- 1Clinical Sciences, College of Veterinary Medicine, North Carolina State University, Raleigh, NC, United States
- 2Molecular and Biomedical Sciences, College of Veterinary Medicine, North Carolina State University, Raleigh, NC, United States
- 3James Cook University, Townsville, QLD, Australia
- 4Galapagos Science Center, Universidad San Francisco de Quito, Quito, Ecuador
- 5Galapagos National Park, Galapagos, Ecuador
- 6Vet Rocket LLC, Santa Clara, CA, United States
- 7Chester Springs, Pennsylvania, PA, United States
- 8Department of Biology, University of North Carolina at Chapel Hill, Chapel Hill, NC, United States
Effective conservation of marine megafauna requires a thorough understanding of the ecology, physiology, population dynamics, and health of vulnerable species. Assessing the health of large, mobile marine animals poses particular challenges, in part because the subjects are difficult to capture and restrain, and in part because standard laboratory and diagnostic tools are difficult to apply in a field setting. Radiography is a critically important diagnostic tool used routinely by veterinarians, but it has seldom been possible to image live marine vertebrates in the field. As a first step toward assessing the feasibility of incorporating radiography into studies of vulnerable species in remote locations, we used portable radiographic equipment to acquire the first digital internal images of living marine iguanas, Amblyrhynchus cristatus, an iconic lizard endemic only to the Galápagos Islands of Ecuador. The radiographic machinery was powered by batteries and performed well on a rocky beach environment of an uninhabited island, despite high heat and humidity. The accuracy of radiographic measurements was validated by computing a snout-vent length (SVL) using bone dimensions and comparing this to standard measurements of SVL made externally with a tape measure. These results demonstrate the feasibility of using radiography to study animals in remote sites, a technique that may prove useful for a variety of physiological, ecological, and biomechanical studies in which reliable measurements of skeletal and soft-tissue dimensions must be acquired under challenging field conditions. Refinements are discussed that will help the technology reach its full potential in field studies.
Introduction
Conserving marine megafauna requires an interdisciplinary, holistic approach that incorporates information from disparate fields, including ecology, physiology, population dynamics, and veterinary science. The need for effective means of assessing the health of vulnerable populations has gained urgency in recent years, as climate change and other factors facilitate the spread of new pathogens, pollutants, and diseases, sometimes at alarming rates (Harvell et al., 1999, 2002; Salazar and Denkinger, 2010; Vinueza et al., 2014). At the same time, evaluating the health of large, mobile marine vertebrates poses daunting challenges because the subjects are difficult to capture and restrain, cannot be transported to a laboratory, and standard diagnostic tools are often impossible to use in a field setting (Seilern-Moy et al., 2017).
Among techniques used widely in health assessments of animals, radiography is a critically important diagnostic tool that remains the most common method for imaging internal anatomy (Banzato et al., 2013; Thrall, 2018). Radiography is an established and clinically important way to assess bone integrity and health through the visualization of bones and internal organs (Mitchell, 2002; Banzato et al., 2013; Thrall, 2018), and can also sometimes be used to non-invasively investigate diet (Banzato et al., 2013; Erlacher-Reid et al., 2013; Beckmann et al., 2015). Despite these advantages, the large size of conventional radiography equipment has typically precluded imaging live marine vertebrates in the field.
As an initial step toward evaluating the feasibility of incorporating radiography into studies of vulnerable species in remote locations, we used portable radiography to acquire the first digital internal images of living marine iguanas, Amblyrhynchus cristatus, while working on an uninhabited island in the Galápagos archipelago of Ecuador. In this article we discuss the promise associated with field radiography by highlighting our success in acquiring bone measurements that can be used to independently reproduce standard body length measurements in iguanas. We also discuss the limitations that must be overcome for the technology to realize its full potential in future research.
