- 1Plymouth Marine Laboratory, Plymouth, Devon, United Kingdom
- 2National Centre for Earth Observation, Plymouth Marine Laboratory, Plymouth, United Kingdom
- 3Lowestoft Laboratory, Centre for Environment, Fisheries and Aquaculture Science, Suffolk, United Kingdom
- 4Scripps Institution of Oceanography, University of California, San Diego, La Jolla, CA, United States
- 5School of Biological and Marine Sciences, University of Plymouth, Plymouth, United Kingdom
- 6Department of Geography and Planning, University of Liverpool, Liverpool, United Kingdom
- 7Chatham and Clarendon Grammar School, Ramsgate, United Kingdom
- 8Sir Alister Hardy Foundation for Ocean Science, Plymouth, United Kingdom
Accurate observations of the Earth system are required to understand how our planet is changing and to help manage its resources. The aquatic environment—including lakes, rivers, wetlands, estuaries, coastal and open oceans—is a fundamental component of the Earth system controlling key physical, biological, and chemical processes that allow life to flourish. Yet, this environment is critically undersampled in both time and space. New and cost-effective sampling solutions are urgently needed. Here, we highlight the potential to improve aquatic sampling by tapping into recreation. We draw attention to the vast number of participants that engage in aquatic recreational activities and argue, based on current technological developments and recent research, that the time is right to employ recreational citizens to improve large-scale aquatic sampling efforts. We discuss the challenges that need to be addressed for this strategy to be successful (e.g., sensor integration, data quality, and citizen motivation), the steps needed to realize its potential, and additional societal benefits that arise when engaging citizens in scientific sampling.
1. Introduction
Integrated Earth system science requires systematic and sustained observations (Alverson and Baker, 2006). The aquatic environment, incorporating both marine and fresh-water, plays a vital role in the functioning of our planet. For instance, the ocean has taken up 90% of the energy imbalance caused by global warming (Trenberth and Fasullo, 2013), is responsible for ~50% of net primary production (Field et al., 1998), and has absorbed a quarter of anthropogenic CO2 emissions (Le Quéré et al., 2015). Inland waters impact the global cycling of key climatically-relevant elements and compounds, such as carbon and methane (Battin et al., 2009; Bastviken et al., 2011; Raymond et al., 2013), are regions of high biodiversity (Dudgeon et al., 2006), support a range of ecosystem services (Postel and Carpenter, 1997), and are sensitive to environmental change (Williamson et al., 2008). Therefore, monitoring the status of the aquatic environment is essential in understanding how our planet is changing.
Key environmental variables, or environmental indicators (Platt and Sathyendranath, 2008), are used to track the state of the aquatic environment. These include physical (e.g., temperature, salinity, turbidity), biological (e.g., phytoplankton abundance and composition, macrophyte abundance), and chemical (e.g., nutrient concentrations, oxygen, pH, toxic contaminants) indicators (Ferreira et al., 2007). There is a requirement for observations of these indicators for monitoring climate and biodiversity, and for management of water quality, conservation and resources (Borja, 2005; Ferreira et al., 2007). Yet, despite the demand for observations and its important role in Earth system science, the aquatic environment is critically undersampled in time and space (Huang and Xia, 2001; Alverson and Baker, 2006; Davidson et al., 2007; Defeo et al., 2009), impeding our ability to understand how our planet functions, how it is responding to human activity, and how to manage its resources sustainably.
In recent years, satellite remote-sensing (e.g., ocean-color, thermal radiometry, and altimetry) and autonomous observations (e.g., Argo floats and gliders) have drastically improved sampling efforts and our understanding of the role the aquatic environment plays in the Earth system (McClain, 2009; Merchant, 2013; Watson et al., 2015; Dall'Olmo et al., 2016; Riser et al., 2016). Yet, these techniques have their limitations, often require independent in situ observations for calibration and verification, and are limited by the number of indicators that can be measured. Furthermore, future support to maintain these monitoring systems remains uncertain (Kintisch, 2015). New forms of innovation are needed urgently to help meet the requirements of systematic and sustained aquatic observations (Smith et al., 2015), one of which could be making use of citizens who regularly frequent the aquatic environment (Brewin et al., 2015; Bresnahan et al., 2016; Hut et al., 2016; Wright et al., 2016).
Citizen science typically involves recruiting a network of citizens to undertake a scientific task that would otherwise be performed by professionals. It has been found to: successfully tackle difficult, expensive, and laborious fieldwork (Dickinson et al., 2012; Schwartz et al., 2012; Gura, 2013); promote public understanding of science (Trumbull et al., 2000; Brossard et al., 2005; Wiggins and Crowston, 2011; Schläppy et al., 2017); and contribute to marine policy (Hyder et al., 2015). Considering the high cost of traditional aquatic sampling (e.g., ship or boat hire), citizen science has much untapped potential in limnology and oceanography (Lauro et al., 2014; Cigliano et al., 2015; Busch et al., 2016b; Garcia-Soto et al., 2017; Hyder et al., 2017).
To date, the majority of aquatic citizen science projects have been targeted studies focused on animals and flora, or issues such as contamination and marine litter, typically in accessible coastal habitats (Schrope, 2012; Thiel et al., 2014; Nelms et al., 2017). Recently, techniques have been developed to attach miniaturized sensors to recreational citizens who immerse themselves in the aquatic environment in order to measure key indicators such as water temperature (e.g., Brewin et al., 2015; Bresnahan et al., 2016; Hut et al., 2016; Wright et al., 2016), akin to attaching sensors to marine mammals (Fedak, 2004). In this paper, we highlight the potential in this approach. We discuss the vast number of recreational water users directly interacting with the aquatic environment, the regional extent of these activities, and recent technological advances that could help realize this potential. We discuss the impact, benefits, and the challenges that need to be overcome for successful integration of such an approach into existing monitoring systems.
2. Participation in Aquatic Recreation
Given the economic, social, and ecological impact of recreational activities on the environment (Cisneros-Montemayor and Sumaila, 2010; Papathanasopoulou et al., 2016), there have been efforts to quantify participation and revenue generated by these activities globally. For instance, focusing on three recreational activities (whale watching, diving and recreational fishing), Cisneros-Montemayor and Sumaila (2010) estimate 121 million people participate per year, generating 47 billion USD income and supporting one million jobs. Globally, it has been estimated that there are around 10 million surfers (Buckley, 2002) and 10 million divers (SCUBA and snorkeling; Cisneros-Montemayor and Sumaila, 2010), with the population steadily increasing. Yet there are very few studies documenting the entire spectrum of aquatic recreational activities and those that are available, typically focus on specific countries.
