- 1Hellenic Centre for Marine Research, Institute of Oceanography, Anavyssos, Greece
- 2Department of Environmental Sciences, Institute of Marine and Coastal Sciences, Rutgers University, New Brunswick, NJ, United States
- 3Laboratory of Marine Geology and Physical Oceanography, Department of Geology, University of Patras, Patras, Greece
A circulation model is coupled to a Lagrangian particle-tracking model to simulate the transport floating litter particles in the Aegean Sea, Greece (Eastern Mediterranean). Considering different source regions and release dates, simulations were carried out to explore the fate and distribution of floating litter over 1990–2009, taking into account the seasonal and interannual variability of surface circulation. Model results depicted recurrently high concentrations of floating litter particles in the North Aegean plateau, the Saronikos Gulf, and along Evia and Crete islands. Modeled transport pathways of floating litter demonstrated that source regions are interconnected, with Saronikos Gulf being a main receptor of litter from other sources. Notably higher percent of litter exit (~35%) than enter the model domain (~7%) signified that Aegean Sea seems to act as a source rather than receptor of floating litter pollution in the Eastern Mediterranean Sea. Beached litter was found around 10%, mostly located in the western part of the Aegean Sea. This is the first modeling study to explore the transport of floating marine litter in Greek waters.
Introduction
Marine litter is a documented threat for marine and human life, being present in vast quantities in the marine and coastal environments (Barnes et al., 2009). Recent studies have estimated ~5 trillion particles to be present into the world's oceans (Eriksen et al., 2014). Plastics typically enter the ocean from land- and marine-based sources, are carried via oceanic currents and dragged by winds, and finally accumulate in open sea and coastal regions (Galgani, 2015; Sebille et al., 2015; UNEP/MAP, 2016), after covering long and complex pathways (Maximenko et al., 2012; Ryan, 2015). Once deposited into the oceans, plastics are gradually fragmented into smaller particles (<5 mm), due to the synergistic effect of environmental variables and the inherent material instability, and this forms floating marine debris (Thompson et al., 2004; Pastorelli et al., 2014). Floating litter items are found in oceans around the world, such as in the Pacific ocean (Martinez et al., 2009; Law et al., 2014), the southeast Atlantic gyre (Ryan, 2014), the North Atlantic sub-tropical gyre (Reisser et al., 2015), and the Mediterranean Sea (Suaria and Aliani, 2014; Cózar et al., 2015).
General circulation models linked to particle-tracking models are widely used to track the transport of different kinds of passive drifters in the marine environment, such as oil spill pollutants, fish eggs and larvae, marine debris, and buoys (Pollani et al., 2001; Lynch et al., 2014; Mansui et al., 2015). Marine litter modeling is a growing field aiming at understanding of litter sources, fate, transport and accumulation in oceans (NOAA, 2016). Neumann et al. (2014) performed transport simulations, forward and backward in time, to identify accumulation and potential source regions of marine litter in the southern North Sea. Similarly, the drift and beaching of floating marine litter was examined numerically in the Sea of Japan (Yoon et al., 2010). Considering input of marine litter into the North Pacific Ocean by the 2011 Tohoku tsunami, Lebreton and Borrero (2013) suggested that tsunami debris would eventually accumulate to the North Pacific Ocean subtropical gyre. Finally, the study of Kubota (1994) simulated the trajectories of 50 virtual marine debris in the northern Hawaiian Islands and provided potential mechanisms of their accumulation, related to winds, geostrophic currents and Ekman drift.
Available Greek field data indicate that litter come from the Ionian Sea, Patraikos Gulf (Stefatos et al., 1999; Koutsodendris et al., 2008) and the Aegean Sea (Katsanevakis and Katsarou, 2004; Ioakeimidis et al., 2014, 2015; Papadopoulou et al., 2015). These studies have focused on detecting big marine litter items on the seafloor. In contrast, available data on floating litter distribution are scarce, and the ecological problem of floating litter transport and accumulation is largely unknown. Recently, Mansui et al. (2015) proposed specific gyres and regions that could retain and export floating items in the Mediterranean Sea. However, their model set up did not allow to properly resolve the litter dynamics in the Aegean Sea due to its complex coastline which includes hundreds of islands.
Extensive field studies have documented high concentrations of floating plastic pollution in the Mediterranean Sea (Cózar et al., 2015; Pedrotti et al., 2016). Concurrently, modeling studies have identified potential sites of floating litter accumulation in open sea and coastal areas in the Mediterranean Sea (Lebreton et al., 2012; Mansui et al., 2015; Liubartseva et al., 2016). In this study, we link a circulation model with a particle-tracking model to simulate the transport of floating litter particles in the Aegean Sea, Greece (Eastern Mediterranean). Source regions of litter were related to big cities, rivers, the inflow of Black Sea Waters through the Dardanelles strait, and open sea. Different release dates, on monthly and annual scales, were considered to explore the seasonal and interannual variability of floating litter drift over 1990–2009. The main objectives of the study are to: (1) investigate the fate and distribution of floating litter particles after being released from source regions, (2) explore whether and to what extent the Aegean Sea may act as a source or receptor of floating litter pollution in the Mediterranean Sea, and (3) depict the litter distribution in shoreline areas. This is the first modeling study discussing the transport of floating litter particles in the Greek waters.
Materials and Methods
Study Area: The Aegean Sea
The Aegean Sea is located northwest of the Eastern Mediterranean basin, providing a strong hydrodynamic connection between the Eastern Mediterranean and the Black Sea through the Dardanelles Strait (Figure 1). The Aegean Sea exhibits a complicated physiography in terms of seafloor morphology and island configuration. It has an irregular and extended coastline and hundreds of islands scattered all over the region (Theocharis et al., 1993). The Aegean Sea can be divided in three broad areas: (a) the North Aegean Sea which consists of North Aegean trough, the Skyros basin and three main shelf areas: Thermaikos, Samothraki and Limnos; (b) the Central Aegean Sea which is characterized by the Chios basin; and (c) South Aegean Sea which shows a complex physiography consisting of the Myrtoan and Cretan basins, and the Cyclades plateau (Figure 1).
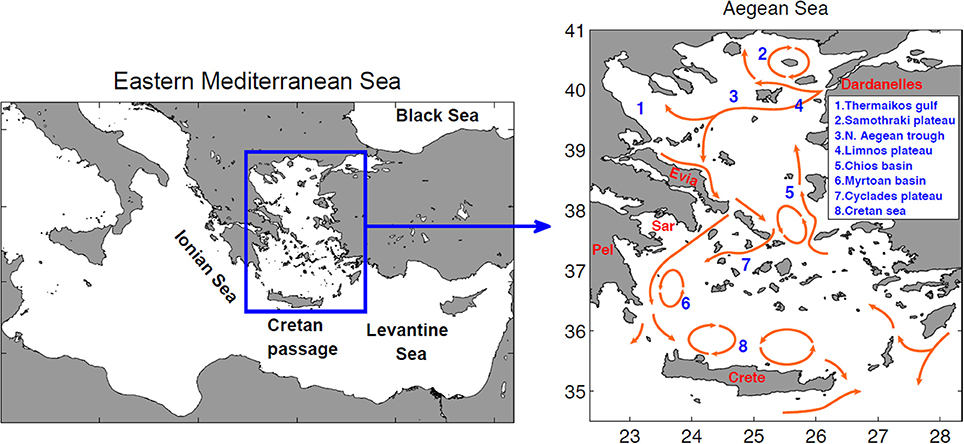
Figure 1. Map of the Aegean Sea. Numbers 1 to 8 denote major basins, gulfs and plateaus, and abbreviations denote place names: Peloponnese (Pel), Saronikos Gulf (Sar), Evia island, Dardanelles strait and Crete island. Schematic representation of the general Aegean Sea upper circulation is also shown (Map modified after Nittis and Perivoliotis, 2002; Nittis et al., 2003; Olson et al., 2007).
The general surface circulation of the Aegean Sea is characterized by complex patterns consisting of basin scale cyclonic flows, sub-basin scale recurrent gyres, transient eddies, and other mesoscale features. The inflow of low-salinity waters from the Black Sea (BSW), through the Dardanelles straits, is considered to be the most significant buoyant input into the Aegean Sea. The BSW subsequently flow westwards with bifurcations to the north toward the north Aegean plateau and to the southwest reaching the south Aegean Sea (Nittis and Perivoliotis, 2002). The northern part of the Aegean Sea is characterized by an overall cyclonic circulation with a semi-permanent large anticyclone, being present in the northeastern part which is significantly influenced by the inflow of low-salinity BSW (Olson et al., 2007). Other important mesoscale features are the cyclonic eddies in the Chios basin, the boundary current along the eastern coast of the Evia island, the Myrtoan Cyclone and the East Cretan Cyclone. The southern Aegean Sea circulation is characterized by two cyclonic gyres, two anticyclonic eddies and other smaller scale structures, interconnected by currents and jets, which are variable in space and time (Theocharis et al., 1999). Figure 1 provides a schematic representation of the main features of the general surface circulation in the Aegean Sea.
Models
Circulation Model
A three-dimensional hydrodynamic model, based on the Princeton Ocean Model (POM, Blumberg and Mellor, 1983), was used to obtain the surface water circulation in the Aegean Sea. POM is a widely used community model (http://www.ccpo.odu.edu/POMWEB/) with numerous applications both for ocean and regional modeling studies. POM has been implemented in the Mediterranean Sea at basin/sub-basin scale (Zavatarelli and Mellor, 1995; Horton et al., 1997; Korres and Lascaratos, 2003, among others) and also on regional studies, including the Aegean Sea (Korres et al., 2010; Androulidakis et al., 2012; among others). It is a primitive equation, sigma coordinate and free surface elevation model that employes a 2.5 turbulence closure scheme (Mellor and Yamada, 1982) for vertical mixing.
