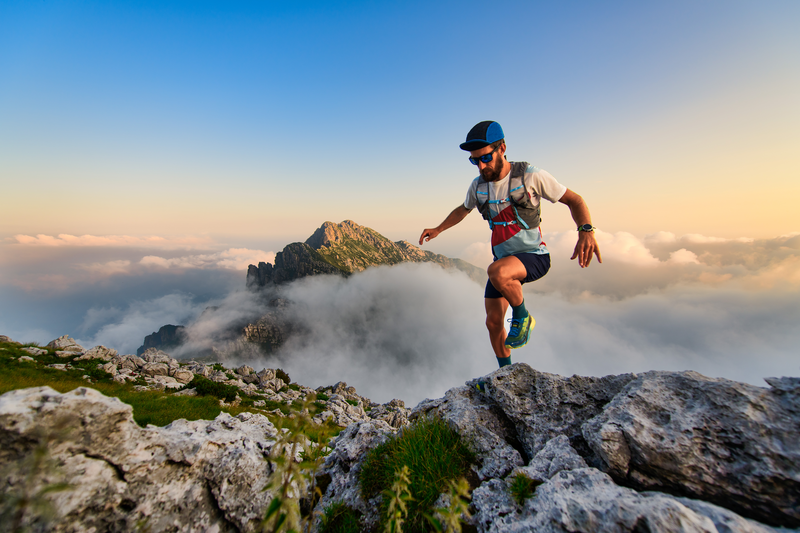
94% of researchers rate our articles as excellent or good
Learn more about the work of our research integrity team to safeguard the quality of each article we publish.
Find out more
ORIGINAL RESEARCH article
Front. Mar. Sci. , 19 February 2018
Sec. Marine Pollution
Volume 5 - 2018 | https://doi.org/10.3389/fmars.2018.00039
This article is part of the Research Topic Impacts of Marine Litter View all 15 articles
A correction has been applied to this article in:
Corrigendum: Frequency of Microplastics in Mesopelagic Fishes from the Northwest Atlantic
Microplastics are a ubiquitous pollutant in our seas today and are known to have detrimental effects on a variety of organisms. Over the past decade numerous studies have documented microplastic ingestion by marine species with more recent investigations focussing on the secondary impacts of microplastic ingestion on ecosystem processes. However, few studies so far have examined microplastic ingestion by mesopelagic fish which are one of the most abundant pelagic groups in our oceans and through their vertical migrations are known to contribute significantly to the rapid transport of carbon and nutrients to the deep sea. Therefore, any ingestion of microplastics by mesopelagic fish may adversely affect this cycling and may aid in transport of microplastics from surface waters to the deep-sea benthos. In this study microplastics were extracted from mesopelagic fish under forensic conditions and analysed for polymer type utilising micro-Fourier Transform Infrared Spectroscopy (micro-FTIR) analysis. Fish specimens were collected from depth (300–600 m) in a warm-core eddy located in the Northwest Atlantic, 1,200 km due east of Newfoundland during April and May 2015. In total, 233 fish gut contents from seven different species of mesopelagic fish were examined. An alkaline dissolution of organic materials from extracted stomach contents was performed and the solution filtered over a 0.7 μm borosilicate filter. Filters were examined for microplastics and a subsample originating from 35 fish was further analysed for polymer type through micro-FTIR analysis. Seventy-three percent of all fish contained plastics in their gut contents with Gonostoma denudatum having the highest ingestion rate (100%) followed by Serrivomer beanii (93%) and Lampanyctus macdonaldi (75%). Overall, we found a much higher occurrence of microplastic fragments, mainly polyethylene fibres, in the gut contents of mesopelagic fish than previously reported. Stomach fullness, species and the depth at which fish were caught at, were found to have no effect on the amount of microplastics found in the gut contents. However, these plastics were similar to those sampled from the surface water. Additionally, using forensic techniques we were able to highlight that fibres are a real concern rather than an artefact of airborne contamination.
As a consequence of decades of marine litter entering our seas (Ryan, 2015), microplastics have been found in coastal and pelagic environments around the globe with an ever increasing distribution (Barnes et al., 2009; Galgani et al., 2015). Considering the prevalence of microplastics, there is now a substantial amount of research effort investigating their abundance in the gastrointestinal tracts of various organisms. For example, some early studies found 83% of Nephrops norvegicus had microplastics in their tracts (Murray and Cowie, 2011) and Lusher et al. (2013) found microplastics in the tracts of 35% of the pelagic and demersal fish species examined. Indeed, Gall and Thompson (2015) have reported that over 690 marine species are impacted by marine litter. More recent studies have moved from quantifying which animals have ingested microplastics to examining the physical and health implications of microplastic ingestion (Rochman et al., 2013; Cole et al., 2014; Pedà et al., 2016). For example, Wright et al. (2013) demonstrated how the ingestion of microplastics by the polychaete Arenicola marina (lugworm), an important ecosystem engineer of Northern Europe's intertidal zones, caused inflammation and decreased feeding and ultimately depleted energy reserves. Such studies have prompted researchers to investigate the impact on ecosystem processes. Indeed, Cole et al. (2016) found that microplastics alter the sinking rates of copepod faecal pellets if ingested and in consequence may affect the downward flux of carbon to the ocean floor. With the increasing evidence that microplastics represent an ecosystem and environmental health concern, UNEP and the EU Commission have established bodies and efforts to guide in decision making and legislation (Galgani et al., 2013; UNEP, 2016). Furthermore, several governments have taken legislative steps by introducing a ban on microbeads in cosmetics and detergents by 2020 (Sutherland et al., 2017).
