B[a]P-LC: 200 particles/kg.; B[a]P-HC: 2000 particles/kg). (A) Lipofuscin content, (B) Catalase activity. *p < 0.05. Data are expressed as mean values ± standard deviation, n = 6. Different letters indicate significant differences between groups of means (post-hoc comparison).
As expected, only exposures involving B[a]P induced a significant increase in lipofuscins with respect to control organisms (Figure 3A, p < 0.05). Interestingly, significantly increases only occurred after 28 days of exposure. The highest values were recorded within treatment B[a]P-HC (+38%, respect to control), followed by B[a]P-LC (+28%) and S-B[a]P (+12%). Post-hoc analysis further discriminated two separate groups of results, with biological responses obtained within B[a]P-LC and B[a]P-HC treatments statistically different to those recorded following S-B[a]P exposure conditions (p < 0.05).
In a similar way, catalase activity was triggered by exposures to low and high concentrations of B[a]P spiked microbeads (p < 0.05). However, different from the distribution of the LPO response, significant increments of this anti-oxidant enzyme were limited to samples collected after 10 days of exposure. On the other hand, due to the large standard variation no significant time related responses were observed across all treatment conditions even if CAT activity values were generally lower in samples collected after 28 days of exposure.
Through different routes, polychaetes were exposed to similar doses of B[a]P. The resulting body burden in the whole body of organisms sampled 10 and 28 days after the beginning of the experiments is presented in Figure 4.
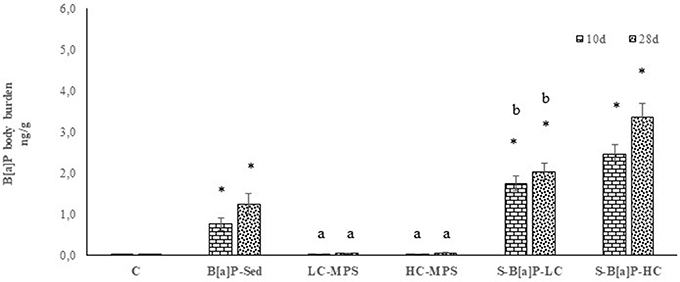
Figure 4. Benzo(a)pyrene body burden accumulation in whole body of H. diversicolor exposed to uncontaminated sediments (C), D sediments spiked with 1 mg/L B[a]P (S-B[a]P), sediments spiked with 200 and 2000 particles/kg virgin PVC (LC-MPS, HC-MPS) and sediments with B[a]P spiked microplastic particles (B[a]P-LC: 200 particles/kg.; B[a]P-HC: 2000 particles/kg). Data are expressed as mean values ± standard deviation, n = 6. Different letters indicate significant differences between groups of means (post-hoc comparison).
Accumulation patterns ranged from below the detection limit (0,1 ng/g ww) to 3.35 ± 0.40 ng/g ww., were observed in organisms exposed to marine sediments with a high loading of B[a]P-spiked plastic particles (2,000 particles/Kg of sediment). Intermediate levels of accumulation ranging from 1.75 to 2.03 ng/g ww., were shown by organisms exposed to 200 particles/Kg of sediments (B[a]P-LC), with organisms treated with B[a]P spiked sediments (S-B[a]P) recording 0.76–1.75 ng/g ww. Organisms treated with low (200 particles/Kg of sediment) and high (2,000 particles/Kg of sediment) levels of virgin plastic beads did not show any accumulation pattern as B[a]P levels were always below the detection limit.
Furthermore, the accumulation of MPS in tissues of organisms was investigated at the end of the exposure and purging processes. Very few, from zero to six, plastic particles were detected in tissues of organisms exposed to all treatments dealing with plastics beads (data not presented).
Data were further analyzed to investigate possible correlations among sampling time and levels of B[a]P-spiked plastic particles among all testing conditions. Three defined groups were identified by the analysis: S-B[a]P, B[a]P-LC and B[a]P-HC (p < 0.05). Furthermore, a direct correlation between B[a]p tissue's accumulation and the levels of B[a]P-spiked particles; while in the meantime, a direct correlation with sampling time turned to be significant only for S-B[a]P and B[a]P-HC (p < 0.05).
All biological and chemical analyses were combined to perform a PCA and Hierarchical Clustering (Figure 5). The PCA produced a two-dimensional pattern explaining 73% of total variance. Parameters like ROx, LPO, LMS, B[a]P accumulation, DNA strand breaks and Mn mainly contributed in the discriminatory power of the first dimension (46% of explained variance. On the other hand, CAT, PhC, MtO, and Lz determined the separation along the second dimension contributing to explain the 27% of the variance.
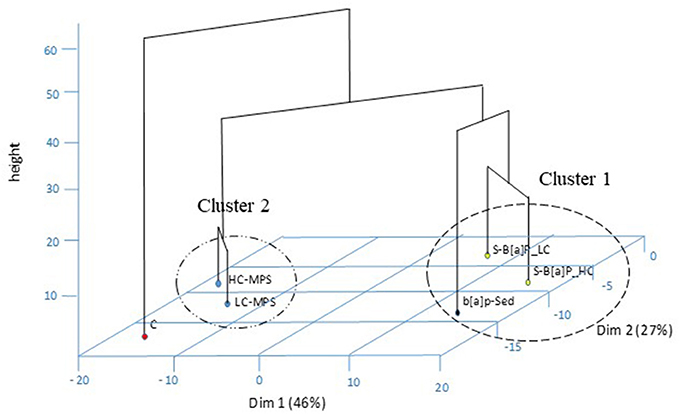
Figure 5. Principal Component Analysis and hierarchical clustering on chemical and biological data in ragworms exposed to various microplastics treatments: uncontaminated sediments (C), D sediments spiked with 1 mg/L B[a]P (S-B[a]P), sediments spiked with 200 and 2000 particles/kg virgin PVC (LC-MPS, HC-MPS) and sediments with B[a]P spiked microplastic particles (B[a]P-LC: 200 particles/kg.; B[a]P-HC: 2000 particles/kg).