The hydrodynamic model domain extends from the East (19.5°–30°) to the North (30°–41°), with a horizontal resolution of 1/15° (~7.5 Km), 25 sigma layers in the vertical, a logarithmic distribution near the surface and bottom, and a time step of 12 min for the external and internal modes. The hydrodynamic properties along the model's open boundaries (eastern/western) are obtained from a basin scale Mediterranean hydrodynamic model (Korres et al., 2007) simulation over the same period. The Dardanelles water exchange (surface inflow of BSW, subsurface outflow of Aegean water) is parameterized through a two-layer open boundary condition (Nittis et al., 2003), with prescribed water inflow/outflow and salinity adopting seasonal climatological data (Tugrul et al., 2002). The atmospheric forcing was obtained from HIRHAM5 climate model hindcast simulation over a 20-year (1990–2009) period (Christensen et al., 2006), downscaling ERA-interim dataset to 1/10° resolution. The heat and freshwater fluxes, at the air-sea interface, are calculated using hourly fields of wind velocity (10 m), relative humidity (2 m), air temperature (2 m), precipitation, net incoming short wave radiation and incoming long wave radiation, using properly tuned bulk formulae set (Korres and Lascaratos, 2003). The circulation model implemented in this study has been validated through various applications with finer (e.g., Kourafalou et al., 2006; Korres et al., 2010) or coarser horizontal resolution (Korres et al., 2007; Tsiaras et al., 2014). The same model is currently operational with a slightly higher resolution (1/30°) as part of the POSEIDON forecasting system (www.poseidon.hcmr.gr).
Particle-Tracking Model
Simulations were conducted using the Lagrangian model described in Pollani et al. (2001). In this study, floating litter particles were treated as passive drifters, exclusively driven by surface circulation and diffusion. We did not include other particle properties (e.g., oil evaporation, vertical dispersion and emulsification, wave drift) used in Pollani et al. (2001) for oil spill prediction. Thus, the horizontal advection of a particle is defined by the surface current velocities provided by the POM. The position of a particle is updated in two dimensions, as follows:
where (x, y) define the two-dimension position of the particle, dt is the time step, (u, v) are the surface current velocities in x, y dimensions, Kh is the horizontal diffusion coefficients, which is dynamically computed in the hydrodynamic model, and (Rx, Ry) are random numbers distributed between −1 and +1.
Simulations
Marine litter usually enters the ocean from land-based (e.g., riverine inputs, large cities, ports) and sea-based sources (e.g., straits, shipping, fisheries) (Yoon et al., 2010; Lebreton et al., 2012; Galgani, 2015; Liubartseva et al., 2016; UNEP/MAP, 2016). In this study, five regions in the Aegean Sea (Table 1, letters: A, B, C, D, E; Figure 2) have been selected as the major sources to initiate the simulations around which particles were uniformly distributed. Two additional marine litter sources outside the Aegean Sea (Figure 2 and Table 1, letters: F and G) were also considered to study the hypothesis if Eastern Mediterranean Sea could act as a source of floating litter for the Aegean Sea.
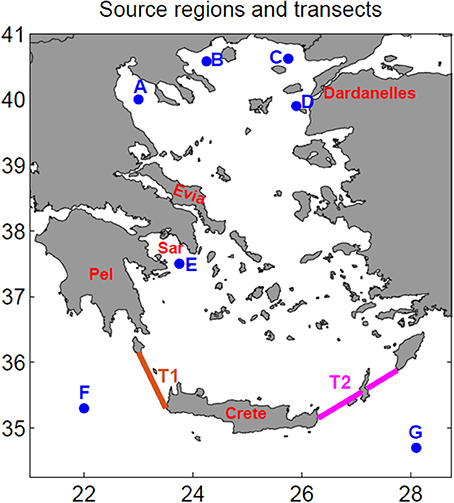
Figure 2. Letters A,B,C,D,E,F, and G denote considered source regions of floating litter. Two transects, marked as T1 (orange) and T2 (magenta) lines, are used to compute the floating litter exchange between the Aegean Sea and the Eastern Mediterranean Sea.
To investigate the fate and distribution of floating litter particles, we conducted three experiments (Table 1). All experiments were run for 1 year. We then repeated the annual simulations for multiple years (from 1990 to 2009) to take into account the inter-annual variability of surface circulation in our analysis. The particle-tracking model was on-line coupled with the circulation model and resolved with a 12 min time step; outputs were saved and plotted daily, and a total number of 9,000 particles was considered sufficient to obtain a statistically valid representation of the results, while maintaining a reasonable computational cost. The setup of the three experiments is as follows:
Experiment A
Particles are released from the sources A, B, C, D, and E (Figure 2), assuming four different release dates; i.e., January 1st (Case A1), April 1st (Case A2), July 1st (Case A3), and October 1st (Case A4) (Table 1). The goal is to track the fate and distribution of floating litter particles, after being released from the source regions. Different release dates aimed to explore the role of the surface circulation seasonal variability on particle drift. The main interest of this experiment is to explore the contribution of source regions to the final concentration of particles in specific areas.
Experiment B
Particles are released from sources A, B, C, D, and E (Figure 2), on the first of every month and tracked for 1 year. The objective is to identify areas in which particles tend to concentrate, considering a more realistic scenario of periodic inputs of litter in the model domain.
Experiment C
Particles are released from sources F and G (Figure 2) on the first of every month along and tracked for 1 year. The objective is to test to what extent the Aegean Sea may act as a receptor of floating litter particles originated outside the Aegean Sea.
Stranding of particles is not included in our simulations, so when particles were found on land cells due to random movement, they were bounced back to the sea, still considered to be part of the computational process. A particle is marked as “beached” if it drifted in cells adjacent to land, following Lebreton et al. (2012). However, beached particles may still drift at any point of the simulation process, during which offshore surface currents occur. The number of beached litter particles was calculated to assess the distribution of floating litter along shoreline areas.
Outputs
Average and standard deviation maps of particle distribution were produced over 1990–2009 period, on a grid of 30 × 90 cells. Then, these maps were combined to classify the distribution of particles into three categories: recurrent, occasional and rare. To accomplish this, each cell was assigned to a category following the classification approach presented in Saraux et al. (2014). This classification has been previously applied on multi-annual fish egg distribution data to characterize favorable spawning habitats (Bellier et al., 2007; Giannoulaki et al., 2013; Saraux et al., 2014). The approach assumes that cells with high mean and low standard deviation are considered as recurrent, signifying high concentration of litter particles for most of the years. Cells with high standard deviation are considered as occasional, implying that litter particles are not necessarily found every year. Cells with low mean and medium standard deviation are defined as rare, implying that litter particles are rarely found in these areas.
The limiting thresholds, which define low and high values for the average and standard deviation maps, were calculated using K-means clustering algorithm (Jain, 2010). K-means provides partitions of a given data set into a number of clusters, and provides cluster indices for each data point. K-means is applied on the average and standard deviation maps, assuming three clusters. This results in cut-off points which define intervals of low, medium and high values, based on the number of particles found in the cells for the average map, and the values of standard deviation for the standard deviation map. The calculations were performed with k-means clustering function provided from Matlab R2012 (www.Mathworks.com). The code is available upon request. The threshold values that define the classification areas are listed in Table A.1 (Appendix A).
Two transects, marked as T1 (orange) and T2 (green) lines (Figure 2), were used to calculate the percentage of particles daily exiting the Aegean Sea from the western and eastern part, respectively; these particles were considered as “escaped.” The variability in percentage of escaped particles from repeated annual simulation over 1990–2009 was represented with boxplots. In addition, particles found below 33°N, western of 19°E, or eastern of 30°E were considered as “lost”; the movement of these particles was detained during the analysis of the results.
Results
Simulated Circulation in the Aegean Sea
Seasonal averages of the simulated near surface circulation, were calculated over the 1990–2009 period (Figure 3). As a validation exercise, simulated drifter tracks with the present model were compared (Figure 4) against available observed drifters in the North Aegean during 2002 (Olson et al., 2007), adequately reproducing known circulation features, discussed below. The overall cyclonic circulation simulated in the Aegean Sea is in agreement with previous observations (Theocharis and Georgopoulos, 1993; Olson et al., 2007). This is related to the northward flow of more saline water from the Levantine sea, carried out by a branch of the Asia Minor current along the eastern side of the Aegean and particularly to the pathway of low-salinity BSW (Figures 3B–D). Simulated current fields depict that BSW, initially spreads westwards branching around Limnos Island and then either follows a pathway to the west toward Evia island and Thermaikos Gulf (Figures 3B–D, 4C,D) or to the North East, forming the semi-permanent Samothraki anticyclone from July to October (Figures 3C,D, 4B). This pathway agrees with observations obtained by Olson et al. (2007) and Zervakis and Georgopoulos (2002). In autumn, and particularly during the summer period, the BSW moves mostly south of Limnos Island in accordance with Zervakis and Georgopoulos (2002), due to strong northerly Etesian winds creating a larger anticyclone covering the entire North East area. The westward BSW branch flows either toward the Thermaikos Gulf or directly to the west, in both cases resulting in the formation of a southward coastal current along Evia (Figures 3A,B,D, 4A) consistent with Olson et al. (2007). In January and October (Figures 3A,D), this Evia jet appears stronger in the model simulation, passing toward the Southern Aegean Sea mainly through the strait south of Evia Island, while in spring and summer (Figures 3B,C), there is a more diffuse flow from Cyclades plateau, contributed also by redirected Levantine water from the Eastern Aegean.
