- 1Lowestoft Laboratory, The Centre for the Environment, Fisheries and Aquaculture Science, Lowestoft, UK
- 2Department of Environment and Health, VU Amsterdam, Amsterdam, Netherlands
- 3Marine and Coastal Systems, Deltares, Delft, Netherlands
- 4Animal Science Unit—Aquatic Environment and Quality, Institute for Agricultural and Fisheries Research, Oostende, Belgium
- 5Ifremer, LEMAR UMR 6539 UBO-Centre National de la Recherche Scientifique-IRD-Ifremer, Plouzane, France
- 6Centre National de la Recherche Scientifique-Institute of Ecology and the Environment, Plouzane, France
Microplastic contamination was determined in sediments of the Southern North Sea and floating at the sea surface of North West Europe. Floating concentrations ranged between 0 and 1.5 microplastic/m3, whereas microplastic concentrations in sediments ranged between 0 and 3,146 particles/kg dry weight sediment. In sediments, mainly fibers and spheres were found, whereas at the sea surface fragments were dominant. At the sea surface, concentrations of microplastics are lower and more variable than in sediments, meaning that larger sample sizes and water volumes are required to find detectable concentrations. We have calculated the widths of the confidence intervals (CI) for different sample sizes, to give a first indication of the necessary sample size for a microplastic survey at the water surface. Higher concentrations of floating microplastics were found near estuaries. In sediments, estuaries and areas with a high organic carbon content were likely hotspots. Standardization of monitoring methods within marine regions is recommended to compare and assess microplastics pollution over time.
Introduction
Marine litter accumulating in the marine environment may be one of the greatest threats facing the planet. The exact quantity of plastic in the ocean and volumes entering the ocean from waste generated on land is unknown. Recent studies estimate that 275 million metric tons (MT) of plastic waste was generated in 192 coastal countries in 2010, of which 4.8–12.7 million MT could have entered the ocean (Jambeck et al., 2015). It has been estimated there are 5.25 trillion pieces of plastic debris in the ocean, of that mass, 269,000-ton float on the sea surface (Cózar et al., 2014; Eriksen et al., 2014; van Sebille et al., 2015). Due to UV radiation and mechanical forces, this plastic slowly break down into smaller and smaller fragments below 5 mm, also known as microplastics (GESAMP, 2015). The origin of these fragments can be broken down fishing nets or lines, plastic films and bottles, remains of oxo-biodegradable plastic, industrial raw material like pellets, but also synthetic fibers from textiles as a result of washing clothes or other particular direct sources of microplastics, for example facial cleansers (Derraik, 2002; Arthur et al., 2009; Barnes et al., 2009; Fendall and Sewell, 2009; Browne et al., 2011; Leslie et al., 2011). In Norway, they found that abrasion from tires and roadmarking was the biggest source of microplastics, followed by dust and particles from plastic based paint (Sundt et al., 2014). Next to breakdown, city storm water effluent and road runoff could thus be another major pathway for microplastics (Eriksen et al., 2013a; McCormick et al., 2014). Some of these microplastics will escape water treatment (Cheung and Fok, 2016) and can be transported via rivers downstream to estuaries and the marine environment (Moore et al., 2011; Lechner et al., 2014; Lima et al., 2014; Rech et al., 2014). In Brazil, the highest amount of microplastics was observed during the late rainy season, when the environment is under influence of the highest river flow, which induces the runoff of plastic fragments to the lower estuary (Lima et al., 2014). Microplastic fibers can even be deposited by atmospheric fallout (Dris et al., 2016).
A large proportion of plastics normally float on the surface being less dense than seawater, however the buoyancy and density of plastics depend on polymer type and may change during their residence at sea due to weathering and biofouling and therefore spread across surface, water column and sediments (Ye and Andrady, 1991; Morishige et al., 2007). Recent studies have demonstrated that pollution of microplastics, particles <5 mm, has spread at the surface of oceans, in the water column and in sediments, even in the deep sea (Woodall et al., 2014). Concentrations at the water surface range from thousands to hundred thousand of particles km−2. Because of their size microplastics are available to a broad range of organisms and have already been shown to be ingested by several species (Cole et al., 2011). The ingestion of microplastics by species at the base of the food web causes human food safety concerns as little is known about their effects and transfer across trophic levels (Vethaak and Leslie, 2016). Moreover, plastics can leach toxic additives and accumulate persistent organic pollutants (POPs) while residing in the marine environment. Some of these POPs are known to have endocrine disruptive and carcinogenic effects (Rios Mendoza and Jones, 2015). Furthermore, plastic particles create habitats for micro-organisms and other species, allowing potential invasive species to transfer to new areas of the ocean (Gregory, 2009; Keswani et al., 2016).
International attention is focusing more and more on the problem of marine litter, including microplastics. In Europe, marine litter and microplastics are included in the Marine Strategy Framework Directive (MSFD), specific information in relation to trends in the amount, distribution and, where possible, composition of micro-particles (in particular microplastics) is requested (criterion 10.1.3 of the MSFD; Galgani et al., 2013). There are several other actions and measures directly related to microplastics and their sources e.g., microbead bans and thus baseline studies are urgently needed to produce appropriate regional baselines to monitor future amounts of microplastics and follow progress of action plans and where required assess potential impacts on the marine environment (Galgani et al., 2014).
This study presents the outcomes of two baseline studies, looking at microplastics in sediments of the Southern North Sea and floating at the water surface in seas of North West Europe. Samples from the surface layers of the North Sea, Irish Sea, Celtic Sea, and Channel Area were analyzed and compared with sediment samples in approximately the same region. Even though sampling locations do not overlap exactly in terms of spatio-temporal scale, it is the first study in the North Sea region in which results from both matrices are compared. Since sediment is thought to be a sink (Morét-Ferguson et al., 2010; Foekema et al., 2013; Van Cauwenberghe et al., 2013; Cózar et al., 2014) for microplastics, research on the occurrence and relationship between floating and deposited microplastics is paramount in understanding the physical processes acting on plastic particles and predicting hotspots for monitoring and clean-up (Gago et al., 2016).
Materials and Methods
Sediment
Sampling took place on the Dutch continental shelf in 2014; on the Belgian continental shelf in 2013 and 2014; in the North Sea and English Channel area of the UK in 2013 and 2014 (Figure 1); and in the French part of the English Channel in 2014. In total, 27 locations were sampled (Table 1). The sample size differed per country; the UK had the smallest number of sampling stations (4 stations), whereas the Netherlands had the highest number of stations (11 stations).
