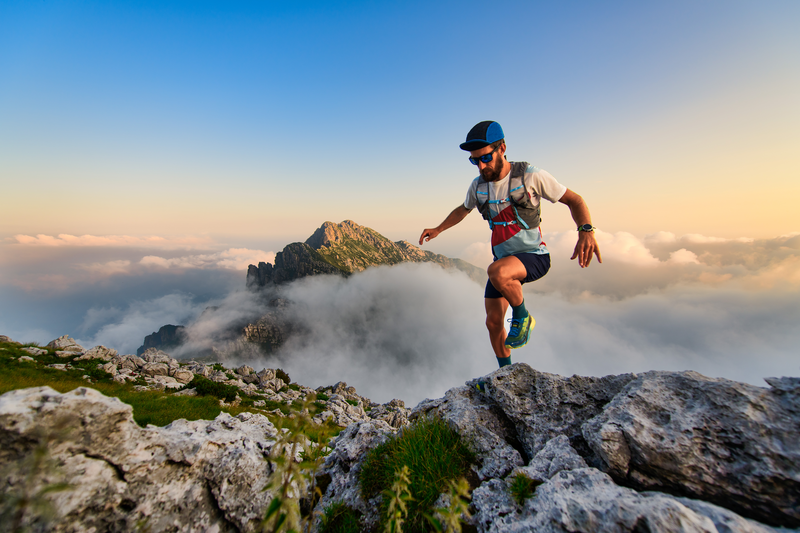
95% of researchers rate our articles as excellent or good
Learn more about the work of our research integrity team to safeguard the quality of each article we publish.
Find out more
ORIGINAL RESEARCH article
Front. Mar. Sci. , 20 February 2018
Sec. Marine Megafauna
Volume 5 - 2018 | https://doi.org/10.3389/fmars.2018.00015
During breeding, seabirds are central place foragers and are sensitive to changes in local prey availability. As the breeding season progresses, foraging behavior and distribution is expected to change in response to possible changes in local prey availability. In addition, adult gender, and the increasing nutritional demands of a growing chick may also influence the foraging behavior of individuals. At present, relatively few studies have assessed the foraging behavior of adult birds during the late post-guard stages of chick rearing. Through a combination of GPS tracking and diet sampling we investigated the foraging distances, spatial distribution, and prey composition of adult Cape gannets (Morus capensis) during the guard and post-guard stages of chick rearing. We found no clear evidence for consistent sex-specific differences in foraging distances and spatial distribution during the guard stage, although marginal differences in the location of core foraging areas during the post-guard stage were apparent. Results, however, revealed a clear increase in foraging range from the early guard to the late post-guard stage of chick rearing. During December the diet was comprised almost exclusively of anchovy (Engraulis encrasicolus), the proportion of which had decreased significantly in the diet by January. This was mirrored by a substantial increase in the proportion of saury (Scomberesox saurus). These results suggest that Cape gannets show flexibility in the foraging behavior and diet, which may be related to changes in the abundance and distribution of prey or may reflect changes in the energetic requirements of the growing offspring. This study provides the first assessment of Cape gannet foraging behavior and spatial distribution during the post-guard stage of chick rearing. The importance of considering intra-annual variability in foraging distribution when using seabird tracking data in trophic and marine spatial planning studies are highlighted.
Animals often forage in environments characterized by heterogeneity in resource availability and distribution (Russell et al., 1992; Johnson et al., 2001). This is particularly evident within the marine environment, where high levels of spatio-temporal variability in the physical environment are expected to influence the distribution and availability of prey (Cherel and Hobson, 2007; Weimerskirch, 2007). During the breeding season, marine predators such as seals and seabirds are central place foragers and must balance periods of foraging at sea with regular periods of offspring attendance (Ricklefs, 1983; Guinet et al., 2001; Weimerskirch et al., 2009). This places constraints on their foraging behavior, increasing the vulnerability of individuals to changes in local environmental conditions and prey availability (e.g., Shaffer et al., 2003; Harding et al., 2007). However, behavioral plasticity may allow individuals to buffer the effects of resource limitation (Granadeiro et al., 1998; Sommerfeld et al., 2015). In this regard, range extensions (Furness and Birkhead, 1984; Burke and Montevecchi, 2009; Hennicke and Weimerskirch, 2014) and dietary shifts (Ainley et al., 2003; Lescroel et al., 2004; Elliott et al., 2010; Owen et al., 2013) have been well documented. However, when foraging ranges are restricted, individuals may instead increase the amount of time and effort allocated to foraging during periods of reduced prey availability (Harding et al., 2007; Angel et al., 2015). In addition, several studies have documented the onset of bimodal/dual foraging strategies (Wanless et al., 1994; Weimerskirch et al., 1994; Magalhães et al., 2008; Welcker et al., 2009; Jakubas et al., 2012). In these cases, birds alternate between short and long trips which generally, but not always (Carpenter-Kling et al., 2017), relate to chick and self-provisioning, respectively (Granadeiro et al., 1998; Weimerskirch, 1998). Understanding the range of behavioral expressions associated with foraging plasticity is important for population and ecosystem-based management.
Evidence of foraging and dietary plasticity in response to changes in the local environment has provided significant impetus for the use of seabirds as sentinels of change in the marine environment (Cairns, 1987; Piatt and Sydeman, 2007; Durant et al., 2009). However, in addition to the influence of local environmental conditions and resource availability, various intrinsic factors may also influence foraging behavior. For example, sex-specific differences in foraging distributions (González-Solís et al., 2000; Pinet et al., 2012) behavior (Lewis et al., 2002; Zavalaga et al., 2007; Pichegru et al., 2013), and diet (Forero et al., 2002; Owen et al., 2013) have been documented across various seabird taxa. In addition, foraging behavior, and preferences in diet may also reflect the changes in the nutritional requirements of the growing chicks (Cairns, 1987; Dall'Antonia et al., 2001; Shaffer et al., 2003; Harding et al., 2007). Indeed, changes in adult foraging behavior and distribution between the incubation, guard and post-guard stages of chick rearing have been identified in several seabird species (Stahl and Sagar, 2000; Huin, 2002; Rishworth et al., 2014b; Widmann et al., 2015). Understanding the extent to which these factors influence foraging behavior is therefore important if seabirds are to be used effectively as indicator species.
The monomorphic Cape gannet (Morus capensis) is a breeding endemic to southern Africa (Crawford et al., 1983). The distribution of its breeding colonies is limited to five islands on the west coast of South Africa and Namibia, and a single island off South Africa's south coast (Crawford et al., 2007). A pair generally produces a single offspring (Jarvis, 1974; Rishworth and Pistorius, 2015) and alternate periods of nest attendance and foraging during incubation and the early stages of chick rearing (Bijleveld and Mullers, 2009; Mullers and Tinbergen, 2009). However, when the chick is around 40–50 days old, the transition between the guard and post-guard phase takes place and both parents start spending progressively longer periods simultaneously at sea, returning to the nest for brief periods to feed the chick (Nelson, 1978; Rishworth et al., 2014b).