Despite this substantial increase in studies investigating the ingestion of microplastics and their associated impacts, there are still important taxa playing key roles in ecosystem functioning that have not been well-studied. Mesopelagic fish inhabiting the disphotic zone of the pelagic realm (200–1,000 m depth) from the Arctic to the Antarctic (Gjøsaeter and Kawaguchi, 1980) are one of these understudied groups. Many species are known to undergo diurnal vertical migrations by residing at depth during the day before migrating to the surface at night to feed (Gjøsaeter and Kawaguchi, 1980). Smaller mesopelagic fish such as Myctophum punctatum and Benthosema glaciale feed by filtering zooplankton, predominantly copepods, euphausiids, amphipods, eggs, and larvae over their gill rakers (Scotto di Carlo et al., 1982; Roe and Badcock, 1984). Larger mesopelagic fish such as Stomias boa and Serrivomer beanii also actively target decapods and fish using their anterior vertebrae and branchial apparatus to swallow larger prey (Roe and Badcock, 1984; Bauchot, 1986). Thus, mesopelagic fish are exposed to microplastics either through the direct consumption of a microplastic mistakenly identified as prey item, or indirectly, through the consumption of a prey item (e.g., copepod or euphausiid) that had already consumed microplastics.
As mesopelagic fish undergo large vertical migrations, they are known to play a key role in the cycling of carbon and nutrients to the deep ocean (Radchenko, 2007; Davison et al., 2013). For instance, Radchenko (2007) has shown that such species in the Bering Sea transport 15,000 tonnes of carbon daily to the deep ocean. Therefore, the ingestion of microplastics by mesopelagic fish may disrupt carbon cycling and aid in the transport of microplastics to deeper waters, as suggested by Lusher et al. (2016).
The importance of mesopelagic fish was recently further highlighted in studies by Kaartvedt et al. (2012) and Irigoien et al. (2014) who found that the mesopelagic fish biomass in the global oceans may have previously been underestimated by at least one order of magnitude due to avoidance behaviour and mesh extrusion. Because they make up such a large biomass in the pelagic realm they provide an important food source for a variety of predatory fish and marine mammals which, through trophic transfer from their mesopelagic fish prey, may suffer from the impacts of microplastics and associated toxins (Lusher et al., 2016). Some of the species preying on mesopelagic fish such as tuna and swordfish (Scott and Tibbo, 1968; Varela et al., 2013) are commercially important food sources and thus toxins and microplastics transferred to these species may also pose a danger to human health. To date mesopelagic fish have not been exploited as a human food source due to the high levels of wax esters in their tissue (Gjøsaeter and Kawaguchi, 1980). This may change in the near future as the demand for fish protein increases and new policies (e.g., Blue Growth Strategy by the European Union) encourage sustainable exploitation of potential resources (St. John et al., 2016). Furthermore, the food safety issues concerned with microplastics and the associated toxin exposure through the consumption of commercially exploited fish have recently been outlined in an extensive report by the Food and Agriculture Organization of the United Nations drawing attention to the potential threat of microplastics to human health (Lusher et al., 2017).
However, to date, only a few studies have investigated microplastic ingestion by mesopelagic fish: one in the North Atlantic (Lusher et al., 2016) and two in the North Pacific Gyre region (Boerger et al., 2010; Davison and Ash, 2011). Since then, new and improved methodologies for microplastic extraction have been developed with an emphasis on ultra-clean techniques in order to prevent airborne contamination (Wesch et al., 2017).
This study set out to quantify microplastic ingestion by mesopelagic fish from an eddy region in the Northwest Atlantic, known to be a hot spot for mesopelagic fish (McKelvie, 1985; Fennell and Rose, 2015) and potentially microplastics (Yu et al., 2018). Specifically, this study investigated whether: (1) species, stomach fullness, and the depth at which fish were caught at had an effect on the amount of microplastics found in the gut contents of mesopelagic fish, and (2) how the type, shape, and size of microplastics found in the gut contents compared to those found in the surface waters. Importantly, we applied strict measures to prevent microplastic contamination during extraction and identified microplastic type using micro-FTIR spectroscopy.
Mesopelagic fish samples were collected during a Northern Atlantic crossing (CE15007) from Galway, Ireland to St. John's, Newfoundland aboard the RV Celtic Explorer between the 20th of April and the 5th of May 2015. In total, eight 30-min pelagic trawls were carried out during daylight hours at a towing speed of 4 knots (Figure 1). The opening of the net was fitted with a Scanmar depth sensor to enable three trawls to be conducted in the upper mesopelagic zone between 300 and 350 m (shallow) and five in the lower mesopelagic zone between 500 and 650 m (deep). Once hauled aboard, a random subsample of 35 intact mesopelagic fish was taken from each trawl.
Figure 1. Trawl locations during CE15007 survey aboard the RV Celtic Explorer; red rectangular box in the inset corresponds to outer figure margin.
Furthermore, surface water samples were taken during each trawl by utilising the ship's underway water pumping system with its intake located at 3 m depth. The intake water initially passed through a 1 mm mesh and was then pumped into the lab facilities, where the underway hose was positioned to allow water to pass through a 180 μm plankton sieve. Sieved particles were then washed down with 0.2 μm filtered ultrapure water into cylindrical aluminium containers (5 cm Ø) which were then folded over at the top. The flow rate of water through the underway pump was measured at 10 L min−1 and the volume of water filtered was estimated using the flow rate and duration of each trawl. Fish and water samples were stored in Ziploc® bags and immediately transferred into a −20°C freezer and stored there until the vessel returned to Galway on the 15th of May 2015 when samples were transferred to a −20°C freezer at the National University Ireland, Galway.
Fish were taken dead from midwater trawls carried out to ground truth the backscatter from a Simrad EK60 scientific echo sounder investigating the deep scattering layer in the Northwest Atlantic, and are thus exempt from ethical approval, dealing with regulated animals, that is live vertebrates and higher invertebrates.