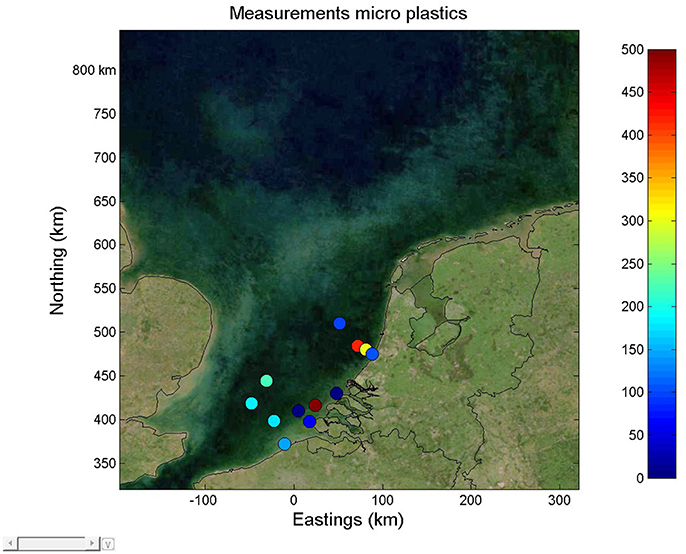
Figure 1. Visual representation of amounts of microplastic particles found per location/kg dry weight sediment.
Sediment samples were collected from shallow (wadable) locations using a scoop (FR) and from deeper locations with a van Veen grab (NL, BE, UK). At those deeper locations, three sediment grabs were taken from which the upper 5 cm layer of sediment was collected and pooled into one sample. Samples were collected in 1l glass jars with plastic lids and cooled (4°C). Any visible biota was removed. Upon arrival on shore, samples were frozen at −20°C until further analysis.
Samples were analyzed by the Institute for Environmental Studies (Amsterdam, the Netherlands). Sediment samples were thawed and homogenized, subsamples were taken for microplastic analysis and determination of dry weight. To extract microplastics from sediments, a modified method of Thompson et al. (2004) was followed. The sediment (25 g) was added to an Erlenmeyer with MilliQ water and a saturated NaCl solution (1.2 kg/L). The suspension was stirred for 2 min using a Teflon stirrer at the bottom of the Erlenmeyer flask. This allowed the sample material to suspend and enabled density separation of the sediment and particle material. Post-stirring, the suspension was left for 1 h, allowing the heavier sediment particles to sink while the lighter particles start to float on the saturated salt solution. The suspension was filtered over a 0.7 μm Whatman GF/C glass filter, followed by a rinsing step with hydrogen peroxide (30%) to remove any residual organic material. Alongside each batch of samples, two blanks, and two duplicate analyses were performed. The filters were examined using light microscopy and measured the length of the particles with MicroCamLab for Microsoft. Microplastics were counted and corrected for background levels determined by the blank samples. The dry weight of the sediments was determined gravimetrically after freeze-drying a 5 g subsample of the homogenized sample until a constant weight was observed. Microplastic concentrations were expressed as number of particles per kg of dry sediment and sorted into three categories “fibers/kg DW,” “spheres/kg DW,” and “fragments/kg DW.”
Sediment organic matter or total organic carbon (TOC) on the upper layer sediment was measured using the “dichromate method” (Mebius, 1960). Carbonate content was measured on the same sediment fraction as “loss on ignition” (Dean, 1974). Grain size distribution was calculated using laser diffraction particle sizing. All samples were analyzed by means of a Malvern Mastersizer 2000G hydro version 5.40 (ISO 13320:2009)1. Grain size fractions were determined as volume percentages according to the Wentworth scale (Wentworth, 1922): clay (<4 μm), silt (4–63 μm), very fine sand (63–125 μm), fine sand (125–250 μm), medium sand (250–500 μm), coarse sand (500–1000 μm), very coarse sand (1–2 mm), and gravel (>2 mm). Throughout this study, the clay and silt fractions have been combined as clay/silt (<63 microns).
Sea Surface
Floating microplastic sampling was carried out during existing fisheries surveys in the UK Channel, North, and Celtic Sea area from January to March 2011 (Figure 5). Samples were collected from surface waters in between fisheries stations using a high-speed manta trawl with a rectangular opening 50 cm high by 15.5 cm wide, and a 4.5 m long 333 μm net with a 30 × 10 cm cylindrical collecting bag. Collection took place in winter time, when low biomass facilitated sampling, during the following three Cefas cruises: Cend3/11, Cend4/11, and Cend5/11 (Table 3). In the Atlantic Ocean the water flow is predominantly from west to east driven by the northern and southern branches of the North Atlantic Drift. In the shelf areas currents are predominately generated by tides and wind, but the main water flow is from south to north (Pollard et al., 1996). The sea state on the Beaufort Scale remained between 1 and 3 for all sample sites. Water surface samples were only collected during calm sea conditions with wave heights below 50 cm.
The sampled transects were not equidistant, but sampling periods were each 60 min long. Coordinates of start and stop positions were registered, along with the number of rotations of the flow meter inside the lower part of the mouth of the manta trawl. The area sampled was calculated firstly by calculating the distance between start and stop coordinates and secondly by using the onboard knotmeter, which takes into account the ground speed and measures the number of nautical miles traveled over a defined distance, to measure the actual length of sea surface trawled in the 60-min period. These tow lengths multiplied by the width of the trawl mouth provided the area sampled, allowing for the particle abundance per square kilometer to be calculated in two different ways. Next to this, we also calculated the total number of particles by volume sampled as indicated by the flowmeter. The lower part of the manta trawl opening was fitted with a GO environmental flowmeter (http://cce.lternet.edu/docs/data/methods/M2-1314e%20Mechanical%20flowmeter.pdf) with a standard speed rotor constant of 26,873 and 1 rotor revolution equaling 10 counts. The trawled distance in meters equals the count between rotation numbers multiplied by 26,873 divided by 999,999. Marks were made on the side of the high speed mantatrawl to visually estimate the depth of the opening during transects. The sample surface of the net is 15.5 by 50 cm but for the majority of the duration of the transects the net was only half submerged while operating as a result of the repetitive wave oscillation. Based on these observations, the net surface was calculated as 0.155 by 0.25 m. These assumptions allowed us to calculate the measured volume in cubic meters by multiplying the sample surface of the net in meters by the trawled distance obtained by the calculations above.
The manta net was rinsed from the outside with a hose to concentrate the sample in the cod end. The cod end was removed over a bucket, to prevent any spillage and the sample was transferred into a large bowl. The cod end was inverted and washed out from the outside using very little water. Leftovers were gently removed by using a long metal spoon which was rinsed into the bowl. Samples were put into a glass container and preserved in 10% formalin. A yellow waterproof label with the trawl number, date, and time was included in all containers. The lids were covered with aluminum foil and the lids labeled again with a waterproof marker from the outside of the sample container.