Two countries where such surveys have been attempted are the UK and the US. Table 1 shows estimates of the number of UK and US participants (for the US these numbers are for salt-water only, including mixed fresh-salt-water in tidal portions of rivers and bays) for a selection of recreational activities that directly interact with the aquatic environment, based on two surveys (Leeworthy and Wiley, 2001; Arkenford, 2015). Though these are rough numbers computed by combining watersports surveys with demographic analysis (Leeworthy and Wiley, 2001; Arkenford, 2015), they suggest nearly 125 million people engage in aquatic recreational activities in the UK and US under the selection of activities listed in Table 1. If we estimate the frequency of participation as roughly 10 times a year—based on the average number of days an individual participated in activities listed in Table 1 for the Leeworthy and Wiley (2001) study—we predict participants interact with the aquatic environment approximately 1.25 billion times per year in the UK and US. Considering participation numbers in the US surveys (Leeworthy and Wiley, 2001) exclude purely fresh-water, this estimate is likely conservative. If even a small fraction of this population were equipped with sensors to measure environmental indicators, there is potential to acquire vast volumes of data (e.g., 1% of participants could acquire approximately 12.5 million independent measurements of environmental indicators per year). This could drastically enhance sampling coverage of environmental indicators in UK and US waters, and other countries passionate about aquatic recreation.
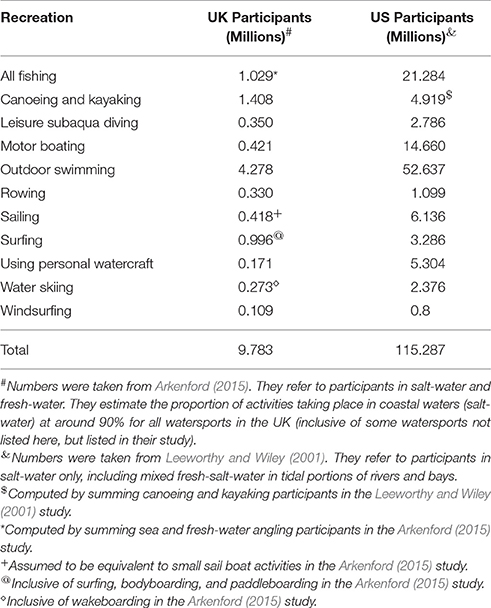
Table 1. Number of UK and US participants for various recreational activities that directly interact with the aquatic environment.
3. Geographical Distribution of Aquatic Recreation
Aquatic recreational activities occur along vast stretches of the coastline and throughout many inland water bodies. Figure 1 shows an example of sites for two recreational activities: surfing and SCUBA diving. These two recreational activities alone cover a wide expanse of the global coastline and many lakes. Virtually the entire coastlines of North America and Europe have surfing beaches or dive sites (Figures 1A,B,D). Regions with limited aquatic monitoring infrastructure in the Caribbean (Figure 1C), Southeast Asia (Figure 1E), South America, Central America, India, Africa, and Pacific Islands are populated by surfing and SCUBA diving sites (Figure 1A). Many of these regions are highly vulnerable to climate change. A radical increase in the spatial coverage of environmental indicators in coastal and inland waters could be possible through tapping into aquatic recreational activities such as surfing and SCUBA diving.
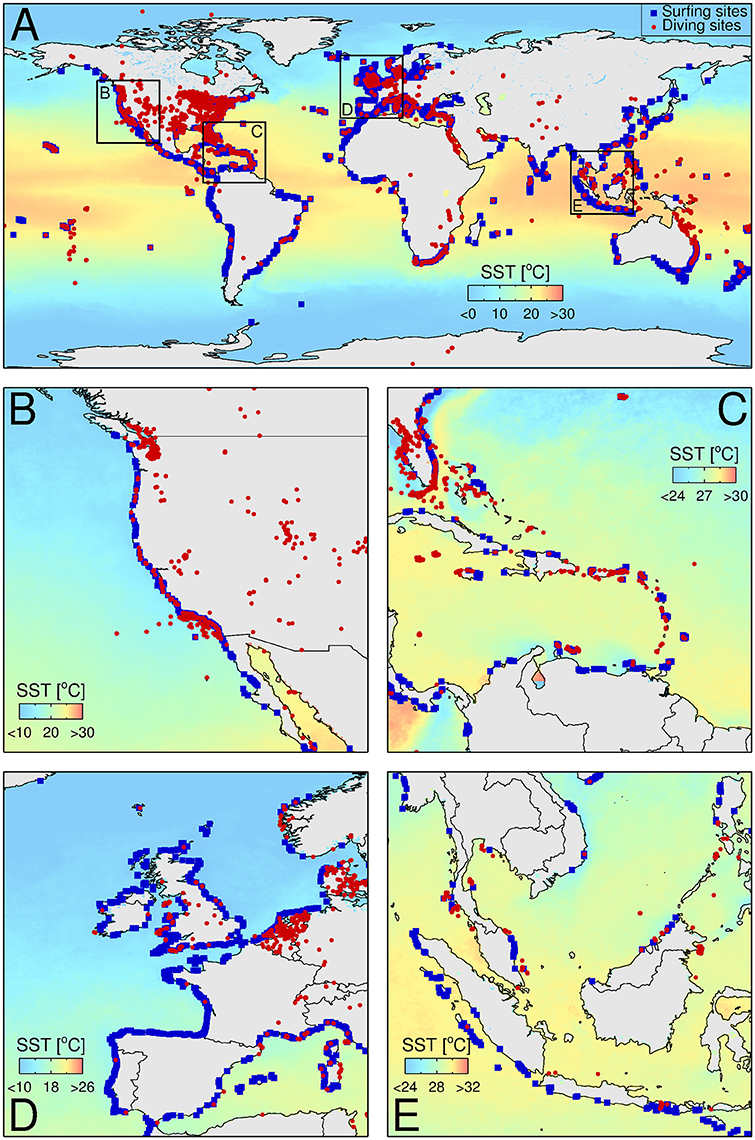
Figure 1. Locations of surfing and dive sites around the globe. Positions were extracted from a wave prediction website (http://magicseaweed.com/) and from an open database of dive sites (http://www.divesites.com/) on the 18th July 2017. Panel (A) shows locations over the global ocean, (B) focusing on the west coast of North America, (C) the Caribbean, (D) western Europe and (E) Southeast Asia. Background image is an annual sea surface temperature (SST) composite for the year 2016 from the NASA MODIS-Aqua sensor (NASA, 2014). Note that the color scales varies between sub-figures.