The hierarchical clustering within the PCA pattern indicated a clear separation among control, B[a]P treated (cluster 1) and virgin plastics exposed ragworms (cluster 2). Furthermore, within cluster 1 a sub-separation among B[a]P spiked sediments and sediments spiked with B[a]P pre-incubated plastic particles was observed.
Discussion
Several recent studies have confirmed that plastic micro fragments may act as vectors for organic pollutant dispersion in aquatic environments (Rios et al., 2010; da Costa et al., 2016; Lohmann, 2017). Further research has shown that plastic debris sorb, concentrate and transport POPs in the marine environment that can subsequently be ingested by marine organisms (Teuten et al., 2009; Zarfl and Matthies, 2010; Browne et al., 2013; Schirinzi et al., 2016). However, little is known about pollutant's desorption rate during digestive processes hence chemicals' potential ability to accumulate in tissue and organs and disrupt key ecophysiological processes in organism of ecological relevance (Bakir et al., 2014). In our study we focused on the benthic marine environment as sediments acts as relevant repository compartments for a broad range of organic and inorganic pollutants as well as final destination of large amounts of different plastic polymers. The present investigation aimed at unveiling the contribution of ingesting microplastic vs. sediments as vectors for pollutants to the tissues as well as its derived ecotoxicological implications to aquatic organisms. The ragworm H. diversicolor was selected as candidate model organism as its biological responses to several different toxicants such as PaHs, heavy metals, surfactants, pharmaceuticals, engineered nanoparticles, etc., have been extensively investigated in the past (Catalano et al., 2012; Browne et al., 2013; Buffet et al., 2014a,b; Mouneyrac et al., 2014) and thus supporting its use both in laboratory and environmental monitoring worldwide (Mouneyrac et al., 2003; Durou et al., 2007; Gomes et al., 2013). Early studies with PAHs spiked sediments using Arenicola marina pointed out that the solubilisation of organic chemicals is significantly increased in the presence of digestive fluids compared to seawater alone, thus increasing their bioavailability (Voparil and Mayer, 2000). (Rios et al., 2007; Karapanagioti et al., 2011). The observed behavior supports the potential of plastic micro litter in trapping and facilitating the distribution of pollutants in aquatic environments, as previously addressed by several studies aiming at calculating the partition coefficients of organic chemicals on various typologies of plastic polymers (Zarfl and Matthies, 2010; Bakir et al., 2012; Heskett et al., 2012; Mizukawa et al., 2013; Lee et al., 2014; Avio et al., 2015; Kedzierski et al., 2018). On the other hand, the results of the body burden analysis on ragworms exposed to the different testing conditions clearly show a direct time- and dose-dependent accumulation of B[a]P in tissues of organisms treated with pre-incubated PVC microbeads. Furthermore, the highest accumulation peaks were observed within exposures where the organic molecule model was carried by plastic beads respect to those where the chemical was carried by the sediments. To exclude any possible contribution of un-excreted contaminated particles in the bioaccumulation assessment, the residual occurrence on MPS on tissues of investigated individuals were performed allowing to exclude any significant contribution. Therefore, the obtained results with exposed ragworms provided the clear evidence that B[a]P adsorbed on contaminated microplastics can be transferred to organisms under physiological gut conditions and concentrated in tissues. Similar conclusions were drawn by Browne et al. (2013) after exposing the annelid A. marina to sand with PVC microplastics pre-sorbed with nonylphenol and phenanthrene. The observed desorption realized during digestive processes has ecotoxicological implications since it has been speculated that the bioconcentrated chemicals may induce adverse biological effects in aquatic organisms potentially able to unpair key ecophysiological process (Oliveira et al., 2013; Bakir et al., 2014; Luís et al., 2015). To determine whether plastic microparticles are capable of transporting levels of pollutants able to disrupt functions of ragworms, we used established bioassays for mortality, immune system efficiency estimation, index of sub cellular functioning and oxidative stress assessment. At the environmentally realistic levels of dissolved benzo(a)pyrene no mortality was reported within all testing condition, leading to classify any potential biological effect at sub lethal level. Similar results were reported by Browne et al. (2013) in A. marina exposed to nonylphenol or phenanthrene. On the other hand, at sub lethal level both treatments with sediments spiked with virgin plastics as well as those treated with benzo(a)pyrene showed a biphasic effect on phagocytosis activities of NR-conjugated particles. Since phagocytosis is frequently used as a proxy for immunocompetence in aquatic organisms (Ellis et al., 2011), data on coelomocytes phagocytic activity may reproduce the global impact on the immune system. A significant stimulation in exposures dealing with virgin plastic was observed; while in the meantime an opposite inhibition of the phagocytotic activity was reported in coelomocytes of individuals treated with sediments spiked with B[a]P pre-incubated plastic particles. Since natural sediments used in the present study were not autoclaved to preserve at best their chemical and physical properties, this could have promoted a biofilm formation in virgin particles (Zettler et al., 2013). Such phenomena already reported in marine environments by Lobelle and Cunliffe (2011) could have facilitate the transport and the exposure of bacteria and other microorganisms already naturally occurring in the sediment triggering the immune system. On the other hand, B[a]P transferred by microplastics clearly inhibited the phagocytosis activities of coelomocytes of exposed ragworms. Similar conclusions are drawn by Gopalakrishnan et al. (2011) in hemocytes of the gastropod