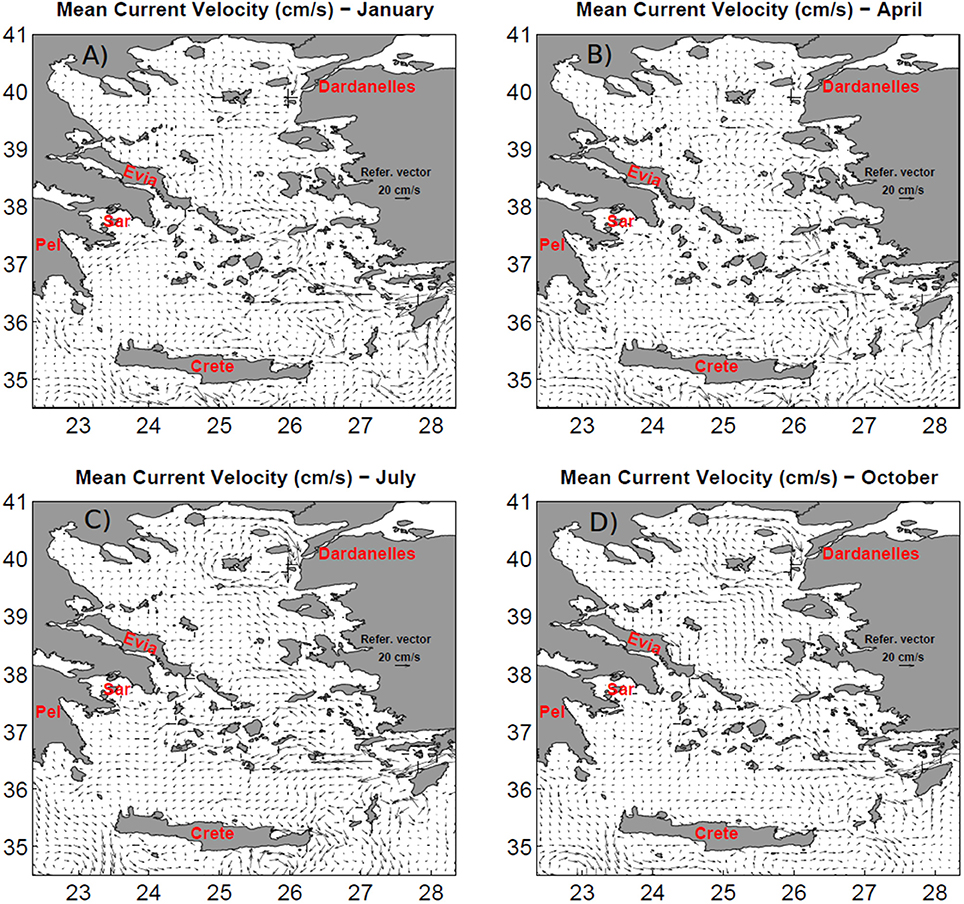
Figure 3. Mean current field every fourth grid point averaged from 1990 to 2009 over (A) January, (B) April, (C) July, and (D) October.
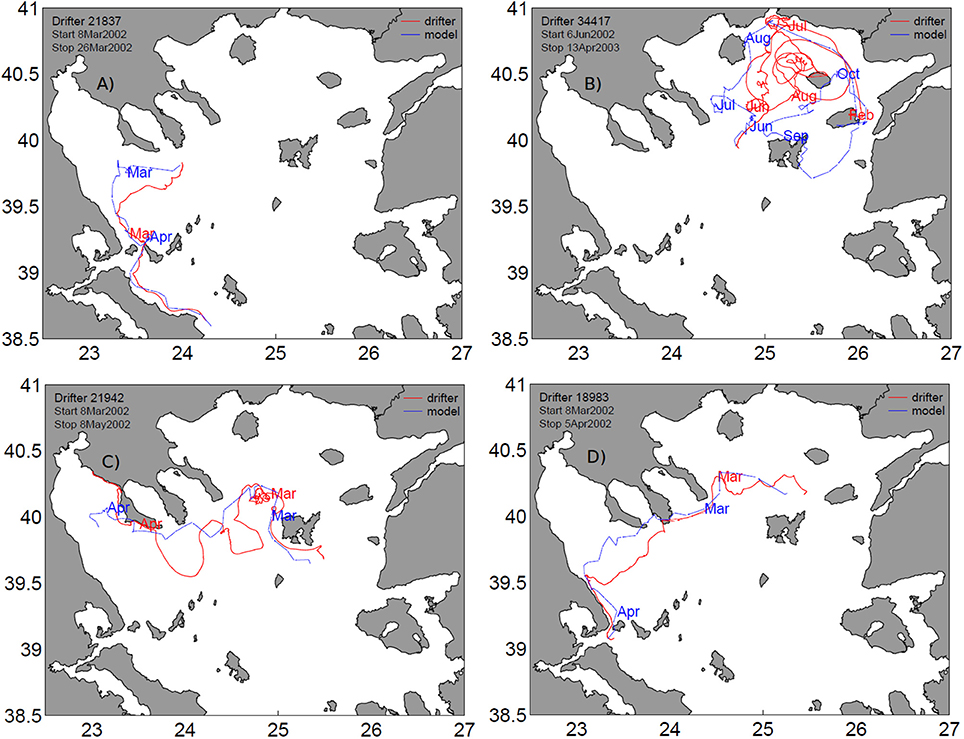
Figure 4. Simulated drifter tracks (blue line) against observed drifter tracks (red line) in 2002 (Olson et al., 2007). Fifty model drifters are released on the same time period and within a small radius from the actual release location to remove sensitivity from initial release. The best model drifters in reproducing the observed patterns are shown: (A) drifter 21837, (B) drifter 34417, (C) drifter 21942, and (D) drifter 18983. The month is indicated on its 15th day along the simulated (blue color) and observed (red color) drifter tracks.
Other known circulation features reproduced by the model are the permanent cyclonic gyre in Chios basin (Nittis and Perivoliotis, 2002; Olson et al., 2007), the Skyros cyclonic eddy (Olson et al., 2007) and the cyclonic circulation in Sporades basin (Kontoyiannis et al., 2003). The later has been found to be occasionally anticyclonic (Olson et al., 2007), as simulated during July (Figure 3C). The Myrtoan cyclone (Nittis and Perivoliotis, 2002) is mainly reproduced during winter, while it has been occasionally observed to turn into anticyclonic during spring and autumn (Olson et al., 2007; Sayin et al., 2011). The known cyclonic circulation in the Cretan Sea (Nittis and Perivoliotis, 2002) is also partly reproduced, along the dominant eastward current on the coast of Crete island (Figure 3).
Distribution of Floating Litter Particles
The combination of average and standard deviation maps of particle distributions over 1990–2009, resulted in classification maps associated with recurrent, occasional and rare presence of floating litter particles (Figure 5). For Cases A1-A4, high concentration of litter particles was found in areas closely related to the southward pathway of waters from the North Aegean Sea, where the most important sources of floating litter particles were located. These areas include the North Eastern Aegean Sea, receiving inputs from Evros/Strymonikos Rivers and the Dardanelles strait, with the semi-permanent anticyclonic circulation in Samothraki and Limnos plateau resulting in the relatively higher residence time of litter particles. Other areas where litter were found to accumulate are the Sporades basin and the eastern coast of Evia, being in the pathway of waters coming from North Eastern Aegean Sea, including BSW, and also receiving inputs from the Thermaikos Gulf. Further south, increased litter particle accumulation was found in the Saronikos Gulf and the Myrtoan basin, and occasionally in the Cyclades plateau and the Cretan Sea.
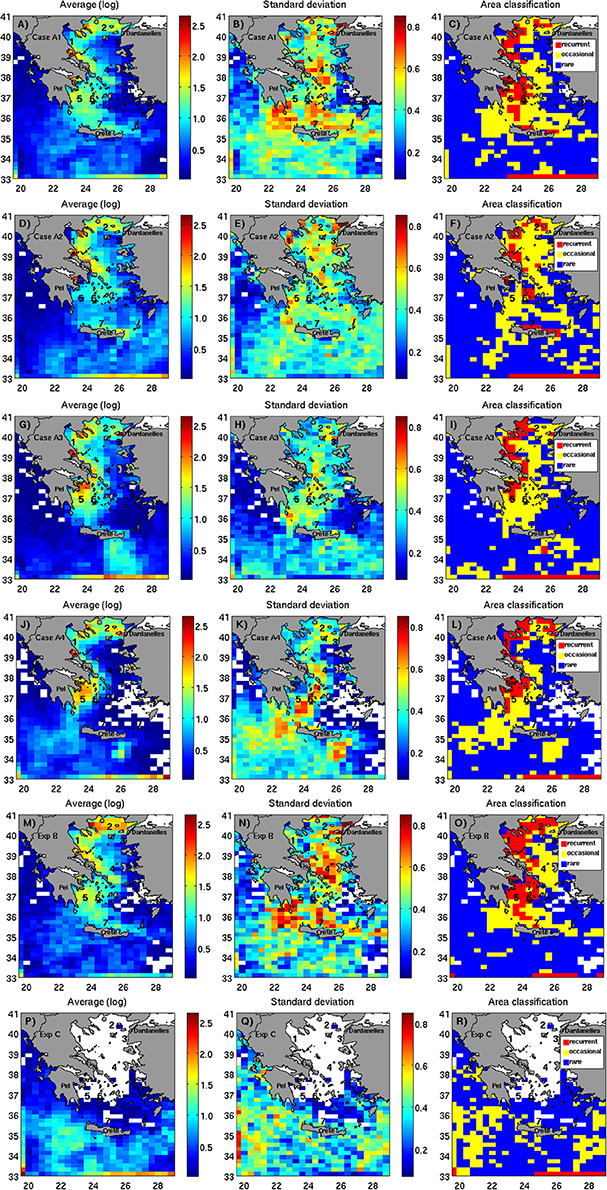
Figure 5. Average, standard deviation and classification maps of floating litter distributions for Cases A1 (A–C), A2 (D–F), A3 (G–I), and A4 (J–L), Experiment B (M–O), and Experiment C (P–R). First row refers to Case A1, second row to Case A2, third row to Case A3, fourth row to Case A4, fifth row to Experiment B and sixth row to Experiment C.