Coastal and inland waters, where many of these activities take place, support high levels of aquatic biodiversity (Davies et al., 2008; Tittensor et al., 2010), provide a wide range of ecosystem services to humans (Costanza et al., 1997, 2014; Adrian et al., 2009; van den Belt, 2011), and are important to global biogeochemical cycles (Cai, 2011; Bauer et al., 2013; Regnier et al., 2013). These waters are also susceptible to anthropogenic impacts (Halpern et al., 2008), such as eutrophication (Callisto et al., 2014; Rabalais et al., 2014), marine pollution (Islam and Tanaka, 2004) and acidification (Cai et al., 2011; Kelly et al., 2011; Phillips et al., 2015). They can be difficult to sample using conventional aquatic monitoring methods, which are not designed to capture the high temporal and spatial variability of these regions, and are expensive to operate and maintain. New cost effective monitoring systems are required, which could include harnessing aquatic recreation.
4. Existing Studies and Technological Developments
There is an emerging field of research investigating the use of aquatic recreational sports as platforms for environmental data collection. Lauro et al. (2014) highlighted the potential for harnessing the thousands of sailing vessels that continuously cruise remote parts of the oceans, for oceanographic monitoring. Sensor packages for automatic oceanic measurements on sailing boats have been developed1 and programs established to help realize the potential of using sailing vessels for environmental monitoring2.
Wright et al. (2016) obtained over 7,000 temperature profiles from recreational dive computers worn by SCUBA divers, demonstrating that dive computers can augment existing monitoring systems across the globe, but especially in under-sampled or highly variable coastal environments. Boss and Zaneveld (2003) measured the optical properties of a variety of shallow waters in the Bahamas by mounting an optical sampling package to a SCUBA diver, observing significant variations in optical properties related to the bottom substrate and associated biogeochemical processes. Successful efforts have been made to use observations and video camera data from divers and spear-fishermen, for assessing fish assemblages and for fisheries management (Ward-Paige and Lotze, 2011; Ward-Paige et al., 2011; Bulleri and Benedetti-Cecchi, 2014; Goetze et al., 2015; Scyphers et al., 2015). These observations have the potential to support existing citizen science programs focused on monitoring coral reef health3. Hut et al. (2016) have developed a prototype temperature-sensing pair of waders, typically worn by fishermen, that are designed to measure the temperature at the stream-bed, a useful variable for studying the hyporheic zone.
Bresnahan et al. (2016) developed the WavepHOx, a compact pH, oxygen, and temperature sensor package designed to be mounted on small mobile platforms such as stand up paddleboards (SUPs). They used the WavepHOx and SUP to map variability in oxygen and carbonate chemistry across a shallow and densely vegetated seagrass ecosystem that would have been difficult to access with conventional platforms. Brewin et al. (2015, 2017) equipped a small group of surfers with GPS recorders and temperature sensors to measure sea-surface temperature (SST). They used the data to reveal a significant reduction in performance of satellites at retrieving SST at the coastline (Brewin et al., 2017), and suggested measurements collected by surfers and other recreational watersports could help improve satellite algorithms in nearshore regions. Recently, a surfboard fin (Smartfin4) has been developed to measure temperature, motion, and geo-location, with plans to integrate biogeochemical sensors, beginning with pH. The fin is ideally suited for widespread citizen science use, making use of wireless data transfer capabilities (Bluetooth), mobile data upload, and cloud-based data storage and processing.
These examples, the majority of which have appeared in the past few years, highlight a growing momentum to harness aquatic recreation for environmental sampling. With a push toward environmental sensors becoming smaller and cheaper, there is scope to expand the range of environmental indicators that could be measured, for instance, phytoplankton fluorescence (Leeuw et al., 2013; Zeng and Li, 2015), light attenuation (Bardaji et al., 2016), water color (Busch et al., 2016a), pH and oxygen (Larsen et al., 2011). Sensor developments for monitoring nutrient concentrations (Beaton et al., 2011) and toxic pollutants (Lafleur et al., 2010) may one day be feasible from such platforms. New manufacturing techniques, including 3D printers (Mohammed, 2016), small low cost electronics (Leeuw et al., 2013)5, embedded computing (Williams et al., 2014)6 and mobile phones (Friedrichs et al., 2017) are expanding possibilities further. With growing developments in wireless sensor networks (Albaladejo et al., 2010), cloud data storage, and visualization (Müller et al., 2016), the feasibility of harnessing recreational sports for aquatic monitoring is increasing. Coupling these measurements with conventional in situ monitoring (e.g., ships and buoys) and other developing in situ technologies, such as autonomous beach buoy systems (Shively et al., 2016), tagging of marine vertebrates (Fedak, 2004), and coastal gliders (Rudnick et al., 2004), could drastically improve aquatic monitoring.
5. Additional Impacts and Benefits
Tagging recreational watersports participants with environmental sensors (for instance, surfers sampling in the nearshore during storm conditions, or divers sampling shallow and otherwise inaccessible coral reefs) can offer a cost effective option for data collection in otherwise inaccessible or energetic environments. There is an increasing reliance placed on using models and satellite observations in coastal regions, to understand impacts of climate change, ocean acidification, and sea-level rise, despite not being well verified with field data (Livingston, 2014). Data collected by watersports participants could be used for validating and improving satellite observations and model simulations in coastal regions. Additionally, such high resolution coastal data could augment our understanding of coastal physics (e.g., small scale patchiness and mass and heat transfer in the dynamic coastal zone) and coastal ecology (e.g., habitat mapping; Scales et al., 2017).
Shifting public opinion on issues surrounding global environmental change can be challenging (Lorenzoni et al., 2007; Brulle et al., 2012). It has been shown that individuals involved in citizen science are more likely to engage in community development, local issues, engage constructively in policy, and become stewards of the environment (Garcia-Soto et al., 2017). Involvement in citizen science projects and recreational activities can influence behavior, break down social barriers, and address recognized gaps in ocean literacy (Gelcich et al., 2014), giving participants the opportunity to inform policy, stimulate their intellectual capacity, engage in science, and help address issues facing the next generation (Garcia-Soto et al., 2017).
6. Challenges
Instrumenting watersports participants with environmental sensors is likely to be successful if certain challenges are dealt with. To ensure maximum uptake, the design of the sensor package requires a series of careful considerations. For instance:
• The sensor package must be tailored to the specific activity to minimize impact on the activity, with implications for weight and size requirements.