Samples were processed between September 2015 and June 2016. Fish samples were defrosted ~3 h prior to processing and identified by counting number of dorsal, pectoral, and anal fin rays and number of gill rakers as well as prominent features such as photophores and barbels (Marine Species Identification Portal, 2015). Fish which displayed visible physical damage to their digestive tract were excluded from analysis. The standard length (to the nearest millimetre) of each fish was recorded. Fish were rinsed with 0.2 μm filtered MilliQ™ water (18.2 MΩ cm−1) (Millipore, Bedford, USA) and weighed (to the nearest 0.0001 g) before being transferred into a borosilicate container located inside a laminar flow hood (AirClean600®: ISO class 5) where part of their alimentary tract, the oesophagus to the duodenum, was extracted. The extracted alimentary tract was then opened and the gut contents emptied into 20 ml borosilicate scintillation vials and the alimentary tract lining thoroughly washed with 0.2 μm filtered MilliQ™. The removed alimentary tract and the dissected fish were then weighed (to the nearest 0.0001 g) to obtain gut contents weight. Vials containing gut contents were filled with MilliQ™ water and sodium hydroxide (Certified analytical reagent for analysis, Fisher Scientific, UK) to give a 1 M concentration and subsequently incubated at room temperature for 24 h, following an effective and cost efficient microplastic extraction protocol outlined by Cole et al. (2014). Water samples were processed in a similar fashion whereby the frozen contents of the aluminium containers were emptied into glass scintillation vials and organic materials digested also using a 1 M solution of sodium hydroxide solution over 24 h.
After incubation, vial contents were filtered over borosilicate filters (42 mm Ø, 0.7 μm mesh) using a vacuum pump and Büchner flask; filters were then rinsed with 200 ml of 0.2 μm filtered MilliQ™ water to rinse sodium hydroxide from the filter and retained particles. Filters were kept in borosilicate glass petri dishes, covered with a lid and examined for microplastics using an Olympus SZX16 stereo microscope (Olympus, SZX16) with a digital camera attached (Olympus, DP17). Once all potential microplastics were identified on the filter, the glass lid was removed and potential plastics were examined and manually manipulated to confirm polymer characteristics (brittleness, softness, transparency). Plastic particle colours and sizes were recorded (to the nearest μm) using CellSense Standard software package (Olympus, version 1.2). Two microplastic fibres were gold coated (Emitech K550, Quorum Technologies Ltd., West Sussex, United Kingdom) and subjected to scanning electron microscopy (SEM) in secondary electron mode using a Hitachi model S-4700 (Hitachinaka, Japan). The analyses were performed at an acceleration voltage of 20 kv, an emission current (Ic) of 10 μA and a working distance of 12 mm (Morrison et al., 2009).
Five individuals of each species were randomly selected and microplastics originating from their gut contents, as well as those originating from one randomly selected surface water sample, were further analysed for polymer identification using micro-Fourier-transform infrared spectroscopy (micro-FTIR). The absorbance for each polymer was obtained using a Perkin Elmer Spotlight 200i FT-IR Microscopy System (Perkin Elmer, USA) at 100 μm−1 resolution with spectra collected over the wavenumber range of 7,800–600 cm−1.
The extraction of microplastics and subsequent examination of the filters was performed in compliance with the most recent findings in microplastic contamination prevention methodologies (Woodall et al., 2015; Wesch et al., 2017). All equipment used was pre-rinsed with 0.2 μm filtered MilliQ™ water and all clothing worn during laboratory work was of non-polymer nature. Furthermore, samples and filters were not at any time air exposed and always kept under a clean air laminar flow hood (HEPA filter, class ISO5) or maintained within covered borosilicate petri dishes. During dissections and filtrations on each day a wet filter (blank) was kept in a borosilicate petri dish inside the laminar flow hood for control purposes. After filtration of all samples on each day the filter was then also assembled within the Büchner flask and 200 ml of 0.2 filtered MilliQ™ water were filtered through it and the filter was later assessed for microplastics for quality assurance purposes.
A stomach fullness index (FI) was calculated for each fish by dividing the weight of the gut content by the weight of the fish.
To test whether stomach fullness had any effect on microplastics being present or not in the alimentary tract of the fish, a Mann-Whitney-U-test (as the distribution of FI was non-parametric) was carried out using R (R Development Core Team, 2017) and compared the median stomach fullness value for fish that had microplastics with those that did not.
As the microplastic count data were non-parametric, a Kruskal–Wallis test (using R) was used to test whether there was any difference in the abundance of microplastics between the seven different species. A Mann–Whitney-U-test (using R), was used to test whether more microplastics were identified from fish found in shallow compared with those found in deep waters.
A total of 280 fish was captured of which 233 were examined for the presence of microplastics in their gut contents. The most common species amongst the subsampled fish were the spotted lantern fish M. punctatum (with 86 individuals, or 37% of catch), the glacier lantern fish B. glaciale (69 indiv., 29%) and the white-spotted lantern fish Diaphus rafinesquii (34 indiv., 15%). The remaining species were the Rakery beaconlamp Lampanyctus macdonaldi (16 indiv., 7%), the stout sawpalate S. beanii (14 indiv., 6%), the scaly dragonfish S. boa (9 indiv., 4%) and Gonostoma denudatum (5 indiv., 2%). Where information on sexual maturity size exists (M. punctatum, B. glaciale, D. rafinesquii, G. denudatum), every sampled fish was assessed as being sexually mature.
Overall 73% of fish contained plastics in their stomachs with G. denudatum having the highest frequency of occurrence (100%), followed by S. beanii (93%) and L. macdonaldi (75%) (Table 1). In total, 452 microplastic fragments were extracted from the fish gut contents, with an average of 1.8 microplastic fragments per fish. The highest average number of microplastics in the gut contents was recorded in S. beanii (2.36), followed by M. punctatum (2.28), and G. denudatum (2.2) (Table 1).
Table 1. Fish species, numbers and length examined for microplastic ingestion and associated microplastic abundances in gut contents.
There was no significant difference between the median stomach fullness indices of fishes which had microplastics in their stomachs and those that did not (W = 5253, P = 0.976). Furthermore, there was no significant difference in median microplastic counts among the seven different species (H = 10.904, d.f. = 6, P = 0.091), nor between fish caught in shallower and deeper waters (U = 5877, P = 0.389).
