- 1The Centre for Environment, Fisheries and Aquaculture Science (Cefas), Lowestoft, United Kingdom
- 2Isles of Scilly Inshore Fisheries and Conservation Authority, Council of the Isles of Scilly, Isles of Scilly, United Kingdom
- 3Department of Coastal Systems, NIOZ Royal Netherlands Institute for Sea Research, Utrecht University, Den Burg, Netherlands
- 4Cornwall Inshore Fisheries and Conservation Authority, Chi Gallos, Hayle Marine Renewables Business Park, Hayle, United Kingdom
- 5Devon and Severn Inshore Fisheries and Conservation Authority, Brixham Laboratory, Brixham, United Kingdom
- 6RAS Aquaculture Research Ltd., Llandwrog, United Kingdom
Knowledge of larval dispersal, population dynamics and connectivity in relation to the management and conservation of commercially important species is vital if existing fisheries are to remain sustainable into the future. Larval dispersal of the commercially exploited spiny lobster, Palinurus elephas, was modeled from Marine Protected Areas located in the southwest of England for a 16-month period using a General Individuals Transport Model (GITM). The model included physical particle advection based on current fields from a 3D hydrodynamics model and a larval behavior module. Our results demonstrate the overall dispersal patterns of P. elephas larvae and highlight populations capable of self-seeding and those which are seemingly reliant on larvae from more distant populations. The results indicate where further research may be required to fully understand how populations of P. elephas are maintained at regional, national and international scales while providing us with the opportunity to discuss the effectiveness of current approaches to conservation and fisheries management.
Introduction
Background, Aims, and Approach
There are two species of spiny lobster, Palinurus elephas and Palinurus mauritanicus, that have been recorded in UK landings. Of these, P. elephas is by far the more prevalent and is the only species discussed herein. Due to its distribution, P. elephas has traditionally been the preferred target of inshore fisheries due to its accessibility and high commercial value (Goñi and Latroutte, 2005; Babbucci et al., 2010). P. elephas inhabits shallow waters to a depth of 200 m (Groeneveld et al., 2013) and is fished throughout its distribution along the eastern Atlantic coast from Norway to Morocco and throughout the Mediterranean (Kittaka and Ikegami, 1988; Holthuis, 1991; Goñi et al., 2003; Babbucci et al., 2010). The collapse of P. elephas population abundance within southwest fisheries has been attributed to a change in capture gear from pots and traps to the use of less selective tangle and trammel nets (Hepper, 1977; Goñi and Latroutte, 2005; Amengual-Ramis et al., 2016). The species was once commercially exploited throughout the region but there is evidence that the P. elephas fishery is now in an unfavorable condition in all southwest waters due to stock depletion (Goñi and Latroutte, 2005). The decline in abundance of P. elephas has resulted in very few targeted fisheries for the species remaining in the UK. One stock of P. elephas still being commercially exploited is found inhabiting the Isles of Scilly, an archipelago made up of 5 inhabited islands and approximately 140 rocky islets located in the Atlantic Ocean, 45 km off the south-western tip of the Cornish peninsula of Great Britain.
Currently, there are no Total Allowable Catch (TAC) limits or quotas applied to this species. The only international regulation pertaining to the management of this fishery is the European Union (EU) Minimum Landing Size (MLS) of 95 mm carapace length [Council Regulation (EC) No 1967/2006]. Common management measures include the setting of MLS, the return of ovigerous females, a limitation of fishing effort and closed areas (Mallol et al., 2014). However, the implementation of such regulations varies from local to national jurisdictions resulting in a confused management strategy.
In England, the key fisheries found within the 6-nautical mile limit for P. elephas are managed by the Inshore Fisheries Conservation Authorities (IFCAs). Current regulations implemented by IFCA's in England include; a minimum size of 110 mm carapace length, a Fishing For Profit Permit scheme [covering Lobster, Spiny Lobster (Crawfish), and Crab]; a prohibition on removing ovigerous females and a voluntary agreement to tag and release undersized specimens.
P. elephas is classed as “Vulnerable” under the International Union for Conservation of Nature (IUCN) designation system, meaning that it is neither endangered nor critically endangered but is facing a high risk of extinction in the wild in the medium-term future. It is listed and designated as a protected species under the UK Biodiversity Action Plan (JNCC, 20171), as a Species of Principal Importance (Marlin, 20172) and as a Species of Conservation Importance with a conservation objective of recover within several Marine Conservation Zones (MCZs) (Natural England, 20173). Although there have been no scientific stock assessments undertaken, the long-term trends in fishery statistics indicate significant overfishing. For example, landings of P. elephas into England have been falling from a peak in 1969 at over 100 tons (Hepper, 1977), to only 12 tons in 2014 (MMO, Fisheries Activity Database, 20174).
The lifecycle of most decapod species includes a dispersive planktonic larval stage (Sandifer, 1975). The duration of this larval stage or Planktonic Larval Duration (PLD) is one of the key factors that determines dispersal patterns and population connectivity (Shanks et al., 2003; Palero et al., 2008; Ayata et al., 2010). In the case of P. elephas, the PLD has been estimated to range from 5 to 12 months depending on the region and seawater temperature (Groeneveld et al., 2013). This length of PLD means P. elephas larvae have the potential to cover thousands of km before finally settling out of the water column and metamorphosing into juveniles. Although vital to the conservation and management of this commercially important species knowledge of larval dispersal, population dynamics and connectivity is currently lacking (Connell, 1985; Underwood and Fairweather, 1989; Kough et al., 2013).
A method of overcoming this lack of knowledge is to develop and use models which, by definition and necessity, are simplifications of reality. Nevertheless, provided that the simplifications and model limitations are appreciated, the simulated results are useful. This is because of their potential to illustrate processes and scenarios such as extended planktonic larval dispersal stages that cannot, or only partially and at great cost, be observed (IPCC, 2014; Van der Molen et al., 2016). For instance, in the current case it is nearly impossible to derive where P. elephas larvae originate from, or to follow individual or groups of larvae for months at sea through observation.
This study aims to assess the dispersal of P. elephas larvae and identify connectivity of key populations and Marine Protected Areas (MPAs) that may support P. elephas in the southwest of England using a General Individuals Transport Model (GITM). The biological development and behavior of P. elephas larvae are included using the behavior module of the GITM. The model outputs will augment local fisheries management and conservation strategies currently being implemented. This will be achieved by evaluating the effectiveness of current measures for maintaining local P. elephas populations. In turn, the outputs will be reviewed in the context of ensuring that economically viable fisheries persist whilst improving the conservation status of P. elephas. The model outputs will also provide an opportunity to discuss the need for fisheries management and conservation strategies at both national and international scales. We will consider if further fisheries management and conservation measures would be beneficial and at what scale these would be most effective. Model outputs will also assist in identifying where further research may be required to fully understand how populations of P. elephas are maintained across multiple scales.
Study Area
The model domain covered a region of the north-eastern Atlantic Ocean, centered on the Celtic Sea and encompassing areas of the western English Channel and southern Irish Sea (Figure 1). The study area was selected due to limited resources and the desire to conduct a locally focussed study aimed at addressing questions posed by local stakeholders. The current study area encompasses existing fisheries and known populations of P. elephas in the southwest of England. The MPA network considered here included Special Areas of Conservation (SAC) and MCZs that had either been designated for the conservation of P. elephas or that contained rocky reef suitable for the successful recruitment of juveniles.
The Life History of Palinurus elephas
P. elephas is reported to reach reproductive maturity at 3–5 years of age and at a carapace length of 80–100 mm (Hunter et al., 1996). Mating occurs between June and October with a peak in egg bearing females being observed in September–October (Mercer, 1973). P. elephas is thought to be less fecund than other species of spiny lobster with an estimated 119 eggs/g which can equate to between 23,000 and 202,000 eggs/female (Goñi et al., 2003). Eggs are incubated for a period of 6–10 months with hatching occurring between March and June (Groeneveld et al., 2013). Eggs hatch into leaf-like transparent phyllosoma (Kittaka et al., 2001) approximately 2.9–3.9 mm in length which are then dispersed on ocean currents. The PLD has been estimated at between 5 and 12 months and is thought to include between 6 and 9 instars. It should be noted that little is currently known about this phase of the larval life cycle in terms of larval behavior and developmental times between instars. Studies aimed at culturing the species identified temperature, photoperiod, food quantity, and quality as factors that strongly influence instar duration and survivability (Kittaka, 1997; Fitzgibbon and Battaglene, 2012; Matsuda et al., 2012). The final phyllosoma stage metamorphoses into a free swimming puerulus which settles on to the seabed before molting into the first benthic juvenile stage (Groeneveld et al., 2013). Juveniles molt between 8 and 12 times in the first year, decreasing to 2–6 times in subsequent years and even further after maturity is reached (Hunter, 1999). P. elephas has an adult mean annual growth rate of 10–12 mm/year and is reported to be capable of reaching a total length of up to 500 mm with a life span exceeding 25 years (Marlin, 20172).