Foraging Cape gannets often travel several 100 km in search of prey (Grémillet et al., 2004; Moseley et al., 2012; Green et al., 2015), which generally comprises small pelagic fishes such as sardine (Sardinops sagax) and anchovy (Engraulis encrasicolus) (Berruti et al., 1993; Adams and Klages, 1999; Green et al., 2014). Both inter and intra-annual changes in the foraging behavior and diet of Cape gannets in response to variability in the availability and distribution of preferred prey have been identified previously (Mullers et al., 2007; Pichegru et al., 2010; Green et al., 2014, 2015). Despite this, no studies have attempted an explicit comparison of foraging behavior and spatial distribution between the guard and post-guard stages of chick rearing. In addition, while sex-specific differences in spatial distribution appear to be marginal during the early stages of chick rearing (Botha et al., 2017), little is known about the spatial distribution of females and males during the poorly studied post-guard stage.
Nest attendance patterns assessed continuously through VHF monitoring at Bird Island indicate that as the breeding season progresses and chicks age, Cape gannets increase their foraging trip durations (Rishworth et al., 2014b; Pistorius et al., 2015). Furthermore, while trip durations are similar during the early stages of chick rearing, females undertake significantly longer trips than males during the post-guard stage, which may be a result of differences in spatial distribution (Rishworth et al., 2014b). The aim of this study was therefore to provide the first comparative assessment of Cape gannet foraging distribution and diet during the guard and post-guard stages of chick rearing. In addition, we investigate the distribution of females and males during both stages of chick rearing to assess the occurrence of sex-specific spatial segregation during the post-guard stage.
Data collection for the purpose of this study was undertaken in accordance with the ethics clearance reference A10-SCI-ZOO-008, issued by the Research Ethics Committee at the Nelson Mandela University. Previous studies on this species have shown that handling and device attachment do not appear to have a measurable negative impact on the foraging behavior, reproductive output or body condition of study birds (Grémillet et al., 2004; Pichegru et al., 2007; Rishworth et al., 2014c).
Data collection for this study was conducted at Bird Island (33°50′26″S 26°17′10″E), Algoa Bay, South Africa during the 2015/16 austral summer. Bird Island is located in the eastern corner of Algoa Bay and at present hosts the world's largest Cape gannet colony (ca 90,000 breeding pairs) (Crawford et al., 2007). Algoa Bay is one of several log-spiral bays along the South African coastline (Goschen and Schumann, 2011). The oceanography of Algoa Bay and South Africa's south coast is largely influenced by the warm Agulhas current which flows in a southwesterly direction along the continental shelf (Schumann, 1987).
GPS devices were deployed on adult Cape gannets during two separate periods (December and January) of the 2015/16 breeding season. Between 5 and 18 December 2015, 18 adults attending small chicks and between 15 January and 3 February 2016, 26 adult attending large chicks, were each fitted with a GPS unit (CatLog-S, Catnip Technologies, Hong Kong or Axy-trek GiPSy-5, TechnoSmart, Italy). The devices were sealed in heat shrink (CatLog-S) or an epoxy casing (TechnoSmart Axy-trek), with the total package weighing <25 g (~0.9% of adult body mass). Devices were programmed to record a fix at either 1 or 4 min intervals. The deployment procedure involved capturing the birds with a 3 m crooked-pole as they left the nest, following which the GPS unit was attached to the central tail feathers using waterproof Tesa® tape (Tesa, Hamburg, Germany). Wherever possible both partners of a breeding pair were fitted with devices in an attempt to obtain an even sex-ratio (visual discrimination is not possible in this monomorphic species; Rishworth et al., 2014a). Once the device was fitted, the bird was marked using a livestock marker and released. For retrieval, the nests of the marked birds were monitored between sunrise and sunset. When the marked bird returned, it was left to feed the chick, after which it was captured, the device removed, and the bird released safely. Adult birds were generally tracked for a single foraging trip. However, a second foraging trip was obtained for six individuals during the post-guard stage.
Body mass (to the nearest 25 g) of equipped birds was measured at both deployment and retrieval. In addition, culmen (to the nearest 0.1 mm) and wing cord length (to the nearest 1 mm) were recorded only at retrieval. Body condition of adult birds was calculated as the mass of the bird divided by the wing cord length. Morphometric measurements were also recorded for the chicks of all of the equipped birds to calculate chick age at the time of deployment. Chick age at the time of deployment was calculated following Mullers et al. (2009), using either culmen length or wing cord length (see Botha et al., 2017 for further details). All tracked adult birds were sexed by means of genetic analysis using breast feathers from each individual, following the Chelex® extraction method (see Rishworth et al., 2014a for further details). The handling of birds was minimized as much as possible to reduce stress (less than 10 min for adults and 5 min for chicks).
Data processing and analyses were conducted in the R statistical environment (R Core Team, 2015). GPS tracks were cleaned by removing the points located at the colony and a speed filter was applied to the data to remove inaccurate GPS fixes (McConnell et al., 1992). To correct for irregular fix frequencies and differences in sampling rates, all tracks were regularized to 1 min intervals by means of linear interpolation using the package “adehabitatLT” (Calenge, 2006). Second trips obtained from six individuals during the post-guard stage were excluded from further statistical analyses. In four cases, battery failure occurred before birds returned to the nest, resulting in incomplete tracks which were retained for further analyses unless stated otherwise.
Spatial distributions of female and male Cape gannets during the guard and post-guard stages of chick rearing was quantified by means of kernel home range analysis using the “adehabitatHR” package (Calenge, 2006). Differences in sample size and individual contribution to the data may influence home range analysis (Soanes et al., 2013). As such, 9 females and 9 males were randomly selected for home range analysis during each stage. Both complete and incomplete GPS tracks were considered in this selection to reduce potential bias of including only complete (shorter) trips. To represent the total range and core foraging areas, the 95 and 50% volume contours were calculated respectively. Home ranges were calculated with the ad-hoc method as a smoothing parameter over a constant grid cell size of 200 m2. To assess the sex-specific differences in spatial distribution during each breeding stage, a utilization distribution overlap index (UDOI) was calculated as the measure of overlap between females and males (Fieberg and Kochanny, 2005). A randomization approach was then used to test the null hypothesis that there were no sex-specific differences in the spatial distribution during each stage. For the null hypothesis to be true, the degree of overlap calculated from the original data set should not be significantly different from a dataset in which sex was randomly assigned to individuals. We performed a total of 1,000 randomizations and for each permutation, sex was randomly allocated, following which home ranges and overlap indices were calculated. P-values were determined by the proportion of random overlaps that were smaller than the observed overlap (Breed et al., 2006; Cleasby et al., 2015).