In total, 341 particles were found in the surface water samples (8 samples totalling 2,400 L of surface water) resulting in an estimated concentration of 14 microplastic fragments per 100 litres of water. Plastics identified from fish guts were very similar to those found in the surface waters (Figure 2). Ninety-eight percent of microplastics identified from the fish and 99% of those identified from the water samples were classed as fibres with the remainder being flattened fragments of plastics. Recorded microplastic colours included black, grey, blue, green, purple, red, yellow, and white. Black and blue were by far the most common colours, followed by red, making up 67, 18, and 6% and 65, 26, and 5% of sampled plastics from fish guts and surface waters, respectively. Polymers of other colours only made up a minor fraction of the particles in both cases (Figure 2). Likewise, sizes of extracted microplastics were very similar between those found in fish (mean ± SD: 969 ± 1,048) and in surface waters (mean ± SD: 985 ± 1,101). The smallest recorded polymer fragment had a length of 42 μm and the largest a length of 8,150 μm.
Figure 2. Microplastic colours (A,B), length (C,D), and type (E,F) found in mesopelagic fish gut contents (left) and surface waters (right).
Micro-FTIR analysis was successfully carried out for 118 of the 191 microplastic fragments originating from 35 fish and from one surface water sample. The 73 particles which could not be assessed for their polymer nature either fractured when pressure was applied by the diamond of the micro-FTIR machine or did not show a significant fit with any of the materials within the FTIR spectra library and thus were excluded from analysis. Polymers identified from fish and water samples were of similar polymer nature with the majority being polyethylene, followed by methyl cellulose and a relatively small proportion were identified as alginic acids. An exception was nylon, which comprised 10% of the particles found in the fish guts, but was not identified amongst the particles extracted from the surface water (Figure 2). Scanning electron microscopy images of two fibres extracted from fish gut contents had visible signs of polymer fracturing (Figure 3).
Figure 3. Light microscopy and scanning electron microscopy images of a black (A–D) and a green (E–H) microplastic fibre recovered from gut contents of Myctophum punctatum.
No microplastics were found on the filters used as blanks to ensure no airborne contamination or any contamination from the filtration equipment and procedure.
Using forensic methods, this study assessed microplastic frequency of occurrence in mesopelagic fish gut contents from a warm-core eddy in the Northwest Atlantic. We detected a significantly higher occurrence rate of 73% in contrast to previous studies reporting occurrence rates of 11% in the North Atlantic and 9 and 35% in the North Pacific Gyre regions (Boerger et al., 2010; Davison and Ash, 2011). There are several reasons which may explain our much higher frequency of occurrence. Firstly, there are no standardised methods for the extraction of microplastics from gastrointestinal tracts of fish and so different research teams have used different protocols such as visual sorting of gut contents (Boerger et al., 2010), staining of organic materials (Davison and Ash, 2011), and extraction by the use of alkaline dissolution (Lusher et al., 2016). In the latter, the authors used a similar approach to this study but used a more concentrated caustic solution (1.8 vs. 1 M) and incubated samples for a longer time period (14 days vs. 1 day). Cole et al. (2014) assessed many different extraction methodologies and found that the hydrolysis of organic compounds using a caustic solution was an efficient and viable method. However, they noted that extractions using higher concentrations and longer incubation times than recommended damaged and discoloured pH sensitive polymers such as nylon, uPVC, and polyethylene and thus these may have been underestimated previously. Furthermore, we used fine-meshed borosilicate filters in contrast to Lusher et al. (2016) who used a 250 μm filter. As a result, our study detected much smaller microplastics (down to 42 μm) which made up 20% of all detected microplastics.
Another potential explanation for differences among reported microplastic occurrence rates may be due to differences in the abundances of microplastics found in the study areas. Samples for this study were collected around a warm core eddy ~1,200 km off the Newfoundland coast which is known to be an area of enhanced biomass for all trophic levels (Dufois et al., 2016), including mesopelagic fish (McKelvie, 1985; Fennell and Rose, 2015), and may also potentially aggregate microplastics (Yu et al., 2018). Surface water samples collected within this study indeed showed 10 times higher concentrations of microplastics than reported for other regions of the Atlantic (Lusher et al., 2014) where Lusher et al. (2016) collected their samples. The other two studies collected samples at the edge region of the North Pacific Gyre, which while potentially having slightly higher concentrations of plastics, were still not located close to the centre of the gyre, known to be a hot spot for microplastics (Eriksen et al., 2014). At this point it is also important to consider how mesopelagic fish may be exposed to microplastics. All of the seven investigated species migrate to the surface at night to feed and therefore ingestion could happen through the direct consumption of microplastics mistaken as prey items or through trophic transfer from their prey species. Indeed the most common prey of mesopelagic fish are copepods, euphausiids, amphipods, larvae, and decapods and all have been reported to ingest microplastics (Carpenter et al., 1972; Setälä et al., 2014; Desforges and Ross, 2015). Lusher et al. (2016) previously excluded trophic transfer as a likely route of exposure as the average size of microplastics they found in the gut contents was 1.9 mm. This was considered too large to be ingested by their prey species but they also noted that their study only targeted plastic particles over 250 μm in size. In this study the average size of identified microplastics from fish guts was 970 μm with 20% of all plastics being smaller than 250 μm and thus trophic transfer from planktivorous prey species may indeed be a likely route of exposure. In addition to size, it is also worth noting that the colour of any microplastics is unlikely to play an important role in the ingestion of microplastics by mesopelagic fish as the colours of the microplastics identified from the fish gut contents were similar to those identified from the surface waters.