The release of particles on monthly basis, assumed in Experiment B, resulted in high concentration of floating litter particles in the North Aegean plateau, the Myrtoan basin and the areas outside the Saronikos and Thermaikos Gulf (Figure 5O). High values in standard deviation map of litter distribution (Figure 5N) implied occasional presence of floating litter particles mainly in the central Aegean, Cretan Sea and the region between Peloponnese and Crete island. The formulation of these concentration areas is driven by similar effects of surface circulation, as in Experiment A. Nevertheless, Experiment B tended to formulate recurrently high concentration of litter particles close to source regions and with larger geographic range, compare to Experiment A. These patterns were associated with the periodic inputs of litter in the model domain (once a month), which reduced gradually the advection time of particles; for instance, particles released in January tracked for 12 months, whereas particles released in December tracked for 1 month.
Differences in the distribution patterns of floating litter particles were noticed among Cases A1-A4. In Case A1, particles released on January and recurrently concentrated along Evia Island, in the Myrtoan basin, and the coastal waters of Crete island (Figure 5C). During January, the strong Evia coastal current turns southwest following a direct pathway along the Peloponnese coast (Figure 3A) resulting in the occasional retention of floating litter particles south of Peloponnese. Particles were occasionally found also in the central part of the Aegean Sea and in the Cretan Sea. Particles released on April (Figure 5F) were mostly aggregated in the Samothraki plateau, along the Evia coastline and the coast of Crete. In April, an inflow of modified Atlantic water is simulated through the straight between Crete and Peloponnese, also observed by Theocharis et al. (1993) spreading over the Myrtoan and Cretan Sea and thus resulting in relatively low concentration of litter. A similar inflow of Atlantic water was also observed by Theocharis et al. (1993). Meanwhile the southwest current from Evia to Peloponnese, simulated in January is diminished, resulting in a higher accumulation of floating litter particles along Evia coast and further south over the Cyclades plateau.
Simulations initiated in July (Case A3) and October (Case A4) resulted in relatively higher concentration of floating litter particles in the open waters of the Thermaikos Gulf (Figures 5I,L), which was absent in the other experiments. This was related to the anticyclonic circulation in Sporades basin in July, apparently blocking the strong southward Evia jet and thus preventing the fast advection of particles from Thermaikos Gulf. In October (Case A4) this was related to the relatively stronger westward current of BSW. The accumulation of floating litter particles in Saronikos Gulf, simulated in Case A3, can be attributed to the anticyclonic pathway of water coming from Evia Island and Central Aegean Sea and being directed into the gulf. The anticyclonic circulation in Saronikos and Myrtoan basin during October appears also to be responsible for the increase of floating litter particles in the broader area, and resulting to a very low abundance of floating particles in the Cretan Sea.
Classification areas, showing the concentration of floating particles originated separately from each source A, B, C, D, and E, were also depicted in Figures 6–9. Particles released from Source A (Thermaikos Gulf) ended up mostly in Saronikos Gulf and Myrtoan basin. This was particularly observed in Case A1 (January) and Case A4 (October) (Figures 6A,D), in which a strong current from Thermaikos Gulf was simulated along Evia coast and then was directed southwest (Figure 3). A more diffuse distribution of floating litter particles from source A can be observed in Case A2 (April) and Case A3 (July) (Figures 6B,C), associated with the much slower advection of floating particles from Thermaikos Gulf. For the sources B and C, high concentrations of floating particles were found in their corresponding source regions (North East Aegean). In the same extent, significant floating particle distribution was observed along their pathway in all different experiments, mainly along the Evia coast (Figures 7A,B,C), Thermaikos gulf (Figure 7D) and Saronikos Gulf (Figures 7A,C). Occasionally, the eastern part of the Cyclades plateau retained a significant number of floating particles. A somehow similar fate (with sources B, C) was found for particles originating from source D (Dardanelles strait), following a similar southward pathway from North East Aegean (Figure 8). Finally, the fate of floating particle distribution was observed in the Cretan Sea and particularly outside the model domain with no specific pattern (Figure 9).
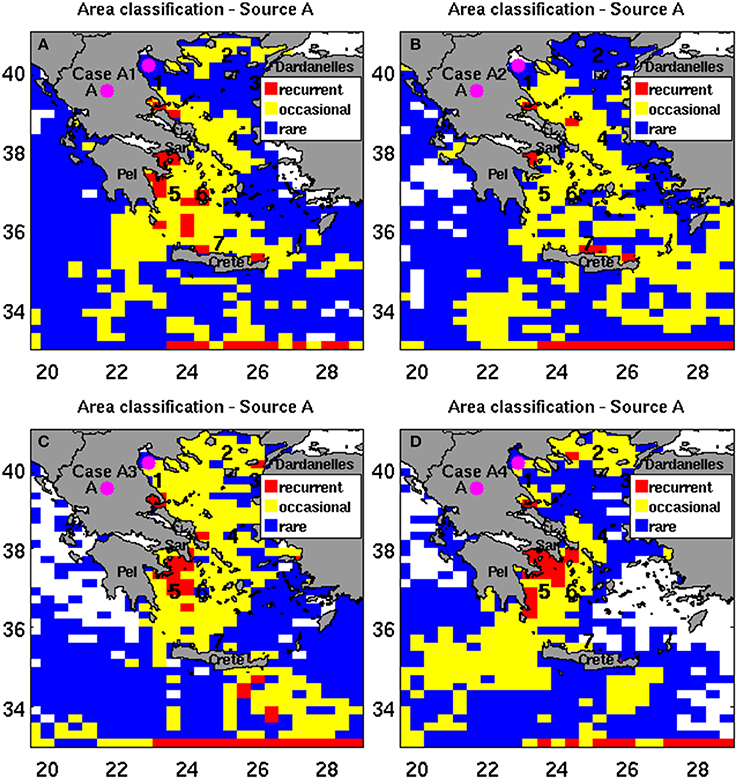
Figure 6. Area classification maps of floating litter particles originated from Source region A (Thermaikos Gulf) in Cases (A) A1, (B) A2, (C) A3, and (D) A4. Magenta dot point indicates the source region A.
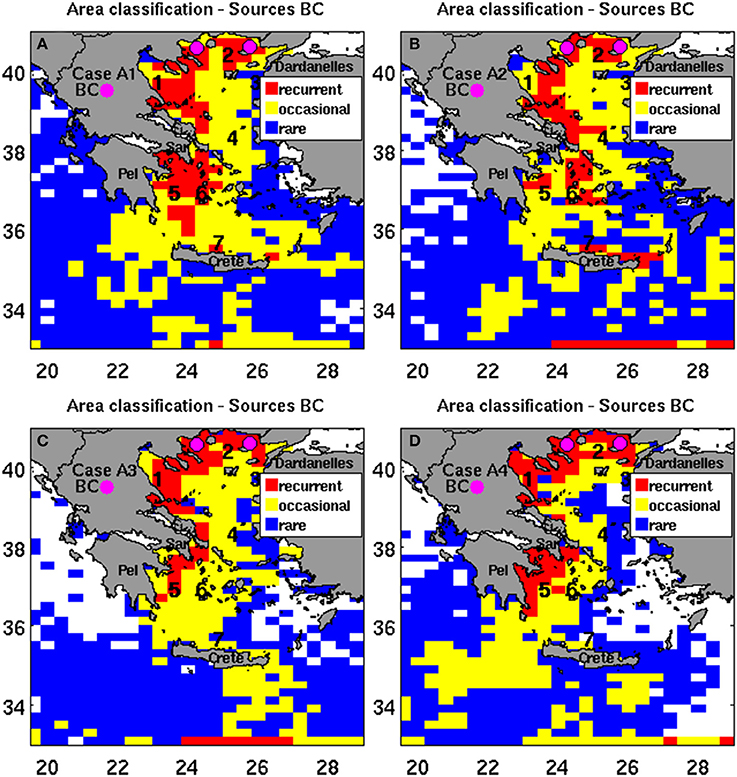
Figure 7. Area classification maps of floating litter particles originated from Source regions B, C (North Aegean) in Cases (A) A1, (B) A2, (C) A3, and (D) A4. Magenta dot points indicate the source regions B,C.
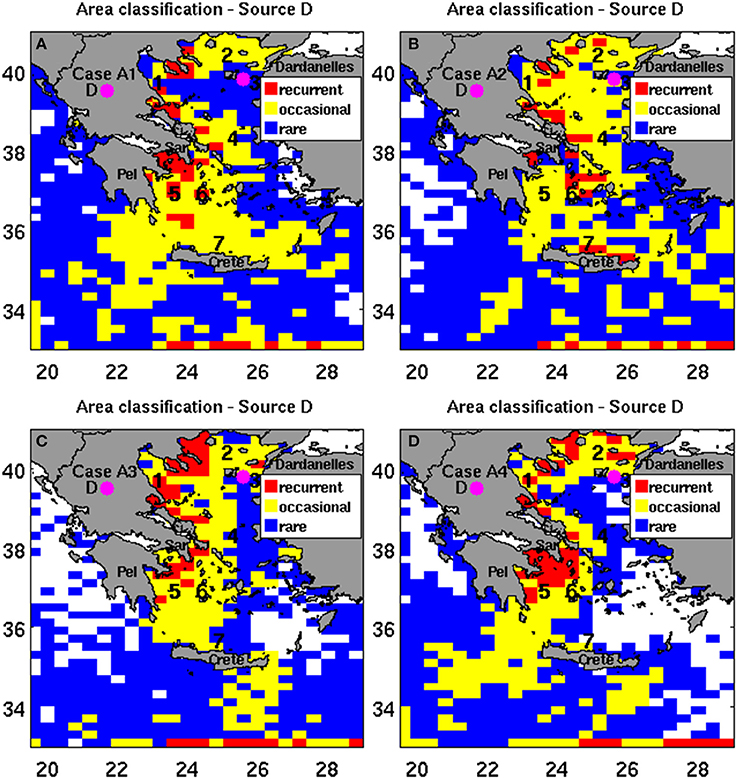
Figure 8. Area classification maps of floating litter particles originated from region D (Dardanelles strait) in Cases (A) A1, (B) A2, (C) A3, and (D) A4. Magenta dot point indicates the source region D.