abalone H. diversicolor, by Liu et al. (2014) in hemocytes of the clam V. philippinarum as well as by Danion et al. (2011) in hemocytes of sea bass D. labrax exposed to B[a]P. According to these authors the immunosuppressive effects of B[a]P could be explained by the substantial disruption of sub cellular processes and organelle's functioning of the immune system's cells in the exposed organism. On the other hand, none of the exposure conditions induced a significant release of lysozyme thus supporting some previously reported conclusions that in hemocytes of invertebrates, the assessment of a sole parameter cannot be considered as entirely illustrative of immunocompetence (Ciacci et al., 2012). On the contrary, all treatments involving B[a]P clearly affected mitochondrial activity of coelomocytes. Such response was not surprising as several authors already reported a similar reduction of mitochondrial functionality in oyster's (P. martensii) coelomocytes, Hep 3B human cell lines and fish haemocytes exposed to polyaromatic hydrocarbons (Yang et al., 2011; Chen et al., 2016). More interesting, even if the reduction of the mitochondrial activity was similar in organisms exposed to both benzo(a)pyrene spiked sediments and sediments spiked low levels of plastic particles pre-incubated with benzo(a)pyrene, higher alterations were reported in organisms exposed to higher levels of pre-incubated particles. Such trend demonstrates the substantial direct-like contribution of microplastics levels in facilitating the pollutants translocation to marine organisms, hence inducing increments of the exposure levels promoting the alteration of key organelles functioning. A similar trend was also observed while assessing the LMS. LMS is a regularly used sub lethal stress index acting as non-specific indicator of the adverse effects of pollutants in aquatic organisms (Viarengo et al., 2007). On the other hand, the capability of benzo(a)pyrene to alter the stability of the lysosomal membranes has been previously reported for unicellular, vertebrate and invertebrate marine organisms (Marigómez and Villacorta, 2003; Moore et al., 2006; Giannapas et al., 2012; Gomiero et al., 2012) including H. diversicolor (Catalano et al., 2012). Similar to the results of mitochondrial activity assessment, highest levels of LMS were observed in testing conditions where polychaete were exposed to high loadings of pre-spiked microplastics. On the other hand, the lower LMS of coelomocytes could further explain the results of the immune system as in ragworms' haemocytes the reduction of lysosomal stability is closely linked with both the impaired cellular immunity and to the over-production of prooxidant reactive oxygen species. Indeed, a pattern of biological responses similar to the results of the LMS was reported for the oxyradical production. Lysosomal membranes are highly vulnerable to oxidative stress induced by reactive oxygen species throughout a complex pattern of direct and indirect mechanisms (Regoli and Giuliani, 2014). On the other hand, early studies report that benzo(a)pyrene and more in general all polyaromatic hydrocarbons are oxidative stress promoters in marine organisms (Bouraoui et al., 2009, 2015). High values of oxyradical production were observed in coelomocytes of organisms treated with sediments spiked with high concentrations of benzo(a)pyrene pre-incubated PVC particles respect to those exposed to both low loadings of the same particles and benzo(a)pyrene spiked sediments. The oxidative stress was also evaluated at tissue level in exposed H. diversicolor and compared to the results observed on coelomocytes aiming at benchmarking the sensitivity, accuracy, and rapidity of the responses in floating cells vs. tissue's cells. Lipofuscins content in tissue cryosections provides an integrated thorough time indication of the membrane's lipid peroxidation levels and of oxidative stress. Oxyradical species reacts with membrane lipids thorough a sequence of lipid peroxidation reactions which produce non-degradable end-products which are continuously incorporated into the lysosomes, where they accumulate as insoluble molecules (Viarengo et al., 2007). In this study, differently to what reported for the oxyradical production in coelomocytes, only organisms exposed to pre-spiked plastic particles showed a significant accumulation of lipofuscins respect to control organisms. On the other hand, lower lipofuscins increments were observed in organisms exposed to sediments spiked directly with B(a)P. This pointing out a relatively lower contribution of the bioconcentration processes thorough ragworms' dwelling activity and skin contact respect to exposures driven by pre-spiked particles. Interestingly, lipofuscins accumulation levels turned to be significant only at the end of the experiments after 28 days of exposure while levels were homogeneously distributed among all treatments during the mid-term sampling, after 10 days of exposure showing therefore a delay of the response. On the contrary, a faster, within 10 days of exposure response, was observed while assessing the catalase activity in annelid's tissues. Catalase is a well-recognized enzyme protecting vertebrate and invertebrate organism against the pro-oxidant activity of organic chemicals like B(a)P (Regoli, 2000; Bouraoui et al., 2009). Significant increments were only observed in exposures involving pre-spiked PVC plastic particles, while no effects were observed in organism exposed to spiked sediments. Such outcomes provide important indications about the exposure routes further supporting the major contribution of plastic particles in the bioavailability and indirectly the toxicity of the adopted organic molecule. Estimated enzymatic activities turned to be homogeneously distributed in organisms sampled at the end of the exposure experiments. As catalase together with other anti-oxidant enzymes are often reported having a bell-shaped trend (Viarengo et al., 2007; Gomiero et al., 2011). Therefore, a possible explanation is that effectiveness of antioxidant defenses was most likely overwhelmed by either the duration or the intensity of B[a]P pre-spiked PVC particles were adopted.