Lastly, different microplastic abundances in the gut contents may be caused by some mesopelagic species being more selective or impacted than others. For example, it is well known that some bird species are more prone to microplastic and marine litter ingestion than others (e.g., petrels: Van Franeker and Bell, 1988). However, our study found no differences in microplastic occurrence rates between the seven mesopelagic fish species examined. Neither did depth seem to explain any variation in microplastic abundances amongst individuals caught at different depths. Therefore, we can conclude that the notably higher occurrence rates reported within this study are likely due to the differences in microplastic extraction methods as well as the fact that the present study was carried out in a hot spot for mesopelagic fish and microplastics alike. While this study reports one of the highest abundances of microplastics in the gut contents of fish, other studies have reported similar results for different species, particularly in polluted areas. For example, Tanaka and Takada (2016) report a 77% encounter rate of microplastics in Japanese anchovies (Engraulis japonicus) sampled from Tokyo Bay and Nadal et al. (2016) found microplastics to occur in 68% of seabream (Boops boops) sampled around the Balearic Islands. It is also noteworthy that while Lusher et al. (2016) and Davison and Ash (2011) reported a lower average microplastic count per individual fish of 0.13 and 0.11, respectively, Boerger et al. (2010) indeed found the average microplastic count per fish to be 2.1, higher than observed by us (1.8).
Such high numbers of microplastics in the gut contents of mesopelagic fish is of great concern. Microplastics have previously been shown to adversely impact invertebrate species such as lugworms, causing weight loss, reduced feeding activity and inflammation (Besseling et al., 2013; Wright et al., 2013), and detrimental effects on the intestinal functioning of seabass (Dicentrarchus labrax) have also been noticed (Pedà et al., 2016). Furthermore, there is growing concern about the effect of chemical pollutants sorbed to microplastics. For example, Mato et al. (2001) reported up to 106 higher concentrations of PCBs on polypropylene pellets than in the surrounding sea water and recently, it has been shown that Japanese rice fish (Oryzias latipes) and rainbow fish (Melanotaenia fluviatilis) readily accumulate chemical pollutants from ingested microplastics (Rochman et al., 2013; Wardrop et al., 2016).
The ingestion of microplastics by mesopelagic fish may also have secondary implications for other species as well as the entire ecosystem. Mesopelagic fish are now known to make up a substantial biomass in the pelagic realm (Kaartvedt et al., 2012) and provide an important food source for many large predators such as dolphins, seals, and tuna as well as sea birds (Cherel et al., 2008; Danielsen et al., 2010; Spitz et al., 2010; Varela et al., 2013). These taxa consume large amounts of mesopelagic fish and consequently ingest the microplastics within them. More importantly, due to trophic transfer, predators of mesopelagic fish may also bioaccumulate chemical pollutants absorbed from ingested microplastics. As some of the species preying on mesopelagic fish are commercial exploited fish the transfer of microplastics and biaccumulated toxins may also pose a threat to human health (Lusher et al., 2017).
Mesopelagic fish are also responsible for a significant amount of carbon and nutrient cycling (Radchenko, 2007). Organic material released as faeces or from dead and decaying organisms, sink very slowly from the upper surface to the deep ocean. A large proportion of this organic material is recycled by other organisms and re-released before it can reach the ocean floor. Mesopelagic fish however, undergo diurnal migrations, quickly travelling long distances from the epipelagic layer where they feed, to the deeper ocean where they deposit their faeces. Therefore, they play a key role in speeding up the downward flux of carbon and nutrients to deeper depth and circumvent recycling by other organisms (Irigoien et al., 2014). As discussed above, we are now aware that microplastic ingestion can have substantial effects on fish health and in particular digestive functions. Therefore, reported microplastic abundances in the fish gut contents may have implications for the cycling of carbon and nutrients by these species. Moreover, as suggested by Lusher et al. (2016), mesopelagic fish may aid in the downward transport of microplastics to the deep-sea benthos and cause potential harm to organisms in this habitat.
In terms of our methods, the applied protocols have successfully extracted very small plastic particles. However, the micro-FTIR spectroscopy analysis identified a large proportion of the analysed microplastics as methyl cellulose and alginic acids. This, while seeming unusual at first, is very likely a consequence of insufficient cleaning of the microplastics after extraction. Methyl cellulose is produced synthetically by heating cellulose with a caustic solution. As we used a caustic solution to hydrolyse organic materials some of the sodium hydroxide seemed to have remained on the plastic particles and potentially skewed the absorbance spectrum. Similarly, alginic acids are likely to be a reading of an outer biofilm coating of the microplastic particles which had not been removed during extraction. For future studies we strongly recommend a more thorough cleaning of plastic particles with filtered, ultrapure water. Furthermore, it is interesting to note that despite taking a forensic approach during the extraction of microplastics (Wesch et al., 2017) we noted a large amount of fibres (98%) amongst the sampled microplastics. This is in agreement with other findings (Lusher et al., 2013, 2016; Neves et al., 2015; Rochman et al., 2015; Bellas et al., 2016). However, fibres are often considered to be a contaminant of airborne nature and are sometimes excluded from analysis (Foekema et al., 2013; Rummel et al., 2016; Tanaka and Takada, 2016). As we did not observe any fibres on the filters used as blanks, we argue that fibres do indeed make up a large proportion of microplastics and are not of airborne nature. In support of this, Rochman et al. (2015) found high numbers of fibres in fish sampled from USA fish markets, but not in those sampled from Indonesian fish markets. The authors suggest that this is due to the large amount of waste water effluents carrying synthetic fibres from washing machines as such machines are more common in developed areas. In fact, the microplastics we identified from the fish gut contents closely overlapped in colour, size, shape, and type with those sampled from the surface water (Figure 2) and we can thus assume that types of microplastics sampled from organisms are a reflection of those found in the environment they inhabit.
While large gyres have been a major focus of microplastic research, this study together with that of Yu et al. (2018) show that mesoscale features such as eddies may also be a hot spot for microplastics and should be further investigated. Furthermore, future studies quantifying microplastic ingestion by predatory fish species should also consider to sampling their putative prey to investigate trophic transfer of microplastics.
In conclusion, this study reports the highest ingestion rates of microplastics in the gastrointestinal tracts of mesopelagic fish. This has important consequences for the health of pelagic ecosystems and biogeochemical cycling in general. Additionally, using forensic techniques, we provide more evidence that fibres are found throughout our oceans rather than being an artefact of airborne contamination.