• The sensors should be appropriately positioned for quality data collection and to minimize damage from issues like the handling of equipment by participants.
• It must be determined whether a given environmental variable can feasibly be measured during a specific activity, at a suitable accuracy and precision for scientific and operational applications.
• Upfront and maintenance-related costs, likely to vary substantially with activity, are important considerations.
• Sufficient power and careful data management are critical.
• Finally, the technology used for data transfer needs to embrace the rapidly evolving field of digital technology, for instance, if mobile applications are used they need to keep up to date and compatible with mobile phone developments.
The sensor packages need to be made easily accessible to the recreational watersports communities, perhaps harnessing the rise in social media to attract interested participants, and be constructed such that the participants do not need to invest much effort in operation, calibration, maintenance, and data transfer.
A major challenge for any project looking to involve citizens in environmental monitoring is citizen motivation. While advocacy is proven to be the biggest motivator in marine citizen science (Garcia-Soto et al., 2017), the influence of gaming and competition can also play a large role (Cooper et al., 2010). The power of accurate positioning can be a big motivator for user uptake. Applications such as Strava, a GPS enabled smart-phone application designed to track recreational activities, are becoming increasingly popular. Coupled with developments in social media (e.g., Twitter and Facebook) and website visualization tools (e.g., Google Earth), users of GPS enabled mobile applications can view and share their GPS track and performance. This has already been shown as a possible tool to motivate surfers to integrate GPS sensors into their equipment (Barlow et al., 2014) and may offer an incentive for environmental data collection (Brewin et al., 2015). Data collectors need to be appropriately recognized for their contribution through regular communication channels that help maintain awareness and engagement.
As with any citizen science-based project, principles of good citizen science need to be considered (Garcia-Soto et al., 2017) as well as ethics (Riesch and Potter, 2014). Cautious uptake of citizen science data by the scientific community is often related to concerns on data quality. It is critical that data quality assurances are in place (Bonney et al., 2014), to ensure confident uptake by the scientific community and demonstrate to participants the power of the data they collect and its scientific value (Garcia-Soto et al., 2017). This can be done through good training practices and protocols, cross-checking with existing datasets and literature values, validation, sensor calibrations, monitoring of sensor drift and stability, database management, and data filtering (Garcia-Soto et al., 2017). To ensure scientific uptake, concerted efforts should be placed on quantifying uncertainties and providing these uncertainties with the data. The data should also be made compliant with existing conventions and standards, stored in appropriate data repositories, and made freely-available through open-access platforms, considering participant confidentiality if required.
Analysis and interpretation of the data collected should carefully consider and identify temporal and spatial data biases (Bonney et al., 2009). For instance, not many recreational watersports occur at night, such that data collected is likely to be biased toward day time conditions. Data collected will be biased toward conditions and locations preferable for that activity which could result in seasonal and geographical biases in data (e.g., for surfing in the UK, see Figure 6 of Brewin et al., 2015). In some cases, transparency about the motives and ambitions of participants might be needed to identify biases in data collection caused by any conflicts of interest. There also needs to be careful consideration on how the datasets can feed into existing monitoring, management, and policy programs, to ensure maximum impact (Hyder et al., 2015). Data collection for scientific research must follow rules of national jurisdiction, for instance, in marine waters data collection within a given Exclusive Economic Zone must conform with the United Nations Convention on the Law of the Sea7 article 56. For many countries, this will require prior authorization from the governing state.
These challenges can only be addressed through coordinated and sustained support from governmental, non-governmental, and private bodies, across national and international boundaries, and through interdisciplinary co-operation and engagement by stakeholders (including recreational participants, scientists, sensor developers, the watersports industry, monitoring agencies, and policy makers). Lessons should be learnt from existing autonomous observation programs (such as the Argo program8) and citizen science initiatives that have faced similar challenges (Garcia-Soto et al., 2017).
7. Summary
The aquatic environment is an essential component of our planet regulating its physics, biology and chemistry. Yet, it is critically undersampled in time and space, limiting our capability to understand and predict its response to natural and human perturbations. In this paper, we highlight the potential to improve aquatic sampling by tapping into recreation, particularly in dynamic and under-sampled shallow water environments. We emphasize the vast number of participants that engage in aquatic recreation and the wide geographical distribution of locations where aquatic recreation takes place, including regions with limited monitoring infrastructure that are highly vulnerable to climate change. We highlight existing research that has used aquatic recreation as a means to improve sampling efforts and discuss the challenges that need to be addressed for successful implementation at large scales. In light of recent technological developments, we are now poised to harness aquatic recreation for wide-scale environmental monitoring.
Author Contributions
The ideas presented stemmed from discussions and contributions by all the authors. RB structured a template for the manuscript with input from KH, prepared the figure with help from TJ, the table, and drafted a first version of the manuscript. All authors then contributed to subsequent versions of the manuscript.
Funding
RB, GD, and DR acknowledge financial support from the UK National Centre for Earth Observation. AA, PB, and TC received financial support from the Smartfin/Lostbird foundation. KH contribution was supported by Defra contracts MF1230 (Citizen Science Investigations), and MI001 (Management of Recreational Marine Fisheries).
Conflict of Interest Statement
AA, PB, and TC received financial support from the Smartfin/Lostbird foundation.
The other authors declare that the research was conducted in the absence of any commercial or financial relationships that could be construed as a potential conflict of interest.
Acknowledgments
The authors would like to thank NASA for MODIS-Aqua products used in Figure 1, and thank the websites http://magicseaweed.com/ and http://www.divesites.com/ that were used to extract locations on surfing and diving sites shown in Figure 1. We acknowledge and recognize all recreational citizens that have contributed to the citizen science projects mentioned in this paper.
Footnotes
1. ^http://subctech.eu/sensor_systems/sailing-meets-science/
2. ^https://plankton-planet.org/ and http://www.secchidisk.org/
3. ^http://www.coralwatch.org/ and http://www.reefcheck.org/
5. ^Cave Pearl Project, https://edwardmallon.wordpress.com/
6. ^https://www.arduino.cc/ and https://www.raspberrypi.org/
References
Adrian, R., O'Reilly, C. M., Zagarese, H., Baines, S. B., Hessen, D. O., Keller, W., et al. (2009). Lakes as sentinels of climate change. Limnol. Oceanogr. 54, 2283–2297. doi: 10.4319/lo.2009.54.6_part_2.2283
Albaladejo, C., Sánchez, P., Iborra, A., Soto, F., López, J. A., and Torres, R. (2010). Wireless sensor networks for oceanographic monitoring: a systematic review. Sensors 10, 6948–6968. doi: 10.3390/s100706948
Alverson, K., and Baker, D. J. (2006). Taking the pulse of the oceans. Science 314:1657. doi: 10.1126/science.1135358
Arkenford (2015). Watersports Participation Survey 2015 Executive Summary. Available online at: http://www.rya.org.uk/SiteCollectionDocuments/sportsdevelopment/Watersports_Participation_Survey_2015_Executive_Summary.pdf (Accessed August 25, 2017).