Materials and Methods
Ethics Statement
This study was conducted on Isla Lobos, a small island separated by a few 100 m from San Cristóbal Island in the Galápagos archipelago of Ecuador. It was carried out as part of a population health assessment authorized by the Galápagos National Park Service (Permit # MAE-DPNG/DGA-2016-0107 to G.A. Lewbart and K.J. Lohmann) and approved by the Universidad San Francisco de Quito ethics and animal handling protocol. All handling and sampling procedures were consistent with standard vertebrate protocols and veterinary practices and were approved by the North Carolina State University and the University of North Carolina Chapel Hill IACUC Committees.
Iguana Capture and Sampling
Seven adult-sized marine iguanas were captured by hand among lava rocks within 25 m of the shore on Isla Lobos (0°51′23.91″ S; 89°34′08.66″W) over a period of 2 h. After capture, each animal was quickly transported to an area shaded by a tarp and located ~100 m from the ocean, where the iguana was measured, weighed, and radiographed. Animals that had not been captured and microchipped during a previous year were microchipped in the left pelvic femoral muscle prior to release (Pellett and Cope, 2013). The lizards were released once all measurements had been taken and all resumed normal activity and behavior within minutes.
Iguana Measurements and Sex Determination
A flexible measuring tape was used to measure along the ventral aspect of the animal, from the most rostral extent of the snout to the mid aspect of the vent (snout-vent length/SVL) and from the most rostral aspect of the snout to the caudal-most portion of the tail (total body length/TBL). Measurements were made to the nearest 0.5 cm. Body weight was measured with a digital scale (precision: 0.1 kg).
The sex of each iguana was determined on the basis of external sexual dimorphism, primarily by femoral pore size and hemipene swellings, and by probing of the hemipene sacs.
Radiography
Digital radiography (Vet Rocket X1, Santa Clara, California 95050, USA) was performed in the field under a protective tarp to reduce light and glare. The radiographic unit weighs 10 kg and has a battery life of 4 h. The detector is capable of recording 140 exposures on its 6.5 h battery. Dorsoventral views were obtained with the animals awake, in sternal recumbency on the digital imaging plate, and aligned as well as possible in field conditions. Images included the snout to the level of the vent (the entire tail was not imaged). To identify the vent (which can be soft tissue or gas opaque depending on contents), a 0.5 cm stainless steel ball bearing was taped externally to the mid-aspect of the vent. A 20 cm (distance between two 0.5 cm diameter ball bearings) calibration bar was placed next to each animal to provide a known standard to correct for magnification on radiographic images.
Radiographic measurements were made on DICOM (Digital Imaging Communication) files (an interoperable system) using Horos (Version 2.0.2, 64 bit) by a veterinary radiologist (EBC). All dorsoventral radiographs were calibrated to the 20 cm calibration bar placed on the digital imaging plate adjacent to each individual iguana (Figure 1). Calibration was performed using dimensions between the leading edges of the calibration ball bearings. Two methods of measurement were used to measure SVL. The first involved measuring SVL from the most rostral extent of the snout soft tissues, using interconnecting linear regions of interest (ROI). Regions of interest were placed to bisect the skull and vertebrae, with as many ROIs as needed to reach the leading edge of the ball bearing placed at the vent. This method was used to account for angulation of the spine due to positioning or deformity.