TD and EM conceptualised the study; EM and AA (chief scientist) participated in the research cruise and collected samples; TD and AW designed the experiments; AW and HB performed the experiments; HB and LM took SEM images; AW, OS, and LM carried out micro-FTIR analysis; AW analysed the data; AW and TD wrote the manuscript with contributions from PC, LM, AA, and EM.
The authors declare that the research was conducted in the absence of any commercial or financial relationships that could be construed as a potential conflict of interest.
We thank the researchers and crew of the CE15007 cruise for assistance in sample collection. Access to the Perkin Elmer Spotlight 200i FT-IR Microscopy System was given by Perkin Elmer, UK. AW was funded by an NUI Galway postgraduate scholarship. This publication has emanated from research supported by the Marine Institute and funded by the Marine Research Programme by the Irish Government under the framework of JPI Oceans (Grant-Aid Agreement no. PBA/ME/15/03, the PLASTOX Project) and a research grant from Science Foundation Ireland (SFI) (Grant-Aid Agreement No. 13/RC/2092) and co-funded under the European Regional Development Fund and by PIPCO RSG and its member companies. The work was performed in the context of the iCRAG (Irish Centre for Research in Applied Geoscience) Marine Spoke (TP2.3).
Barnes, D. K., Galgani, F., Thompson, R. C., and Barlaz, M. (2009). Accumulation and fragmentation of plastic debris in global environments. Philos. Trans. R. Soc. B 364, 1985–1998. doi: 10.1098/rstb.2008.0205
Bauchot, M.-L. (1986). “Serrivomeridae,” in Fishes of the North-Eastern Atlantic and the Mediterranean, eds J. P. J. Whitehead, M.-L. Bauchot, J.-C. Hureau, J. Nielsen, and E. Tortonese (Paris: UNESCO), 548–550.
Bellas, J., Martínez-Armental, J., Martínez-Cámara, A., Besada, V., and Martínez-Gómez, C. (2016). Ingestion of microplastics by demersal fish from the Spanish Atlantic and Mediterranean coasts. Mar. Pollut. Bull. 109, 55–60. doi: 10.1016/j.marpolbul.2016.06.026
Besseling, E., Wegner, A., Foekema, E. M., van den Heuvel-Greve, M. J., and Koelmans, A. A. (2013). Effects of microplastics on fitness and PCB bioaccumulation by the lugworm Arenicola marina (L.). Environ. Sci. Technol. 47, 593–600. doi: 10.1021/es302763x
Boerger, C. M., Lattin, G. L., Moore, S. L., and Moore, C. J. (2010). Plastic ingestion by planktivorous fishes in the North Pacific central gyre. Mar. Pollut. Bull. 60, 2275–2278. doi: 10.1016/j.marpolbul.2010.08.007
Carpenter, E. J., Anderson, S. J., Harvey, G. R., Miklas, H. P., and Peck, B. B. (1972). Polystyrene Spherules in Coastal Waters. Science 178, 749–750. doi: 10.1126/science.178.4062.749
Cherel, Y., Ducatez, S., Fontaine, C., Richard, R., and Guinet, C. (2008). Stable isotopes reveal the trophic position and mesopelagic fish diet of female southern elephant seals breeding on the Kerguelen Islands. Mar. Ecol. Prog. Ser. 370, 239–247. doi: 10.3354/meps07673
Cole, M., Lindeque, P., Fileman, E., Clark, J., Lewis, C., Halsband, C., et al. (2016). Microplastics alter the properties and sinking rates of zooplankton faecal pellets. Environ. Sci. Technol. 6, 3239–3246. doi: 10.1021/acs.est.5b05905
Cole, M., Webb, H., Lindeque, P. K., Fileman, E. S., Halsband, C., and Galloway, T. S. (2014). Isolation of microplastics in biota-rich seawater samples and marine organisms. Sci. Rep. 4:4528. doi: 10.1038/srep04528
Danielsen, J., van Franeker, J. A., Olsen, B., and Bengtson, S.-A. (2010). Preponderance of mesopelagic fish in the diet of the Northern fulmar Fulmarus glacialis around the Faroe Islands. Seabird 23, 66–75. Available online at: http://www.seabirdgroup.org.uk/seabird-23
Davison, P. C., Checkley, D. M., Koslow, J. A., and Barlow, J. (2013). Carbon export mediated by mesopelagic fishes in the northeast Pacific Ocean. Prog. Oceanogr. 116, 14–30. doi: 10.1016/j.pocean.2013.05.013
Davison, P., and Ash, R. G. (2011). Plastic ingestion by mesopelagic fishes in the North Pacific subtropical gyre. Mar. Ecol. Prog. Ser. 432, 173–180. doi: 10.3354/meps09142
Desforges, J. P., and Ross, P. S. (2015). Ingestion of microplastics by zooplankton in the Northeast Pacific Ocean. Arch. Environ. Contam. Toxicol. 69, 320–330. doi: 10.1007/s00244-015-0172-5
Dufois, F., Hardman-Mountford, N. J., Greenwood, J., Richardson, A. J., Feng, M., and Matear, R. J. (2016). Anticyclonic eddies are more productive than cyclonic eddies in subtropical gyres because of winter mixing. Oceanography 2:e1600282. doi: 10.1126/sciadv.1600282
Eriksen, M., Lebreton, L. C. M., Carson, H. S., Thiel, M., Moore, C. J., Borerro, J. C., et al. (2014). Plastic pollution in the World's Oceans: more than 5 trillion plastic pieces weighing over 250,00 tons afloat at sea. PLoS ONE 9:e111913. doi: 10.1371/journal.pone.0111913
Fennell, S., and Rose, G. (2015). Oceanographic influences on deep scattering layers across the North Atlantic. Deep-Sea Res. I 105, 132–141. doi: 10.1016/j.dsr.2015.09.002
Foekema, E. M., De Gruijter, C., Mergia, M. T., van Franeker, J. A., Murk, A. J., and Koelmans, A. A. (2013). Plastic in North sea fish. Environ. Sci. Technol. 47, 8818–8824. doi: 10.1021/es400931b
Galgani, F., Hanke, G., and Maes, T. (2015). “Global distribution, composition and abundance of marine litter,” in Marine Anthropogenic Litter, eds M. Bergmann, L. Gutow, and M. Klanges (Dordrecht: Springer), 29–56.