In the laboratory, samples were rinsed with filtered, distilled water and large floating plastic items were removed. The remaining items were separated on sieves in six size classes and stored in isopropyl alcohol. Size classes above 4.75 mm were hand picked out the sieve and the smaller fractions (>4.75) were sieved over five more sieves to retain ever smaller fractions (0.355–0.499, 0.500–0.709, 0.710–0.999, 1.00–2.79, 2.800–4.749 mm). The fractions were removed by gentle washing of the sieves and concentrated in Petri dishes. A dissecting microscope was used to sort through the remaining debris and organic material. Debris was sorted by category (plastics, non-plastics, plankton, and miscellaneous) and plastics were further categorized and counted (fragment, pellet, line, film, and foam). These size classes were then sorted and quantified into shape type (fragment, pellet, line, film, and foam). The color of each piece of plastic was also recorded (by size class) (BLACK/GRAY, BLUE/GREEN, BROWN/TAN, ORANGE/PINK/RED, TRANSPARENT/TRANSLUCENT, WHITE, YELLOW). Plastic, plankton, and plant material were weighed, then oven dried at 65°C for 24 h and weighed again. The selection of sieve sizes, plastic shapes, and color categories was based on available literature and existing studies (Moore et al., 2005, 2011; Eriksen et al., 2013b).
Data Analysis
The statistical analysis and strength of correlations in the sediment microplastic data were calculated with a 2-tailed Pearson Correlation in SPSS (version 22). We analyzed the floating microplastic data and calculated the widths of CI for different sample sizes using the R package. The graphical representation of the sediment and floating data was produced with Microsoft Excel (2010), except for the histograms which were produced in R.
Results
Sediment Samples
At all stations, apart from UK station (No. 74) and two Dutch station (No. 16 and 19), microplastic particles were found in the sediment. Both the highest and lowest number of microplastics were found in samples from Belgium, respectively at location 3 with 54 particles/kg DW sediment and location 7 with 3,146 particles/kg DW sediment. The overall average amount found across all areas was 421 particles/kg DW sediment. Remarkably, no plastic fragments, only spheres and fibers were observed at any of the locations. Furthermore, the amount of spheres/kg DW of sediment was higher on average across all stations compared to the amount of fibers.
The average amount of fibers/kg DW was the lowest (99 fibers) in the Dutch coastal sediment samples, whereas the highest average amount of fibers/kg DW was found in coastal sediment samples from Belgium (301 fibers). The sediment samples from the French coast of the English Channel had the highest amount of spheres/kg DW on average (350 spheres) while the Dutch samples had the lowest (123 spheres) amount of spheres/kg DW. In terms of the average number of total particles/kg DW, the highest amounts were found in marine sediments collected from coastal zones in Belgium (585 particles) and the lowest amounts in coastal zones from the Netherlands (222 particles). The average amounts of plastic particles/kg DW are in the same order of magnitude between the different countries, indicating that there are no marked differences between counties, however, more samples are required to obtain a clearer picture. In terms of percentage of dry weight of the sediment, samples from France had the lowest level (55%), and samples from the Netherlands had the highest level (76%). An overview of the results is given in Table 2.
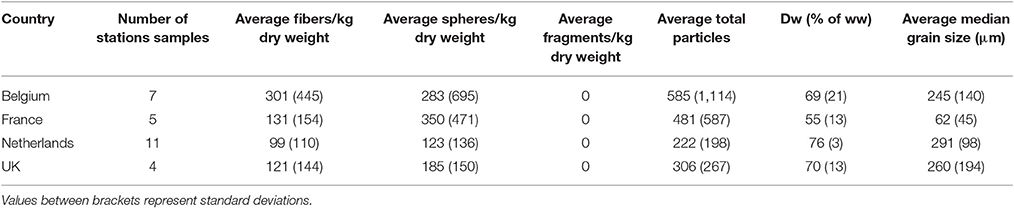
Table 2. Average amounts of microplastics found per country in terms of number of samples, average fibers/kg dry weight sediment, average spheres/kg dry weight sediment, average fragments/kg dry weight sediment, average total particles, dry weight (% of wet weight), average median grain size of the sediment.
An indication of a relationship between the percentage Total Organic Carbon (TOC) and the number of plastic particles/kg dry sediment (R2 = 0.616, p = 0.001), signifies that there are more plastic particles present with higher concentrations of TOC in the sediment (Figure 2).
In all samples, an indication of a negative relationship between the median grain size of the sediment and the number of microplastic items was found (R2 = −0.492, p = 0.009), signifying that at locations with a smaller grain size, more plastic particles can be found (Figure 3).
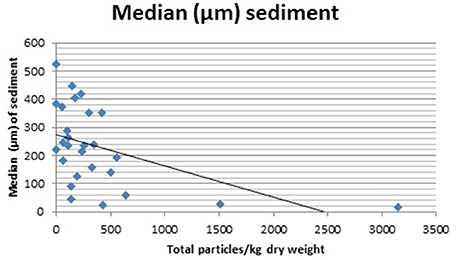
Figure 3. Median size of the sediment grains (in μm) in relation to the total amount of particles found per location/kg dry weight.
The microplastics particles make up a certain fraction in weight of the sediment. Here, the dry weight (DW) of the sediment was determined as a percentage of the wet weight. Similarly, an indication of a negative relationship can be found between DW and the total number of microplastics present (R2 = −0.796, p = 0.000), indicating that at locations with a lower DW, more plastic particles can be found (Figure 4).
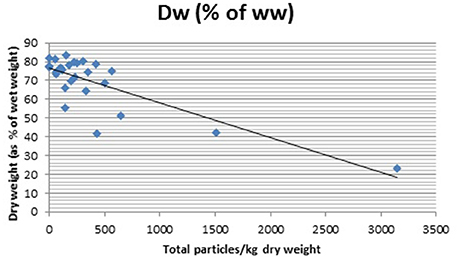
Figure 4. Dry Weight (as % of wet weight) in relation to the total amount of particles found per location/kg dry weight.
Water Samples
A total of 3,597 items were collected from 152 manta trawl transects in the Channel, North, and Celtic Sea with vessels speeds between 1.6 and 8.2 knots (Table 3). We were not able to sample the North-East part of the Channel and parts of the North Sea due to adverse weather conditions in 2011, leading to rough seas, complicating the sampling by manta trawl (Figure 5). Nevertheless, on almost all sampled locations, litter items were found, indicating a general presence of plastic items floating at the sea surface of both the North Sea and Celtic Sea.
Geographical variations in microplastic abundance at the sea surface were observed (Figure 6). The different type of distance measurements available, allowed us to calculate the number of plastic items in a few different ways. We calculated the number of items present per trawled surface area and per volume (Table 4).