For each GPS track, the total distance traveled (km), maximum distance from the colony (km), and total duration (h) of the foraging trip was calculated using the “geosphere” package (Hijmans, 2015). Total distance traveled and total duration, calculated using complete trips, were highly correlated (Pearsons correlation test, r > 0.8). As such, only total distance traveled was selected for further statistical analysis. However, to account for any potential bias associated with including only complete (generally shorter) tracks, maximum distance calculated using both complete and incomplete trips was also included in the analysis. Data normality was assessed by means of a Shapiro–Wilk's test. Data were right skewed and were therefore log-transformed before being incorporated into a linear model which included sex, stage of the breeding season, adult body condition (BC) and the interaction between sex and stage and sex, and body condition as predictor effects. An inspection of the residuals vs. fitted values suggested that the assumption of homogeneity of variance was not violated, and model residuals appeared normally distributed. Model selection was conducted using Akaike Information Criterion (AIC) scores, and the most parsimonious model identified as having the lowest AIC score (Akaike, 1998). Models with a delta AIC score of <2 were also considered among the most parsimonious models (Burnham and Anderson, 1998). All results are presented as Mean ± Standard Error (SE), unless stated otherwise.
Diet samples were collected during both above-mentioned study periods. Samples were generally collected in the morning or late in the afternoon when individuals returned from foraging trips. Birds landing heavily alongside the colony were captured and inverted over a plastic bucket to induce regurgitation. Once a sample was obtained the bird was released. In addition, diet samples were also collected when birds regurgitated spontaneously due to handling, associated with the deployment of tracking devices.
Diet samples were processed on Bird Island. Each sample was weighed to obtain the total mass, after which it was separated according to it's comprising prey species. For each prey species, the total mass, number of individuals (estimated from whole fish and the number of heads and tails), and fork length of whole individuals, were obtained. The constituents of all samples were identifiable to species level.
Data were assessed separately for December and January to determine prey composition during the guard and post-guard phase respectively. Following the methods of Green et al. (2014), prey species were divided into five prey groups, namely anchovy, sardine, saury, other live prey (OLP), and fishery discards. The proportional contribution of each prey group to the diet was compared between December and January based on mass (% Mass), numerical abundance (% NA), and frequency of occurrence (% FO).
Tracking data was recorded for 21 females and 23 males. Of these, 18 foraging trips (9 females, 9 males) were recorded for birds rearing young chicks during the guard stage of chick rearing, whilst 26 foraging trips (12 females, 14 males) were recorded for birds rearing older chicks during the post-guard stage of chick rearing. In total, 4 foraging trips out of the 44 tracked individuals were incomplete as a result of battery failure.
The distribution and home ranges of Cape gannets covered a substantially larger area during the post-guard stage than during the guard stage of chick rearing (Table 1, Figure 1). This was evident both from visual assessment of all the foraging trips and from home ranges of 9 randomly selected individuals per sex and stage. During the guard stage, only a single female traveled well beyond Port Elizabeth (~60 km from Bird Island) (Figure 1). During the post-guard stage, a single female and three males extended their distributions well west of Plettenberg Bay (which is ~250 km from Bird Island). Two devices failed before the birds returned to the colony and as such, it is possible that the foraging ranges of these individuals may have extended even further. The remainder of male Cape gannets appeared to restrict their distribution to the area east of Cape St Francis, while the distributional range of several females extended west of Cape St Francis (Figure 1). The total ranges (95%) of females and males overlapped considerably during both stages (Table 1). Core areas (50%) of females and males overlapped less, particularly during the post-guard stage (UDOI < 0.1). During this period, the bulk of the female core areas generally extended westward while the bulk of the male core areas generally extending eastward of Bird Island (Figure 1). However, results from the randomization test suggested that the observed overlaps between females and males were not significantly lower than the randomly generated overlaps (Table 1). As such, the null hypothesis that there were no sex-specific differences in spatial distribution could not be rejected.
Table 1. Observed and randomized utilization distribution overlap indices (UDOI) (Fieberg and Kochanny, 2005) of the total (95% density contour) and core area (50% density contour) of female and male Cape gannets during the guard and post-guard stages of chick-rearing in the 2015/16 breeding season. Randomized UDOI is indicated as mean ± SD and p is the proportion of random overlaps that were smaller than the observed overlap.
Figure 1. Foraging ranges of female (red) and male (blue) Cape gannets during the guard and post-guard stages of the 2015/16 breeding season. GPS tracks represent all tracked individuals while kernel home range plots represent 9 randomly selected females and males for each breeding stage. Density contours are represented as 95% (unfilled areas) and 50% (filled areas). The overlap of the 50% contours of females and males are indicated by the red and blue striped area. Bird Island is indicated by the yellow star.
The most parsimonious models for the response of total distance traveled and maximum distance from the colony included sex, stage, and body condition as predictor effects (Table 2). The individual effects of both sex and body condition were, however, not significant on either total distance or maximum distance (Table 3). On average, the distance traveled and duration of foraging trips were remarkably similar for females and males during both the guard and post-guard stages of chick rearing (Table 4). Total and maximum distances appeared to be inversely proportional to body condition (Table 3), suggesting that birds in poorer condition traveled further than birds in better condition condition. However, there was no clear linear trend to substantiate this. The individual effect of breeding stage on maximum distance was significant (Table 3). On average, both females and males substantially increased the distances and duration of their foraging trips between the guard and post-guard stage (Table 4). Total distance traveled was not significantly affected by stage of the breeding season (Table 3). This may reflects a bias toward shorter foraging trips as only complete tracks were used to calculate total distance. No interaction terms were retained in the most parsimonious models (Table 2). A second foraging trip was obtained for six individuals (1 female, 5 males) during the post-guard phase. For five of these individuals there was a substantial difference in the total distance, maximum distance and duration between the first and second trip (Table 5, Figure 2). For one individual (Individual 6), the maximum distances of the first and second trip were similar, although the total distance traveled and duration were greater during the second trip (Table 5). During shorter trips, individuals typically remained close to Bird Island (within 80 km) with distributions restricted to the east of Port Elizabeth (Figure 2). During the longer trips, distributions were generally restricted to the area between Cape St Francis and Port Alfred, barring a single individual which reached Mossel Bay, traversing a total distance of over 1,500 km (Table 5, Figure 2).
Table 2. Linear models of the total distance traveled (TD) and maximum distance from the breeding colony (MD) for Cape gannets as a function of sex, stage of the breeding season (stage), body condition (BC) and the interaction between sex and stage and sex and BC.
Table 3. The most parsimonious linear models of the total distance traveled and maximum distance from the breeding colony for Cape gannets as a function of sex, stage, and body condition.
Table 4. Foraging trip parameters and body condition of female and male Cape gannets attending chicks during the guard and post-guard stages of chick rearing in the 2015/16 breeding season at Bird Island, Algoa Bay.