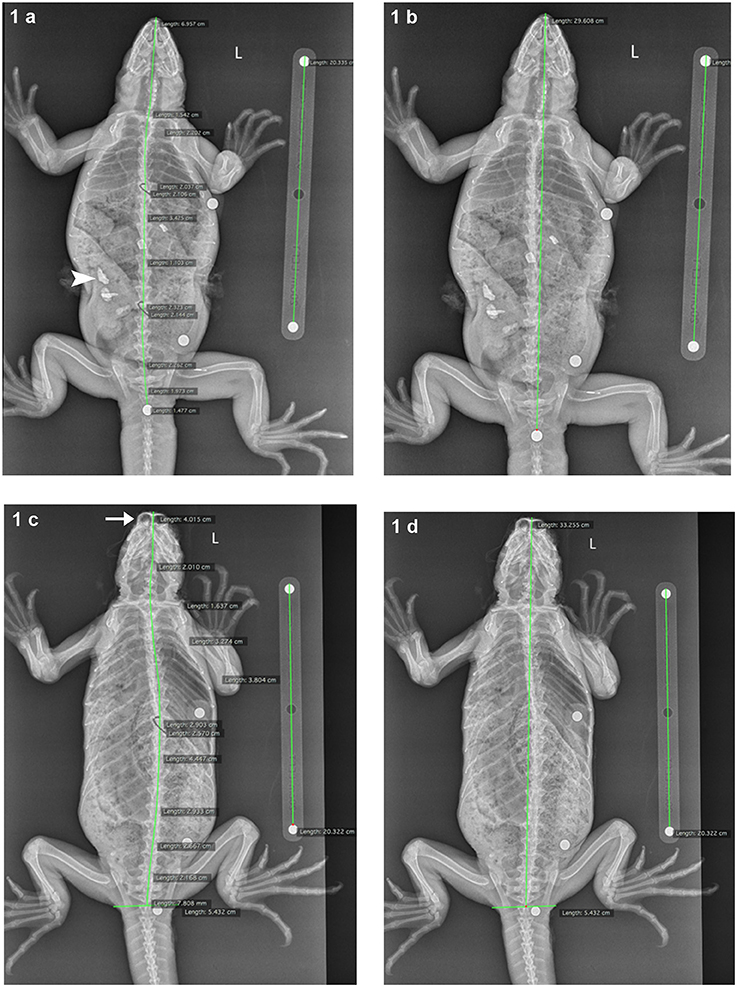
Figure 1. Dorsoventral radiographs of marine iguanas, demonstrating the measurements methods. The 20 cm calibration bar is visible adjacent to each iguana (top: 4653144B3E; bottom: no microchip ID). In Method 1 (a) snout-vent length (SVL) was measured using interconnected linear (regions of interest) ROIs bisecting the skull and vertebrae; this technique corrected for small angulations in the spine from the leading edge of the snout to the leading edge of the BB at the vent. In Method 2 (b) SVL was measured using a single linear ROI from snout to vent BB, with no correction for spinal angulation. (c,d) demonstrate the adjustment of these two measurement methods if the vent BB was not located so that it centrally bisected the vertebra at this level. In such cases, a transverse linear ROI was placed at the leading edge of the vent BB, and the caudal-most linear ROI measuring SVL was extended to the central aspect of this transverse ROI (Method 1 adjusted = c, Method 2 adjusted = d). In (a) angular mineral opaque fragments are visible within the gastrointestinal tract (arrowhead), consistent with ingested substrate. In (c) the frontal sinuses (arrow) are prominently visible, indicating foreshortening of the iguana, secondary to head position.
The second method involved a single linear ROI from the rostral extent of the snout soft tissues to the leading edge of the vent ball bearing, with no correction for spinal malangulation. If the vent ball bearing was not central within the regional soft tissues in a line bisected by linear ROI bisecting the vertebrae, a transverse line was placed at the leading edge of the vent ball bearing, and the caudal-most linear ROIs were extended to the center of the transverse line to mimic the center of the vent. Radiographs were also subjectively assessed with respect to radiographic technique, animal positioning (no foreshortening of the rostrum/snout), and for any body system abnormalities.
Statistical Analysis
Two non-parametric statistical tests were used to compare the tape measure with the measurements made using either of the radiographic methods. The Spearman correlation was used to measure the strength of association between the measurement pairs (whether the two measurements tend to change together or not), while the Wilcoxon test was used to determine if the pairs were statistically the same or not. All statistical analysis were performed in R (version 3.4.3, The R Foundation for Statistical Computing).
Results
Iguana Demographics and Health Status
Measurements were taken from seven marine iguanas (five males, one female, and one individual of unknown sex). The mean tape measure SVL for the entire group was 32.3 cm (range 29.0–35.6 cm). The mean tape measure total body length (tip of nose to tip of tail) was 79.4 cm (range 72.9–84.4 cm). All of the animals appeared to be alert and responsive. In addition, all appeared to have gastrointestinal tracts that contained ingested material (based on palpation, overall appearance, and radiographic results).