Bardaji, R., Sánchez, A. M., Simon, C., Wernand, M. R., and Piera, J. (2016). Estimating the underwater diffuse attenuation coefficient with a low-cost instrument: the KdUINO DIY buoy. Sensors 16:373. doi: 10.3390/s16030373
Barlow, M. J., Grestry, K., Findlay, M., Cooke, C. B., and Davidson, M. (2014). The effect of wave conditions and surfer ability on performance and the physiological response of recreational surfers. J. Strength Condition. Res. 28, 2946–2953. doi: 10.1519/JSC.0000000000000491
Bastviken, D., Tranvik, L. J., Downing, J. A., Crill, P. M., and Enrich-Prast, A. (2011). Freshwater methane emissions offset the continental carbon sink. Science 331:50. doi: 10.1126/science.1196808
Battin, T. J., Luyssaert, S., Kaplan, L. A., Aufdenkampe, A. K., Richter, A., and Tranvik, L. J. (2009). The boundless carbon cycle. Nat. Geosci. 2, 598–600. doi: 10.1038/ngeo618
Bauer, J. E., Cai, W. J., Raymond, P. A., Bianchi, T. S., Hopkinson, C. S., and Regnier, P. (2013). The changing carbon cycle of the coastal ocean. Nature 504, 61–70. doi: 10.1038/nature12857
Beaton, A. D., Sieben, V. J., Floquet, C. F. A., Waugh, E. M., Abi Kaed Bey, S., Ogilvie, I. R. G., et al. (2011). An automated microfluidic colourimetric sensor applied in situ to determine nitrite concentration. Sens. Actuat. B Chem. 156, 1009–1014. doi: 10.1016/j.snb.2011.02.042
Bonney, R., Cooper, C., Dickinson, J., Kelling, S., Phillips, T., Rosenberg, K., et al. (2009). Citizen science: a developing tool for expanding science knowledge and scientific literacy. BioScience 59, 977–984. doi: 10.1525/bio.2009.59.11.9
Bonney, R., Shirk, J., Phillips, T., Wiggins, A., Ballard, H., Miller-Rushing, A., et al. (2014). Next steps for citizen science. Science 343, 1436–1437. doi: 10.1126/science.1251554
Borja, A. (2005). The European water framework directive: a challenge for nearshore, coastal and continental shelf research. Contin. Shelf Res. 25, 1768–1783. doi: 10.1016/j.csr.2005.05.004
Boss, E., and Zaneveld, J. R. V. (2003). The effect of bottom substrate on inherent optical properties: evidence of biogeochemical processes. Limnol. Oceanogr. 48, 346–354. doi: 10.4319/lo.2003.48.1_part_2.0346
Bresnahan, P. J., Wirth, T., Martz, T. R., Andersson, A. J., Cyronak, T., D'Angelo, S., et al. (2016). A sensor package for mapping pH and oxygen from mobile platforms. Methods Oceanogr. 17, 1–13. doi: 10.1016/j.mio.2016.04.004
Brewin, R. J. W., de Mora, L., Billson, O., Jackson, T., Russell, P., Brewin, T. G., et al. (2017). Evaluating operational AVHRR sea surface temperature data at the coastline using surfers. Estuar. Coast. Shelf Sci. 196, 276–289. doi: 10.1016/j.ecss.2017.07.011
Brewin, R. J. W., de Mora, L., Jackson, T., Brewin, T. G., and Shutler, J. (2015). On the potential of surfers to monitor environmental indicators in the coastal zone. PLoS ONE 10:e0127706. doi: 10.1371/journal.pone.0127706
Brossard, D., Lewenstein, B., and Bonney, R. (2005). Scientific knowledge and attitude change: the impact of a citizen science project. Int. J. Sci. Educ. 9, 1099–1121. doi: 10.1080/09500690500069483
Brulle, R. J., Carmichael, J., and Jenkins, J. C. (2012). Shifting public opinion on climate change: an empirical assessment of factors influencing concern over climate change in the US, 2002–2010. Clim. Change 114, 169–188. doi: 10.1007/s10584-012-0403-y
Buckley, R. (2002). Surf tourism and sustainable development in Indo-Pacific Islands. I. The industry and the islands. J. Sustain. Tourism 10, 405–424. doi: 10.1080/09669580208667176
Bulleri, F., and Benedetti-Cecchi, L. (2014). Chasing fish and catching data: recreational spearfishing videos as a tool for assessing the structure of fish assemblages on shallow rocky reefs. Mar. Ecol. Prog. Ser. 506, 255–265. doi: 10.3354/meps10804
Busch, J. A., Bardaji, R., Ceccaroni, L., Friedrichs, A., Piera, J., Simon, C., et al. (2016a). Citizen bio-optical observations from coast-and ocean and their compatibility with ocean colour satellite measurements. Remote Sens. 8:879. doi: 10.3390/rs8110879
Busch, J. A., Price, I., Jeansou, E., Zielinski, O., and van der Woerd, H. J. (2016b). Citizens and satellites: assessment of phytoplankton dynamics in a NW Mediterranean aquaculture zone. Int. J. Appl. Earth Observ. Geoinf. 47, 40–49. doi: 10.1016/j.jag.2015.11.017
Cai, W. J. (2011). Estuarine and coastal ocean carbon paradox: CO2 sinks or sites of terrestrial carbon incineration? Annu. Rev. Mar. Sci. 3, 123–145. doi: 10.1146/annurev-marine-120709-142723
Cai, W. J., Hu, X., Huang, W. J., Murrell, M. C., Lehrter, J. C., Lohrenz, S. E., et al. (2011). Acidification of subsurface coastal waters enhanced by eutrophication. Nat. Geosci. 4, 766–770. doi: 10.1038/ngeo1297
Callisto, M., Molozzi, J., and Barbosa, J. L. E. (2014). “Eutrophication of lakes,” in Eutrophication: Causes, Consequences and Control, eds A. A. Ansari and S. S. Gill (Dordrecht: Springer Netherlands), 55–71.