Methods
Hydrodynamics and Particle Tracking Model
GETM: North-West European Shelf Set-Up
The 3D hydrodynamic General Estuarine Transport Model (GETM, www.getm.eu; Burchard and Bolding, 2002) solves the shallow-water, heat balance and density equations. It uses a General Ocean Turbulence Model (GOTM, Burchard et al., 1999; www.gotm.net) to solve the vertical dimension. GETM was run using the north-west European shelf setup that has been used for multiple purposes (Van et al., 2016, 2017). The set-up includes a spherical grid covering the area 46.4°N−63°N, 17.25°W−13°E with a resolution of 0.08° longitude and 0.05° latitude (approximately 5.5 km), and 25 non-equidistant layers in the vertical. The model bathymetry was based on the NOOS bathymetry (www.noos.cc/index.php?id$=$173). The model was forced with tidal constituents derived from TOPEX-POSEIDON satellite altimetry (Le Provost et al., 1998), atmospheric forcing from ECMWF ERA-Interim (Berrisford et al., 2011; Dee et al., 2011; www.ecmwf.int/en/research/climate-reanalysis/era-interim), interpolated river runoff from a range of observational data sets (the National River Flow Archive (www.ceh.ac.uk/data/nrfa/index.html) for UK rivers, the Agence de l'eau Loire-Bretagne, Agence de l'eau Seine-Normandie and IFREMER for French rivers, the DONAR database for Netherlands rivers, ARGE Elbe, the Niedersächsisches Landesamt für Ökologie and the Bundesanstalt für Gewässerkunde for German rivers, and the Institute for Marine Research, Bergen, for Norwegian rivers; see also Lenhart et al., 2010), and depth-resolved temperature- and salinity boundary conditions from ECMWF-ORAS4 (Mogensen et al., 2012; Balmaseda et al., 2013; https://www.ecmwf.int/en/research/climate-reanalysis/ocean-reanalysis).
A comparison of hydrodynamic model results with observations is provided in Appendix A (Supplementary Materials).
GITM: Particle Tracking
The Individual Behaviour Model GITM includes physical particle advection and diffusion, and biological development and behavior. The advection-diffusion elements of GITM were based on a re-coded version of the Lagrangian semi-analytical, advection-diffusion method that ensures particles follow stream lines (Wolk, 2003). Furthermore, a random walk method with advective correction (Visser, 1997) was included to simulate diffusion (Hunter et al., 1993). This method uses a constant diffusion coefficient in the horizontal direction and a variable diffusion coefficient in the vertical direction. The latter is based on the vertical diffusivity obtained from the turbulence closure model in the hydrodynamics model GETM. The combined hydrodynamics model (GETM) and particle tracking model (GITM) were applied recently to simulate the transport of plaice larvae (Tiessen et al., 2014) and population dynamics of Mnemiopsis leidyi (Van der Molen et al., 2015).
The biological development and behavior module of GITM allows particles to progress through a user-defined number of egg and larval development stages. Stage progression depends on daily growth rates which can be prescribed for each stage as constants or derived from temperature-dependent formulations. During each development stage, a particle can have a specific vertical migration behavior (e.g., neutrally buoyant; floating, sinking; diel migration or tidally cued migration). Particles can be considered as super individuals, representing a large number of individuals that can be subject to mortality functions. GITM runs off-line, using stored hydrodynamics from GETM at hourly intervals to account for the effect of tides.
Model Setup and Scenarios
The overall modeled area (Figure 1) included the western English Channel, the eastern Atlantic and the Celtic Sea. Particle release sites were selected based on local knowledge of existing populations of the P. elephas within MPAs. Particles representing larvae were released from the seabed and nine larval stages were included (Bouvier, 1914; Kittaka and Ikegami, 1988; Kittaka et al., 2001) (see Table 1 for life-history characteristics). Separate model experiments were carried out with constant growth rates, representing the extremes of observed larval durations, i.e., 5 and 12 months (Mercer, 1973; Groeneveld et al., 2013), as larval duration was expected to result in the largest uncertainty in dispersal distances. Larvae were assumed to undertake diel vertical migration between surface waters during the day and several tens of meters during the night (Goñi and Latroutte, 2005; Bermudes and Ritar, 2008). Due to the progressive increase in weight of the larvae and the resulting decrease in buoyancy (Goñi and Latroutte, 2005), latter stage larvae were given progressively higher sinking velocities, lower rising velocities and deeper night depths resulting in a fully bottom-dwelling final stage. No conditional settling behavior was applied to the particles resulting in the final particle position being fixed.
Model scenarios were run for a total of 16 months from March until June of the following year with particles being released at 2 h intervals between 1st of March and 30th of June of the same year to represent the spawning period (Groeneveld et al., 2013). The model was run until the end of June of the following year to allow the last larvae released with a 12-month duration to complete their development. Release locations were chosen at grid points inside rectangular area. Release sites comprised the Isles of Scilly SAC, Skerries Bank, and Surrounds MCZ, Lundy SAC, Bideford to Foreland Point MCZ, The Lizard Point SAC, and Padstow Bay and Surrounds MCZ (Figure 2). In total, 44,640 particles were released for each scenario.
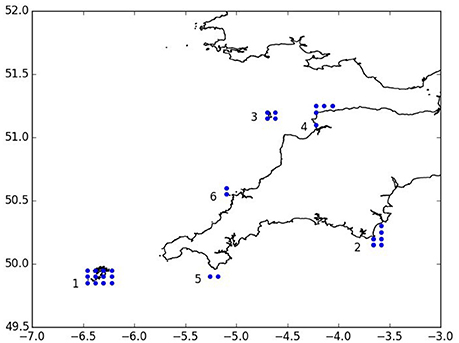
Figure 2. Particle release locations (blue dots): 1. Isles of Scilly SAC, 2. Skerries Bank and Surrounds MCZ, 3. Isle of Lundy SAC, 4. Bideford to Foreland Point MCZ, 5. The Lizard SAC, 6. Padstow Bay and Surrounds MCZ.
One model scenario was run for each of four separate and contrasting years 1996, 1997, 2006, and 2010. The typical age that P. elephas recruit into the fishery is thought to be between years based on a growth rate of 12.2 mm increase in carapace length/year (Mercer, 1973; Hunter, 1999). From this, we assumed that it takes 8 years for a larva to be recruited into the fishery. Years 1996 and 1997 were selected based on landings data (Marine Management Organization Landings data, 20175) of P. elephas from 2002 to 2014, which indicated high catches in 2004 and low catches in 2005. Assuming that larval recruitment was the main determinant for cohort size, this suggests that 1996 may have been a year of high larval recruitment, and 1997 may have been a year of low larval recruitment. The years 1996 and 1997 were also contrasting years for the recruitment of sea bass larvae in the southwest of England, probably because weak average winds with variable direction resulted in relatively low sea-surface temperatures in 1996, whereas substantial average winds from the southwest resulted in relatively high sea-surface temperatures in 1997 (Beraud et al., 2017). Based on anecdotal evidence that increased numbers of P. elephas were being observed by divers and fishermen and to relate the model outputs to the final year of available fisheries landing data, two more recent model years were also selected (2006 and 2010).
Raw model outputs consisted of daily particle positions. These were processed into contour maps of final larval positions for particles released from each release rectangle. Arrow plots were also constructed to represent connectivity between the release areas and MPAs with known populations of P. elephas and those known to or that possibly contain suitable habitat for the species e.g., rocky reef features. The automated post-processing routines only recorded connectivity where these MPAs were reached by particles. This procedure inevitably excluded small coastal MPAs represented by land on the 5 km model grid.