Radiographic Analysis
Radiographs of all seven marine iguanas were evaluated (Figures 1, 2). Radiographic technique was diagnostic (adequate for health assessment) in all of them. No skeletal pathology was identified and two iguanas had open physes. The gastrointestinal tracts of all sampled iguanas were at least somewhat full and included material that was radiographically mineral opaque (radio-opaque). In three iguanas, positioning was favorable for easily assessing SVL. In the others, foreshortening of the rostrum/snout (due to animal head/neck position) complicated the process slightly. The radiographs of the latter group were characterized by increased visibility of the frontal sinuses.
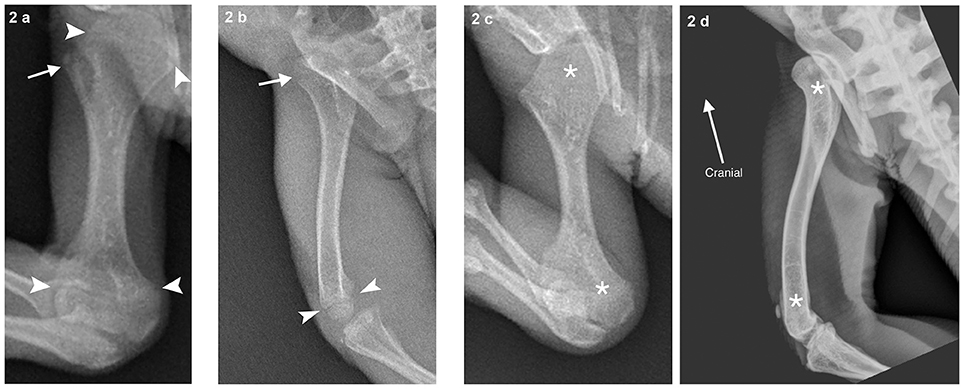
Figure 2. (a) An individual with open proximal and distal humeral physes (growth plates; indicated by arrowheads) evidenced by linear lucencies separating the metaphyses (transition from diaphysis to physis/growth plate) and epiphyses (end of the long bone that supplies the articular surface of the joint). The apohysis (separate center of ossification for attachment of soft tissue structures) of the greater tubercle is also open (arrow). (b) Demonstrates an individual (464E22425F) with visible open distal femoral physis (arrowheads) and proximal femoral physis of the femoral head (arrow). (c) (4669670B06 humerus, asterisks) and (d) (green iguana left femur, asterisks) shows an individual with closed physes in these bones for comparison.
Visual inspection of the data suggests that both types of radiography measurements are qualitatively comparable to, and correlate with, the tape measurements (Figure 3). Results of the non-parametric Spearman correlation tests between tape measure and either of the radiographic methods indicate that both pairs of measurements were significantly correlated (rs = 0.79, p < 0.05). The non-parametric Wilcoxon tests also confirmed that there is no statistical difference between the tape measure and either radiographic method one or two (in both cases p < 0.05).
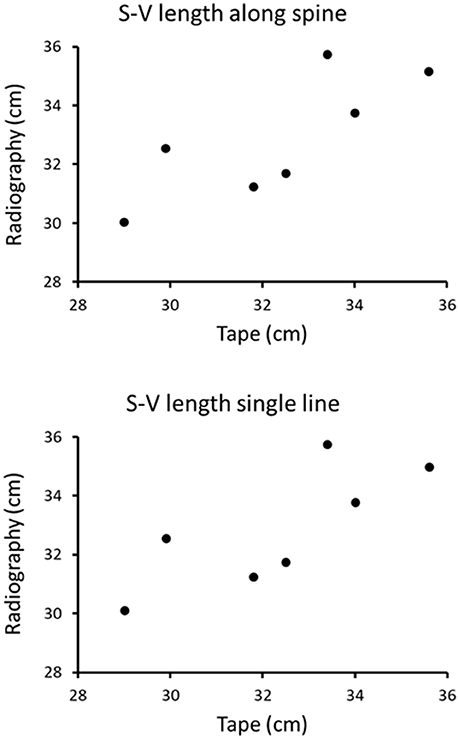
Figure 3. Snout-vent length of marine iguanas measured using tape compared to the snout-vent length measured using radiography along the vertebral column (Top) and using a single measurement line (Bottom).