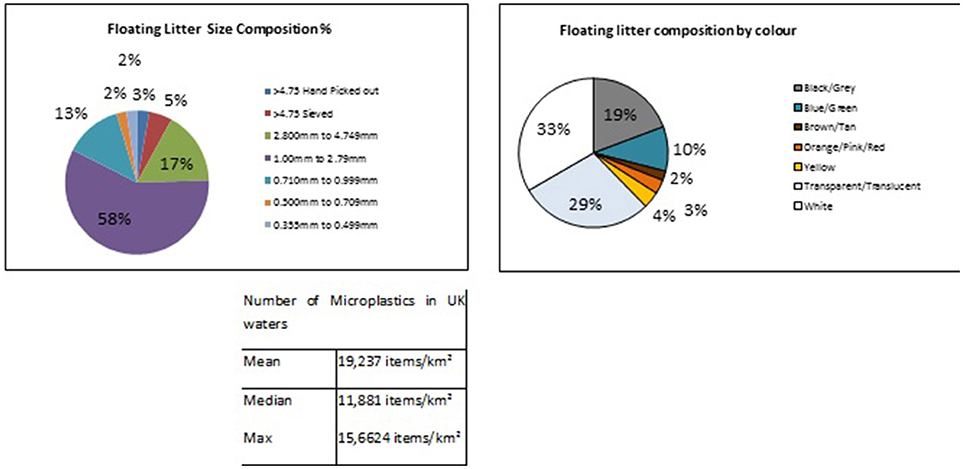
Figure 6. Floating marine litter concentrations along the European coasts, including a table with mean, median, and maximum values.
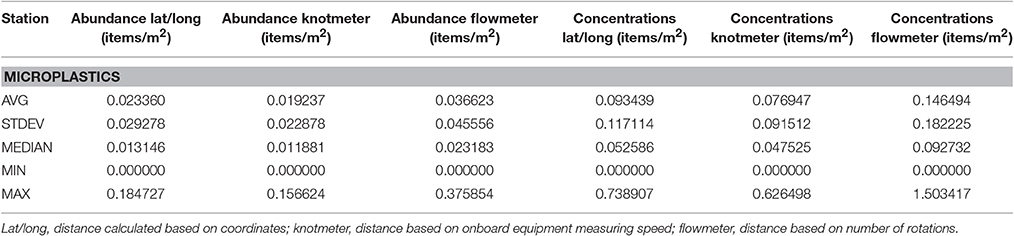
Table 4. Number of microplastics per surface area and per volume using different types of observations.
From the three applied methods to measure distance, the flowmeter results were significantly different from the others (p < 0.005). Abundance ranged from 0 to 185,000 items per km2 using the distance between coordinates, 0 to 157,000 items per km2 when using the actual distance covered by the ship and 0–376,000 items per km2 when using the distance as measured by the flow meter. Expressed as items per m3, this equals to 0–0.7 items per m3 for the coordinates method, 0–0.6 items per m3 using the knotmeter and 0–1.5 items per m3 using the flow meter readings.
The size class 1.00–2.79 mm accounted for the highest proportion of microplastics. In terms of shapes, the most abundant types found were fragments (63%), followed by thin film (14%), pellets (10%), foam (8%), line (5%). The most prominent color was white (33%), but also transparent (29%) and black (19%) The highest catch contained 283 items consisting out of 128 fragments, 28 pellets, 28 pieces of lines, 50 thin films, and 49 foamy items.
Our study did report wind data and indicates average wind speeds of 12.5 mph which only allows for a low amount of mixing (Kukulka et al., 2012). No correlation between the measured wind speed and the observed concentrations was found (R = −0.1497; Figure 7).
We have calculated the widths of the confidence intervals (CI) for different sample sizes so that the mean can be estimated with a certain precision of its value, giving a first indication of the necessary sample size for a microplastic survey at the water surface. From our 152 transects, only two returned with no microplastics. A histogram of the non-zero observations and the natural log of these values is shown in Figure 7. From this it seems reasonable to assume that the non-zero data follows an approximate lognormal distribution (i.e., that the natural log of the data is Gaussian). Thus, we modeled the data as a two-stage process. Firstly, we assumed that a proportion p (where p is estimated by 2/152 = 0.01316) of observations are zero and that the remaining data follows a lognormal distribution. N observations were simulated from this distribution and the width of the bootstrap 95% percentile confidence interval (using 1,000 replications) was calculated. The values of N were 20, 40, 60, 80, 100, 120, 140, 160, 180, 200. This whole process was repeated 500 times and a mean width was determined for each value of N. A plot of these mean widths against N is shown in the bottom left plot of Figure 7. This width represents the precision with which we have calculated the mean number of items per km2. From the original data, the original mean was 19,237 items per km2. Thus, with a sample size of n = 200, we achieve a confidence interval of width (8,000), almost 40% of this mean (Figure 8). Future monitoring programmes for microplastics at the sea surface in coastal waters of North West Europe should thus have a minimum of 200 stations so that the mean can be estimated with a precision of 40% of its value.
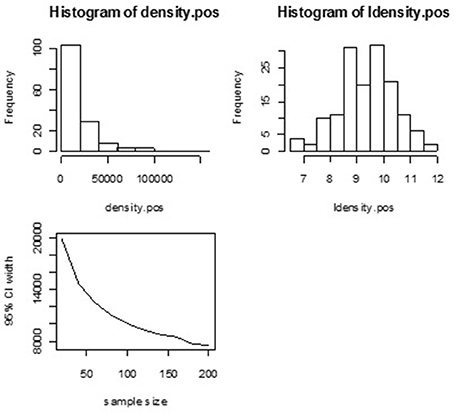
Figure 8. Histograms of non-zero and ln non-zero data (top row), confidence interval widths (bottom row).
Discussion
Microplastics in the Sediment
Microplastics particles were found in 89% of the sediments (24 out of 27) collected from locations in the North Sea and Channel area between BE, NL, FR, and the UK. No plastic fragments were found, most observed plastic particles were spheres, followed by fibers. In the sublittoral zone of the Belgian Continental Shelf, part of the North Sea, an average concentration of 97.2 microplastics particles/kg dry sediment was found (Claessens et al., 2011), lower than the findings in our study. In harbors, however, both studies found markedly higher amounts of microplastics compared with other locations (Claessens et al., 2011). The different amounts of microplastic particles reported by studies in nearby locations (Table 5) might be an indication of the heterogeneous nature of microplastics presence in marine sediments, temporal changes, and/or result from differences in the analysis (Filella, 2015), we filtered over a smaller pore size filter. Apart from the harbor station (nr. 7), results are still in the same order of magnitude and might thus give an indication for the accumulation rate of microplastics at those sites. Results from a tidal flat in Germany showed concentrations ranging between 36 and 136 microplastics per 10 g of sediment (Liebezeit and Dubaish, 2012), a result which falls within a similar range of our highest observations. In the sampled regions, however, due to the regular disturbance of the sediments by natural events such as storms (Carretero et al., 1998) and/or anthropogenic activities such as trawling and dredging (Schratzberger and Jennings, 2002; Allen and Clarke, 2007), the upper sediment layer is regularly mixed, making it difficult to link sedimentation rates with temporal microplastics accumulation (Van Cauwenberghe et al., 2013).
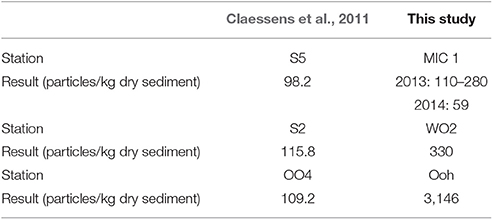
Table 5. Comparison between microplastic numbers at the same stations between Claessens et al. (2011) and the findings in this study.