Exposure to B(a)P also determined the occurrence of several forms of genotoxicity in haemocytes of exposed ragworms. Treatments with virgin plastics did not induced any significant increment of DNA damage while significant alterations were reported within B(a)P administrated treatments. Benzo(a)pyrene like all polycyclic aromatic hydrocarbons is a well-known DNA strand breaks and micronuclei formation. Previous laboratory exposures with 0.1 and 0,5 mg/L B(a)P significantly induced DNA strand breaks and micronuclei formation in H. diversicolor (Catalano et al., 2012). Similar results were reported also by Sforzini et al. (2012) after exposing earthworms to 0.1, 10, and 50 ppm of B(a)P. In our study, while magnitude of the strand breaks was comparable in organisms exposed to B(a)P spiked sediments and organisms exposed to sediments mixed with low concentrations of pre-spiked microplastics, significantly higher values were reported in ragworms exposed to high concentrations of pre-spiked microplastics. On the other hand, nuclear alterations appeared more steadily distributed among all the treatments, resulting in a progressive increment of micronuclei frequency after the exposure to benzo(a)pyrene-contaminated PVC microparticles. This pattern of genotoxic effects allows to hypothesize that DNA strand breaks represent the first form of damage caused by the pro-oxidant properties of B(a)P. A more elevated prooxidant challenge caused by both sediments and PVC contaminated particles compared to virgin polymers would determine an irreversible loss of DNA integrity, leading to enhanced frequency of micronuclei in the most severe exposure conditions. On this context, oxyradical production was already shown to adversely modulate immune responses, lysosomal dysfunction, mitochondrial activity disruption in haemocytes of marine organisms exposed to polyaromatic molecules (Catalano et al., 2012; Browne et al., 2013; Avio et al., 2015). The overall evaluation of biological response and chemical analyses were performed by hierarchical clustering analysis. Such PCA based analysis provided a clear separation between organisms treated as control of exposed to sediments spiked with virgin plastics, sediments directly spiked with B(a)P and sediments spiked with pre-incubated PVC. A fist separation showed two distinctive clusters one dominated by virgin PVC particles and one dominated by B(a)P treatments. A second separation in the B(a)P cluster divided data in two sub-cluster: one represented by results of exposures with sediments spiked with pre-incubated PVC and the other grouping data from sediments spiked directly with the organic molecule model. Such separation shows as B[a]P adsorbed on contaminated microplastics can be more easily transferred to organisms under physiological gut conditions, concentrated in tissues and elicit biological than pollutants adsorbed in sediments and translocated to organism by passive bioconcentration phenomena.
Conclusions
In conclusion, the present study provides evidence that microplastic (PVC) particles adsorb the organic contaminant benzo(a)pyrene from seawater and can assist in transferring this compound to the representative benthic species (H. diversicolor), enhancing its rate of bioaccumulation. Compared to responses at tissue level, coelomocytes of H. diversicolor responded faster to B(a)P in a sensitive manner, helping to both understand the complexity of the induced stress syndrome and unveil the related toxicodynamic. Further endpoint tests of high ecological meaning not investigated within in the present study i.e., the potential impact on burrowing behavior could be considered in future investigations. The use of H.diversicolor for effective ecotoxicological research, both in the laboratory and field, is supported by the presented research.
Ethics Statement
The use of annelids in laboratory experiments did not require the approval of the ethical committee according to the Italian regulations.
Author Contributions
AG and PS: designed the outline of the study; PS and VS: were involved in the exposure setup; PS and GP: were involved in field collection of sediments; VS and GP: conducted statistical analyses; AG: performed chemical and biological analyses supported by VS; AG and GF: wrote the manuscript with input from all other authors.
Conflict of Interest Statement
The authors declare that the research was conducted in the absence of any commercial or financial relationships that could be construed as a potential conflict of interest.
Acknowledgments
The authors are grateful to Dr. S. Bamber for English proofreading.