Cigliano, J. A., Meyer, R., Ballard, H. L., Freitag, A., Phillips, T. B., and Wasser, A. (2015). Making marine and coastal citizen science matter. Ocean Coast. Manag. 115, 77–87. doi: 10.1016/j.ocecoaman.2015.06.012
Cisneros-Montemayor, A. M., and Sumaila, U. R. (2010). A global estimate of benefits from ecosystem-based marine recreation: potential impacts and implications for management. J. Bioecon. 12, 245–268. doi: 10.1007/s10818-010-9092-7
Cooper, S., Khatib, F., Treuille, A., Barbero, J., Lee, J., Beenen, M., et al. (2010). Predicting protein structures with a multiplayer online game. Nature 466, 756–760. doi: 10.1038/nature09304
Costanza, R., d'Arge, R., de Groot, R., Farberk, S., Grasso, M., Hannon, B., et al. (1997). The value of the world's ecosystem services and natural capital. Nature 387, 253–260.
Costanza, R., de Groot, R., Sutton, P., van der Ploeg, S., Anderson, S. J., Kubiszewski, I., et al. (2014). Changes in the global value of ecosystem services. Glob. Environ. Change 26, 152–158. doi: 10.1016/j.gloenvcha.2014.04.002
Dall'Olmo, G., Dingle, J., Polimene, L., Brewin, R. J. W., and Claustre, H. (2016). Substantial energy input to the mesopelagic ecosystem from the seasonal mixed-layer pump. Nat. Geosci. 9, 820–823. doi: 10.1038/ngeo2818
Davidson, M., Koningsveld, M. V., de Kruif, A., Rawson, J., Holman, R., Lamberti, A., et al. (2007). The Coastview project: developing video-derived Coastal State Indicators in support of coastal zone management. Coast. Eng. 54, 463–475. doi: 10.1016/j.coastaleng.2007.01.007
Davies, B. R., Biggs, J., Williams, P. J., Lee, J. T., and Thompson, S. (2008). A comparison of the catchment sizes of rivers, streams, ponds, ditches and lakes: implications for protecting aquatic biodiversity in an agricultural landscape. Hydrobiologia 597, 7–17. doi: 10.1007/s10750-007-9227-6
Defeo, A., McLachlan, A., Schoeman, D. S., Schlacher, T. A., Dugan, J., Jones, A., et al. (2009). Threats to sandy beach ecosystems: a review. Estuar. Coast. Shelf Sci. 81, 1–12. doi: 10.1016/j.ecss.2008.09.022
Dickinson, J. L., Shirk, J., Bonter, D., Bonney, R., Crain, R. L., Martin, J., et al. (2012). The current state of citizen science as a tool for ecological research and public engagement. Front. Ecol. Environ. 10, 291–297. doi: 10.1890/110236
Dudgeon, D., Arthington, A. H., Gessner, M. O., Kawabata, Z. I., Knowler, D. J., Lévêque, C., et al. (2006). Freshwater biodiversity: importance, threats, status and conservation challenges. Biol. Rev. 81, 163–182. doi: 10.1017/S1464793105006950
Fedak, M. (2004). Marine animals as platforms for oceanographic sampling: a “win/win” situation for biology and operational oceanography. Memo. Natl. Inst. Polar Res. 58, 133–147.
Ferreira, J. G., Vale, C., Soares, C. V., Salas, F., Stacey, P. E., Bricker, S. B., et al. (2007). Monitoring of coastal and transitional waters under the E.U. Water Framework Directive. Environ. Monitor. Asses. 135, 195–216. doi: 10.1007/s10661-007-9643-0
Field, C. B., Behrenfeld, M. J., Randerson, J. T., and Falkowski, P. G. (1998). Primary production of the biosphere: integrating terrestrial and oceanic components. Science 281, 237–240. doi: 10.1126/science.281.5374.237
Friedrichs, A., Busch, J. A., van der Woerd, H. J., and Zielinski, O. (2017). SmartFluo: a method and affordable adapter to measure chlorophyll a fluorescence with smartphones. Sensors 17:678. doi: 10.3390/s17040678
Garcia-Soto, C., van der Meeren, G. I., Busch, J. A., Delany, J., Domegan, C., Dubsky, K., et al. (2017). Advancing Citizen Science for Coastal and Ocean Research. Technical report, French, V., Kellett, P., Delany, J., McDonough, N. [Eds.] Position Paper 23 of the European Marine Board, Ostend, Belgium.
Gelcich, S., Buckley, P., Pinnegar, J., Chilvers, J., Lorenzoni, I., Terry, G., et al. (2014). Public awareness, concerns, and priorities about anthropogenic impacts on marine environments. Proc. Natl. Acad. Sci. U.S.A. 111, 15042–15047. doi: 10.1073/pnas.1417344111
Goetze, J. S., Jupiter, S. D., Langlois, T. J., Wilson, S. K., Harvey, E. S., Bond, T., et al. (2015). Diver operated video most accurately detects the impacts of fishing within periodically harvested closures. J. Exp. Mar. Biol. Ecol. 462, 74–82. doi: 10.1016/j.jembe.2014.10.004
Halpern, B., Walbridge, S., Selkoe, K. A., Kappel, C., Micheli, F., D'agrosa, C., et al. (2008). A global map of human impact on marine ecosystems. Science 319, 948–952. doi: 10.1126/science.1149345
Huang, G., and Xia, J. (2001). Barriers to sustainable water-quality management. J. Environ. Manag. 61, 1–23. doi: 10.1006/jema.2000.0394
Hut, R., Tyler, S., and van Emmerik, T. (2016). Proof of concept: temperature-sensing waders for environmental sciences. Geosci. Instrum. Method. Data Syst. 5, 45–51. doi: 10.5194/gi-5-45-2016
Hyder, K., Townhill, B., Anderson, L. G., Delany, J., and Pinnegar, J. K. (2015). Can citizen science contribute to the evidence-base that underpins marine policy? Mar. Policy 59, 112–120. doi: 10.1016/j.marpol.2015.04.022