Results
Model Results
Final densities of larvae released from the Isles of Scilly area (area 1, Figure 3) showed a general trend of dispersal to the north into the Irish Sea and clockwise around the south and west of Ireland for both the 5 month and 12-month larval durations. As expected, larvae with a 12-month duration traveled further, with a substantial proportion leaving the domain to the north. For both larval durations, there was a degree of inter-annual variability, but the main patterns of settling were similar between the years. The majority of the larvae that remained in the domain settled around the Irish coast. For the 5 month duration larvae, high densities settled along the southern shores of Ireland. The settled larvae with 12-month duration showed higher concentrations off Wicklow (south of Dublin), off Dungarvan on the south coast, near the mouth of the Shannon estuary and off Galway on the west coast than elsewhere in the model domian. Twelve-month duration larvae also settled along the west coast of Wales. For the 5-month duration larvae, a small proportion remained within the outer reaches of the Bristol Channel, and a few settled in the vicinity of the Isles of Scilly, suggesting a low potential for self-seeding within the population. For the 12-month duration larvae, self-seeding, though very unlikely, was not entirely impossible.
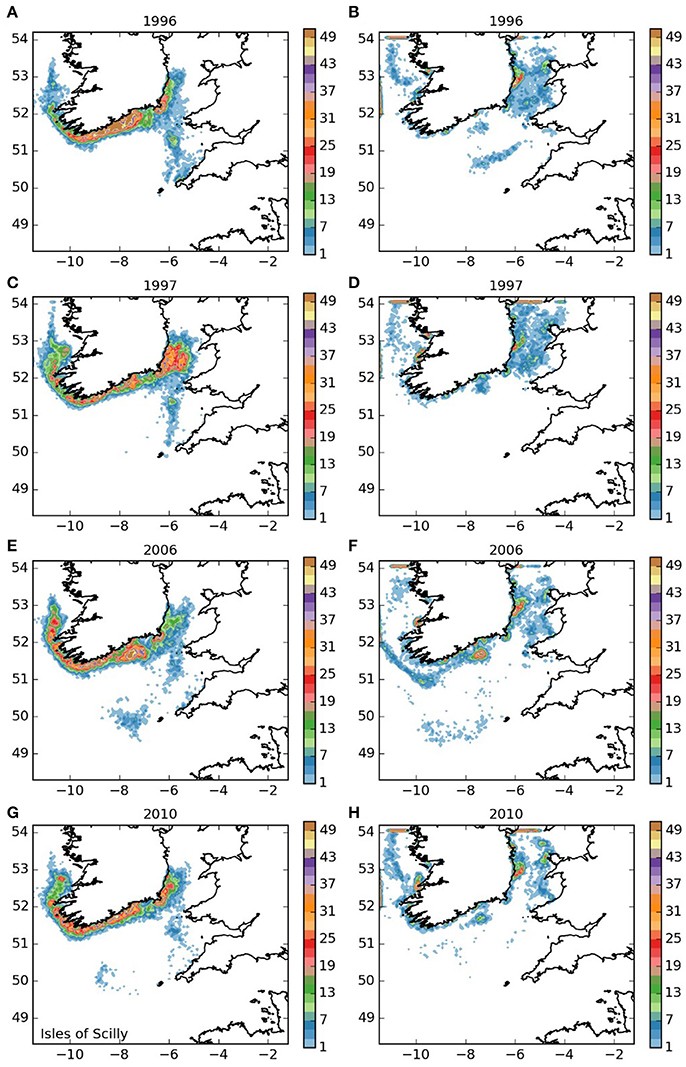
Figure 3. Final number of particles per model grid cell (density) for particles released from the Isles of Scilly SAC for each of the 4 years. (A,C,E,G) 5 months pelagic duration, (B,D,F,H) 12 months pelagic duration.
Larvae released from the Skerries Bank area (area 2, Figure 4), mostly remained within the English Channel, but a proportion were transported to the west and into the Celtic and Irish Seas. For years 1996 and 1997 and both larval durations, only limited numbers entered the Celtic Sea. However, in 2006 numbers entering the Celtic Sea were greater, and some reached similar destinations as those larvae released from the Isles of Scilly. In 2010, increased numbers of larvae appear to have remained distributed throughout the western English Channel. Of the larvae that remained within the English Channel, a proportion varying from substantial (5 month duration) to the majority (12-month duration) were transported out of the domain into the eastern English Channel. The ones that remained within the western English Channel settled mostly in Lyme Bay along the French coast of Normandy, the Channel Islands and Whitsand Bay, suggesting potential for a self-sustaining population in the Skerries Bank area.
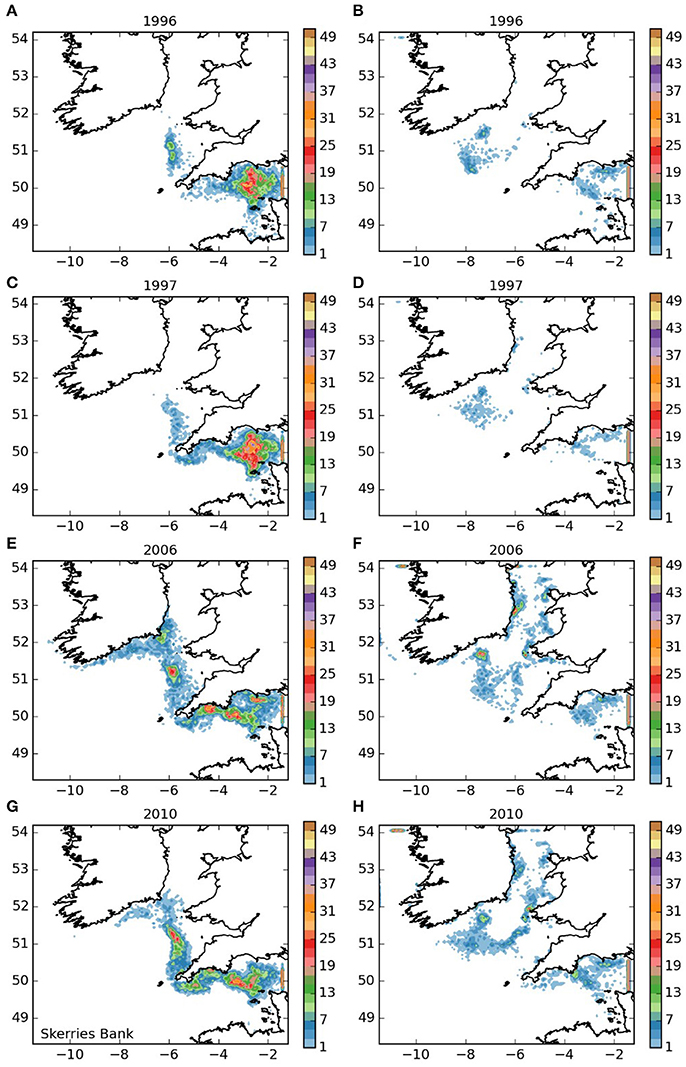
Figure 4. Final number of particles per model grid cell (density) for particles released from Skerries Bank and Surrounds MCZ for each of the 4 years. (A,C,E,G) 5 months pelagic duration, (B,D,F,H) 12 months pelagic duration.
The settling patterns of larvae released from the Lundy Island area and Bideford to Foreland Point (areas 3 and 4, and Figures 5, 6) were very similar, with larvae from Lundy traveling slightly further. Most larvae remained within the Bristol Channel with the majority settling along the south coast of Wales. There were also high concentrations off Hartland on the northern Cornish coast, indicating substantial potential for self-sustaining populations. The larvae that left the Bristol Channel were dispersed in a similar way as those released from the Isles of Scilly (Figure 3).