Table 5. Foraging trip parameters of six adult Cape gannets tracked over two consecutive foraging trips during the post-guard stage of the 2015/16 breeding season.
Figure 2. Foraging trips (GPS tracks) for six individual Cape gannets tracked over two consecutive foraging trips during the post-guard stage of the 2015/16 breeding season. Both the longer (A) and shorter (B) trips are indicated for each individual (unique color). Bird Island is indicated by a yellow star.
Amongst the five major prey groups identified, anchovy and saury were the most dominant, collectively comprising 86.2 and 97.5% to the overall diet of Cape gannets by mass and numerical abundance respectively (Supplementary Table 1, Supplementary Material). However, there was a clear change in the dietary composition between December and January (Table 6). During December, the diet was comprised almost exclusively of anchovy. While the presence of anchovy persisted in the diet during January, there was a substantial increase in the amount of saury in the diet and to a lesser extent, sardine and OLP (Table 6).
Table 6. The proportional contribution by mass (%), numerical abundance (%), and frequency of occurrence (%) of each of the five prey groups to the diet of Cape gannets during December and January of the 2015/16 breeding season.
This study provides the first comparative assessment of Cape gannet foraging distributions and diet during the guard and post-guard stages of chick rearing. Sex-specific differences were not apparent during the early guard stages of chick rearing and there appeared to be only slight differences in the spatial distribution of females and males during the late post-guard stage. There were, however, clear changes in the overall distribution, distances, and dietary composition between the guard and post-guard stage. An additional foraging trip for six individuals during the post-guard phase also suggests the occurrence of a bimodal foraging strategy in Cape gannets.
As recently reported for Cape gannets during the early stages of chick rearing (Botha et al., 2017), we found no clear patterns of sex-specific differences in foraging effort and spatial distribution during the guard stage. In addition, the present study expanded on this work by investigating sex-specific foraging during the post-guard stage. However, the foraging trip distances and the total distributional range were similar for females and males during the post-guard phase as well. Interestingly, Rishworth et al. (2014b) documented clear differences in the foraging trip durations between female and male Cape gannets attending chicks older than 50 days (post-guard stage). This was assumed to be indicative of differences in foraging locations during the later stages of the breeding season. The fact that our results did not reflect such a clear pattern, may be due to our relatively small sample size. However, differences in the distribution of core foraging areas do suggest a marginal level of sex-specific segregation during the post-guard stage.
Sex-specific foraging has been described for a large number of seabirds, including both sexually dimorphic (Lewis et al., 2005; Bearhop et al., 2006) and monomorphic species (Peck and Congdon, 2006; Pinet et al., 2012). These differences are often thought to occur as a result of intra-specific competition, or may reflect sex-specific nutritional requirements or parental investment strategies (Lewis et al., 2002; Phillips et al., 2004; Elliott et al., 2010). While sex-specific differences in foraging trip duration are apparent in Cape gannets (Mullers and Tinbergen, 2009; Mullers and Navarro, 2010), spatial segregation appears to be marginal and context specific, occurring only during periods of apparent resource limitation (present study, Botha et al., 2017). Comparatively, in both northern (Morus bassanus) and Australasian gannets (M. serrator), females are known to forage further offshore, utilizing different habitats to males, which appear to target relatively shallow and inshore areas (Stauss et al., 2012; Cleasby et al., 2015; Angel et al., 2016; Wells et al., 2016). Amongst sulids, documented cases of sex-specific foraging appear to be more common in sexually dimorphic species (Gilardi, 1992; Weimerskirch et al., 2006, 2009). Although previously described as monomorphic, recent evidence suggests that northern and Australasian gannets display a degree of reversed sexual size dimorphism (Stauss et al., 2012; Angel et al., 2015), which may well influence habitat use and facilitate spatial segregation at sea. Interestingly, Cape gannets do not exhibit the same level of dimorphism as its congeners, as apart from differences in culmen length, the body size of females and males are remarkably similar (Rishworth et al., 2014a). Therefore, the lack of clear and consistent niche partitioning between female and male Cape gannets is perhaps not all that surprising considering this high level of monomorphism. Furthermore, the exceptional extension of a single female and three male foraging trips during the post-guard stage clearly indicates that both sexes are capable of traversing considerable distances during breeding.
Foraging trip distances and the spatial range of adult Cape gannets increased substantially between the guard and post-guard stage of breeding. These results are consistent with nest attendance patterns recorded by means of VHF monitoring (Rishworth et al., 2014b; Pistorius et al., 2015). In addition, there appeared to be a clear shift from an anchovy (E. encrasicolus) dominated diet, to a more mixed diet, comprising particularly large quantities of saury (Scomberesox saurus). Although no previous studies have explicitly investigated foraging distributions of Cape gannets during the post-guard stage, intra-seasonal variability in foraging distances and diet have previously been documented along South Africa's west coast (Mullers et al., 2007; Mullers and Navarro, 2010). Collectively these results demonstrate considerable foraging flexibility during the chick rearing period.
Plasticity in foraging behavior allows seabirds to respond to changes in the distribution and availability of prey resources (Montevecchi et al., 2009; Hennicke and Weimerskirch, 2014) and increased levels of intra-specific competition (Lewis et al., 2001; Gaston et al., 2007; Elliott et al., 2009). The observed increase in foraging range of Cape gannets could have been a result of such changes in prey availability, enabled through foraging plasticity. This was also reflected in the diet composition of adults between December and January. Anchovy, a preferred prey species of Cape gannets at Bird Island (Green et al., 2014), entirely dominated the diet during December, but decreased substantially by middle to late January suggesting reduced accessibility to this prey resource for breeding adults or an increase in the availability of alternative prey species. However, a longer-term dataset, in conjunction with prey biomass estimates, would be required to further explore this notion. Alternatively, the observed changes in foraging behavior could be related to offspring development, as adult birds may adjust foraging and prey selection in response to the increasing nutritional demands or fasting abilities of the growing chick (Adams et al., 1991; Berrow and Croxall, 2001; Dall'Antonia et al., 2001; Shaffer et al., 2003).
While the majority of Cape gannets appeared to increase their foraging range during the post-guard phase, several individuals (38%) undertook relatively short foraging trips, remaining within 80 km of Bird Island. This may be related to the increased proportion of saury in the diet of birds during the late post-guard stage, which may have provided an alternative prey source closer to the breeding colony. Following distributional shifts or depletion of preferred prey, Cape gannets may shift their diet to less favorable prey as lower amounts of energy expenditure required to access these species could potentially outweigh their relatively poor nutritional value (Adams and Klages, 1999; Tew Kai et al., 2013). Additionally, the ability of older chicks to consume larger prey items such as saury may further facilitate such a dietary shift. It is also possible that the variability in foraging distribution between individuals during the post-guard stage represents the adoption of a bimodal foraging strategy during the late stages of the breeding cycle (Weimerskirch et al., 1994; Granadeiro et al., 1998; Welcker et al., 2009). For five of the six individuals from which a second foraging trip was recorded during the post-guard stage, the total, and maximum distances as well as the duration of the second trip differed substantially from the first. During the later stages of the breeding season, such strategies could allow adults to balance self-maintenance with provisioning for the increased demands of larger chicks (Weimerskirch, 1998). This warrants further investigation and would require a greater number of individuals to be tracked over multiple foraging trips throughout the breeding season.