There is a large spread of values around the average, indicating a heterogeneous spread of microplastics in sea floor sediments. This inhomogeneity could mean that there are areas where microplastics settle in higher amounts. In the present study, we investigated if a correlation between sediment characteristics and microplastic abundance exists. Our research indicates a relationship between the amount of organic carbon and the amount of microplastics present in the sediment. This finding is supported by a Danish study (Strand et al., 2013) who found a correlation between the content of microplastics in marine sediments and %TOC. Although further research is required, similarities in densities and resulting sedimentation processes might be driving this correlation, %TOC could help to identify potential areas with high microplastic concentrations. From our findings, it seems sensible for future monitoring to target undisturbed patches of fine sediments.
Microplastics at the Sea Surface
The ubiquity of small floating litter items in the UK Channel, North and Celtic Sea is prominently illustrated in this study by the presence of microplastics in all samples except two. The abundance of microplastics appears to be still relatively low in surface waters of the North Sea and Celtic Sea compared to other regions e.g., Pacific gyre. We observed some higher concentrations of microplastics near the coast and river estuaries. This might indicate the relative importance of inputs through rivers (Cheung et al., 2016) or could be a result of higher inputs from industrialized and populated areas nearby (Browne et al., 2011; Naidoo et al., 2015). Nevertheless, plastic particles were also commonly found at the sea surface of the North and Celtic Sea far away from land or potential sources. This could be a result of atmospheric deposition of microplastics (Dris et al., 2016). Microplastic abundance at the sea surface has been shown to vary with wind speed due to vertical mixing (Kukulka et al., 2012; Reisser et al., 2015). Data from the eastern North Pacific suggest that the abundance of suspended plastic within 10–30 m of the sea surface averages two orders of magnitude less than that of surface (Ryan et al., 2009). We found no correlation between wind speed and microplastic concentrations.
The distances measured or calculated by different techniques such as coordinates, knot meter and flow meter result in large differences in reported microplastic concentrations. Our maximum values of 157,000 particles km−2, calculated using the distance given by the onboard instrumentation, are similar to those reported on average in the Mediterranean (Collignon et al., 2012), 116,000 particles km−2 and well below those measured in the Pacific Gyre (Moore et al., 2001), who recorded densities of more than 300,000 particles km−2 in 1999. However, concentrations based on flowmeter data equaled maximum concentrations of 375,854 particles km−2. This indicates the need for standardized marine litter protocols, methodologies and units worldwide. Internationally, various techniques, and principles have been applied to sample and analyse floating microplastics (Filella, 2015). Consequently, available studies have been reporting marine litter abundance in diverse dimensions and scales, making direct comparisons extremely difficult, e.g., the number of microplastics by volume (particles/m3) or by surface area (particles/km2), smaller or bigger than 5 mm, analyzed with microscopes or spectroscopes (Galgani et al., 2015).
We showed above that even within the same study, several ways of expressing microplastic quantities can be used depending on the initial calculation of trawled distance. Only using coordinates could easily lead to errors as it doesn't consider ocean currents and factual sampling distance. When available, using onboard instruments to precisely measure the vessels groundspeed while sampling gives a more accurate estimate of the trawled distance. The flow meter determines the distance based on the water flow through the net. However, there were significant differences between the first two methods and the flowmeter method. The flow meter registered a smaller distance than what was obtained by using coordinates or onboard instrumentation. This could be due to the bow wave effect which has been previously observed when trawling nets at high speeds or a result of the chopping through waves (Chiang et al., 2011), meaning that a far lower volume will be filtered by the manta net compared to what one could calculate from less direct measurements such as coordinates and ship speed. Microplastics are vertically distributed within the upper water column due to wind and temperature driven mixing (Kukulka et al., 2012, 2016). This suggests that microplastic concentrations could be significantly underestimated by traditional surface measurements. To allow for comparison, it is therefore recommended to sample in comparable conditions of calm sea state with low wind and wave intensity. The authors also propose to use flow meters and to report both units, items per km2 and items per m3, in future microplastic studies at the sea surface.
We listed microplastic concentrations from within the same geographical area, using comparable equipment for sampling microplastics (Table 6). Our average value, 0.14 items per m3 and maximum value of 1.5 items per m3, based on the flow meter data, is comparable to previous microplastic studies with manta nets in this region.
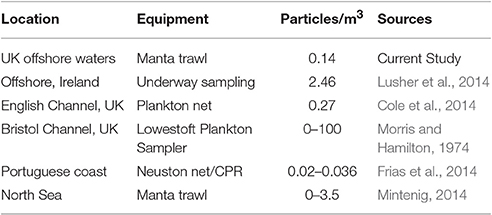
Table 6. Comparison of the current study results with results from research in the same region (Morris and Hamilton, 1974; Lusher et al., 2013; Cole et al., 2014; Frias et al., 2014; Mintenig, 2014).
Comparison Water and Sediment Matrices
Our results indicated that sediments were more contaminated with microplastics, by number of items per volume, than surface waters. The transport of small particles to the seafloor and their deposition in the benthic sediments is facilitated by the colonization of the material by fouling organisms, which increase the density of the particles and force them to sink (Andrady, 2015). Plastics degrade very slowly resulting in high persistence of plastic litter especially at the seafloor (Andrady, 2015). Several microplastics of a few micron were found in marine sediments with a rapid-screening approach based on fluorescent tagging with Nile Red, highlighting the role of marine sediments as a sink (Maes et al., 2017). In our study, most of the microplastics found in the sediments were fibers and spheres, with spheres having the highest average amount/kg sediment. This is in contrast with the findings from the floating microplastics were mainly fragments were found. It seems that for the floating microplastic particles there is a potential influence from rivers. Rivers are both pathways and producers of microplastics (Thiel et al., 2011; Rech et al., 2014). A study on microplastics in European rivers indeed found that fragments (Po and Rhine) and fibers (Danube and Dalålven) were the largest part of the microplastics found (van der Wal et al., 2015). Plastic fragments are breakdown products of larger plastic items via mechanical and/or UV-weathering (Barnes et al., 2009), which occurs when exposed to the sun, wind and other mechanical stresses such as found in a river. Similarly, we observed thousands of fragments in the floating fraction in our study.
The fact that there are mostly spheres and fibers found in the sediments is not so surprising, many spheres and fibers are made from polystyrene and polyacrylamides which are often heavier then seawater and thus readily sink (Cheung and Fok, 2016). The shape of the particle and fiber could influence its settling velocity, however, few studies have been published on this topic. The high amounts of fibers in the sediments, could be a result of the degradation of fishing nets and dolly rope while dragging over the seafloor (Devriese et al., 2015), from the continuous input via sewage and laundry (Browne et al., 2011) or via the disposal of sewage sludge and dredged sediments (Browne et al., 2011). Also Brown shrimp, collected from the same study area, contained mainly fibers (Devriese et al., 2015). It is much more challenging to define the main sources of microplastics in sediment due to the wide variety of potential pathways (Andrady, 2015), including atmospheric depositions (Dris et al., 2016).