Discussion
Our results demonstrate the feasibility of using radiography to examine live animals in a remote, field setting. We were able to acquire the first digital radiographs of marine iguanas while working under field conditions on an uninhabited island in the Galápagos archipelago. Moreover, measurements of SVL acquired using digital radiography and axial skeletal length were found to be equivalent to those obtained using a tape measure on the outside of the iguana's body. Despite the suboptimal rostral/snout position of some animals, no difference was detected between conventional measurements made using a tape, and those based on either of two radiographic measurement techniques. Our findings indicate that both radiographic measurement methods (straight line and partitioned) are essentially equivalent and that both yield accurate measurements.
Anatomical Observations
Although our primary goal was to assess whether accurate measurements of skeletal structures can be obtained with field radiography, our study highlights the potential for radiography to also uncover biological insights in species that cannot easily be transported to a laboratory. For example, the finding that some marine iguanas have closed long bone physes is noteworthy, given the reported capacity for reptiles to grow throughout life (Castanet, 1994). Moodie (1908) suggested that marine iguanas might have more epiphyses than any other lizard and that the humerus alone may have nine epiphyseal centers. It is possible that marine iguanas possess more bone plasticity than other iguanids (Moodie, 1908) and that this feature applies to the vertebra as well as the long bones.
In addition, radiography can sometimes provide a non-invasive way to investigate diet (Schumacher and Toal, 2001; Erlacher-Reid et al., 2013; Beckmann et al., 2015). Mineral opaque material in the gastrointestinal tract is apparent in several of the iguanas (Figures 1a,b). Although the composition of this material is not known, one possibility is that the mineral debris represents substrate such as lava rock that was inadvertently ingested as iguanas grazed on underwater plants attached to rocks (Wikelski et al., 1993).
Although the results of our initial study are encouraging, our attempts to use radiography in the field revealed some limitations that must be considered in the context of future studies. For example, although the radiographic methods appear viable for assessing SVL, a single dorsoventral radiographic view is not sufficient for investigating individual skeletal elements. The view selected in our study was chosen for ease of use with awake iguanas in a field setting, but a drawback is that while the ventral SVL is optimized against the image detector (thus limiting magnification), other skeletal structures that are farther from the imaging plate are not accurately represented. Thus, the dorsally convex curvature of the vertebral column, concave margins of the caudal vertebral endplates, and the cranially convex margin of the cranial vertebral endplates all contribute to a lack of discrimination of the endplate margins, and individual vertebral length cannot be accurately assessed. The curvature of the vertebral column also results in small, but different degrees of magnification, which are not corrected for by a single 20 cm calibration bar on the imaging plate. In the future we recommend placing the animal in lateral recumbency and using the obtained images to quantify the axial skeletal elements.
For marine iguanas, one interesting application of field radiography in the future might be to investigate the mechanisms that enable these animals to reduce their body length during periods of extreme food deprivation (Wikelski and Thom, 2000). The mechanism for this shrinkage is unknown, but is thought to involve shortening of the axial skeleton or at least portions thereof (Wikelski and Thom, 2000). It is also unknown whether this is exclusively a skeletal phenomenon, or if it also involves associated soft tissues. It is possible that multiple radiographic measurements of individuals over time could help explain how marine iguanas are able to shrink, although such an undertaking would require precise measurements of individual bone lengths to ensure accuracy and repeatability.
Another potentially interesting use of field radiography is to investigate changes in the structure of invertebrate shells and exoskeletons as climate change alters the acidity of the ocean (Byrne, 2011; Aranda and Manzano, 2017). Recent studies have revealed that animals with body structures composed of calcium carbonate, including many crustaceans, molluscs, and corals, are affected by the pH of ocean water; in some cases exoskeletons can become thinner and more brittle, while in others the exoskeleton can thicken (Orr et al., 2005; Aranda and Manzano, 2017). Such changes can potentially be detected with field radiography, facilitating the assessment of climate change impacts of animal physiology in remote locations around the world.