References
ASTM (2013). Standard Guide for Conducting Sediment Toxicity Tests with Polychaetous Annelids. E1611
Avio, C. G., Gorbi, S., Milan, M., Benedetti, M., Fattorini, D., d'Errico, G., et al. (2015). Pollutants bioavailability and toxicological risk from microplastics to marine mussels. Environ. Pollut. 198, 211–222. doi: 10.1016/j.envpol.2014.12.021
Bakir, A., Rowland, S. J., and Thompson, R. C. (2012). Competitive sorption of persistent organic pollutants onto microplastics in the marine environment. Mar. Pollut. Bull. 64, 2782–2789. doi: 10.1016/j.marpolbul.2012.09.010
Bakir, A., Rowland, S. J., and Thompson, R. C. (2014). Enhanced desorption of persistent organic pollutants from microplastics under simulated physiological conditions. Environ. Pollut. 185, 16–23. doi: 10.1016/j.envpol.2013.10.007
Bihari, N., Fafandel, M., and Piškur, V. (2007). Polycyclic aromatic hydrocarbons and ecotoxicological characterization of seawater, sediment, and mussel Mytilus galloprovincialis from the Gulf of Rijeka, the Adriatic Sea, Croatia. Arch. Env. Contam. Toxicol. 52, 379–387. doi: 10.1007/s00244-005-0259-5
Bouraoui, Z., Banni, M., Ghedira, J., Clerandeau, C., Narbonne, J. F., Boussetta, H., et al. (2009). Evaluation of enzymatic biomarkers and lipoperoxidation level in Hediste diversicolor exposed to copper and benzo [a] pyrene. Ecotoxicol. Env. Saf. 72, 1893–1898. doi: 10.1016/j.ecoenv.2009.05.011
Bouraoui, Z., Ghedira, J., and Boussetta, H. (2015). Biomarkers responses in different body regions of the polychaeta Hediste diversicolor (Nereidae, Polychaete) exposed to copper. Rev. Gestão Costeira Integr. J. Integr. Coast. Zone Manage. 15, 371–376. doi: 10.5894/rgci594
Browne, M. A., Niven, S. J., Galloway, T. S., Rowland, S. J., and Thompson, R. C. (2013). Microplastic moves pollutants and additives to worms, reducing functions linked to health and biodiversity. Curr. Biol. 23, 2388–2392. doi: 10.1016/j.cub.2013.10.012
Buffet, P. E., Poirier, L., Zalouk-Vergnoux, A., Lopes, C., Amiard, J. C., Gaudin, P., et al. (2014a). Biochemical and behavioural responses of the marine polychaete Hediste diversicolor to cadmium sulfide quantum dots (CdS QDs): waterborne and dietary exposure. Chemosphere 100, 63–70. doi: 10.1016/j.chemosphere.2013.12.069
Buffet, P. E., Zalouk-Vergnoux, A., Châtel, A., Berthet, B., Métais, I., Perrein-Ettajani, H., et al. (2014b). marine mesocosm study on the environmental fate of silver nanoparticles and toxicityeffects on two endobenthic species: the ragworm Hediste diversicolor and the bivalve mollusc Scrobicularia plana. Sci. Tot. Environ. 470, 1151–1159. doi: 10.1016/j.scitotenv.2013.10.114
Carson, H. S. (2013). The incidence of plastic ingestion by fishes: from the prey's perspective. Mar. Pollut. Bull. 74, 170–174. doi: 10.1016/j.marpolbul.2013.07.008
Catalano, B., Moltedo, G., Martuccio, G., Gastaldi, L., Virno-Lamberti, C., Lauria, A., et al. (2012). Can Hediste diversicolor (Nereidae, Polychaete) be considered a good candidate in evaluating PAH contamination? A multimarker approach. Chemosphere 86, 875–882. doi: 10.1016/j.chemosphere.2011.10.040
Chen, H., Song, Q., Diao, X., and Zhou, H. (2016). Proteomic and metabolomic analysis on the toxicological effects of Benzo [a] pyrene in pearl oyster Pinctada martensii. Aquat. Toxicol. 175, 81–89. doi: 10.1016/j.aquatox.2016.03.012
Chu, F. L. E., and La Peyre, J. F. (1989). Effect of environmental factors and parasitism on hemolymph lysozyme and protein of American oysters (Crassostrea virginica). J. Invert. Pathol. 54, 224–232. doi: 10.1016/0022-2011(89)90032-3
Ciacci, C., Canonico, B., Bilanicovă, D., Fabbri, R., Cortese, K., Gallo, G., et al. (2012). Immunomodulation by different types of N-oxides in the hemocytes of the marine bivalve Mytilus galloprovincialis. PLoS ONE 7:E36937. doi: 10.1371/journal.pone.0036937
Claessens, M., Van Cauwenberghe, L., Vandegehuchte, M. B., and Janssen, C. R. (2013). New techniques for the detection of microplastics in sediments and field collected organisms. Mar. Pollut. Bull. 70, 227–233. doi: 10.1016/j.marpolbul.2013.03.009
Clark, J. R., Cole, M., Lindeque, P. K., Fileman, E., Blackford, J., Lewis, C., et al. (2016). Marine microplastic debris: a targeted plan for understanding and quantifying interactions with marine life. Front. Ecol. Environ. 14, 317–324. doi: 10.1002/fee.1297
da Costa, J. P., Santos, P. S., Duarte, A. C., and Rocha-Santos, T. (2016). (Nano) plastics in the environment–sources, fates and effects. Sci. Tot. Environ. 566, 15–26. doi: 10.1016/j.scitotenv.2016.05.041
Danion, M., Le Floch, S., Kanan, R., Lamour, F., and Quentel, C. (2011). Effects of in vivo chronic hydrocarbons pollution on sanitary status and immune system in sea bass (Dicentrarchus labrax L.). Aquat. Toxicol. 105, 300–311. doi: 10.1016/j.aquatox.2011.06.022
Davison, P., and Asch, R. G. (2011). Plastic ingestion by mesopelagic fishes in the North Pacific Subtropical, Gyre. Mar. Ecol. Prog. Ser. 432, 173–180. doi: 10.3354/meps09142
Durou, C., Poirier, L., Amiard, J. C., Budzinski, H., Gnassia-Barelli, M., Lemenach, K., et al. (2007). Biomonitoring in a clean and a multi-contaminated estuary based on biomarkers and chemical analyses in the endobenthic worm Nereis diversicolor. Environ. Pollut. 148, 445–458. doi: 10.1016/j.envpol.2006.12.022
Ellis, R. P., Parry, H., Spicer, J. I., Hutchinson, T. H., Pipe, R. K., and Widdicombe, S. (2011). Immunological function in marine invertebrates: responses to environmental perturbation. Fish Shellfish Immunol. 30, 1209–1222. doi: 10.1016/j.fsi.2011.03.017
Eyambe, G. S., Goven, A. J., Fitzpatrick, L. C., Venables, B. J., and Cooper, E. L. (1991). A non-invasive technique for sequential collection of earthworm (Lumbricus terrestris) leukocytes during subchronic immunotoxicity studies. Lab. Anim. 25, 61–67. doi: 10.1258/002367791780808095
Gaspar, T. R. (2017). Polystyrene Particle Uptake and Their Potential to Act as a Trojan Horse for Pyrene to Oysters. Doctoral dissertation, The University of North Carolina at Charlotte, Crassostrea Virginica.