Hyder, K., Wright, S., Kirby, M., and Brant, J. (2017). The role of citizen science in monitoring small-scale pollution events. Mar. Pollut. Bull. 120, 51–57. doi: 10.1016/j.marpolbul.2017.04.038
Islam, M., and Tanaka, M. (2004). Impacts of pollution on coastal and marine ecosystems including coastal and marine fisheries and approach for management: a review and synthesis. Mar. Pollut. Bull. 48, 624–649. doi: 10.1016/j.marpolbul.2003.12.004
Kelly, R., Foley, M., Fisher, W., Feely, R., Halpern, B., Waldbusser, G., et al. (2011). Mitigating local causes of ocean acidification with existing laws. Science 332, 1036–1037. doi: 10.1126/science.1203815
Kintisch, E. (2015). A moment of truth arrives for U.S. ocean science. Science 347:463. doi: 10.1126/science.347.6221.463
Lafleur, J. P., Rackov, A. A., Mc Auley, S., and Salin, E. D. (2010). Miniaturised centrifugal solid phase extraction platforms for in-field sampling, pre-concentration and spectrometric detection of organic pollutants in aqueous samples. Talanta 81, 722–726. doi: 10.1016/j.talanta.2009.12.001
Larsen, M., Borisov, S. M., Grunwald, B., Klimant, I., and Glud, R. N. (2011). A simple and inexpensive high resolution color ratiometric planar optode imaging approach: application to oxygen and pH sensing. Limnol. Oceanogr. Methods 9, 348–360. doi: 10.4319/lom.2011.9.348
Lauro, F. M., Senstius, S. J., Cullen, J., Neches, R., Jensen, R. M., Brown, M. V., et al. (2014). The Common oceanographer: crowdsourcing the collection of oceanographic data. PLoS Biol. 12:e1001947. doi: 10.1371/journal.pbio.1001947
Le Quéré, C., Moriarty, R., Andrew, R. M., Canadell, J. G., Sitch, S., Korsbakken, J. I., et al. (2015). Global carbon budget 2015. Earth Syst. Sci. Data 7, 349–396. doi: 10.5194/essd-7-349-2015
Leeuw, T., Boss, E. S., and Wright, D. L. (2013). In situ measurements of phytoplankton fluorescence using low cost electronics. Sensors 13, 7872–7883. doi: 10.3390/s130607872
Leeworthy, V. R., and Wiley, P. C. (2001). Current Participation Patterns in Marine Recreation, National Survey on Recreation and the Environment (NSRE) 2000. Technical report, National Oceanic and Atmospheric Administration, National Ocean Service, Special Projects Office, Silver Springs, MD.
Livingston, R. J. (2014). Climate Change and Coastal Ecosystems: Long-Term effects of Climate and Nutrient Loading on Trophic Organization. Boca Raton, FL: CRC Press.
Lorenzoni, I., Nicholson-Cole, S., and Whitmarsh, L. (2007). Barriers perceived to engaging with climate change among the UK public and their policy implications. Global Environ. Change 17, 445–459. doi: 10.1016/j.gloenvcha.2007.01.004
McClain, C. R. (2009). A decade of satellite ocean color observations. Annu. Rev. Mar. Sci. 1, 19–42. doi: 10.1146/annurev.marine.010908.163650
Merchant, C. J. (2013). “Thermal remote sensing of sea surface temperature,” in Thermal Infrared Remote Sensing, eds C. Kuenzer and S. Dech (Dordrecht: Springer), 287–313. doi: 10.1007/978-94-007-6639-6_15
Mohammed, J. S. (2016). Applications of 3D printing technologies in oceanography. Methods Oceanogr. 17, 97–117. doi: 10.1016/j.mio.2016.08.001
Müller, R. D., Qin, X., Sandwell, D. T., Dutkiewicz, A., Williams, S. E., Flament, N., et al. (2016). The GPlates portal: cloud-based interactive 3D visualization of global geophysical and geological data in a web browser. PLoS ONE 11:e0150883. doi: 10.1371/journal.pone.0150883
NASA (2014). Data from: NASA Goddard Space Flight Center, Ocean Ecology Laboratory, Ocean Biology Processing Group. Moderate-resolution Imaging Spectroradiometer (MODIS) Aqua Ocean Color Data; 2014 Reprocessing. NASA OB.DAAC. Greenbelt, MD. doi: 10.5067/AQUA/MODIS_OC.2014.0
Nelms, S., Coombes, C., Foster, L., Galloway, T., Godley, B., Lindeque, P., et al. (2017). Marine anthropogenic litter on British beaches: a 10-year nationwide assessment using citizen science data. Sci. Total Environ. 579, 1399–1409. doi: 10.1016/j.scitotenv.2016.11.137
Papathanasopoulou, E., White, M. P., Hattam, C., Lannin, A., Harvey, A., and Spencer, A. (2016). Valuing the health benefits of physical activities in the marine environment and their importance for marine spatial planning. Mar. Policy 63, 144–152. doi: 10.1016/j.marpol.2015.10.009
Phillips, J. C., McKinley, G. A., Bennington, V., Bootsma, H. A., Pilcher, D. J., Sterner, R. W., et al. (2015). The potential for CO2-induced acidification in freshwater: a great lakes case study. Oceanography 28, 136–145. doi: 10.5670/oceanog.2015.37
Platt, T., and Sathyendranath, S. (2008). Ecological indicators for the pelagic zone of the ocean from remote sensing. Remote Sens. Environ. 112, 3426–3436. doi: 10.1016/j.rse.2007.10.016
Postel, S., and Carpenter, S. (1997). Nature's Services: Societal Dependence on Natural Ecosystems. Washington, DC: Island Press.