In summary, this study provides the first comparative spatial assessment of foraging Cape gannets during different stages of chick rearing. While evidence of sex-specific segregation was minimal, our results indicate a substantial range extension between the early and late stages of chick rearing. Additionally, our results documented a shift in the dietary composition of adult Cape gannets during the late post-guard stages of chick rearing. These results suggest that Cape gannets may be able to buffer against the effects of resource variability throughout chick rearing through foraging and dietary flexibility. These strategies may also reflect changes in response to the needs of growing offspring. Irrespective of the mechanism driving the observed changes, this study highlights the importance of considering intra-seasonal variability when studying the behavior, foraging distribution and diet in seabirds.
JB and PP: conceptualized and designed the study; JB: collected the data and performed the data analysis; JB and PP: wrote the paper.
This research was funded by the National Research Foundation (NRF).
The authors declare that the research was conducted in the absence of any commercial or financial relationships that could be construed as a potential conflict of interest.
We would like to thank The National Research Foundation (NRF) for the bursary provided to JB. South African National Parks (SANParks) is thanked for providing logistical support and accommodation on Bird Island. We are also grateful to the many volunteers who assisted with data collection in the field and made this work possible.
The Supplementary Material for this article can be found online at: https://www.frontiersin.org/articles/10.3389/fmars.2018.00015/full#supplementary-material
Adams, N. J., Abrams, R. W., Siegfried, W. R., Nagy, K. A., and Kaplan, I. R. (1991). Energy expenditure and food consumption by breeding Cape gannets Morus capensis. Mar. Ecol. Prog. Ser. 70, 1–9. doi: 10.3354/meps070001
Adams, N. J., and Klages, N. T. W. (1999). Foraging effort and prey choice in Cape gannets. South Afr. J. Mar. Sci. 21, 157–163. doi: 10.2989/025776199784126060
Ainley, D. G., Ballard, G., Barton, K. J., Karl, B. J., Rau, G. H., Ribic, C. A., et al. (2003). Spatial and temporal variation of diet within a presumed metapopulation of Adélie penguins. Condor 105, 95–106. doi: 10.1650/0010-5422(2003)105[95:SATVOD]2.0.CO;2
Akaike, H. (1998). “Information theory and an extension of the maximum likelihood principle,” in Selected Papers of Hirotugu Akaike, eds E. Parzen, K. Tanabe, and G. Kitagawa (New York, NY: Springer Science & Business Media), 199–213.
Angel, L. P., Berlincourt, M., and Arnould, J. P. (2016). Pronounced inter-colony variation in the foraging ecology of Australasian gannets: influence of habitat differences. Mar. Ecol. Prog. Ser. 556, 261–272. doi: 10.3354/meps11845
Angel, L. P., Wells, M. R., Rodríguez-Malagón, M. A., Tew, E., Speakman, J. R., and Arnould, J. P. Y. (2015). Sexual size dimorphism and body condition in the Australasian Gannet. PLoS ONE 10:e0142653. doi: 10.1371/journal.pone.0142653
Bearhop, S., Phillips, R. A., McGill, R., Cherel, Y., Dawson, D. A., and Croxall, J. P. (2006). Stable isotopes indicate sex-specific and long-term individual foraging specialisation in diving seabirds. Mar. Ecol. Prog. Ser. 311, 157–164. doi: 10.3354/meps311157
Berrow, S. D., and Croxall, J. P. (2001). Provisioning rate and attendance patterns of wandering albatrosses at Bird Island, South Georgia. Condor 103, 230–239. doi: 10.1650/0010-5422(2001)103[0230:PRAAPO]2.0.CO;2
Berruti, A., Underhill, L. G., Shelton, P. A., Moloney, C., and Crawford, R. J. M. (1993). Seasonal and interannual variation in the diet of two colonies of the Cape gannet (Morus capensis) between 1977-78 and 1989. Colon. Waterbirds 16, 158–175. doi: 10.2307/1521434
Bijleveld, A. I., and Mullers, R. H. E. (2009). Reproductive effort in biparental care: an experimental study in long-lived Cape gannets. Behav. Ecol. 20, 736–744. doi: 10.1093/beheco/arp054
Botha, J. A., Rishworth, G. M., Thiebault, A., Green, D. B., and Pistorius, P. A. (2017). Sex-specific foraging over space and time in Cape gannets during chick rearing. Mar. Ecol. Prog. Ser. 579, 157–167. doi: 10.3354/meps12299
Breed, G. A., Bowen, W., McMillan, J., and Leonard, M. L. (2006). Sexual segregation of seasonal foraging habitats in a non-migratory marine mammal. Proc. R. Soc. Lond. B 273, 2319–2326. doi: 10.1098/rspb.2006.3581
Burke, C., and Montevecchi, W. (2009). The foraging decisions of a central place foraging seabird in response to fluctuations in local prey conditions. J. Zool. 278, 354–361. doi: 10.1111/j.1469-7998.2009.00584.x
Burnham, K. P., and Anderson, D. R. (1998). Model Selection and Inference: A Practical Information-Theoretic Approach. New York, NY: Springer-Verlag.