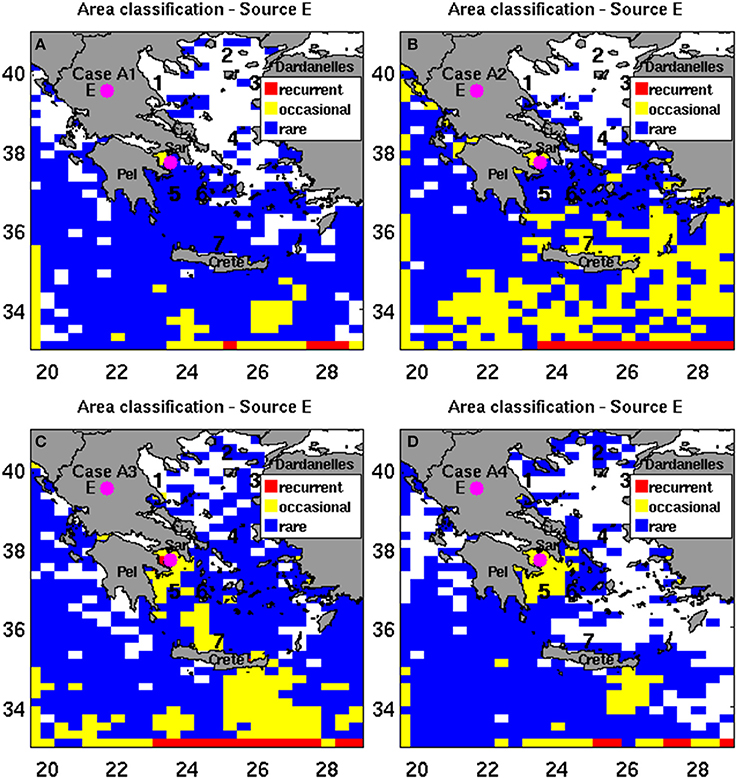
Figure 9. Area classification maps of floating litter particles originated from Source region E (Saronikos Gulf) in Cases (A) A1, (B) A2, (C) A3, and (D) A4. Magenta dot point indicates the source region E.
Finally, classification areas of floating particle distribution, derived from Experiment C, showed that litter particles were largely dispersed, leading to many cells with high standard deviation (Figure 5Q), and consequently to occasional areas without notable recurrent patterns of floating particle distribution (Figure 5R). Most particles were advected southern of the model domain; <7% particles, finally ended in the Aegean Sea.
Floating Litter Particles Escaping the Aegean Sea
The percentage of floating litter particles, escaping toward the western and eastern side of the Aegean Sea across the two transects T1 and T2, varied among the different source regions and experiments (Figure 10). Particles from all source regions escaped on average by 19.5% from transect T1 and by 14.5% from transect T2. With respect to source regions, particles originated from Source E (Saronikos Gulf) displayed the highest percent of escape: 47% from T1 and 42% from T2 (Figures 10A,B). In contrast, particles originated from Sources B and C escaped to a small extent: by 10.3% from T1 and by 5.8% from T2. In addition, Source A displayed higher percent of escaped litter (26.6% from T1, 20% from T2) compared to source D (18% from T1, 12.6% from T2) (Figures 10A,B). In Experiment B, particles showed low percentage of escape: by 11% from T1 and by 4% from T2 (Figure 10C).
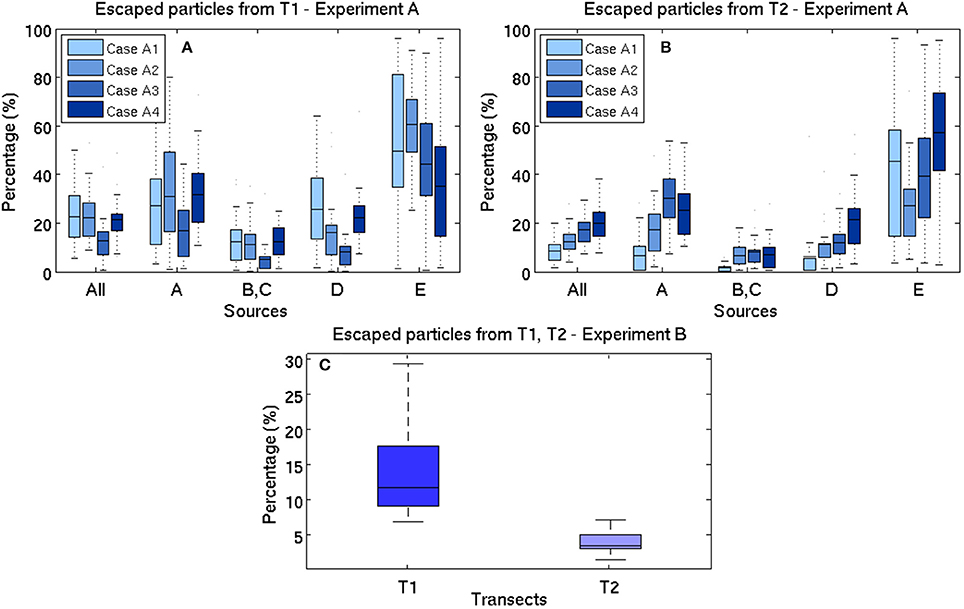
Figure 10. Box plots depicting the percentages of escaped particles across: (A) transect T1 in Experiment A; (B) transect T2 in Experiment A, and (C) transects T1, T2 in Experiment B.
Figure 11 shows the daily percent of escaped litter from transects T1, T2, averaged from annual simulations over 1990–2009. Particles started to escape from T1 after day-50 in Cases A2, A3 and day-20 in Cases A1 and A4 (Figure 11A). For all Cases A1-A4, particles escaped from transect T2 after day-80 (Figure 11B). Different release dates induced notable differences in the seasonal variations of escaped particles from transect T2; their final percent ranged from 4% (Figure 11B: Experiment B, green line) to 20% (Figure 11B: Case A4, yellow line). Experiment B illustrated that litter particles escaped mostly after day-20 from T1 and after day-80 from T2 (Figure 11, green lines).
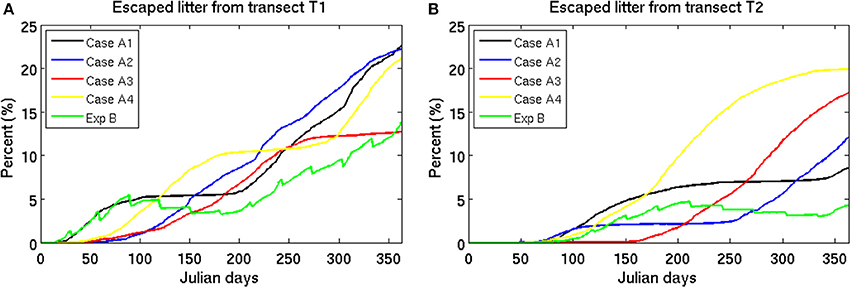
Figure 11. Daily percent of escaped particles across transects (A) T1 and (B) T2 in Cases A1-A4 and Experiment B.
Beaching of Floating Litter Particles
The distribution of floating litter particles, ending up in shoreline cells (define here as “beached”), was also depicted. Beached litter particles were mainly found along the coastline of Saronikos Gulf (Sar), eastern Peloponnese (Pel) and Pagasitikos Gulf (Pagas) (Figure 12). The topography of Aegean Sea, including hundreds of islands, favored also the beaching of some particles in several islands in Cyclades plateau, and specific gulfs in Crete island (Figures 12C,D), whereas few particles found all along the eastern part of Aegean Sea. Different release dates of particles did not change markedly the beaching patterns. The percent of beached particles changed slightly among the experiments: Case A1: 13.8%; Case A2: 11.4%; Case A3: 9.1%; Case A4: 9.9%; and Experiment B: 5.6%.
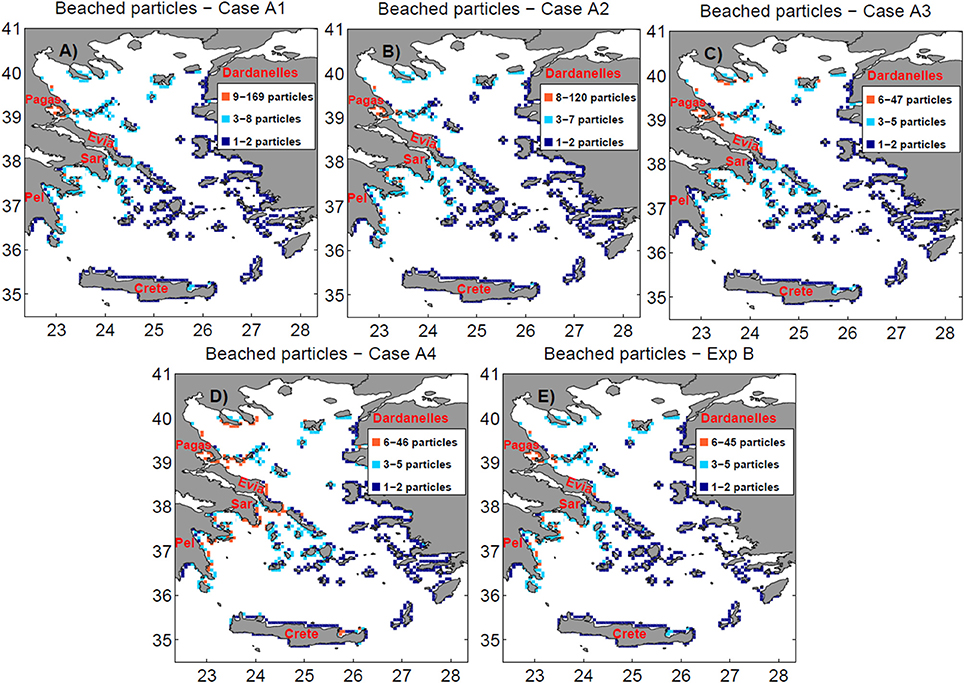
Figure 12. Number of beached particles (count) in Cases (A) A1, (B) A2, (C) A3, (D) A4, and (E) Experiment B. A particle is considered as “beached” here if it drifted in cells adjacent to land.