Finally, having field radiography can potentially support targeted health assessments in remote populations of nearly any species that can be restrained and handled for a few seconds. Digital field radiography can be used to assess bone and mineral density, diagnose fractures, detect anatomical anomalies, and locate foreign bodies like fish hooks or bullets. The technique can also be used to locate microchips, surgically-implanted internal radio transmitters with depleted batteries, or other implanted devices.
In the present study we successfully obtained high quality radiographs from wild, unsedated marine iguanas in the field. The method allowed us to reveal skeletal health status and obtain accurate measurements of the vertebral column and head. We further confirmed that marine iguanas appear to consistently ingest rock and/or calcium carbonate. Although improvements in technique are needed for the technology to achieve its full promise, the availability of field radiography lays the foundation for a wealth of interesting new applications relevant to basic research, conservation biology, and health assessments of vulnerable populations.
Author Contributions
GL: Conceived and designed the experiments, performed the experiments, analyzed the data, wrote sections of the paper, reviewed drafts of the paper, acting as corresponding author. EC: Conceived and designed the experiments, analyzed the data, wrote sections of the paper, prepared figures and/or tables, reviewed drafts of the paper. MH: Conceived and designed the experiments, analyzed the data, reviewed drafts of the paper. JM-P: Performed the experiments, analyzed the data, facilitated the field work, reviewed drafts of the paper. JG: Performed the experiments, facilitated the field work, reviewed drafts of the paper. AF: Performed the experiments, analyzed the data, wrote sections of the paper, reviewed drafts of the paper. EPC: Analyzed the data, wrote sections of the paper, reviewed drafts of the paper. KL: Conceived and designed the experiments, analyzed the data, wrote sections of the paper, reviewed drafts of the paper.
Funding
Atlantic Productions Inc. provided financial and logistical support and Vet Rocket Inc. LLC donated equipment and supplies.
Conflict of Interest Statement
The Vet Rocket X1 was used to perform this work and AF works for Vet Rocket Inc. LLC.
The other authors declare that the research was conducted in the absence of any commercial or financial relationships that could be construed as a potential conflict of interest.
Acknowledgments
We wish to thank the following people for their support and assistance: Daniela Alarcón-Ruales, Jason Guillermo Castañeda, Eduardo Espinoza, Alexander Gaos, Carlos Mena, Philip Page, Kent Passingham, Sylvia Sotamba, Stephen Walsh, Manolo Yépez, and Maryuri Yépez. In addition, we thank the Galápagos National Park (GNP), Galápagos Science Center (GSC), and Atlantic Productions, Inc.
References
Aranda, D. A., and Manzano, N. B. (2017). Effects of near-future-predicted ocean temperatures on early development and calcification of the queen conch Strombus gigas. Aquacult. Int. 25, 1869–1881. doi: 10.1007/s10499-017-0153-y
Banzato, T., Hellebuyck, T., Van Caelenberg, A., Saunders, J. H., and Zotti, A. (2013). A review of diagnostic imaging of snakes and lizards. Vet. Rec. 173, 43–49. doi: 10.1136/vr.101450
Beckmann, M. C., Gilliam, J. F., and Langerhans, R. B. (2015). X-ray imaging as a time-saving, non-invasive technique for diet analysis. Fish. Res. 161, 1–7. doi: 10.1016/j.fishres.2014.05.015
Byrne, M. (2011). Impact of ocean warming and ocean acidification on marine invertebrate life history stages: vulnerabilities and potential for persistence in a changing ocean. Oceanogr. Mar. Biol. 49, 1–42. doi: 10.1201/b11009-2