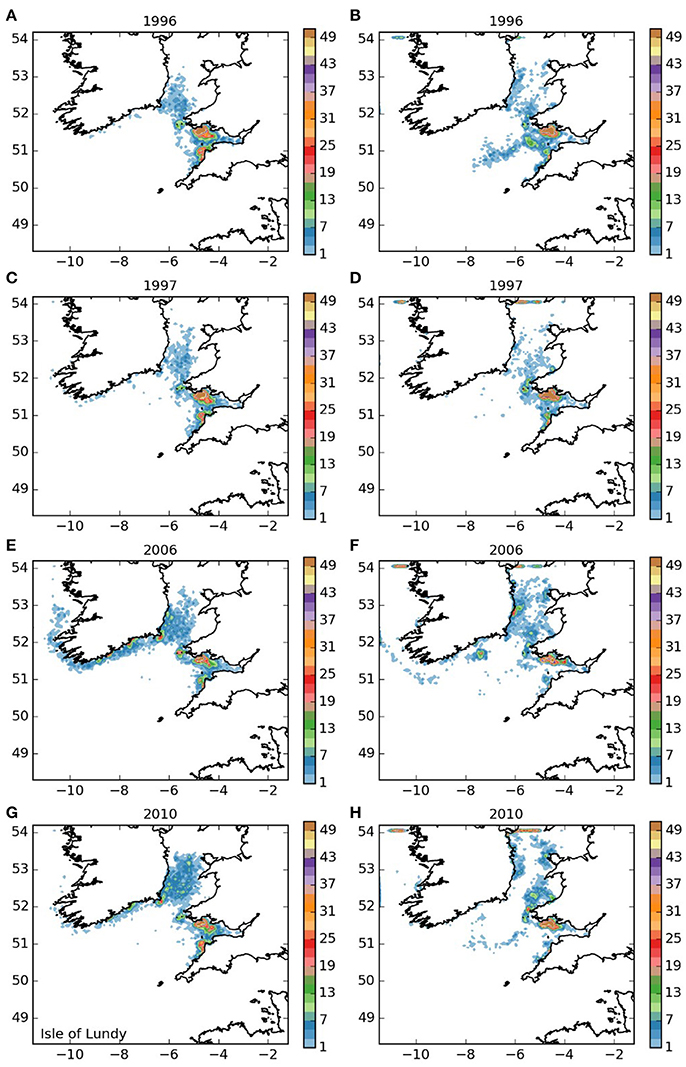
Figure 5. Final number of particles per model grid cell (density) for particles released from Lundy SAC and Surrounds MCZ for each of the 4 years. (A,C,E,G) 5 months pelagic duration, (B,D,F,H) 12 months pelagic duration.
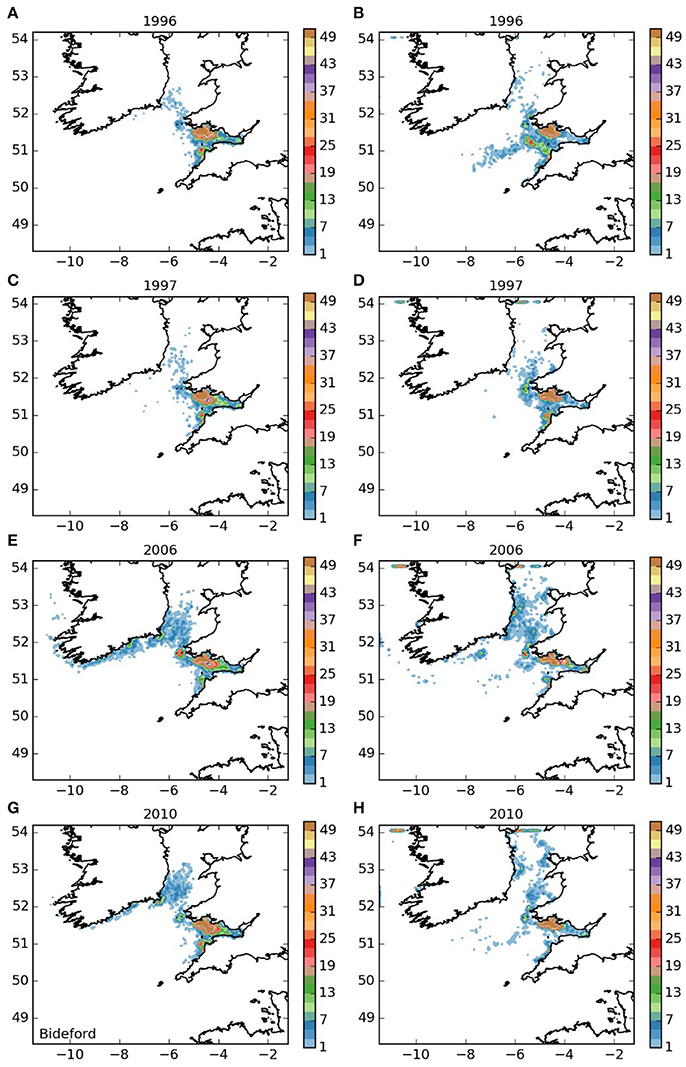
Figure 6. Final number of particles per model grid cell (density) for particles released from Bideford to Foreland Point MCZ for each of the 4 years. (A,C,E,G) 5 months pelagic duration, (B,D,F,H) 12 months pelagic duration.
Larvae released off the Lizard (area 5, Figure 7) displayed a settling pattern very similar to those from the near-by Isles of Scilly. However, some remained in the English Channel, suggesting limited potential for a self-sustaining element to the population off the Lizard, which would be particularly dependent on short-duration larvae. Finally, most of the larvae from the Padstow Bay area (area 6, Figure 8) were also transported to the same areas as those from the Isles of Scilly. A proportion, however, remained in the Bristol Channel, and settled off the south coast of Wales and the north coast of Cornwall, with some evidence of self-seeding.
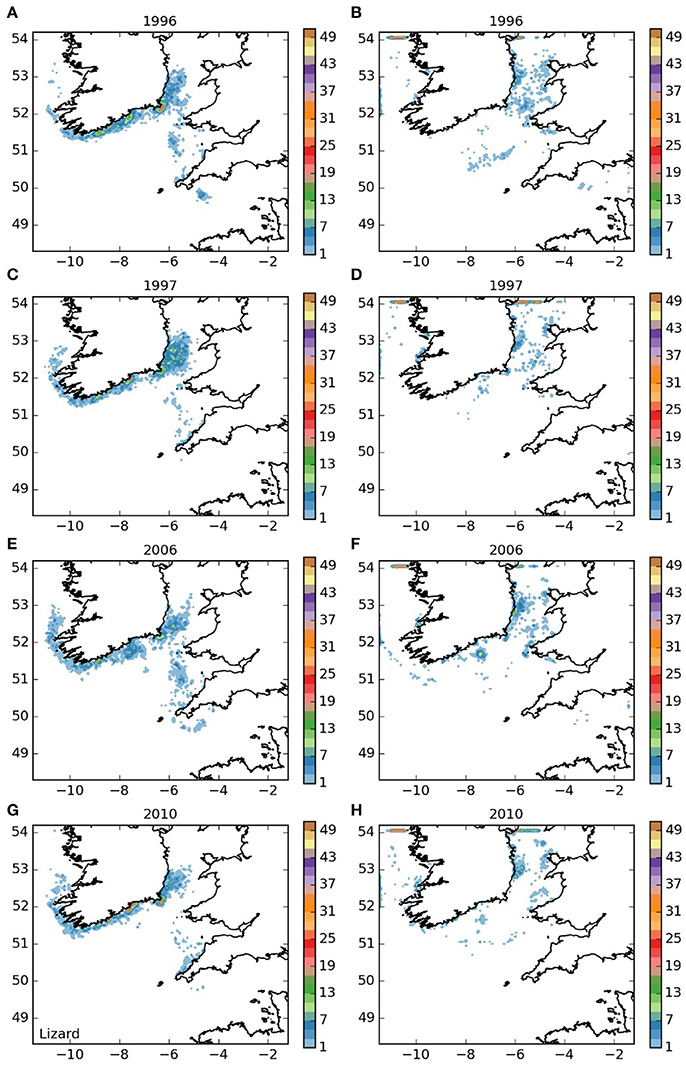
Figure 7. Final number of particles per model grid cell (density) for particles released from Lizard Point SAC for each of the 4 years. (A,C,E,G) 5 months pelagic duration, (B,D,F,H) 12 months pelagic duration.