Due to wind and currents, floating microplastics are more mobile compared to those found in sediments (Kukulka et al., 2012), which act as a stable sink (Woodall et al., 2014). In this study, microplastic concentrations in different surface transects varied between a few tens to a few thousands. Due to this variability, large sample sizes, above 200 stations, are required to ensure that the mean can be estimated with a precision of 40% of its value. North West European seas in the North-East Atlantic are periodically impacted by geologically significant storms, which have a marked influence on water circulation but also affect terrigenous sediment supply, flood deposition, and long-term accumulation of fine-grained sediment on the continental shelf (Green et al., 1995). Also, fisheries activities disturb the sediment and homogenize the upper sediment layers by trawling (Schratzberger and Jennings, 2002). So far, there are no studies considering the impact of these physical processes on microplastic distribution in water or sediment samples. Our results indicated that the number of microplastics in sediment samples were less variable, especially at locations with high %TOC, in comparison to those found at the sea surface. To look at temporal trends, it seems sensible for future monitoring to target undisturbed patches of fine sediments with high %TOC. Such monitoring could be combined with the monitoring of hazardous substances, since these surveys are well established and targeting fine sediments (<64 microns) to monitor persistent organic pollutants (Davies et al., 2012).
Monitoring of Microplastics
This study is one of the first to determine baseline values for microplastics in North West European seas. Based on our findings, we see a potential for microplastics monitoring in combination with existing environmental surveys. Standardized methods resulted in a comparable outcome between the project partners of the Interreg 2 Seas MICRO IVa project (van der Meulen et al., 2015). The standardization of methods for collecting, processing, and analysis of samples is required to achieve comparable outcomes within one region. When counting microplastics, different types of equipment like regular microscopy or spectroscopy can be applied, causing under or over estimations which possibly influence the final numbers (Löder and Gerdts, 2015). To monitor and compare spatial and temporal trends of microplastics, simple, cost-effective and standardized protocols, capable of efficiently and accurately sampling, and enumerating microplastics in a variety of environmental matrices are recommended (Maes et al., 2017; Underwood et al., 2017). Without this it will remain impossible to make direct comparisons among studies and habitats, because such comparisons could be confounded with methods used (Underwood et al., 2017).
The results of this study demonstrate that microplastics were present at the sea surface and in sediments of the UK Channel, North Sea and Celtic Sea. Different shapes and types of plastics were found in both matrices. Monitoring of both matrices had certain advantages and disadvantages which must be considered when designing future monitoring programmes. Microplastic monitoring in sediments can easily be combined with existing contaminant surveys sampling fine sediments. Water column and sea surface monitoring might be more appropriate for determining effect concentrations for certain marine biota. Because the concentrations of microplastics in the water are lower and the variability is higher than in sediments, more water must be sampled to achieve a comparable sample size to sediments or other seafloor indicators. We recommend to install a flow meter near the lower edge of the manta net frame to give additional information on the number of items per cubic meter.
Areas with high concentrations of floating microplastics were found in the estuarine and coastal areas. For the sediment, we observed high concentrations of microplastics in estuarine areas and in organic sediments, supported by the correlation with high total organic carbon content. Hotspot areas are thus likely situated in areas with fine muds since these generally contain high concentrations of organic materials and are made up of smaller grain sizes. The settling of microplastics might be following similar sedimentation processes as those observed in fine sediments. A previous study of microplastics in the deep sea suggested that aggregation of microplastics with organic matter, such as marine snow and fecal pellets of marine organisms, could play a role in the sinking processes (Van Cauwenberghe et al., 2013). This also indicates that benthic organisms burrowing and feeding in muddy environments, are likely exposed to higher concentrations of microplastics than benthic organisms in areas with a larger grain size and lower TOC. Pooled sampling, repeated over time, is advisable to determine trends while minimizing spatial heterogeneity. Determination of sediment characteristics will enlarge our understanding of underlying sedimentation processes and could help with the identification of potential microplastic hotspots. We suggest that future programs of monitoring continue to distinguish the type of microplastic particles as well as the sampled size fractions, and we advise to monitor microplastics in sediments with standard mesh sizes and equipment such as the van Veen grab to allow future spatio-temporal comparison of microplastic abundance across wider marine environments.
Conclusions
This study presents a baseline for the monitoring of microplastic in coastal sediments and surface waters of North West European seas. Floating concentrations ranged between zero and 1.5 microplastic per m3, whereas microplastic concrentrations in sediments ranged between zero and a few thousands per kg dw sediment. In sediments, mainly fibers and spheres were found, whereas at the sea surface fragments were dominant. For the water phase concentrations of microplastics are lower and more variable than in sediments, meaning that larger sampled water volumes are required to find detectable concentrations. Future monitoring programmes for microplastics at the sea surface in coastal waters of North West Europe should have a minimum of 200 stations to estimate the mean with a precision of 40% of its value. Standardization of monitoring methods within OSPAR and EU is recommended to aid in the implementation of the MSFD and the assessment of the microplastics pollution of Northern European waters over time. High concentrations of microplastics in the water can be found in estuaries. For sediments, estuaries and areas with a high organic carbon content are likely hotspots.
Author Contributions
TM: sediment and water sampling/analysis, and manuscript writing. MV: sediment sampling and manuscript writing. LD, AH, and LF: sediment sampling. HL: sediment analysis. JR: project administration. AV: sediment sampling, review and guidance.
Conflict of Interest Statement
The authors declare that the research was conducted in the absence of any commercial or financial relationships that could be construed as a potential conflict of interest.
Acknowledgments
Support for this research came from the EU Interreg 2 Seas IVa project MICRO (MICRO 09-002-BE). This study was partly funded by Defra (UK) and the Dutch Ministry of Infrastructure and the Environment (NL). Shiptime on RV Simon Stevin was provided by VLIZ and DAB Vloot. Some floating litter data was collected on board of the RV Cefas Endeavour and funded by Defra (UK) project ME5415. Many thanks to Manuel Nicolaus (Cefas), Jon Barry (Cefas), and all researchers involved in the project, as well as the VU University Institute for Environmental Studies, the Cefas Plankton lab, and ILVO lab of chromatography.