Galgani, F., Hanke, G., Werner, S., and De Vrees, L. (2013). Marine litter within the European marine strategy framework directive. ICES J. Mar. Sci. 70, 1055–1064. doi: 10.1093/icesjms/fst122
Gall, S. C., and Thompson, R. C. (2015). The impact of debris on marine life. Mar. Pollut. Bull. 92, 170–179. doi: 10.1016/j.marpolbul.2014.12.041
Gjøsaeter, J., and Kawaguchi, K. (1980). A review of the world resources of mesopelagic fish. FAO Fish. Tech. Pap. 193, 1–151.
Irigoien, X., Klevjer, T. A., Røstad, A., Martinez, U., Boyra, G., Acuña, J. L., et al. (2014). Large mesopelagic fish biomass and trophic efficiency in the open ocean. Nat. Commun. 5:3271. doi: 10.1038/ncomms4271
Kaartvedt, S., Staby, A., and Aksnes, D. L. (2012). Efficient trawl avoidance by mesopelagic fishes causes large underestimation of their biomass. Mar. Ecol. Prog. Ser. 456, 1–5. doi: 10.3354/meps09785
Lusher, A. L., Burke, A., O'Connor, I., and Officer, R. (2014). Microplastic pollution in the Northeast Atlantic Ocean: validated and opportunistic sampling. Mar. Pollut. Bull. 88, 325–333. doi: 10.1016/j.marpolbul.2014.08.023
Lusher, A. L., Hollman, P. C. H., and Mendoza-Hill, J. J. (2017). Microplastics in Fisheries and Aquaculture: Status of Knowledge on their Occurrence and Implications for Aquatic Organisms and Food Safety. Rome: FAO Fisheries and Aquaculture Technical Paper. No. 615.
Lusher, A. L., McHugh, M., and Thompson, R. C. (2013). Occurrence of microplastics in the gastronintestinal tract of pelagic and demersal fish from the English channel. Mar Pollut. Bull. 67, 94–99. doi: 10.1016/j.marpolbul.2012.11.028
Lusher, A. L., O'Donnel, C., Officer, R., and O' Connor, I. (2016). Microplastic interactions with North Atlantic mesopelagic fish. ICES J. Mar. Sci. 73, 1214–1225. doi: 10.1093/icesjms/fsv241
Marine Species Identification Portal (2015). Available online at: http://species-identification.org/ (Accessed September 15, 2015).
Mato, Y., Isobe, T., Takada, H., Kanehiro, H., Ohtake, C., and Kaminuma, T. (2001). Plastic resin pellets as a transport medium for toxic chemicals in the marine environment. Environ. Sci. Technol. 35, 318–324. doi: 10.1021/es0010498
McKelvie, D. S. (1985). The mesopelagic fish fauna of the newfoundland basin. Can. J. Zool. 63, 2176–2182. doi: 10.1139/z85-321
Morrison, L., Feely, M., Stengel, D. B., Blamey, N., Dockery, P., Sherlock, A., et al. (2009). Seaweed attachment to bedrock: biophysical evidence for a new geophycology paradigm. Geobiology 7, 477–487. doi: 10.1111/j.1472-4669.2009.00206.x
Murray, F., and Cowie, P. R. (2011). Plastic contamination in the decapod crustacean Nephrops norvegicus (Linnaeus, 1758). Mar. Pollut. Bull. 62, 1207–1217. doi: 10.1016/j.marpolbul.2011.03.032
Nadal, M. A., Alomar, C., and Deudero, S. (2016). High levels of microplastic ingestion by the semipelagic fish bogue Boops boops (L.) around the Balearic Islands. Environ. Pollut. 214, 517–523. doi: 10.1016/j.envpol.2016.04.054
Neves, D., Sobral, P., Ferreira, J. L., and Pereira, T. (2015). Ingestion of microplastics by commercial fish off the portuguese coast. Mar. Pollut. Bull. 101, 119–126. doi: 10.1016/j.marpolbul.2015.11.008
Pedà, C., Caccamo, L., Fossi, M. C., Gai, F., Andaloro, F., Genovese, L., et al. (2016). Intestinal alterations in European sea bass Dicentrarchus labrax (Linnaeus, 1758) exposed to microplastics: preliminary results. Environ. Pollut. 212, 251–256. doi: 10.1016/j.envpol.2016.01.083
Radchenko, V. I. (2007). Mesopelagic fish community supplies “Biological Pump.” Raffles B Zool. 14, 265–271. Available online at: https://lkcnhm.nus.edu.sg/rbz/supplement-no-14/
R Development Core Team (2017). R: A Language and Environment for Statistical Computing. Vienna: R Foundation for Statistical Computing. Available online at: https://www.R-project.org/
Rochman, C. M., Hoh, E., Kurobe, T., and Teh, S. J. (2013). Ingested plastic transfers hazardous chemicals to fish and induces hepatic stress. Sci. Rep. 3:3263. doi: 10.1038/srep03263
Rochman, C. M., Tahir, A., Williams, S. L., Baxa, D. V., Lam, R., Miller, J. T., et al. (2015). Anthropogenic debris in seafood: plastic debris and fibers from textiles in fish and bivalves sold for human consumption. Sci. Rep. 5:14340. doi: 10.1038/srep14340
Roe, H. S. J., and Badcock, J. (1984). The diel migrations and distributions within a mesopelagic community in the North East Atlantic. 5. Vertical migrations and feeding of fish. Prog. Oceanogr. 13, 389–424. doi: 10.1016/0079-6611(84)90014-4
Rummel, C. D., Löder, M. G. J., Fricke, N. F., Lang, T., Griebeler, E.-M., Janke, M., et al. (2016). Plastic ingestion by pelagic and demersal fish from the North sea and Baltic sea. Mar. Pollut. Bull. 102, 134–141. doi: 10.1016/j.marpolbul.2015.11.043
Ryan, P. G. (2015). “A brief history of marine litter research,” in Marine Anthropogenic Litter, eds M. Bergmann, L. Gutow, and M. Klanges (Dordrecht: Springer), 1–25.