Giannapas, M., Karnis, L., and Dailianis, S. (2012). Generation of free radicals in haemocytes of mussels after exposure to low molecular weight PAH components: immune activation, oxidative and genotoxic effects. Comp. Biochem. Physiol. C Toxicol. Pharmacol. 155, 182–189. doi: 10.1016/j.cbpc.2011.08.001
Gomes, T., Gonzalez-Rey, M., Rodríguez-Romero, A., Trombini, C., Riba, I., Blasco, J., et al. (2013). Biomarkers in Nereis diversicolor (Polychaeta: Nereididae) as management tools for environmental assessment on the southwest Iberian coast. Sci. Mar. 77, 69–78. doi: 10.3989/scimar.03731.27F
Gomiero, A., Da Ros, L., Nasci, C., Meneghetti, F., Spagnolo, A., and Fabi, G. (2011). Integrated use of biomarkers in the mussel Mytilus galloprovincialis for assessing off-shore gas platforms in the Adriatic Sea: results of a two-year biomonitoring program. Mar. Pollut. Bull. 62, 2483–2495. doi: 10.1016/j.marpolbul.2011.08.015
Gomiero, A., Sforzini, S., Dagnino, A., Nasci, C., and Viarengo, A. (2012). The use of multiple endpoints to assess cellular responses to environmental contaminants in the interstitial marine ciliate Euplotes crassus. Aquat. Toxicol. 114, 206–216. doi: 10.1016/j.aquatox.2012.02.030
Gopalakrishnan, S., Huang, W. B., Wang, Q. W., Wu, M. L., Liu J., and Wang, K. J., et al. (2011). Effects of tributyltin and benzo [a] pyrene on the immune-associated activities of hemocytes and recovery responses in the gastropod abalone, Haliotis diversicolor. Comp. Biochem. Toxicol. Pharmacol. 154, 120–128. doi: 10.1016/j.cbpc.2011.04.004
Gregory, M. R. (2009). Environmental implications of plastic debris in marine settings—entanglement, ingestion, smothering, hangers-on, hitch-hiking and alien invasions. Philos. Trans. R. Soc. B Biol. Sci. 364, 2013–2025. doi: 10.1098/rstb.2008.0265
Berry, K. L. E., Rintoul, L., and Hoogenboom, M. O. (2015). Microplastic ingestion by scleractinian corals. Mar. Biol. 162, 725–732. doi: 10.1007/s00227-015-2619-7
Hanvey, J. S., Lewis, P. J., Lavers, J. L., Crosbie, N. D., Pozo, K., Clarke, B. O., et al. (2017). A review of analytical techniques for quantifying microplastics in sediments. Anal. Methods 9, 1369–1383. doi: 10.1039/C6AY02707E
Heskett, M., Takada, H., Yamashita, R., Yuyama, M., Ito, M., Geok, Y. B., et al. (2012). Measurement of persistent organic pollutants (POPs) in plastic resin pellets from remote islands: toward establishment of background concentrations for International Pellet Watch. Mar. Poll. Bull 64, 445–448. doi: 10.1016/j.marpolbul.2011.11.004
IARC Working Group on the Evaluation of Carcinogenic Risks to Humans, and World Health, Organization. (1987). Overall Evaluations of Carcinogenicity: an Updating of IARC Monographs Volumes 1 to 42 (Vol. 7). World Health Organization.
Ivosev, G., Burton, L., and Bonner, R. (2008). Dimensionality reduction and visualization in principal component analysis. Anal. Chem. 80, 4933–4944. doi: 10.1021/ac800110w
Jørgensen, A., Giessing, A. M., Rasmussen, L. J., and Andersen, O. (2008). Biotransformation of polycyclic aromatic hydrocarbons in marine polychaetes. Mar. Environ. Res. 65, 171–186. doi: 10.1016/j.marenvres.2007.10.001
Karapanagioti, H. K., Endo, S., Ogata, Y., and Takada, H. (2011). Diffuse pollution by persistent organic pollutants as measured in plastic pellets sampled from various beaches in Greece. Mar. Pollut. Bull. 62, 312–317. doi: 10.1016/j.marpolbul.2010.10.009
Kedzierski, M., D'Almeida, M., Magueresse, A., Le Grand, A., Duval, H., César, G., et al. (2018). Threatof plastic ageing in marine environment. Adsorption/desorption of micropollutants. Mar Pollut. Bull 127, 684–694. doi: 10.1016/j.marpolbul.2017.12.059
Kühn, S., Rebolledo, E. L. B., and van Franeker, J. A. (2015). “Deleterious effects of litter on marine life,” in Marine Anthropogenic Litter, (Springer International Publishing), 75–116.