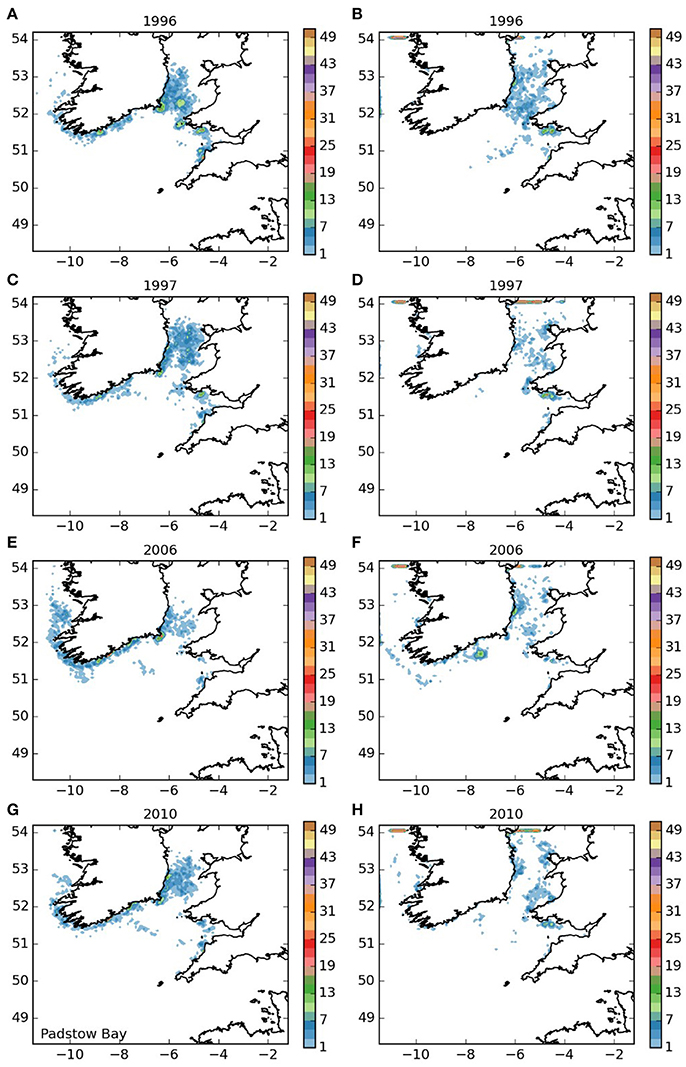
Figure 8. Final number of particles per model grid cell (density) for particles released from Padstow Bay and Surrounds MCZ for each of the 4 years. (A,C,E,G) 5 months pelagic duration, (B,D,F,H) 12 months pelagic duration.
Figures 9, 10 represent the potential connectivity between particle release sites and the surrounding network of MPAs for larvae with both a 5 and 12 month PLD. The figures are an aggregation of the 4 years assessed during this study. The figures demonstrate both the potential for regional and far-field connectivity between release sites, in particular with Irish MPAs. Note that the connectivity between the Skerries Bank and Surrounds MCZ and South Wight Maritime (Figure 10B) is an artifact of the latter being located on the boundary of the particle tracking region.
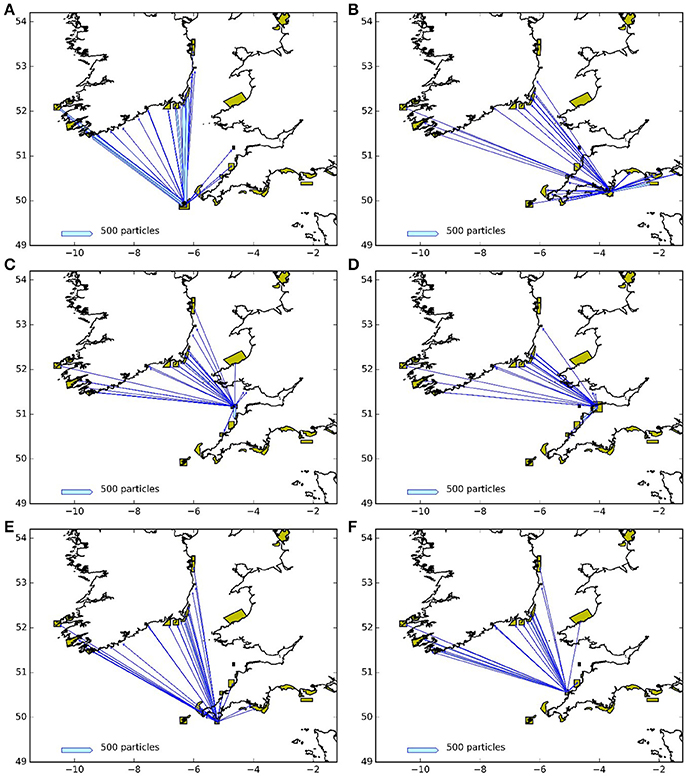
Figure 9. Connectivity between release areas and MPAs (green polygons) for the particles with 5 month larval duration: (A) Isles of Scilly SAC, (B) Skerries Bank and Surrounds MCZ, (C) Lundy SAC, (D) Bideford to Foreland Point MCZ, (E) The Lizard Point SAC, (F) Padstow Bay and Surrounds MCZ. The width of the arrows is proportional to the number of particles.
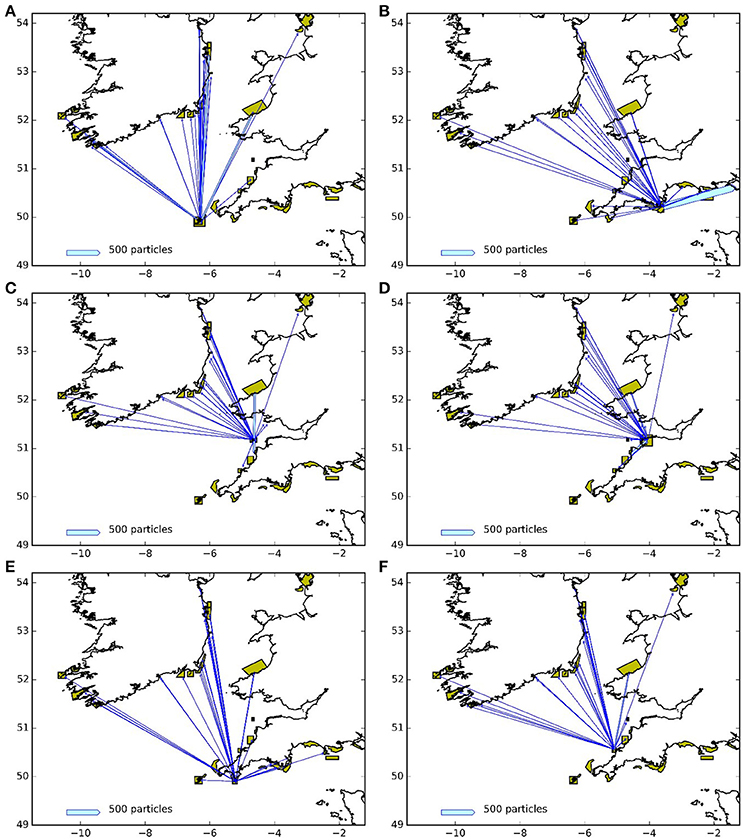
Figure 10. Connectivity between release areas and MPAs (green polygons) for the particles with 12 month larval duration: (A) Isles of Scilly SAC, (B) Skerries Bank and Surrounds MCZ, (C) Lundy SAC, (D) Bideford to Foreland Point MCZ, (E) The Lizard Point SAC, (F) Padstow Bay and Surrounds MCZ. The width of the arrows is proportional to the number of particles.
Discussion
Comparison of modeled and observed tides, and of the modeled residual circulation with observations (Brown et al., 2003) and an earlier model (Young et al., 2004) indicated that the model provides a reasonable representation of the regional hydrodynamics and is suitable for regional-scale (tens to hundreds of km) particle tracking experiments (Appendix A in Supplementary Materials). It is possible that the simulations overlook local, topography-driven retention mechanisms, both in the release areas and along the coasts because the model does not capture the local-scale (up to 10 km) bathymetry and associated hydrodynamics in detail. Hence, a proportion of larvae, particularly those that come very near to the coast, may not travel as far as suggested by the model.
Substantial numbers of particles, in particular those with 12 month larval duration, were taken out of the computation at the northern edge of the modeled domain. These larvae would have traveled further north, potentially reaching more northern parts of Ireland and Scotland. Particles were also taken out of the computation at the domain boundary in the English Channel. However, because as the next known populations of P. elephas found in Norway, it is likely that these larvae would be lost to the population or settle in suitable habitats encountered during their protracted PLD. It is likely that these larvae would be lost to the population or settle in suitable habitats encountered during their protracted PLD. In reality, most larvae are likely to have a pelagic duration between the extremes of 5 and 12 months, and hence would travel some intermediate distance. However, as the final particle density and MPA connectivity patterns of these two extremes are not dissimilar, they can still be used as a fair indication of actual larval dispersal.
For the model experiments, both for simplicity and because of the lack of more detailed information, particle releases were distributed evenly in time. In reality, larval release is likely to be a more complex function of time, potentially with one or more release peaks. If multiple, unevenly distributed release events were combined with the fixed larval durations used in this study it would likely result in slightly more concentrated final particle densities than our results using even particle release patterns.