Calenge, C. (2006). The package adehabitat for the R software: a tool for the analysis of space and habitat use by animals. Ecol. Modell. 197, 516–519. doi: 10.1016/j.ecolmodel.2006.03.017
Carpenter-Kling, T., Handley, J., Green, D. B., Reisinger, R. R., Crawford, R. J. M., Makhado, A., et al. (2017). A novel foraging strategy in gentoo penguins breeding at sub-Antarctic Marion Island. Mar. Biol. 164:33. doi: 10.1007/s00227-016-3066-9
Cherel, Y., and Hobson, K. A. (2007). Geographical variation in carbon stable isotope signatures of marine predators: a tool to investigate their foraging areas in the Southern Ocean. Mar. Ecol. Prog. Ser. 329, 281–287. doi: 10.3354/meps329281
Cleasby, I. R., Wakefield, E. D., Bodey, T. W., Davies, R. D., Patrick, S. C., Newton, J., et al. (2015). Sexual segregation in a wide-ranging marine predator is a consequence of habitat selection. Mar. Ecol. Prog. Ser. 518, 1–12. doi: 10.3354/meps11112
Crawford, R. J. M., Dundee, B. L., Dyer, B. M., Klages, N. T. W., Meýer, M. A., and Upfold, L. (2007). Trends in numbers of Cape gannets (Morus capensis), 1956/1957–2005/2006, with a consideration of the influence of food and other factors. ICES J. Mar. Sci. 64, 169–177. doi: 10.1093/icesjms/fsl011
Crawford, R. J. M., Shelton, P. A., Cooper, J., and Brooke, R. K. (1983). Distribution, population size and conservation of the Cape gannet Morus capensis. South Afr. J. Mar. Sci. 1, 153–174. doi: 10.2989/025776183784447458
Dall'Antonia, L., Gudmundsson, G. A., and Benvenuti, S. (2001). Time allocation and foraging pattern of chick-rearing razorbills in northwest Iceland. Condor 103, 469–480. doi: 10.1650/0010-5422(2001)103[0469:TAAFPO]2.0.CO;2
Durant, J. M., Hjermann, D. Ø., Frederiksen, M., Charrassin, J. B., Le Maho, Y., Sabarros, P. S., et al. (2009). Pros and cons of using seabirds as ecological indicators. Climate Research 39, 115–129. doi: 10.3354/cr00798
Elliott, K. H., Gaston, A. J., and Crump, D. (2010). Sex-specific behavior by a monomorphic seabird represents risk partitioning. Behav. Ecol. 21, 1024–1032. doi: 10.1093/beheco/arq076
Elliott, K. H., Woo, K. J., Gaston, A. J., Benvenuti, S., Dall'Antonia, L., and Davoren, G. K. (2009). Central-place foraging in an arctic seabird provides evidence for Storer-Ashmole's halo. Auk 126, 613–625. doi: 10.1525/auk.2009.08245
Fieberg, J., and Kochanny, C. O. (2005). Quantifying home-range overlap: the importance of the utilization distribution. J. Wildl. Manage. 69, 1346–1359. doi: 10.2193/0022-541X(2005)69[1346:QHOTIO]2.0.CO;2
Forero, M. G., Hobson, K. A., Bortolotti, G. R., Donázar, J. A., Bertellotti, M., and Blanco, G. (2002). Food resource utilisation by the Magellanic penguin evaluated through stable-isotope analysis: segregation by sex and age and influence on offspring quality. Mar. Ecol. Prog. Ser. 234, 289–299. doi: 10.3354/meps234289
Furness, R., and Birkhead, T. (1984). Seabird colony distributions suggest competition for food supplies during the breeding season. Nature 311, 655–656. doi: 10.1038/311655a0
Gaston, A. J., Ydenberg, R. C., and Smith, G. J. (2007). Ashmole's halo and population regulation in seabirds. Mar. Ornithol. 35, 119–126.
Gilardi, J. D. (1992). Sex-specific foraging distributions of brown boobies, in the Eastern Tropical Pacific. Colon. Waterbirds 15, 148–151. doi: 10.2307/1521367
González-Solís, J., Croxall, J. P., and Wood, A. G. (2000). Foraging partitioning between giant petrels Macronectes spp. and its relationship with breeding population changes at bird Island, South Georgia. Mar. Ecol. Prog. Ser. 204, 279–288. doi: 10.3354/meps204279
Goschen, W. S., and Schumann, E. H. (2011). The Physical Oceanographic Processes of Algoa Bay, with Emphasis on the Western Coastal Region. South African Environmental Observation Network (SAEON) and the Institute of Maritime Technology (IMT). IMT document number: PO106-10000-730002, 1–84.
Granadeiro, J., Nunes, M., Silva, M., and Furness, R. (1998). Flexible foraging strategy of Cory's shearwater, Calonectris diomedea, during the chick-rearing period. Anim. Behav. 56, 1169–1176. doi: 10.1006/anbe.1998.0827
Green, D. B., Coetzee, J. C., Rishworth, G. M., and Pistorius, P. A. (2015). Foraging distributions of Cape gannets in relation to oceanographic features, prey availability and marine protected areas. Mar. Ecol. Prog. Ser. 537, 277–288. doi: 10.3354/meps11428
Green, D. B., Klages, N. T. W., Crawford, R. J. M., Coetzee, J. C., Dyer, B. M., Rishworth, G. M., et al. (2014). Dietary change in Cape gannets reflects distributional and demographic shifts in two South African commercial fish stocks. ICES J. Mar. Sci. 72, 771–781. doi: 10.1093/icesjms/fsu203
Grémillet, D., Dell'Omo, G., Ryan, P. G., Peters, G., Ropert-Coudert, Y., and Weeks, S. J. (2004). Offshore diplomacy, or how seabirds mitigate intra-specific competition: a case study based on GPS tracking of Cape gannets from neighbouring colonies. Mar. Ecol. Prog. Ser. 268, 265–279. doi: 10.3354/meps268265
Guinet, C., Dubroca, L., Lea, M. A., Goldsworthy, S., Cherel, Y., Duhamel, G., et al. (2001). Spatial distribution of foraging in female Antarctic fur seals Arctocephalus gazella in relation to oceanographic variables: a scale-dependent approach using geographic information systems. Mar. Ecol. Prog. Ser. 219, 251–264. doi: 10.3354/meps219251
Harding, A. M., Piatt, J. F., and Schmutz, J. A. (2007). Seabird behavior as an indicator of food supplies: sensitivity across the breeding season. Mar. Ecol. Prog. Ser. 352, 269–274. doi: 10.3354/meps07072
Hennicke, J. C., and Weimerskirch, H. (2014). Coping with variable and oligotrophic tropical waters: foraging behaviour and flexibility of the Abbott's booby Papasula abbotti. Mar. Ecol. Prog. Ser. 499, 259–273. doi: 10.3354/meps10664
Huin, N. (2002). Foraging distribution of the black-browed albatross, Thalassarche melanophris, breeding in the Falkland Islands. Aquat. Conserv. 12, 89–99. doi: 10.1002/aqc.479