Footnotes
References
Allen, J. I., and Clarke, K. R. (2007). Effects of demersal trawling on ecosystem functioning in the North Sea: a modelling study. Mar. Ecol. Prog. Ser. 336, 63–75. doi: 10.3354/meps336063
Andrady, A. L. (2015). “Persistence of plastic litter in the oceans,” in Marine Anthropogenic Litter, eds M. Bergmann, L. Gutow, and M. Klages (Cham: Springer International Publishing), 57–72. doi: 10.1007/978-3-319-16510-3_3
Arthur, C., Baker, J., and Bamford, H. (eds.). (2009). Proceedings of the International Research Workshop on the Occurrence, Effects, and Fate of Microplastic Marine Debris. NOAA Technical Memorandum NOS-OR&R-30. Available online at: https://marinedebris.noaa.gov/file/2192/download?token=5dvqb-YY
Barnes, D. K. A., Galgani, F., Thompson, R. C., and Barlaz, M. (2009). Accumulation and fragmentation of plastic debris in global environments. Philos. Trans. R. Soc. Lond. B Biol. Sci. 364, 1985–1998. doi: 10.1098/rstb.2008.0205
Browne, M. A., Crump, P., Niven, S. J., Teuten, E., Tonkin, A., Galloway, T., et al. (2011). Accumulation of microplastic on shorelines woldwide: sources and sinks. Environ. Sci. Technol. 45, 9175–9179. doi: 10.1021/es201811s
Carretero, J. C., Gomez, M., Lozano, I., De Elvira, A. R., Serrano, O., Iden, K., et al. (1998). Changing waves and storms in the Northeast Atlantic? Bull. Am. Meteorol. Soc. 79, 741–760. doi: 10.1175/1520-0477(1998)079<0741:CWASIT>2.0.CO;2
Cheung, P. K., and Fok, L. (2016). Evidence of microbeads from personal care product contaminating the sea. Mar. Pollut. Bull. 109, 582–585. doi: 10.1016/j.marpolbul.2016.05.046
Cheung, P. K., Cheung, L. T. O., and Fok, L. (2016). Seasonal variation in the abundance of marine plastic debris in the estuary of a subtropical macro-scale drainage basin in South China. Sci. Total Environ. 562, 658–665. doi: 10.1016/j.scitotenv.2016.04.048
Chiang, K.-P., Wu, C.-J., and Shin, C.-M. (2011). Does the mesh size of the plankton net affect the result of statistical analyses of the relationship between the copepod community and water masses? Crustaceana 84, 1069–1083. doi: 10.1163/001121611X586701
Claessens, M., De Meester, S., Van Landuyt, L., De Clerck, K., and Janssen, C. R. (2011). Occurrence and distribution of microplastics in marine sediments along the Belgian coast. Mar. Pollut. Bull. 62, 2199–2204. doi: 10.1016/j.marpolbul.2011.06.030
Cole, M., Lindeque, P., Halsband, C., and Galloway, T. S. (2011). Microplastics as contaminants in the marine environment: A review. Mar. Pollut. Bull. 62, 2588–2597. doi: 10.1016/j.marpolbul.2011.09.025
Cole, M., Webb, H., Lindeque, P. K., Fileman, E. S., Halsband, C., and Galloway, T. S. (2014). Isolation of microplastics in biota-rich seawater samples and marine organisms. Sci. Rep. 4:4528. doi: 10.1038/srep04528
Collignon, A., Hecq, J. H., Glagani, F., Voisin, P., Collard, F., and Goffart, A. (2012). Neustonic microplastic and zooplankton in the North Western Mediterranean Sea. Mar. Pollut. Bull. 64, 861–864. doi: 10.1016/j.marpolbul.2012.01.011
Cózar, A., Echevarría, F., González-Gordillo, J. I., Irigoien, X., Ubeda, B., Hernández-León, S., et al. (2014). Plastic debris in the open ocean. Proc. Natl. Acad. Sci. U.S.A. 111, 10239–10244. doi: 10.1073/pnas.1314705111
Davies, I. M., Gubbins, M., Hylland, K., Maes, T., Martinez-Gomez, C., Moffat, C., et al. (2012). Guidelines for the Integrated Monitoring and Assessment of Contaminants and Their Effects. Available online at: https://research.vu.nl/en/publications/guidelines-for-the-integrated-monitoring-and-assessment-of-contam (Accessed April 7, 2017).
Dean, W. E. J. (1974). Determination of carbonate and organic matter in calcareous sediments and sedimentary rocks by loss on ignition: comparison with other methods. J. Sediment. Petrol. 44, 242–248.
Derraik, J. G. B. (2002). The pollution of the marine environment by plastic debris. Mar. Pollut. Bull. 44, 842–852. doi: 10.1016/S0025-326X(02)00220-5
Devriese, L. I., van der Meulen, M. D., Maes, T., Bekaert, K., Paul-Pont, I., Frère, L., et al. (2015). Microplastic contamination in brown shrimp (Crangon crangon, Linnaeus 1758) from coastal waters of the Southern North Sea and Channel area. Mar. Pollut. Bull. 98, 179–187. doi: 10.1016/j.marpolbul.2015.06.051
Dris, R., Gasperi, J., Saad, M., Mirande, C., and Tassin, B. (2016). Synthetic fibers in atmospheric fallout: a source of microplastics in the environment? Mar. Pollut. Bull. 104, 290–293. doi: 10.1016/j.marpolbul.2016.01.006
Eriksen, M., Lebreton, L. C. M., Carson, H. S., Thiel, M., Moore, C. J., Borerro, J. C., et al. (2014). Plastic pollution in the world's oceans: more than 5 trillion plastic pieces weighing over 250,000 tons afloat at sea. PLoS ONE 9:e111913. doi: 10.1371/journal.pone.0111913
Eriksen, M., Mason, S., Wilson, S., Box, C., Zellers, A., Edwards, W., et al. (2013a). Microplastic pollution in the surface waters of the Laurentian Great Lakes. Mar. Pollut. Bull. 77, 177–182. doi: 10.1016/j.marpolbul.2013.10.007
Eriksen, M., Maximenko, N., Thiel, M., Cummins, A., Lattin, G., Wilson, S., et al. (2013b). Plastic pollution in the South Pacific subtropical gyre. Mar. Pollut. Bull. 68, 71–76. doi: 10.1016/j.marpolbul.2012.12.021
Fendall, L. S., and Sewell, M. A. (2009). Contributing to marine pollution by washing your face: microplastics in facial cleansers. Mar. Pollut. Bull. 58, 1225–1228. doi: 10.1016/j.marpolbul.2009.04.025
Filella, M. (2015). Questions of size and numbers in environmental research on microplastics: methodological and conceptual aspects. Environ. Chem. 12, 527–538. doi: 10.1071/EN15012
Foekema, E. M., De Gruijter, C., Mergia, M. T., van Franeker, J. A., Murk, A. J., and Koelmans, A. A. (2013). Plastic in north sea fish. Environ. Sci. Technol. 47, 8818–8824. doi: 10.1021/es400931b
Frias, J. P. G. L., Otero, V., and Sobral, P. (2014). Evidence of microplastics in samples of zooplankton from Portuguese coastal waters. Mar. Environ. Res. 95, 89–95. doi: 10.1016/j.marenvres.2014.01.001
Gago, J., Galgani, F., Maes, T., and Thompson, R. C. (2016). Microplastics in Seawater: recommendations from the marine strategy framework directive implementation process. Front. Mar. Sci. 3:219. doi: 10.3389/fmars.2016.00219
Galgani, F., Hanke, G., and Maes, T. (2015). “Global distribution, composition and abundance of marine litter,” in Marine Anthropogenic Litter, eds M. Bergmann, L. Gutow, and M. Klages (London: Springer), 29–56.