A more natural distribution of larval duration would be expected to smooth the concentration of settling densities observed for the 5- and 12-month duration results presented here.
The larval dispersal model was run for four separate and contrasting years which were selected based upon species catch data. Differences in inter-annual dispersal patterns were apparent for some sites (Figures 3–8) but appear to be release-site specific with no clear differences in overall inter-annual dispersal pattern being observed across 6 of the 7-release sites. The exception was the Skerries Bank and Surrounds MCZ release site where, in 2010 (Figure 4), more particles appear to remain distributed throughout the western Channel. If the assumptions made, which include a 7–9-year development time and that larval recruitment is a key determining factor in cohort size, are correct then this could corroborate anecdotal evidence from fishermen and divers of increased numbers of spiny lobsters currently being landed and observed in the region.
The larval developmental and behavioral parameterization file used in the model was based on existing literature (Bouvier, 1914; Mercer, 1973; Kittaka and Ikegami, 1988; Kittaka et al., 2001; Goñi and Latroutte, 2005; Bermudes and Ritar, 2008; Groeneveld et al., 2013). When the PLD was set at the shortest duration of 5 months, many of the P. elephas populations demonstrated self-seeding. However, when the larval PLD was set at the maximum duration of 12 months, fewer of the P. elephas populations demonstrated the ability to self-seed. This emphasizes the importance of and the requirement for accurate data and life history information when setting up model parameterization files to ensure that the model outputs are realistic and plausible. If models are to be used in the development of future fisheries spatial management and conservation strategies then outputs need to be considered carefully and in conjunction with other information including fecundity, mortality rates, habitat preferences, and ecological interactions pertaining to the species in question (Butler, 2003; Graham et al., 2008).
The management and conservation of shellfish stocks within the UK is primarily based on effort and landings data (Mallol et al., 2014) and rarely considers how targeted populations are connected with one another (Kough et al., 2013). The protracted PLD of many shellfish species is a key factor in determining dispersal patterns and population connectivity (Palero et al., 2008). When dealing with distinct stocks and fragmented populations of key shellfish species biophysical modeling is a valuable tool capable of illustrating larval dispersal patterns, recruitment hotspots and population connectivity (Babbucci et al., 2010). It is therefore critical that in the future, outputs from such models are used to develop truly spatial fisheries management and future conservation initiatives.
The populations of P. elephas included in this study demonstrated varying dependencies relating to larval supply and the ability to self-seed as would be expected when the concept of sink and source populations is considered (Lipcius et al., 2008, 2015). Several of the populations of P. elephas, primarily those released from Skerries Bank and Surrounds MCZ, Lundy SAC, Bideford to Foreland Point MCZ and Padstow Bay and Surrounds MCZ (Figures 4–6, 8 respectively) demonstrated an ability to self-seed while other populations including the Isles of Scilly SAC and Lizard Point SAC (Figures 3, 7 respectively) were clearly dependent on other local and more distant populations.
These findings are aligned with current studies that have demonstrated that invertebrate species that exhibit long larval dispersal phases probably exist as metapopulations made up of connected subpopulations rather than discrete populations (Lipcius et al., 1997, 2001; Puckett and Eggleston, 2016). This suggests that when developing site-specific conservation strategies, the network scale should be considered, especially if there is an assumption that sites are ecologically connected. An example of this is the current inshore fishery for P. elephas that exists around the Isles of Scilly, of which much of the fishing effort occurs within the Isles of Scilly SAC. It is clear from the larval dispersal model that this population is potentially an extremely important source of larvae for other areas which may be disrupted without effective local fisheries management.
The current approach to the management of the Isles of Scilly spiny lobster fishery, although successful at the local scale, does not guarantee the continued supply of larvae to this population. Based on the outputs from the larval dispersal model, the Isles of Scilly population of P. elephas is a source population with very limited evidence of self-recruitment and as such appears to be reliant on other P. elephas populations for its larval supply. It is highly likely that if current populations of P. elephas around the Isles of Scilly and the southwest of England were to decrease further, local fisheries management and conservation strategies being implemented would come under scrutiny, when in fact, management and conservation strategies being implemented elsewhere could be at fault. This highlights the importance of managing fisheries across their full potential geographic range (Planes et al., 2009) and the need to manage species such as P. elephas in a way that takes into account their whole life cycle, prioritizing the stages of the cycle which could have the greatest benefits in terms of future recruitment. Failure to do so would not only result in the loss of a commercially sustainable fishery but has the potential for wide ranging effects on the distribution of P. elephas throughout the southwest of England, Ireland and Wales.
The conservation network considered here included MCZs and SACs that had either been designated for the conservation of P. elephas or contained suitable P. elephas habitat. The extent to which the MPAs are potentially connected (Figures 9, 10) in terms of larval dispersal emphasizes the role that the existing MPA network, if managed correctly, could have in the future enhancement and management of existing P. elephas populations through increased biomass exportation and habitat conservation (Crowder et al., 2000; Tuck and Possingham, 2000; Garcia-Charlton et al., 2008; Di Lorenzo et al., 2016). This is particularly apparent for the MPAs located on the North Cornwall coast and within the Bristol Channel where a large proportion of the released particles remain within the local area during both the modeled 5 and 12 month PLD.
It is clear that the design and management of MPAs can have implications on how the overall network performs. For example, evidence shows that increasing the size of MPAs can influence larval retention and that increasing the number of MPAs within a network can increase inter-reserve connectivity (Puckett and Eggleston, 2016). Due to this connectivity, failure to protect a feature or manage an activity at just one key site could have unforeseen effects on the overall conservation network (Edwards et al., 2010; Kininmonth et al., 2011). It is also important to remember that one size does not fit all and that whilst designing conservation networks requires consideration of a multitude of factors, species population connectivity is of the utmost importance.
Recommendations
Our study demonstrates the requirement for further research and additional modeling and empirical studies to:
1. Increase our understanding of the lifecycles and ecology of key fisheries species which will in turn increase our ability to successfully manage commercially important species which exist as discrete yet ecologically connected populations.
2. Increase our understanding of the true levels and scale of connectivity that potentially exist between discrete populations of vulnerable species and across existing MPA networks.
3. To further inform future network scale management approaches.
Author Contributions
PW, DH, and JV: conceived and developed the idea; JV: Carried out the modeling work; PW, JV, DH, and DF: Verified the model outputs and put them into context; DF, CT, and SC: All provided real time data and observations to help validate the model outputs. All authors discussed the results and contributed to the final manuscript.
Funding
This work was partially supported by a grant from the Defra Biodiversity Impacts and Evidence Group, a grant from the European Maritime and Fisheries Fund, the Isles of Scilly Council, Isles of Scilly IFCA, Cornwall IFCA and Devon and Severn IFCA.
Conflict of Interest Statement
The authors declare that the research was conducted in the absence of any commercial or financial relationships that could be construed as a potential conflict of interest.
Acknowledgments
The model runs and analysis were carried out under Cefas contract code C7522. ECMWF and BADC are thanked for making the atmospheric forcing available. BODC provided the tidal harmonic constituents from current meter data, www.bodc.ac.uk/data/information_and_inventories/edmed/report/581/, NW European Shelf Tidal Current Constituent Data Bank (1970–1988). River runoff data from a range of sources was processed for input into the model by Sonja van Leeuwen. Natural England are thanked for providing information on the designated features within Marine Protected Areas. Thanks also to Peter Mitchell (Cefas) for providing simplified boundary files of the Marine Protected Areas used in this study and to Alex Callaway (Cefas) and Michelle-Mary Moorton (Isles of Scilly) for undertaking very thorough and valuable reviews of earlier drafts of this manuscript. We would also like to thank the Isles of Scilly Fishermen's Association for information provided on the P. elephas population and fishery of the Isles of Scilly.
Supplementary Material
The Supplementary Material for this article can be found online at: https://www.frontiersin.org/articles/10.3389/fmars.2018.00058/full#supplementary-material
Footnotes
1. ^Available online at: http://jncc.defra.gov.uk/page-5155, (Accessed June, 2017).
2. ^Available online at: http://www.marlin.ac.uk/biotic/browse.php?sp=4113 (Accessed 14 June, 2017).
3. ^Available online at: https://designatedsites.naturalengland.org.uk/Marine/MarineSiteDetail.aspx?SiteCode=MCZ0008-03&SiteName=&countyCode=&responsiblePerson (Accessed June 2017).