Rabalais, N. N., Cai, W. J., Carstensen, J., Conley, D. J., Fry, B., Hu, X., et al. (2014). Eutrophication-driven deoxygenation in the coastal ocean. Oceanography 27, 172–183. doi: 10.5670/oceanog.2014.21
Raymond, P. A., Hartmann, J., Lauerwald, R., Sobek, S., McDonald, C., Hoover, M., et al. (2013). Global carbon dioxide emissions from inland waters. Nature 503, 355–359. doi: 10.1038/nature12760
Regnier, P., Friedlingstein, P., Ciais, P., Mackenzie, F. T., Gruber, N., Janssens, I. A., et al. (2013). Anthropogenic perturbation of the carbon fluxes from land to ocean. Nat. Geosci. 6, 597–607. doi: 10.1038/ngeo1830
Riesch, H., and Potter, C. (2014). Citizen science as seen by scientists: Methodological, epistemological and ethical dimensions. Public Understand. Sci. 23, 107–120. doi: 10.1177/0963662513497324
Riser, S., Freeland, H., Roemmich, D., Wijffels, S., Troisi, A., Belbèoch, M., et al. (2016). Fifteen years of ocean observations with the global Argo array. Nat. Clim. Change 6, 145–153. doi: 10.1038/nclimate2872
Rudnick, D., Davis, R. E., Eriksen, C. C., Fratantoni, D. M., and Perry, M. J. (2004). Underwater gliders for ocean research. Mar. Technol. Soc. J. 38, 73–84. doi: 10.4031/002533204787522703
Scales, K. L., Hazen, E. L., Jacox, M. G., Edwards, C. A., Boustany, A. M., Oliver, M. J., et al. (2017). Scale of inference: on the sensitivity of habitat models for wide-ranging marine predators to the resolution of environmental data. Ecography 40, 210–220. doi: 10.1111/ecog.02272
Schläppy, M.-L., Loder, J., Salmond, J., Lea, A., Dean, A. J., and Roelfsema, C. M. (2017). Making waves: marine citizen science for impact. Front. Mar. Sci. 4:146. doi: 10.3389/fmars.2017.00146
Schwartz, M. D., Betancourt, J. L., and Weltzin, J. F. (2012). From Caprio's lilacs to the USA National Phenology Network. Front. Ecol. Environ. 20, 324–327. doi: 10.1890/110281
Scyphers, S. B., Powers, S. P., Akins, J. L., Drymon, J. M., Martin, C. W., Schobernd, Z. H., et al. (2015). The role of citizens in detecting and responding to a rapid marine invasion. Conserv. Lett. 8, 242–250. doi: 10.1111/conl.12127
Shively, D. A., Nevers, M. B., Breitenbach, C., Phanikumar, M. S., Przybyla-Kelly, K., Spoljaric, A. M., et al. (2016). Prototypic automated continuous recreational water quality monitoring of nine Chicago beaches. J. Environ. Manag. 166, 285–293. doi: 10.1016/j.jenvman.2015.10.011
Smith, K. L. Jr., Messié, M., Sherman, A. D., Huffard, C. L., Hobson, B. W., Ruhl, H. A., et al. (2015). Navigating the uncertain future of global oceanic time series. Eos Trans. Am. Geophys. Union. doi: 10.1029/2015EO038095
Thiel, M., Penna-Dòaz, M. P., Luna-Jorquera, G., Salas, S., Sellanes, J., and Stotz, W. (2014). Citizen scientists and marine research: volunteer participants, their contributions, and projection for the future. Oceanogr. Mar. Biol. Annu. Rev. 52, 257–314. doi: 10.1201/b17143-6
Tittensor, D. P., Mora, C., Jetz, W., Lotze, H. K., Ricard, D., Berghe, E. V., et al. (2010). Global patterns and predictors of marine biodiversity across taxa. Nature 466, 1098–1103. doi: 10.1038/nature09329
Trenberth, K. E., and Fasullo, J. T. (2013). An apparent hiatus in global warming? Earth's Fut. 1, 19–32. doi: 10.1002/2013EF000165
Trumbull, D. J., Bonney, R., Bascom, D., and Carbral, A. (2000). Thinking scientifically during participation in a citizen-science project. Sci. Educ. 84, 265–275. doi: 10.1002/(SICI)1098-237X(200003)84:2<265::AID-SCE7>3.0.CO;2-5
van den Belt, M. (2011). “Ecological economics of estuaries and coasts,” in Treatise on Estuarine and Coastal Science, eds E. Wolanski and D. McLusky (London: Elsevier), 482.
Ward-Paige, C., and Lotze, H. (2011). Assessing the value of recreational divers for censusing elasmobranchs. PLoS ONE 6:e25609. doi: 10.1371/journal.pone.0025609
Ward-Paige, C., Pattengill-Semmens, C., Myers, R., and Lotze, H. (2011). Spatial and temporal trends in yellow stingray abundance: evidence from diver surveys. Environ. Biol. Fish. 90, 263–276. doi: 10.1007/s10641-010-9739-1
Watson, C. S., White, N. J., Church, J. A., King, M. A., Burgette, R. J., and Legresy, B. (2015). Unabated global mean sea-level rise over the satellite altimeter era. Nat. Clim. Change 5, 565–568. doi: 10.1038/nclimate2635
Wiggins, A., and Crowston, K. (2011). “From conservation to crowdsourcing: a typology of citizen science,” in Proceedings of the 2011 44th Hawaii International Conference on System, (Washington, DC: IEEE Computer Society).
Williams, K., De Robertis, A., Berkowitz, Z., Rooper, C., and Towler, R. (2014). An underwater stereo-camera trap. Methods Oceanogr. 11, 1–12. doi: 10.1016/j.mio.2015.01.003
Williamson, C. E., Dodds, W., Kratz, T. K., and Palmer, M. A. (2008). Lakes and streams as sentinels of environmental change in terrestrial and atmospheric processes. Front. Ecol. Environ. 6, 247–254. doi: 10.1890/070140
Wright, S., Hull, T., Sivyer, D. B., Pearce, D., Pinnegar, J. K., Sayer, M. D. J., et al. (2016). SCUBA divers as oceanographic samplers: the potential of dive computers to augment aquatic temperature monitoring. Sci. Rep. 6, 1–8. doi: 10.1038/srep30164
Keywords: aquatic, observations, sampling, recreation, citizen science
Citation: Brewin RJW, Hyder K, Andersson AJ, Billson O, Bresnahan PJ, Brewin TG, Cyronak T, Dall'Olmo G, de Mora L, Graham G, Jackson T and Raitsos DE (2017) Expanding Aquatic Observations through Recreation. Front. Mar. Sci. 4:351. doi: 10.3389/fmars.2017.00351
Received: 01 September 2017; Accepted: 18 October 2017;
Published: 02 November 2017.
Edited by:
Isabel Iglesias, Centro Interdisciplinar de Pesquisa Marine e Ambiental (CIIMAR), PortugalReviewed by:
Lígia Pinto, Universidade de Lisboa, PortugalSabine Schmidt, Centre National de la Recherche Scientifique (CNRS), France
Copyright © 2017 Brewin, Hyder, Andersson, Billson, Bresnahan, Brewin, Cyronak, Dall'Olmo, de Mora, Graham, Jackson and Raitsos. This is an open-access article distributed under the terms of the Creative Commons Attribution License (CC BY). The use, distribution or reproduction in other forums is permitted, provided the original author(s) or licensor are credited and that the original publication in this journal is cited, in accordance with accepted academic practice. No use, distribution or reproduction is permitted which does not comply with these terms.
*Correspondence: Robert J. W. Brewin, cm9ickBwbWwuYWMudWs=