Discussion
Fate and Concentration of Floating Litter Particles
Our simulations investigated possible scenarios regarding the fate and distribution of floating litter particles originated from specific source regions in the Aegean Sea. High concentration of floating litter was recurrently found in the North Aegean Sea (Cases A1-A4, Experiment B), alongside the northeastern side of Evia island and in the wider area of Saronikos Gulf (Cases A1, A3, A4; Experiment B), and alongside Crete island (Cases A1, A2) (Figure 5). Despite differences in the occasional presence of litter items among the experiments, floating litter particles were exhibited occasional presence in most Cases in the Myrtoan basin (southwestern Aegean Sea) and the area between Crete and Peloponnese. In contrast, the eastern Aegean Sea, was identified as a region with relatively rare presence of floating litter particles (Figure 5). This area is found on the pathway of Levantine inflowing water, which has been assumed to be more or less free of floating litter in the simulations.
The transfer of floating litter particles between the different sea areas is another challenging task that the present study attempted to undertake, particularly related with the corresponding exchanges of floating litter particles with the eastern Mediterranean Sea. Simulation results demonstrated that particles escaped on average by 35% for experiment A and by 15% for experiment B from the Aegean Sea toward the Eastern Mediterranean Sea (Figure 10). In contrast, only 7% of the floating litter particles was found to end up in the Aegean Sea, after being released outside the model domain (Figure 5, Experiment C). These findings suggest that Aegean Sea might act mostly as a source rather than a receptor of floating litter pollution in the Eastern Mediterranean. Another factor that was investigated was the floating litter particles ending up on beaches (i.e., beaching). In the present study, on average 10% of floating litter particles were found in coastline cells. Most of them were found in the western Aegean Sea (Figure 12), which is in accordance with the areas where floating litter particles were found.
Transport Pathways
The major transport pathways of floating litter particles illustrated that source regions are interconnected (Figure 13). A characteristic example is the case of the Saronikos Gulf (Source Region E), from which 92% of particles escaped through transects T1 and T2 to eastern Mediterranean Sea, whereas it received particles from sources A, B, C, and D. In the same extent, source A (Thermaikos Gulf) received floating litter particles originating from the North Aegean Sea (sources B, C), while floating litter particles released from source D tended to occasionally be directed toward sources B and C. In addition, the northeastern area alongside the Evia island received particles that were released from sources B, C and D. Cretan Myrtoan Similar to other model studies (Yoon et al., 2010; Neumann et al., 2014; Mansui et al., 2015; Liubartseva et al., 2016), our results identified source-receptor transport pathways among the different sub-regions, demonstrating the transboundary character of the problem, with particles traveling far from their initial source points and finally concentrating into new regions.
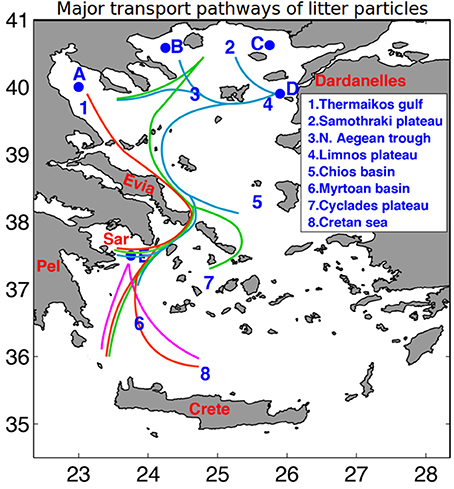
Figure 13. Schematic representation of major pathways of floating litter transport among the source regions. Different colors represent pathways originated from source regions A,B,C,D, and E.
Model Accuracy
One of the most critical point of the marine litter modeling studies is the validation of the results with data from surveys. Data on the distribution of floating litter are sparse in the Aegean Sea to validate rigorously the simulations and it is a problem that most of the relevant studies are facing. In this study, we evaluated the accuracy of the model by comparing qualitatively the simulated distribution of floating litter particles with available data compiled from different sub-regions and studies, marked as R1 to R6 in Figure 14. Observed floating litter abundance and the corresponding references are listed in Table 2. Although there is no direct way to distinguish if the measurements are the result of litter transport or source of litter, the model was adequate to predict high concentration of litter in the Saronikos Gulf (sub-region R1) and Crete island (sub-region R4). Similar to data, model predicted low litter concentration and beached particles in the eastern coastline of Aegean Sea (sub-region R3). Results for sub-region R3 (Topcu et al., 2010) refer to bigger floating litter particles. However, they have been included in the verification of the model knowing that different sized items have different buoyancy properties, but also knowing that the transportation mechanisms still remain the same. The model did not predict high floating litter particles distribution in the northern part of Thermaikos Gulf (sub-region R2); however it was considered correctly as a source of litter through source region A. Finally, occasional presence of litter predicted by the model in the area between Crete island and Peloponnese agrees with observed floating litter in sub-regions R5 and R6. This signifies that this area may be indeed a passage of escaped litter, since it does not appear as an evident source of litter pollution.
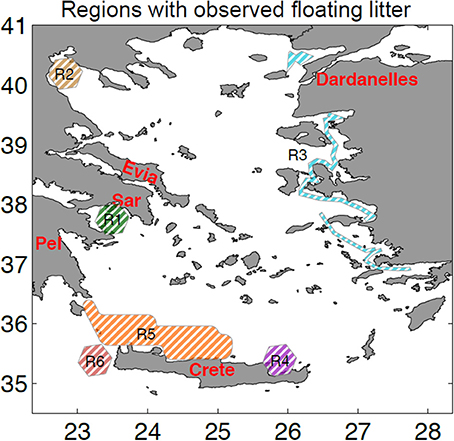
Figure 14. Subregions of sampled floating litter in the Aegean Sea; R1: Saronikos Gulf (Valavanidis and Vlachogianni, 2012; MERMAID Report, 2016), R2: Thermaikos Gulf (Valavanidis and Vlachogianni, 2012), R3: Eastern Aegean Sea (Topcu et al., 2010), R4: Crete island (Cózar et al., 2015), R5: Southwest Aegean Sea (Kornilios et al., 1998), and R6: Crete island (Cózar et al., 2015).
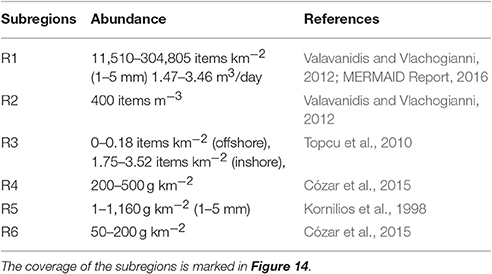
Table 2. Measurements of floating litter abundance from different subregions (R1 to R6) in the Aegean Sea.
Model Results and Hypotheses
Model results are subject to assumptions taken into consideration during the model setup. First, different source regions were considered as the starting point of the floating litter particles, based on big coastal cities, major rivers, ports, maritime and fisheries activity and coastal activity (e.g., tourism). However, including other unspecified sources (e.g., maritime and shipping, small-scale fisheries, or potential sources in eastern coastline of the Aegean Sea) might partially modify the obtained information regarding the distribution of floating litter particles in the Aegean Sea. Second, beaching of litter particles is another factor which significantly contributes to the congregation of litter particles on the coastlines (Yoon et al., 2010; Lebreton et al., 2012; Mansui et al., 2015). Herein, particles did not strand when found on land cells, following Neumann et al. (2014) who treated coasts as reflective boundaries. This assumption was essential, since the complex topography of the Aegean Sea, with hundred of islands, caused the vast majority of particles to be stuck on them due to random part of the particle-tracking model. In addition, our study refers to floating particles and not to bigger litter for which wash up on shore is common. Exploring how beaching of particles affect the model performance is, however, a worthy issue for future consideration. Third, assuming simulations with integration time of 1 year, we included the effect of seasonal surface circulation variability. This integration time was also proper for studying the drift of litter particles on regional scale, as proposed by Mansui et al. (2015). Identification of long-term trends and possible permanent accumulation areas would require simulations with multi-annual integration time (Lebreton et al., 2012). Fourth, floating litter particles have been considered here as passive drifters advected exclusively by surface currents. So, we did not include other particle properties related to buoyancy (Yoon et al., 2010; Carlson et al., 2017), windage (Neumann et al., 2014), subsurface movement, and sinking (Hardesty et al., 2017). All these properties need to be better understood and taken into account in further modeling.
Summary and Conclusion
The Mediterranean Sea has been described as one of the areas most affected by marine litter in the world (UNEP/MAP, 2016). The Aegean Sea is an archipelagos with extended coastline and numerous islands and no information on the exact pathways of floating litter transport and distribution exists. The use of coupled ocean circulation and particle-tracking models is important to better understand these processes and guide scientists on surveying and monitoring floating litter particles (Yoon et al., 2010; Neumann et al., 2014; Liubartseva et al., 2016).
In this study, we conducted a series of transport simulations to describe the fate and distribution of floating litter particles, after being released from specific source regions in the Aegean Sea. Model analysis pictured that floating litter particles tend to concentrate mostly over in the north Aegean Sea, in the Saronikos Gulf and Evia and Crete islands, while the eastern part of the Aegean archipelagos was the least affected. Beached washed up litter items were mainly found along the coastline of Saronikos Gulf, eastern Peloponnese and Pagasitikos Gulf while the Aegean Sea physiography favored the beaching of litter items in Cyclades island and in specific gulfs of Crete island. The beaches and offshore areas of high floating litter concentration may be considered under priority for future surveys; this will help importantly to assess the status of floating litter pollution in the Aegean Sea, improve the credibility of future model studies in our study area, and after all guide science and policy judgment.
Author Contributions
DP: Conceptualized and guided the study, analyzed the results and wrote parts of the paper; CI: Analyzed the results and wrote parts of the paper; GP: Contributed to the discussion of the results; KT: Performed the simulations, analyzed the results and wrote parts of the paper. All authors read and edited the paper.