4. ^Marine Management Organization (MMO) Fisheries Activity Internal Database (2017). (Accessed 10 May, 2017).
5. ^Available online at: https://www.gov.uk/government/collections/uk-sea-fisheries-annual-statistics (Accessed June, 2017).
References
Amengual-Ramis, J. F., Vazquez-Archdale, M., Canovas-Perez, C., and Morales-Nin, B. (2016). The artisanal fishery on the spiny lobster Palinurus elephas in Cabera National Park, Spain: comparative study in traditional and modern traps with trammel nets. Fish. Res. 179, 23–32. doi: 10.1016/j.fishres.2016.01.022
Ayata, S. D., Lazure, P., and Thiebaut, E. (2010). How does the connectivity between populations mediate range limits of marine invertebrates? A case study of larval dispersal between the Bay of Biscay and the English channel (North-East Atlantic). Prog. Oceanogr. 87, 18–36. doi: 10.1016/j.pocean.2010.09.022
Babbucci, M., Buccoli, S. M., Cau, A., Cannas, R., Go-i, R., Diaz, D., et al. (2010). Population structure, demographic history, and selective processes: contrasting evidences from mitochondrial and nuclear markers in the European spiny lobster Palinurus elephas (Fabricus, 1787). Mol. Phylogenet. Evol. 56, 1040–1050. doi: 10.1016/j.ympev.2010.05.014
Balmaseda, M. A., Trenberth, K. E., and Kallen, E. (2013). Distinctive climate signals in reanalysis of global ocean heat content. Geophys. Res. Lett. 40, 1754–1759. doi: 10.1002/grl.50382
Beraud, C., van der Molen, J., Armstrong, M., Hunter, E., Fonseca, L., and Hyder, K. (2017). The influence of oceanographic conditions and larval behaviour on settlement success - the European sea bass Dicentrarchus labrax (L.). ICES J. Mar. Sci. doi: 10.1093/icesjms/fsx195. [Epub ahead of print].
Bermudes, M., and Ritar, A. J. (2008). Response of early stage spiny lobster Jasus edwardsii phyllosoma larvae to changes in temperature and photoperiod. Aquaculture 281, 63–69. doi: 10.1016/j.aquaculture.2008.05.035
Berrisford, P., Dee, D. P., Poli, P., Brugge, R., Fielding, K., Fuentes, M., et al. (2011). The ERA-interim archive Version 2.0. ERA Report Series 1, ECMWF, Reading 23pp.
Bouvier, M. E. L. (1914). Recherches sur le developpement post-embryonnaire de la langouste commune (Palinurus vulgaris). J. Mar. Biol. Assoc. U.K. 10, 179–193. doi: 10.1017/S0025315400007700
Brown, J., Carillo, L., Fernand, L., Horsburgh, K. J., Hill, A. E., Young, E. F., et al. (2003). Observations of the physical structure and seasonal jet-like circulation of the Celtic Sea and St George's Channel of the Irish Sea. Cont. Shelf Res. 23, 533–561. doi: 10.1016/S0278-4343(03)00008-6
Burchard, H., and Bolding, K. (2002). GETM – a general estuarine transport model, scientific documentation, Technical Report. EUR 20253 EN, European Commission, Ispra, Italy.
Burchard, H., Bolding, K., and Villareal, M. R. (1999). GOTM—a General Ocean Turbulence Model, Theory, Applications and Test Cases, Technical Report. EUR 18745 EN, European Commission.
Butler, M. J. (2003). Incorporating ecological process and environmental change into spiny lobster population models using a spatially-explicit individual-based approach. Fish. Res. 65, 63–79. doi: 10.1016/j.fishres.2003.09.007
Connell, J. (1985). The consequences of variation in initial settlement vs. post settlement mortality in rocky intertidal communities. J. Exp. Mar. Biol. Ecol. 93, 11–45. doi: 10.1016/0022-0981(85)90146-7
Crowder, L. B., Lyman, S. J., Figueira, W. F., and Priddy, J. (2000). Source-Sink population dynamics and the problem of siting marine reserves. Bull. Mar. Sci. 66, 799–820.
Dee, D. P., Uppala, S. M., Simmons, A. J., Berrisford, P., Poli, P., Kobayashi, S., et al. (2011). The ERA-interim reanalysis: configuration and performance of the data assimilation system. Q. J. R. Meteorol. Soc. 137, 553–597. doi: 10.1002/qj.828
Di Lorenzo, M., Claudet, J., and Guidetti, P. (2016). Spillover from marine protected areas to adjacent fisheries has an ecological and a fishery component. J. Nat. Conserv. 32, 62–66. doi: 10.1016/j.jnc.2016.04.004
Edwards, H. J., Elliott, I. A., Pressey, R. L., and Mumby, P. J. (2010). Incorporating ontogenetic dispersal, ecological process and conservation into reserve design. Biol. Conserv. 143, 457–470. doi: 10.1016/j.biocon.2009.11.013
Fitzgibbon, Q. P., and Battaglene, S. C. (2012). Effect of water temperature on the development and energetics of early, mid and late-stage phyllosoma larvae of spiny lobster Sagmariasus verreauxi. Aquaculture 344–349, 153–160. doi: 10.1016/j.aquaculture.2012.03.008
Garcia-Charlton, J. A., Perez-Ruzafa, A., Marcos, C., Claudet, J., Badalamenti, F., Benedetti-Ceechi, L., et al. (2008). Effectiveness of European Atlanto-Mediterranean MPAs: do they accomplish the expected effects on populations, communities and ecosystems? J. Nat. Conserv. 16, 193–221. doi: 10.1016/j.jnc.2008.09.007
Goñi, R., and Latroutte, D. (2005). Review of the biology, ecology and fisheries of Palinurus spp. Species of European waters: Palinurus elephas (Fabricius, 1787) and Palinurus mauritanicus (Gruvel, 1911). Cah. Biol. Mar. 46, 127–142.
Goñi, R., Quetglas, A., and Renones, O. (2003). Size at maturity, fecundity and reproductive potential of a protected population of spiny lobster Palinurus elephas (Fabricius 1787) from the Western Mediterranean. Mar. Biol. 143, 583–592. doi: 10.1007/s00227-003-1097-5
Graham, E. M., Baird, A. H., and Connolly, S. (2008). Survival dynamics of scleractinian coral larvae and implications for dispersal. Coral Reefs 27, 529–539. doi: 10.1007/s00338-008-0361-z
Groeneveld, J. C., Go-i, R., and Diaz, D. (2013). “Chapter 11: Palinurus Species,” in Lobsters: Biology, Management, Aquaculture and Fisheries, 2nd Edn, ed B. Philips (Chichester, UK: John Wiley & Sons LTD), 326–356.
Hepper, B. T. (1977). The fishery of crawfish, Palinurus elephas, off the coast of Cornwall. J. Mar. Biol. Assoc. U.K. 57, 925–941. doi: 10.1017/S0025315400026023
Holthuis, L. B. (1991). FAO Species Catalogue. Vol 13. Marine Lobsters of the World. An Annotated and Illustrated Catalogue of Species of Interest to Fisheries Known to date. FAO Fisheries Synopses 125, Vol 13. Rome: FAO.
Hunter, E. (1999). Biology of the European spiny lobster, Palinurus elephas (Fabricius 1787) (Decapod, Palinuroidea). Crustaceana 72, 545–565. doi: 10.1163/156854099503609
Hunter, E., Shackely, S. E., and Bennett, D. B. (1996). Recent studies on the crawfish Palinurus elephas in South Wales and Cornwall. J. Mar. Biol. Assoc. U.K. 76, 963–983. doi: 10.1017/S0025315400040911
Hunter, J. R., Craig, P. D., and Philips, H. E. (1993). On the use of random walk models with spatially variable diffusivity. J. Comput. Phys. 106, 366–376. doi: 10.1016/S0021-9991(83)71114-9
IPCC. (2014). Climate Change 2014: Synthesis Report. Contributing to Working Groups I, II and III to the Fifth Assessment Report of the Intergovernmental Panel on Climate Change. Geneva: IPCC.