Scott, W. B., and Tibbo, S. N. (1968). Food and feeding habits of swordfish, Xiphias gladius, in the Western North Atlantic. J. Fish. Res. Board Can. 25, 903–919. doi: 10.1139/f68-084
Scotto di Carlo, B., Constanzo, G., Fresi, E., Guglielmo, L., and Ianora, A. (1982). Feeding ecology and stranding mechansims in two lanternfishes, Hygophum benoiti and Myctophum punctatum. Mar. Ecol. Prog. Ser. 9, 13–24. doi: 10.3354/meps009013
Setälä, O., Fleming-Lehtinen, V., and Lehtiniemi, M. (2014). Ingestion and transfer of microplastics in the planktonic food web. Envirn. Pollut. 185, 77–83. doi: 10.1016/j.envpol.2013.10.013
Spitz, J., Mourocq, E., Leauté, J.-P., Quéro, J.-C., and Ridoux, V. (2010). Prey selection by the common dolphin: fulfilling high energy requirements with high quality food. J. Exp. Biol. 390, 73–77. doi: 10.1016/j.jembe.2010.05.010
St. John, M. A., Borja, A., Chust, G., Heath, M., Grigorov, I., Mariani, P., et al. (2016). A dark hole in our understanding of marine ecosystems and their services: perspectives from the mesopelagic community. Front. Mar. Sci. 3:31. doi: 10.3389/fmars.2016.00031
Sutherland, W. J., Barnard, P., Broad, S., Clout, M., Connor, M., Côte, I. M., et al. (2017). A 2017 horizon scan of emerging issues for global conservation and biological diversity. Trends. Ecol. Evol. 32, 31–40. doi: 10.1016/j.tree.2016.11.005
Tanaka, K., and Takada, H. (2016). Microplastic fragments and microbeads in digestive tracts of planktivorous fish from urban coastal waters. Sci. Rep. 6:34351. doi: 10.1038/srep34351
UNEP (2016). Marine Plastic Debris and Microplastics – Global Lessons and Research to Inspire Action and Guide Policy Change. Nairobi: United Nations Environment Programme.
Van Franeker, J. A., and Bell, P. J. (1988). Plastic ingestion by petrels breeding in Antarctica. Mar. Pollut. Bull. 19, 672–674. doi: 10.1016/0025-326X(88)90388-8
Varela, J. L., Rodríguez-Marín, E., and Medina, A. (2013). Estimating diets of pre-spawning Atlantic Bluefin tuna from stomach content and stable isotope analyses. J. Sea Res. 76, 187–192. doi: 10.1016/j.seares.2012.09.002
Wardrop, P., Shimeta, J., Nugegoda, D., Morrison, P. D., Miranda, A., Tang, M., et al. (2016). Chemical pollutants sorbed to ingested microbeads from personal care products accumulate in fish. Environ. Sci. Technol. 50, 4037–4044. doi: 10.1021/acs.est.5b06280
Wesch, C., Elert, A. M., Wörner, M., Braun, U., Klein, R., and Paulus, M. (2017). Assuring quality in microplastic monitoring: about the value of clean-air devices as essentials for verified data. Sci. Rep. 7:5424. doi: 10.1038/s41598-017-05838-4
Woodall, L. C., Gwinnett, C., Packer, M., Thompson, R. C., Robinson, L. F., and Paterson, G. L. J. (2015). Using a forensic science approach to minimize environmental contamination and to identify microfibers in marine sediments. Mar. Pollut. Bull. 95, 40–46. doi: 10.1016/j.marpolbul.2015.04.044
Wright, S. L., Rowe, D., Thompson, R. C., and Galloway, T. S. (2013). Microplastic ingestion decreases energy reserves in marine worms. Curr. Biol. 23, 1031–1033. doi: 10.1016/j.cub.2013.10.068
Keywords: myctophids, marine litter, micro-FTIR, fibres, eddy, deep sea, biogeochemical cycling, carbon sequestration
Citation: Wieczorek AM, Morrison L, Croot PL, Allcock AL, MacLoughlin E, Savard O, Brownlow H and Doyle TK (2018) Frequency of Microplastics in Mesopelagic Fishes from the Northwest Atlantic. Front. Mar. Sci. 5:39. doi: 10.3389/fmars.2018.00039
Received: 08 December 2017; Accepted: 26 January 2018;
Published: 19 February 2018.
Edited by:
Angel Borja, Centro Tecnológico Experto en Innovación Marina y Alimentaria (AZTI), SpainReviewed by:
Monica F. Costa, Universidade Federal de Pernambuco, BrazilCopyright © 2018 Wieczorek, Morrison, Croot, Allcock, MacLoughlin, Savard, Brownlow and Doyle. This is an open-access article distributed under the terms of the Creative Commons Attribution License (CC BY). The use, distribution or reproduction in other forums is permitted, provided the original author(s) and the copyright owner are credited and that the original publication in this journal is cited, in accordance with accepted academic practice. No use, distribution or reproduction is permitted which does not comply with these terms.
*Correspondence: Alina M. Wieczorek, QWxpbmEuV2llY3pvcmVrQG51aWdhbHdheS5pZQ==
Disclaimer: All claims expressed in this article are solely those of the authors and do not necessarily represent those of their affiliated organizations, or those of the publisher, the editors and the reviewers. Any product that may be evaluated in this article or claim that may be made by its manufacturer is not guaranteed or endorsed by the publisher.
Research integrity at Frontiers
Learn more about the work of our research integrity team to safeguard the quality of each article we publish.