Conflict of Interest Statement
The authors declare that the research was conducted in the absence of any commercial or financial relationships that could be construed as a potential conflict of interest.
Acknowledgments
Support for this research came from the LIFE DEBAG project (LIFE14 GIE/GR/001127). The drifter data were obtained from a study funded by the U.S. Office of Naval Research (ONR grants N000140110134 and N000140310439), in collaboration with Villy Kourafalou, Don Olson and Bill Johns of the University of Miami. The manuscript was greatly improved by the three reviewers.
References
Androulidakis, Y. S., Krestenitis, Y. N., and Kourafalou, V. H. (2012). Connectivity of North Aegean circulation to the Black Sea water budget. Cont. Shelf Res. 48, 8–26. doi: 10.1016/j.csr.2012.08.019
Barnes, D. K. A., Galgani, F., Thompson, R. C., and Barlaz, M. A. (2009). Accumulation and fragmentation of plastic debris in global environments. Phil. Trans. R. Soc. B 364, 1985–1998. doi: 10.1098/rstb.2008.0205
Bellier, E., Planque, B., and Petitgas, P. (2007). Historical fluctuations in spawning location of anchovy (Engraulis encrasicolus) and sardine (Sardina pilchardus) in the Bay of Biscay during 1967-73 and 2000-2004. Fish. Oceanogr. 16, 1–15. doi: 10.1111/j.1365-2419.2006.00410.x
Blumberg, A. F., and Mellor, G. L. (1983). Diagnostic and prognostic numerical circulation studies of the South Atlantic Bight. J. Geophys. Res. 88, 4579–4592. doi: 10.1029/JC088iC08p04579
Carlson, D. F., Suaria, G., Aliani, S., Fredj, E., Fortibuoni, T., Griffa, A., et al. (2017). Combing litter observations with a regional ocean model to identify sources and sinks of floating debris in a semi-enclosed basin: the adriatic sea. Front. Mar. Sci. 4:78. doi: 10.3389/fmars.2017.00078
Christensen, O. B., Drews, M., Christensen, J. H., Dethloff, K., Ketelsen, K., Hebestadt, I., et al. (2006). The HIRHAM Regional Climate Model, Version 5 (β). Techical Report. Available online at: www.dmi.dk/dmi/tr06-17.
Cózar, A., Sanz-Martín, M., Martí, E., González-Gordillo, J. I., Ubeda, B., Gálvez, J. Á., et al. (2015). Plastic accumulation in the Mediterranean Sea. PLoS ONE 10:e0121762. doi: 10.1371/journal.pone.0121762
Eriksen, M., Lebreton, L. C. M., Carson, H. S., Thiel, M., Moore, C. J., Borerro, J. C., et al. (2014). Plastic pollution in the world's oceans: more than 5 trillion plastic pieces weighing over 250,000 Tons Afloat at Sea. PLoS ONE 9:e111913. doi: 10.1371/journal.pone.0111913
Galgani, F. (2015). Marine litter, future prospects for research. Front. Mar. Sci. 2:87. doi: 10.3389/fmars.2015.00087
Giannoulaki, M., Iglesias, M., Tugores, M. P., Bonanno, A., Patti, B., De Felice, A., et al. (2013). Characterizing the potential habitat of European anchovy Engraulis encrasicolus in the Mediterranean Sea, at different life stages. Fish. Oceanogr. 22, 69–89. doi: 10.1111/fog.12005
Hardesty, B. D., Harari, J., Isobe, A., Lebreton, L., Maximenko, N., Potemra, J., et al. (2017). Using numerical model simulations to improve the understanding of micro-plastic distribution and pathways in the marine environment. Front. Mar. Sci. 4:30. doi: 10.3389/fmars.2017.00030
Horton, C., Clifford, M., Schmitz, J., and Kantha, L. H. (1997). A real time oceanographic nowcast/forecast system for the Mediterranean Sea. J. Geophys. Res. 102, 25123–25156. doi: 10.1029/97JC00533
Ioakeimidis, C., Papatheodorou, G., Fermeli, G., Streftaris, N., and Papathanassiou, E. (2015). Use of ROV for assessing marine litter on the seafloor of Saronikos Gulf (Greece): a way to fill data gaps and deliver environmental education. Springerplus 4:463. doi: 10.1186/s40064-015-1248-4
Ioakeimidis, C., Zeri, C., Kaberi, H., Galatchi, M., Antoniadis, K., Streftaris, N., et al. (2014). A comparative study of marine litter on the seafloor of coastal areas in the Eastern Mediterranean and Black Seas. Mar. Pollut. Bull. 89, 296–304. doi: 10.1016/j.marpolbul.2014.09.044
Jain, A. K. (2010). Data clustering: 50 years beyond K-means. Pattern Recogn. Lett. 31, 651–666. doi: 10.1016/j.patrec.2009.09.011
Katsanevakis, S., and Katsarou, A. (2004). Influences on the distribution of marine debris on the seafloor of shallow coastal areas in Greece (Eastern Mediterranean). Water Air Soil Pollut. 158, 325–337. doi: 10.1023/B:WATE.0000049183.17150.df
Kontoyiannis, H., Kourafalou, V. H., and Papadopoulos, V. (2003). The seasonal characteristics of the hydrology and circulation in the Northwest Aegean Sea (Eastern Mediterranean): observations and modeling. J. Geophys. Res. 108:3302. doi: 10.1029/2001JC001132
Kornilios, S., Drakopoulos, P. G., and Dounas, C. (1998). Pelagic tar, dissolved/dispersed petroleum hydrocarbons and plastic distribution in the Cretan Sea, Greece. Mar. Pollut. Bull. 36, 989–993. doi: 10.1016/S0025-326X(98)00102-7
Korres, G., Hoteit, I., and Triantafyllou, G. (2007). Data assimilation into a Princeton Ocean Model of the Mediterranean Sea using advanced Kalman filters. J. Mar. Syst. 65, 84–104. doi: 10.1016/j.jmarsys.2006.09.005
Korres, G., and Lascaratos, A. (2003). A one-way nested, eddy resolving model of the Aegean and Levantine basins: implementation and climatological runs. Ann. Geophys. 21, 205–220. doi: 10.5194/angeo-21-205-2003
Korres, G., Nittis, K., Perivoliotis, L., Tsiaras, K., Papadopoulos, A., Triantafyllou, G., et al. (2010). Forecasting the Aegean Sea hydrodynamics within the POSEIDON-II operational system. J. Oper. Oceanogr. 3, 37–49. doi: 10.1080/1755876X.2010.11020112
Kourafalou, V., Tsiaras, K., Olson, D., Johns, B., Papadopoulos, A., Kontoyiannis, H., et al. (2006). “North Aegean circulation: results from a drifter array and model simulations,” in Proceedings of 8th Panhellenic Symposium on Oceanography and Fisheries (Thessaloniki), 515–518.
Koutsodendris, A., Papatheodorou, A., Kougiourouki, O., and Georgiadis, M. (2008). Benthic marine litter in four Gulfs in Greece, Eastern Mediterranean; abundance, composition and source identification. Estuarine Coast. Shelf Sci. 77, 501–512. doi: 10.1016/j.ecss.2007.10.011
Kubota, M. (1994). A mechanism for the accumulation of floating marine debris north of Hawaii. J. Phys. Ocean. 24, 1059–1604. doi: 10.1175/1520-0485(1994)024<1059:AMFTAO>2.0.CO;2
Law, K. L., Morét-Ferguson, S. E., Goodwin, D. S., Zettler, E. R., DeForce, E., Kukulka, T., et al. (2014). Distribution of surface plastic in the eastern Pacific Ocean from an 11-year data set. Environ. Sci. Technol. 48, 4732–4738. doi: 10.1021/es4053076
Lebreton, L. C. M., and Borrero, J. C. (2013). Modeling the transport and accumulation floating debris generated by the 11 March 2011 Tohoku tsunami. Mar. Pollut. Bull. 66, 53–58. doi: 10.1016/j.marpolbul.2012.11.013
Lebreton, L. C. M., Greer, S. D., and Borrero, J. C. (2012). Numerical modeling of floating debris in the worlds oceans. Mar. Pollut. Bull. 64, 653–661. doi: 10.1016/j.marpolbul.2011.10.027
Liubartseva, S., Coppini, G., Lecci, R., and Creti, S. (2016). Regional approach to modeling the transport of floating plastic litter in the Adriatic Sea. Mar. Pollut. Bull. 103, 115–127. doi: 10.1016/j.marpolbul.2015.12.031
Lynch, D. R., Greenberg, D. A., Bilgili, A., McGillicuddy, D. J., Manning, J. P., and Aretxabaleta, A. L. (2014). Particles in the Coastal Ocean: Theory and Applications. Cambridge University Press, 560.
Mansui, J., Molcard, A., and Ourmières, Y. (2015). Modeling the transport and accumulation of floating marine debris in the Mediterranean basin. Mar. Pollut. Bull. 91, 249–257. doi: 10.1016/j.marpolbul.2014.11.037
Martinez, E., Maamaatuaiahutapu, K., and Taillandier, V. (2009). Floating marine debris surface drift: convergence and accumulation toward the South Pacific subtropical gyre. Mar. Pollut. Bull. 58, 1347–1355. doi: 10.1016/j.marpolbul.2009.04.022
Maximenko, N., Hafner, J., and Niiler, P. (2012). Pathways of marine litter derived from trajectories of Langarian drifters. Mar. Pollut. Bull. 65, 51–62. doi: 10.1016/j.marpolbul.2011.04.016
Mellor, G. L., and Yamada, T. (1982). Development of a turbulence closure model for geophysical fluid problems. Rev. Geophys. 20, 851–875, doi: 10.1029/RG020i004p00851
MERMAID Report (2016). “Test the designed monitoring programs applied in the study areas (Deliverable 3.3),” in Marine Environmental Targets Linked to Regional Management Schemes Based on Indicators Developed for the Mediterranean (MERMAID), eds O. Guven (IMS-METU), B. Salihoğlu (IMS-METU), F. Witkowski (IFREMER), R. Verney (IFREMER), S. Bonhommeau (IFREMER), B. Andral (IFREMER), M. Pantazi (HCMR), H. Kaberi (HCMR), C. Zeri (HCMR), C. Tsangaris (HCMR), E. Prifti (HCMR), C. Ioakeimidis (HCMR).Ioakeimidis C., Zeri C., Tsangkari K. et al. (Athens: Assessment of Microplastic Abundance in the Saronikos Gulf).