Kininmonth, S., Beger, M., Bode, M., Peterson, E., Adams, V. M., Dorfman, D., et al. (2011). Dispersal connectivity and reserve selection for marine conservation. Ecol. Modell. 222, 1272–1282. doi: 10.1016/j.ecolmodel.2011.01.012
Kittaka, J. (1997). Application of ecosystem culture method for complete development of phyllosomas of spiny lobster. Aquaculture 155, 319–331. doi: 10.1016/S0044-8486(97)00100-2
Kittaka, J., and Ikegami, E. (1988). Culture of the Palinurid Palinurus elephas from egg stage to puerulus. Nippon Suisan Gakkaishi 54, 1149–1154. doi: 10.2331/suisan.54.1149
Kittaka, J., Kudo, R., Onoda, S., Kanemaru, K., and Mercer, J. P. (2001). Larval culture of the European spiny lobster Palinurus elephas. Mar. Freshw. Res. 52, 1439–1444. doi: 10.1071/MF01188
Kough, A. S., Paris, C. B., and Butler, M. J. (2013). Larval connectivity and the international management of fisheries. PLoS ONE 8:e64970. doi: 10.1371/journal.pone.0064970
Lenhart, H. J., Mills, D. K., Baretta-Bekker, H., van Leeuwen, S. M., van der Molen, J., Baretta, J. W., et al. (2010). Predicting the consequences of nutrient reduction on the eutrophication status of the North Sea. J. Mar. Syst. 81, 148–170. doi: 10.1016/j.jmarsys.2009.12.014
Le Provost, C., Lyard, F., Genco, M. L., and Rabilloud, F. (1998). A hydrodynamic ocean tide model improved by assimilation of a satellite altimeter-derived data set. J. Geophys. Res. 103, C3, 5513–5529. doi: 10.1029/97JC01733
Lipcius, R. N., Burke, R. P., Mculloch, D. N., Schreiber, S. J., Schulte, D. m., Seitz, R. D., et al. (2015). Overcoming restoration paradigms: value of the historical record and metapopulation dynamics in native oyster restoration. Front. Mar. Sci. 2:65. doi: 10.3389/fmars.2015.00065
Lipcius, R. N., Eggleston, D. B., Schreiber, S. J., Seitz, R. D., Shen, J., Sisson, M., et al. (2008). Importance of metapopulation connectivity to restocking and restoration of marine species. Rev. Fish. Sci. 16, 101–110. doi: 10.1080/10641260701812574
Lipcius, R. N., Stockhausen, W. T., and Eggleston, D. B. (2001). Marine reserves for Caribbean spiny lobster: empirical evaluation and theoretical metapopulation dynamics. Mar. Freshw. Res. 52, 1589–1598. doi: 10.1071/MF01193
Lipcius, R. N., Stockhausen, W. T., Eggleston, D. B., Marshall, L. S. Jr, and Hickey, B. (1997). Hydrodynamic decoupling of recruitment, habitat quality and adult abundance in the Caribbean spiny lobster: source-sink dynamics? Mar. Freshw. Res. 48, 807–815. doi: 10.1071/MF97194
Mallol, S., Diaz, D., Sobrado, F., and Go-i, R. (2014). First V-Notching experience of a spiny lobster: V-Notch recovery and impact on health and growth of Palinurus elephas. J. Crustacean Biol. 34, 25–30. doi: 10.1163/1937240X-00002203
Matsuda, H., Abe, F., and Tanaka, S. (2012). Effect of photoperiod on metamorphosis from phyllosoma larvae to puerulus post-larvae in the Japanese spiny lobster Panulirus japonicas. Aquaculture 326–329, 136–140. doi: 10.1016/j.aquaculture.2011.11.027
Mercer, J. P. (1973). Studies on the Spiny Lobster Crustacean, Decapod, Palinuridae of the West Coast of Ireland, with Particular Reference Palinurus elephas Fabricius 1973. Thesis, University College Galway.
Mogensen, K., Alonso Balmaseda, M., and Weaver, A. (2012). The NEMOVAR Ocean Data Assimilation System as Implemented in the ECMWF Ocean Analysis for System4. ECMWF Technical Memorandum 668, Toulouse.
Palero, F., Abello, P., Macpherson, E., Gristina, M., and Pascual, M. (2008). Phylogeography of the European spiny lobster (Palinurus elephas): influence of current oceanographical features and historic processes. Mol. Phylogenet. Evol. 48. 708–711. doi: 10.1016/j.ympev.2008.04.022
Planes, S., Jones, G. P., and Thornold, S. R. (2009). Larval dispersal connects fish populations in a network of marine protected areas. Proc. Natl. Acad. Sci. U.S.A. 106, 5693–5697. doi: 10.1073/pnas.0808007106
Puckett, B. J., and Eggleston, D. B. (2016). Metapopulation dynamics guide marine reserve design: importance of connectivity, demographics, and stock enhancement. Ecosphere 7:e01322. doi: 10.1002/ecs2.1322
Sandifer, P. A. (1975). The role of pelagic larvae in recruitment to populations of adult decapod crustaceans in the York River estuary and adjacent lower Chesapeake Bay, Virginia. Estuarine Coastal Mar. Sci. 3, 269–279. doi: 10.1016/0302-3524(75)90028-6
Shanks, A., Grantham, B., and Carr, M. (2003). Propagule dispersal distance and the size and spacing of marine reserves. Ecol. Appl. 13, 159–169. doi: 10.1890/1051-0761(2003)013[0159:PDDATS]2.0.CO;2
Tiessen, M. C. H., Fernand, L., Gerkema, T., van der Molen, J., Ruardij, P., and van der Veer, H. W. (2014). Numerical modelling of physical processes governing larval transport in the southern North Sea. Ocean Sci. 10, 357–376. doi: 10.5194/os-10-357-2014
Tuck, G. N., and Possingham, H. P. (2000). Marine protected areas for spatially structured exploited stocks. Mar. Ecol. Prog. Ser. 192, 89–101. doi: 10.3354/meps192089
Underwood, A. J., and Fairweather, P. G. (1989). Supply-side ecology and benthic marine assemblages. Trends Ecol. Evol. 4, 16–21. doi: 10.1016/0169-5347(89)90008-6
Van der Molen, J., Ruardij, P., and Greenwood, N. (2016). Potential environmental impact of tidal energy extraction in the Pentland Firth at large spatial scales: results of a biogeochemical model. Biogeosciences 13, 2593–2609. doi: 10.5194/bg-13-2593-2016
Van der Molen, J., Ruardij, P., and Greenwood, N. (2017). A 3D SPM model for biogeochemical modelling, with application to the northwest European continental shelf. J. Sea Res. 127, 63–81. doi: 10.1016/j.seares.2016.12.003
Van der Molen, J., van Beek, J., Augustine, S., Vandsteenbrugge, L., van Walraven, L., Langenberg, J., et al. (2015). Modelling survival and connectivity of Mnemiopsis leidyi in the southern North Sea and Scheldt estuaries. Ocean Sci. 11, 405–424. doi: 10.5194/os-11-405-2015
Visser, A. W. (1997). Using random walk models to simulate the vertical distribution of particles in a turbulent water column. Mar. Ecol. Prog. Ser. 158, 275–281. doi: 10.3354/meps158275
Wolk, F. (2003). Three-Dimensional Lagrancian Tracer Modelling in Wadden Sea Areas, Diploma thesis, Carl von Ossietzky University Oldenburg, Hamburg.
Keywords: spiny lobster (Palinurus elephas), larval dispersal, conservation measures, fisheries management, marine protected areas
Citation: Whomersley P, Van der Molen J, Holt D, Trundle C, Clark S and Fletcher D (2018) Modeling the Dispersal of Spiny Lobster (Palinurus elephas) Larvae: Implications for Future Fisheries Management and Conservation Measures. Front. Mar. Sci. 5:58. doi: 10.3389/fmars.2018.00058
Received: 24 August 2017; Accepted: 09 February 2018;
Published: 06 March 2018.
Edited by:
Romuald Lipcius, Virginia Institute of Marine Science, United StatesReviewed by:
Patricia Briones-Fourzan, Universidad Nacional Autónoma de México, MexicoKevin Alexander Hovel, San Diego State University, United States
Copyright © 2018 Whomersley, Van der Molen, Holt, Trundle, Clark and Fletcher. This is an open-access article distributed under the terms of the Creative Commons Attribution License (CC BY). The use, distribution or reproduction in other forums is permitted, provided the original author(s) and the copyright owner are credited and that the original publication in this journal is cited, in accordance with accepted academic practice. No use, distribution or reproduction is permitted which does not comply with these terms.
*Correspondence: Paul Whomersley, cGF1bC53aG9tZXJzbGV5QGNlZmFzLmNvLnVr