Lee, H., Shim, W. J., and Kwon, J. H. (2014). Sorption capacity of plastic debris for hydrophobic organic chemicals. Sci. Total Environ. 470–471, 1545–1552. doi: 10.1016/j.scitotenv.2013.08.023
Liu, N., Pan, L., Gong, X., Tao, Y., Hu, Y., and Miao, J. (2014). Effects of benzo (a) pyrene on differentially expressed genes and haemocyte parameters of the clam Venerupis philippinarum. Ecotoxicology 23, 122–132. doi: 10.1007/s10646-013-1157-7
Lobelle, D., and Cunliffe, M. (2011). Early microbial biofilm formation on marine plastic debris. Mar. Pollut. Bull. 62, 197–200. doi: 10.1016/j.marpolbul.2010.10.013
Lohmann, R. (2017). Microplastics are not important for the cycling and bioaccumulation of organic pollutants in the oceans—but should microplastics be considered POPs themselves. Int. Environ. Assess.Manage. 13, 460–465. doi: 10.1002/ieam.1914
Lowry, O. H., Rosebrough, N. J., Farr, A. L., and Randall, R. J. (1951). Protein measurement with the Folin phenol reagent. J. Biol. Chem. 193, 265–275.
Luís, L. G., Ferreira, P., Fonte, E., Oliveira, M., and Guilhermino, L. (2015). Does the presence of microplastics influence the acute toxicity of chromium (VI) to early juveniles of the common goby (Pomatoschistus microps)? A study with juveniles from two wild estuarine populations. Aquat. Toxicol. 164, 163–174. doi: 10.1016/j.aquatox.2015.04.018
Maria, V. L., and Bebianno, M. J. (2011)Antioxidant lipid peroxidation responses in Mytilus galloprovincialis exposed to mixtures of benzo (a) pyrene copper. Comp. Biochem. Physiol. C Toxicol. Pharmacol. 154, 56–63. doi: 10.1016/j.cbpc.2011.02.004
Marigómez, I., and Villacorta, L. B. (2003). Pollutant-specific and general lysosomal responses in digestive cells of mussels exposed to model organic chemicals. Aquat. Toxicol.64. 235–257 doi: 10.1016/S0166-445X(03)00056-0
Mizukawa, K., Takada, H., Ito, M., Geok, Y. B., Hosoda, J., Yamashita, R., et al. (2013). Monitoring of a wide range of organic micropollutants on the Portuguese coast using plastic resin pellets. Mar. Pollut. Bull. 70, 296–302. doi: 10.1016/j.marpolbul.2013.02.008
Moore, M. N. (1988). Cytochemical responses of the lysosomal system and NADPH-ferrihemoprotein reductase in molluscan digestive cells to environmental and experimental exposure to xenobiotics. Mar. Ecol. Prog. Ser. 46, 81–89. doi: 10.3354/meps046081
Moore, M. N., and Icarus, A. J. (2006). Environmental prognostics: an integrated model supporting lysosomal stress responses as predictive biomarkers of animal health status. Mar. Environ. Res. 61, 278–304. doi: 10.1016/j.marenvres.2005.10.005
Mouneyrac, C., Buffet, P. E., Poirier, L., Zalouk-Vergnoux, A., Guibbolini, M., Risso-de Faverney, C., et al. (2014). Fate and effects of metal-based nanoparticles in two marine invertebrates, the bivalve mollusc Scrobicularia plana and the annelid polychaete Hediste diversicolor 21, 7899–7912. doi: 10.1007/s11356-014-2745-7
Mouneyrac, C., Mastain, O., Amiard, J. C., Amiard-Triquet, C., Beaunier, P., Jeantet, A. Y., et al. (2003). Trace-metal detoxification and tolerance of the estuarine worm Hediste diversicolor chronically exposed in their environment 143, 731–744. doi: 10.1007/s00227-003-1124-6
Nuelle, M. T., Dekiff, J. H., Remy, D., and Fries, E. (2014). A new analytical approach for monitoring microplastics in marine sediments. Environ. Pollut. 184, 161–169. doi: 10.1016/j.envpol.2013.07.027
Oliveira, M., Ribeiro, A., Hylland, K., and Guilhermino, L. (2013). Single and combined effects of microplastics and pyrene on juveniles (0+ group) of the common goby Pomatoschistus microps (Teleostei, Gobiidae). Ecol. Indic. 34, 641–647. doi: 10.1016/j.ecolind.2013.06.019
Paul-Pont, I., Lacroix, C., Fernández, C. G., Hégaret, H., Lambert, C., Le Goïc, N., et al. (2016). Exposure of marine mussels Mytilus spp. to polystyrene microplastics: toxicity and influence on fluoranthene bioaccumulation. Environ. Pollut. 216, 724–737. doi: 10.1016/j.envpol.2016.06.039
Pellini, G., Gomiero, A., Fortibuoni, T., Ferrà, C., Grati, F., Tassetti, N., et al. (2018). Characterization of microplastic litter in the gastrointestinal tract of Solea solea from the Adriatic Sea. Environ. Pollut. 234, 943–952. doi: 10.1016/j.envpol.2017.12.038
Regoli, F. (2000). Total oxyradical scavenging capacity (TOSC) in polluted and translocated mussels: a predictive biomarker of oxidative stress. Aquat. Toxicol. 50, 351–361. doi: 10.1016/S0166-445X(00)00091-6
Regoli, F., and Giuliani, M. E. (2014). Oxidative pathways of chemical toxicity and oxidative stress biomarkers in marine organisms. Mar. Environ. Res. 93, 106–117. doi: 10.1016/j.marenvres.2013.07.006
Rios, L. M., Jones, P. R., Moore, C., and Narayan, U. V. (2010). Quantitation of persistent organic pollutants adsorbed on plastic debris from the Northern Pacific Gyre's “eastern garbage patch. J. Environ. Month 12, 2226–2236. doi: 10.1039/c0em00239a
Rios, L. M., Moore, C., and Jones, P. R. (2007). Persistent organic pollutants carried by synthetic polymers in the ocean environment. Mar. Pollut. Bull. 54, 1230–1237. doi: 10.1016/j.marpolbul.2007.03.022
Scaduto, R. C., and Grotyohann, L. W. (1999). Measurement of mitochondrial membrane potential using fluorescent rhodamine derivatives. Biophys. J. 76, 469–477. doi: 10.1016/S0006-3495(99)77214-0
Schirinzi, G., Llorca, M., Sanchís, J., Farré, M., and Barceló, D. (2016). “Analytical characterization of microplastics and assessment of their adsorption capacity for organic contaminants,” in Monitoring for a Sustainable Management of Marine Resources, eds G. Sacchettini, and M. Giulivo (Fidenza: Mattioli 1885 S.p.A.) 15–19.