Castanet, J. (1994). Age estimation and longevity in reptiles. Gerontology 40, 174–192. doi: 10.1159/000213586
Erlacher-Reid, C., Norton, T. M., Harms, C. A., Thompson, R., Reese, D. J., Walsh, M. T., et al. (2013). Intestinal and cloacal strictures in free-ranging and aquairum-maintained green sea turtles (Chelonia mydas). J. Zoo Wild Med. 44, 408–429. doi: 10.1638/2012-0071R.1
Harvell, C. D., Burkholder, J. M., Colwell, R. R., Epstein, P. R., Grimes, D. J., Hofmann, E. E., et al. (1999). Emerging marine diseases, climate links and anthropogenic factors. Science 285, 1505–1510. doi: 10.1126/science.285.5433.1505
Harvell, C. D., Mitchell, C. E., Ward, J. R., Altizer, S., Dobson, A. P., Ostfeld, R. S., et al. (2002). Climate warming and disease risks for terrestrial and marine biota. Science 296, 2158–2162. doi: 10.1126/science.1063699
Mitchell, M. (2002). Diagnosis and management of reptile orthopedic injuries. Vet. Clin. North Am. Exot. Anim. Pract. 5, 97–114. doi: 10.1016/S1094-9194(03)00048-3
Orr, J. C., Fabry, V. J., and Aumont, O. (2005). Anthropogenic ocean acidification over the twenty-first century and its impact on calcifying organisms. Nature 437, 681–686. doi: 10.1038/nature04095
Pellett, S., and Cope, I. (2013). Identification using microchips in exotic species. Comp. Anim. 18, 172–176. doi: 10.12968/coan.2013.18.4.172
Salazar, S., and Denkinger, J. (2010). Possible effects of climate change on the populations of Galapagos pinnipeds. Galap. Res. 67, 45–49.
Schumacher, J., and Toal, R. L. (2001). Advanced radiography and ultrasonography in reptiles. Sem. Avian Exot. Pet Med. 10, 162–168. doi: 10.1053/saep.2001.24671
Seilern-Moy, K., Vielgrader, H., Gerritsmann, H., and Walzer, C. (2017). Radiography in the field: assessing a lightweight, handheld, battery-powered dentistry unit for field diagnostic applications. J. Zoo Wild Med. 48, 31–39. doi: 10.1638/2016-0034.1
Thrall, D. E. (2018). Textbook of Veterinary Diagnostic Radiology, 7th Edn. Philadelphia, PA: Elsevier, 1000.
Vinueza, L. R., Menge, B. A., Ruiz, D., and Palacios, D. M. (2014). Oceanographic and climatic variation drive top-down/bottom-up coupling in the Galápagos intertidal meta-ecosystem. Ecol. Monogr. 84, 411–434. doi: 10.1890/13-0169.1
Wikelski, M., and Thom, C. (2000). Marine iguanas shrink to survive El Ni-o. Nature 403, 37–38. doi: 10.1038/47396
Keywords: marine iguana, Amblyrhynchus cristatus, radiography, shrinking, skeletal anatomy
Citation: Lewbart GA, Cohen EB, Hirschfeld M, Muñoz-Pérez JP, García J, Fu A, Chen EP and Lohmann KJ (2018) Field-Based Radiographic Imaging of Marine Megafauna: Marine Iguanas (Amblyrhynchus cristatus) as a Case Study. Front. Mar. Sci. 5:40. doi: 10.3389/fmars.2018.00040
Received: 30 September 2017; Accepted: 26 January 2018;
Published: 13 February 2018.
Edited by:
Peter H. Dutton, National Oceanic and Atmospheric Administration (NOAA), United StatesReviewed by:
Antonios D. Mazaris, Aristotle University of Thessaloniki, GreecePhil J. Bouchet, University of Western Australia, Australia
Copyright © 2018 Lewbart, Cohen, Hirschfeld, Muñoz-Pérez, García, Fu, Chen and Lohmann. This is an open-access article distributed under the terms of the Creative Commons Attribution License (CC BY). The use, distribution or reproduction in other forums is permitted, provided the original author(s) and the copyright owner are credited and that the original publication in this journal is cited, in accordance with accepted academic practice. No use, distribution or reproduction is permitted which does not comply with these terms.
*Correspondence: Gregory A. Lewbart, Z3JlZ19sZXdiYXJ0QG5jc3UuZWR1