Neumann, D., Callies, U., and Matthies, M. (2014). Marine litter ensemble transport simulations in the southern North Sea. Mar. Pollut. Bull. 86, 219–228. doi: 10.1016/j.marpolbul.2014.07.016
Nittis, K., Lascaratos, A., and Theocharis, A. (2003). Dense water formation in the Aegean Sea: numerical simulations during the Eastern Mediterranean Transient. J. Geophys. Res. 108:8120. doi: 10.1029/2002JC001352
Nittis, K., and Perivoliotis, L. (2002). Circulation and hydrological characteristics of the North Aegean Sea: a contribution from real-time buoy measurements. Med. Mar. Sci. 3, 21–32. doi: 10.12681/mms.255
NOAA (2016). National Oceanic and Atmospheric Administration Marine Debris Program. Report on Modeling Oceanic Transport of Floating Marine Litter. Silver Spring, MD. 21.
Olson, D. B., Kourafalou, V. H., Johns, W. E., Samuels, G., and Veneziani, M. (2007). Aegean Surface Circulation from a Satellite-Tracked Drifter Array. J. Phys. Ocean. 37, 1898–1917. doi: 10.1175/JPO3028.1
Papadopoulou, K.-N., Anastasopoulou, A., Mytilineou, C. H., Smith, C. J., and Stamouli, C. (2015). “Seabed marine litter, comparison of 4 Aegean trawling grounds,” in Proceedings of 11th Panhellenic Symposium of Oceanography and Fisheries (Lesvos), 381–385.
Pastorelli, G., Cucci, C., Garcia, O., Piantanida, G., Elnaggar, A., Cassar, M., et al. (2014). Environmentally induced colour change during natural degradation of selected polymers. Polym. Degrad. Stab. 107, 198–209. doi: 10.1016/j.polymdegradstab.2013.11.007
Pedrotti, M. L., Petit, S., Elineau, A., Bruzaud, S., Crebassa, J.-C., Dumontet, B., et al. (2016). Changes in the floating plastic pollution of the Mediterranean Sea in relation to the distance to land. PLoS ONE 11:e0161581. doi: 10.1371/journal.pone.0161581
Pollani, A., Triantafyllou, G., Petihakis, G., Konstantinos, N., Dounias, K., and Koutitas, C. (2001). The Poseidon operational tool for the prediction of floating pollutant transport. Mar. Pollut. Bull. 43, 270–278. doi: 10.1016/S0025-326X(01)00080-7
Reisser, J., Slat, B., Noble, K., du Plessis, K., Epp, M., Proietti, M., et al. (2015). The vertical distribution of buoyant plastics at sea: an observational study in the North Atlantic Gyre. Biogeosciences 12, 1249–1256. doi: 10.5194/bg-12-1249-2015
Ryan, P. G. (2014). Litter survey detects the South Atlantic “garbage patch.” Mar. Pollut. Bull. 79, 220–224. doi: 10.1016/j.marpolbul.2013.12.010
Ryan, P. G. (2015). Does size and buoyancy affect the long-distance transport of floating litter? Environ. Res. Lett. 10:084019. doi: 10.1088/1748-9326/10/8/084019
Saraux, C., Fromentin, J.-M., Bigot, J.-L., Bourdeix, J.-H., Morfin, M., Roos, D., et al. (2014). Spatial Structure and Distribution of Small Pelagic Fish in the Northwestern Mediterranean Sea. PLoS ONE 9:e111211. doi: 10.1371/journal.pone.0111211
Sayin, E., Eronat, C., Uçkaç, S., and Beŧiktepe, Åđ. T. (2011). Hydrography of the eastern part of the Aegean Sea during the East Mediterranean Transient (EMT). J. Mar. Syst. 88, 502–515. doi: 10.1016/j.jmarsys.2011.06.005
Sebille, E., Wilcox, C., Lebreton, L., Maximenko, N., Hardesty, B. D., Franeker, J. A., et al. (2015). A global inventory of small floating plastic debris. Environ. Res. Lett. 10:124006. doi: 10.1088/1748-9326/10/12/124006
Stefatos, A., Charalampakis, M., Papatheodorou, G., and Ferentinos, G. (1999). Marine debris on the seafloor of the Mediterranean Sea: examples from two enclosed gulfs in Western Greece. Mar. Pollut. Bull. 36, 389–393. doi: 10.1016/S0025-326X(98)00141-6
Suaria, G., and Aliani, S. (2014). Floating debris in the Mediterranean Sea. Mar. Pollut. Bull. 86, 494–504. doi: 10.1016/j.marpolbul.2014.06.025
Theocharis, A., Balopoulos, E., Kioroglou, S., Kontoyiannis, H., and Iona, A. (1999). A synthesis of the circulation and hydrography of the south Aegean Sea and the Straits of the Cretan Arc (March 1994-January 1995). Progr. in Ocean. 44, 469–509. doi: 10.1016/S0079-6611(99)00041-5
Theocharis, A., and Georgopoulos, D. (1993). Dense water formation over the Samothraki and Limnos plateaux in the North Aegean Sea (Eastern Mediterranean Sea). Cont. Shelf Res. 13, 919–939. doi: 10.1016/0278-4343(93)90017-R
Theocharis, A., Georgopoulos, D., Lascaratos, A., and Nittis, K. (1993). Water masses and circulation in the central region of the eastern Mediterranean: eastern Ionian, south Aegean and northwest Levantine, 1986-1987. Deep-Sea Res. Pt II 40, 1121–1142. doi: 10.1016/0967-0645(93)90064-T
Thompson, R. C., Olsen, Y., Mitchell, R. P., Davis, A., Rowland, S. J., John, A. W. G., et al. (2004). Lost at sea: where is all the plastic? Science 304, 838. doi: 10.1126/science.1094559
Topcu, E. D., Tonay, A. M., and Öztürk, B. (2010). Preliminary study on marine litter in the Aegean Sea. Rapp. Comm. int. Mer Médit. 39:804.
Tsiaras, K. P., Petihakis, G., Kourafalou, V. H., and Triantafyllou, G. (2014). Impact of the river nutrient load variability on the North Aegean ecosystem functioning over the last decades. J. Sea Res. 86, 97–109. doi: 10.1016/j.seares.2013.11.007
Tugrul, S., Besiktepe, S., and Salihoglu, I. (2002). Nutrient exchange fluxes between the Aegean and Black Seas through the Marmara Sea. Mediterr. Mar. Sci. 3, 33–42. doi: 10.12681/mms.256
UNEP/MAP (2016). Marine litter assessment in the Mediterranean 2015. United Nations Environment Programme Mediterranean Action Plan (UNEP/MAP), 86. Available online at: http://hdl.handle.net/20.500.11822/7098
Valavanidis, A., and Vlachogianni, T. (2012). MARINE LITTER: Man-made Solid Waste Pollution in the Mediterranean Sea and Coastline. Abundance, Composition and Sources Identification. Science Advances on Environmental Chemistry, Toxicology and Ecotoxicology.
Yoon, J. H., Kawanob, S., and Igawa, S. (2010). Modeling of marine litter drift and beaching in the Japan Sea. Mar. Pollut. Bull. 60, 448–463. doi: 10.1016/j.marpolbul.2009.09.033
Zavatarelli, M., and Mellor, G. L. (1995). A numerical study of the Mediterranean Sea circulation. J. Phys. Oceanogr. 25, 1384–1414. doi: 10.1175/1520-0485(1995)025<1384:ANSOTM>2.0.CO;2
Zervakis, V., and Georgopoulos, D. (2002). Hydrology and circulation in the north Aegean (eastern Mediterranean) throughout 1997-1998. Mediterr. Mar. Sci. 3, 7–21. doi: 10.12681/mms.254
Appendix
List of Threshold Values That Define Classification Areas of Floating Litter Distribution
The limiting thresholds that defined the low, medium and high values of average and standard deviation maps of floating litter distribution were calculated using the K-means clustering algorithm (Jain, 2010). The limiting values are listed in Table A1 and used to classify the distribution of particles into three categories: recurrent, occasional, and rare, following the approach of Saraux et al. (2014). The classification criteria are also presented in Table A1.
Keywords: floating marine litter, particle-tracking model, Aegean Sea, fate, distribution, pathways
Citation: Politikos DV, Ioakeimidis C, Papatheodorou G and Tsiaras K (2017) Modeling the Fate and Distribution of Floating Litter Particles in the Aegean Sea (E. Mediterranean). Front. Mar. Sci. 4:191. doi: 10.3389/fmars.2017.00191
Received: 29 November 2016; Accepted: 01 June 2017;
Published: 20 June 2017.
Edited by:
Christopher Kim Pham, University of the Azores, PortugalReviewed by:
Stefano Aliani, Consiglio Nazionale Delle Ricerche, ItalyGiovanni Coppini, Fondazione Centro Euro-Mediterraneo sui Cambiamenti Climatici, Italy
Joseph Harari, Instituto Oceanográfico da USP, Brazil
Copyright © 2017 Politikos, Ioakeimidis, Papatheodorou and Tsiaras. This is an open-access article distributed under the terms of the Creative Commons Attribution License (CC BY). The use, distribution or reproduction in other forums is permitted, provided the original author(s) or licensor are credited and that the original publication in this journal is cited, in accordance with accepted academic practice. No use, distribution or reproduction is permitted which does not comply with these terms.
*Correspondence: Christos Ioakeimidis, Y2lvYWtlaW1AaGNtci5ncg==