Schumacher, B. A. (2002). Methods for the Determination of Total Organic Carbon (TOC) in Soils and Sediments. Ecological Risk Assessment Support Center. 1–23.
Sforzini, S., Boeri, M., Dagnino, A., Oliveri, L., Bolognesi, C., Viarengo, A., et al. (2012). Genotoxicity assessment in Eisenia andrei coelomocytes: a study of the induction of DNA damage and micronuclei in earthworms exposed to B [a] P-and TCDD-spiked soils. Mutat. Res. 746, 35–41. doi: 10.1016/j.mrgentox.2012.02.011
Syberg, K., Khan, F. R., Selck, H., Palmqvist, A., Banta, G. T., Daley, J., et al. (2015). Microplastics: addressing ecological risk through lessons learned. Environ. Toxicol. Chem. 34, 945–953. doi: 10.1002/etc.2914
Teuten, E. L., Saquing, J. M., Knappe, D. R., Barlaz, M. A., Jonsson, S., Björn, A., et al. (2009). Transport and release of chemicals from plastics to the environment and to wildlife. Philos. Trans. R. Soc. Biol. Sci. 364, 2027–2045. doi: 10.1098/rstb.2008.0284
Vianello, A., Boldrin, A., Guerriero, P., Moschino, V., Rella, R., Sturaro, A., et al. (2013). Microplastic particles in sediments of Lagoon of Venice, Italy: first observations on occurrence, spatial patterns and identification. Estuar. Coast. Shelf Sci. 130, 54–61. doi: 10.1016/j.ecss.2013.03.022
Viarengo, A., Lowe, D., Bolognesi, C., Fabbri, E., and Koehler, A. (2007). The use of biomarkers in biomonitoring: a 2-tier approach assessing the level of pollutant-induced stress syndrome in sentinel organisms. Comp. Biochem. Physiol. C Toxicol. Pharmacol. 146, 281–300. doi: 10.1016/j.cbpc.2007.04.011
Voparil, I. M., and Mayer, L. M. (2000). Dissolution of sedimentary polycyclic aromatic hydrocarbons into the lugworm's (Arenicola marina) digestive fluids. Environ. Sci. Technol. 34, 1221–1228. doi: 10.1021/es990885i
Wright, S. L., Thompson, R. C., and Galloway, T. S. (2013). The physical impacts of microplastics on marine organisms: a review. Environ. Pollut. 178, 483–492. doi: 10.1016/j.envpol.2013.02.031
Yang, F., Zhang, L., Huo, X. S., Yuan, J. H., Xu, D., Yuan, S. X., et al. (2011). Long noncoding RNA high expression in hepatocellular carcinoma facilitates tumor growth through enhancer of zeste homolog 2 in humans. Hepatology 54, 1679–1689. doi: 10.1002/hep.24563
Zarfl, C., and Matthies, M. (2010). Are marine plastic particles transport vectors for organic pollutants to the Arctic? Mar. Pollut. Bull. 60, 1810–1814. doi: 10.1016/j.marpolbul.2010.05.026
Keywords: microplastics, trojan horse effect, coelomocytes, hediste diversicolor, exposure assessment
Citation: Gomiero A, Strafella P, Pellini G, Salvalaggio V and Fabi G (2018) Comparative Effects of Ingested PVC Micro Particles With and Without Adsorbed Benzo(a)pyrene vs. Spiked Sediments on the Cellular and Sub Cellular Processes of the Benthic Organism Hediste diversicolor. Front. Mar. Sci. 5:99. doi: 10.3389/fmars.2018.00099
Received: 15 January 2018; Accepted: 12 March 2018;
Published: 05 April 2018.
Edited by:
Cristina Panti, University of Siena, ItalyReviewed by:
Catherine Mouneyrac, UCO Angers, FranceTim David Williams, University of Birmingham, United Kingdom
Copyright © 2018 Gomiero, Strafella, Pellini, Salvalaggio and Fabi. This is an open-access article distributed under the terms of the Creative Commons Attribution License (CC BY). The use, distribution or reproduction in other forums is permitted, provided the original author(s) and the copyright owner are credited and that the original publication in this journal is cited, in accordance with accepted academic practice. No use, distribution or reproduction is permitted which does not comply with these terms.
*Correspondence: Alessio Gomiero, YWxlc3Npby5nb21pZXJvQGlyaXMubm8=; YWxlc3Npby5nb21pZXJvQGdtYWlsLmNvbQ==