Jakubas, D., Iliszko, L., Wojczulanis-Jakubas, K., and Stempniewicz, L. (2012). Foraging by little auks in the distant marginal sea ice zone during the chick-rearing period. Polar Biol. 35, 73–81. doi: 10.1007/s00300-011-1034-x
Jarvis, M. (1974). The ecological significance of clutch size in the South African Gannet (Sula capensis (Lichtenstein). J. Anim. Ecol. 43, 1–17. doi: 10.2307/3154
Johnson, C. J., Parker, K. L., and Heard, D. C. (2001). Foraging across a variable landscape: behavioral decisions made by woodland caribou at multiple spatial scales. Oecologia 127, 590–602. doi: 10.1007/s004420000573
Lescroel, A., Ridoux, V., and Bost, C. A. (2004). Spatial and temporal variation in the diet of the gentoo penguin (Pygoscelis papua) at Kerguelen Islands. Polar Biol. 27, 206–216. doi: 10.1007/s00300-003-0571-3
Lewis, S., Benvenuti, S., Dall'Antonia, L., Griffiths, R., Money, L., Sherratt, T. N., et al. (2002). Sex-specific foraging behaviour in a monomorphic seabird. Proc. R. Soc. Lond. B 269, 1687–1693. doi: 10.1098/rspb.2002.2083
Lewis, S., Schreiber, E. A., Daunt, F., Schenk, G. A., Orr, K., Adams, A., et al. (2005). Sex-secific foraging in tropical boobies: does size matter? IBIS 147, 408–414. doi: 10.1111/j.1474-919x.2005.00428.x
Lewis, S., Sherratt, T., Hamer, K., and Wanless, S. (2001). Evidence of intra-specific competition for food in a pelagic seabird. Nature 412, 816–819. doi: 10.1038/35090566
Magalhães, M., Santos, R., and Hamer, K. (2008). Dual-foraging of Cory's shearwaters in the Azores: feeding locations, behaviour at sea and implications for food provisioning of chicks. Mar. Ecol. Prog. Ser. 359, 283–293. doi: 10.3354/meps07340
McConnell, B., Chambers, C., and Fedak, M. (1992). Foraging ecology of southern elephant seals in relation to the bathymetry and productivity of the Southern Ocean. Antarct. Sci. 4, 393–398. doi: 10.1017/S0954102092000580
Montevecchi, W., Benvenuti, S., Garthe, S., Davoren, G., and Fifield, D. (2009). Flexible foraging tactics by a large opportunistic seabird preying on forage-and large pelagic fishes. Mar. Ecol. Prog. Ser. 385, 295–306. doi: 10.3354/meps08006
Moseley, C., Grémillet, D., Connan, M., Ryan, P. G., Mullers, R. H. E., van der Lingen, C. D., et al. (2012). Foraging ecology and ecophysiology of Cape gannets from colonies in contrasting feeding environments. J. Exp. Mar. Biol. Ecol. 422–423, 29–38. doi: 10.1016/j.jembe.2012.04.002
Mullers, R. H. E., and Navarro, R. A. (2010). Foraging behaviour of Cape gannets as an indicator of colony health status. Endanger. Species Res. 12, 193–202. doi: 10.3354/esr00306
Mullers, R. H. E., Navarro, R. A., Crawford, R. J. M., and Underhill, L. G. (2009). The importance of lipid-rich fish prey for Cape gannet chick growth: are fishery discards an alternative? ICES J. Mar. Sci. 66, 2244–2252. doi: 10.1093/icesjms/fsp210
Mullers, R. H. E., Navarro, R. A., Underhill, L. G., and Visser, G. H. (2007). “Breeding in a Dynamic System: intra- and Inter-seasonal Variability in Foraging Behaviour and Chick Growth of Cape Gannets,” in Final Report of the BCLME (Benguela Current Large Marine Ecosystem) Project on Top Predators as Biological Indicators of Ecosystem Change in the BCLM, ed S. P. Kirkman (Cape Town: Avian Demography Unit, University of Cape Town).
Mullers, R. H. E., and Tinbergen, J. M. (2009). Parental provisioning behaviour predicts survival of Cape gannet chicks under poor conditions. Ardea 97, 89–98. doi: 10.5253/078.097.0111
Nelson, J. B. (1978). “African or Cape gannet,” in The Sulidae Gannets and boobies, ed J. Nelson (Oxford: Oxford University Press), 231–265.
Owen, E., Daunt, F., Moffat, C., Elston, D. A., Wanless, S., and Thompson, P. (2013). Analysis of fatty acids and fatty alcohols reveals seasonal and sex-specific changes in the diets of seabirds. Mar. Biol. 160, 987–999. doi: 10.1007/s00227-012-2152-x
Peck, D. R., and Congdon, B. C. (2006). Sex-specific chick provisioning and diving behaviour in the wedge-tailed shearwater Puffinus pacificus. J. Avian Biol. 37, 245–251. doi: 10.1111/j.2006.0908-8857.03558.x
Phillips, R., Silk, J., Phalan, B., Catry, P., and Croxall, J. (2004). Seasonal sexual segregation in two Thalassarche albatross species: competitive exclusion, reproductive role specialization or foraging niche divergence? Proc. R. Soc. Lond. Ser. B 271, 1283–1291. doi: 10.1098/rspb.2004.2718
Piatt, J. F., and Sydeman, W. J. (2007). Seabirds as indicators of marine ecosystems. Mar. Ecol. Prog. Ser. 352, 199–309. doi: 10.3354/meps07070
Pichegru, L., Cook, T., Handley, J., Voogt, N., Watermeyer, J., Nupen, L., et al. (2013). Sex-specific foraging behaviour and a field sexing technique for endangered African penguins. Endanger. Species Res. 19, 255–264. doi: 10.3354/esr00477
Pichegru, L., Ryan, P. G., Crawford, R. J., van der Lingen, C. D., and Grémillet, D. (2010). Behavioural inertia places a top marine predator at risk from environmental change in the Benguela upwelling system. Mar. Biol. 157, 537–544. doi: 10.1007/s00227-009-1339-2
Pichegru, L., Ryan, P. G., van der Lingen, C. D., Coetzee, J., Ropert-Coudert, Y., and Grémillet, D. (2007). Foraging behaviour and energetics of Cape gannets Morus capensis feeding on live prey and fishery discards in the Benguela upwelling system. Mar. Ecol. Prog. Ser. 350, 127–136. doi: 10.3354/meps07128
Pinet, P., Jaquemet, S., Phillips, R. A., and Le Corre, M. (2012). Sex-specific foraging strategies throughout the breeding season in a tropical, sexually monomorphic small petrel. Anim. Behav. 83, 979–989. doi: 10.1016/j.anbehav.2012.01.019
Pistorius, P. A., Hindell, M. A., Tremblay, Y., and Rishworth, G. M. (2015). Weathering a dynamic seascape: influences of wind and rain on a seabird's year-round activity budgets. PLoS ONE 10:e0142623. doi: 10.1371/journal.pone.0142623
R Core Team (2015). R: A Language and Environment for Statistical Computing. Vienna: R Foundation for Statistical Computing.
Ricklefs, R. E. (1983). Some considerations on the reproductive energetics of pelagic seabirds. Stud. Avian Biol. 8, 84–94.
Rishworth, G. M., Connan, M., Green, D. B., and Pistorius, P. A. (2014a). Sex differentiation based on the gular stripe in the apparently monomorphic Cape gannet. Afr. Zool. 49, 107–112. doi: 10.3377/004.049.0115
Rishworth, G. M., and Pistorius, P. A. (2015). Protection and provisioning: the role of parental behaviour in terms of chick growth and survival in a pelagic seabird. Mar. Ecol. Prog. Ser. 530, 153–162. doi: 10.3354/meps11317
Rishworth, G. M., Tremblay, Y., Green, D. B., Connan, M., and Pistorius, P. A. (2014b). Drivers of time-activity budget variability during breeding in a pelagic seabird. PLoS ONE 9:e116544. doi: 10.1371/journal.pone.0116544
Rishworth, G. M., Tremblay, Y., Green, D. B., and Pistorius, P. A. (2014c). An automated approach towards measuring time-activity budgets in colonial seabirds. Methods Ecol. Evol. 5, 854–863. doi: 10.1111/2041-210X.12213
Russell, R. W., Hunt Jr., G. L., Coyle, K. O., and Cooney, R. T. (1992). Foraging in a fractal environment: spatial patterns in a marine predator-prey system. Landsc. Ecol. 7, 195–209. doi: 10.1007/BF00133310
Schumann, E. (1987). The coastal ocean off the east coast of South Africa. Trans. R. Soc. South Afr. 46, 215–229. doi: 10.1080/00359198709520125
Shaffer, S. A., Costa, D. P., and Weimerskirch, H. (2003). Foraging effort in relation to the constraints of reproduction in free-ranging albatrosses. Funct. Ecol. 17, 66–74. doi: 10.1046/j.1365-2435.2003.00705.x
Soanes, L. M., Arnould, J. P., Dodd, S. G., Sumner, M. D., and Green, J. A. (2013). How many seabirds do we need to track to define home-range area? J. Appl. Ecol. 50, 671–679. doi: 10.1111/1365-2664.12069
Sommerfeld, J., Kato, A., Ropert-Coudert, Y., Garthe, S., Wilcox, C., and Hindell, M. A. (2015). Flexible foraging behaviour in a marine predator, the Masked booby (Sula dactylatra), according to foraging locations and environmental conditions. J. Exp. Mar. Biol. Ecol. 463, 79–86. doi: 10.1016/j.jembe.2014.11.005
Stahl, J., and Sagar, P. (2000). Foraging strategies of southern Buller's albatrosses Diomedea b. bulleri breeding on The Snares, New Zealand. J. R. Soc. N.Z. 30, 299–318. doi: 10.1080/03014223.2000.9517624
Stauss, C., Bearhop, S., Bodey, T. W., Garthe, S., Gunn, C., Grecian, W. J., et al. (2012). Sex-specific foraging behaviour in northern gannets Morus bassanus: incidence and implications. Mar. Ecol. Prog. Ser. 457, 151–162. doi: 10.3354/meps09734
Tew Kai, E., Benhamou, S., Lingen, C. D., Coetzee, J. C., Pichegru, L., Ryan, P. G., et al. (2013). Are Cape gannets dependent upon fishery waste? A multi-scale analysis using seabird GPS-tracking, hydro-acoustic surveys of pelagic fish and vessel monitoring systems. J. Appl. Ecol. 50, 659–670. doi: 10.1111/1365-2664.12086
Wanless, S., Harris, M. P., and Morris, J. A. (1994). Factors affecting daily ctivity budgets of South Georgian shags during chick rearing at Bird Island, South Georgia. Condor 97, 550–558. doi: 10.2307/1369040
Weimerskirch, H. (1998). How can a pelagic seabird provision its chick when relying on a distant food resource? Cyclic attendance at the colony, foraging decision and body condition in sooty shearwaters. J. Anim. Ecol. 67, 99–109. doi: 10.1046/j.1365-2656.1998.00180.x
Weimerskirch, H. (2007). Are seabirds foraging for unpredictable resources? Deep Sea Res. Part II 54, 211–223. doi: 10.1016/j.dsr2.2006.11.013
Weimerskirch, H., Chastel, O., Ackermann, L., Chaurand, T., Cuenot-Chaillet, F., Hindermeyer, X., et al. (1994). Alternate long and short foraging trips in pelagic seabird parents. Anim. Behav. 47, 472–476. doi: 10.1006/anbe.1994.1065
Weimerskirch, H., Le Corre, M., Ropert-Coudert, Y., Kato, A., and Marsac, F. (2006). Sex-specific foraging behaviour in a seabird with reversed sexual dimorphism: the red-footed booby. Oecologia 146, 681–691. doi: 10.1007/s00442-005-0226-x
Weimerskirch, H., Shaffer, S. A., Tremblay, Y., Costa, D. P., Gadenne, H., Kato, A., et al. (2009). Species- and sex-specific differences in foraging behaviour and foraging zones in blue-footed and brown boobies in the Gulf of California. Mar. Ecol. Prog. Ser. 391, 267–278. doi: 10.3354/meps07981
Welcker, J., Harding, A., Karnovsky, N. J., Steen, H., Strøm, H., and Gabrielsen, G. W. (2009). Flexibility in the bimodal foraging strategy of a high Arctic alcid, the little auk Alle alle. J. Avian Biol. 40, 388–399. doi: 10.1111/j.1600-048X.2008.04620.x
Wells, M. R., Angel, L. P., and Arnould, J. P. Y. (2016). Habitat-specific foraging strategies in Australasian gannets. Biol. Open 5, 921–927. doi: 10.1242/bio.018085
Widmann, M., Kato, A., Raymond, B., Angelier, F., Arthur, B., Chastel, O., et al. (2015). Habitat use and sex-specific foraging behaviour of Adélie penguins throughout the breeding season in Adélie Land, East Antarctica. Mov. Ecol. 3, 1–10. doi: 10.1186/s40462-015-0052-7
Keywords: Morus capensis, foraging ecology, prey shifts, sex-specific, behavioral plasticity, chick rearing
Citation: Botha JA and Pistorius PA (2018) Variability in the Foraging Distribution and Diet of Cape Gannets between the Guard and Post-guard Phases of the Breeding Cycle. Front. Mar. Sci. 5:15. doi: 10.3389/fmars.2018.00015
Received: 25 September 2017; Accepted: 16 January 2018;
Published: 20 February 2018.
Edited by:
Rob Harcourt, Macquarie University, AustraliaReviewed by:
Richard Reina, Monash University, AustraliaCopyright © 2018 Botha and Pistorius. This is an open-access article distributed under the terms of the Creative Commons Attribution License (CC BY). The use, distribution or reproduction in other forums is permitted, provided the original author(s) and the copyright owner are credited and that the original publication in this journal is cited, in accordance with accepted academic practice. No use, distribution or reproduction is permitted which does not comply with these terms.
*Correspondence: Jonathan A. Botha, Ym90aGFqYUBnbWFpbC5jb20=
Disclaimer: All claims expressed in this article are solely those of the authors and do not necessarily represent those of their affiliated organizations, or those of the publisher, the editors and the reviewers. Any product that may be evaluated in this article or claim that may be made by its manufacturer is not guaranteed or endorsed by the publisher.
Research integrity at Frontiers
Learn more about the work of our research integrity team to safeguard the quality of each article we publish.