Galgani, F., Hanke, G., Werner, S., and De Vrees, L. (2013). Marine litter within the European marine strategy framework directive. ICES J. Mar. Sci. 70, 1055–1064. doi: 10.1093/icesjms/fst122
Galgani, F., Hanke, G., Werner, S., Oosterbaan, L., Nilsson, P., Fleet, D., et al. (2014). Guidance on Monitoring of Marine Litter in European Seas. JRC, 1–128. Available online at: http://publications.jrc.ec.europa.eu/repository/bitstream/JRC83985/lb-na-26113-en-n.pdf
GESAMP (2015). Sources, Fate and Effects of Microplastics in the Marine Environment: A Global Assessment. Available online at: http://www.gesamp.org/data/gesamp/files/media/Publications/Reports_and_studies_90/gallery_2230/object_2500_large.pdf
Green, M. O., Vincent, C. E., McCave, I. N., Dickson, R. R., Rees, J. M., and Pearsons, N. D. (1995). Storm sediment transport: observations from the British North Sea shelf. Cont. Shelf Res. 15, 889–912. doi: 10.1016/0278-4343(95)80001-T
Gregory, M. R. (2009). Environmental implications of plastic debris in marine settings–entanglement, ingestion, smothering, hangers-on, hitch-hiking and alien invasions. Philos. Trans. R. Soc. Lond. B Biol. Sci. 364, 2013–2025. doi: 10.1098/rstb.2008.0265
Jambeck, J. R., Geyer, R., Wilcox, C., Siegler, T. R., Perryman, M., Andrady, A., et al. (2015). Plastic waste inputs from land into the ocean. Science 347, 768–770. doi: 10.1126/science.1260352
Keswani, A., Oliver, D. M., Gutierrez, T., and Quilliam, R. S. (2016). Microbial hitchhikers on marine plastic debris: human exposure risks at bathing waters and beach environments. Mar. Environ. Res. 118, 10–19. doi: 10.1016/j.marenvres.2016.04.006
Kukulka, T., Law, K. L., and Proskurowski, G. (2016). Evidence for the influence of surface heat fluxes on turbulent mixing of microplastic marine debris. J. Phys. Oceanogr. 46, 809–815. doi: 10.1175/jpo-d-15-0242.1
Kukulka, T., Proskurowski, G., Morét-Ferguson, S., Meyer, D. W., and Law, K. L. (2012). The effect of wind mixing on the vertical distribution of buoyant plastic debris. Geophys. Res. Lett. 39:L07601. doi: 10.1029/2012gl051116
Lechner, A., Keckeis, H., Lumesberger-Loisl, F., Zens, B., Krusch, R., Tritthart, M., et al. (2014). The Danube so colourful: a potpourri of plastic litter outnumbers fish larvae in Europe's second largest river. Environ. Pollut. 188, 177–181. doi: 10.1016/j.envpol.2014.02.006
Leslie, H. A., van der Meulen, M. D., Kleissen, F. M., and Vethaak, A. D. (2011). Microplastic Litter in the Dutch Marine Environment Providing Facts and Analysis for with Marine Microplastic Litter. Deltares, 104.
Liebezeit, G., and Dubaish, F. (2012). Microplastics in beaches of the East Frisian Islands Spiekeroog and Kachelotplate. Bull. Environ. Contam. Toxicol. 89, 213–217. doi: 10.1007/s00128-012-0642-7
Lima, A. R. A., Costa, M. F., and Barletta, M. (2014). Distribution patterns of microplastics within the plankton of a tropical estuary. Environ. Res. 132, 146–155. doi: 10.1016/j.envres.2014.03.031
Löder, M. G. J., and Gerdts, G. (2015). “Methodology used for the detection and identification of microplastics—a critical appraisal,” in Marine Anthropogenic Litter, eds M. Bergmann, L. Gutow, and M. Klages (London: Springer), 201–227.
Lusher, A. L., Burke, A., O'Connor, I., and Officer, R. (2014). Microplastic pollution in the Northeast Atlantic Ocean: validated and opportunistic sampling. Mar. Pollut. Bull. 88, 325–333. doi: 10.1016/j.marpolbul.2014.08.023
Lusher, A. L., McHugh, M., and Thompson, R. C. (2013). Occurrence of microplastics in the gastrointestinal tract of pelagic and demersal fish from the English Channel. Mar. Pollut. Bull. 67, 94–99. doi: 10.1016/j.marpolbul.2012.11.028
Maes, T., Jessop, R., Wellner, N., Haupt, K., and Mayes, A. G. (2017). A rapid-screening approach to detect and quantify microplastics based on fluorescent tagging with Nile Red. Sci. Rep. 7:44501. doi: 10.1038/srep44501
McCormick, A., Hoellein, T. J., Mason, S. A., Schluep, J., and Kelly, J. J. (2014). Microplastic is an abundant and distinct microbial habitat in an urban river. Environ. Sci. Technol. 48, 11863–11871. doi: 10.1021/es503610r
Mebius, L. J. (1960). A rapid method for the determination of organic carbon in soil. Anal. Chim. Acta 22, 120–124. doi: 10.1016/S0003-2670(00)88254-9
Mintenig, S. (2014). Microplastic in Plankton of the North- and Baltic Sea. Epic. Available online at: http://epic.awi.de/36907/#.WOgwPNsXQHk.mendeley&title=MicroplasticinplanktonoftheNorth-andBalticSea-ePIC (Accessed April 8, 2017).
Moore, C. J., Lattin, G. L., and Zellers, A. F. (2011). Quantity and type of plastic debris flowing from two urban rivers to coastal waters and beaches of Southern California. Rev. Gestão Costeira Integr. 11, 65–73. doi: 10.5894/rgci194
Moore, C. J., Moore, S. L., Leecaster, M. K., and Weisberg, S. B. (2001). A comparison of plastic and plankton in the north Pacific central gyre. Mar. Pollut. Bull. 42, 1297–1300. doi: 10.1016/S0025-326X(01)00114-X
Moore, C., Lattin, G., and Zellers, A. (2005). Density of Plastic Particles Found in Zooplankton Trawls from Coastal Waters of California to the North Pacific Central Gyre. …, Redon. Beach, CA. Available online at: http://alguita.com/pdf/Density-of-Particles.pdf
Morét-Ferguson, S., Law, K. L., Proskurowski, G., Murphy, E. K., Peacock, E. E., and Reddy, C. M. (2010). The size, mass, and composition of plastic debris in the western North Atlantic Ocean. Mar. Pollut. Bull. 60, 1873–1878. doi: 10.1016/j.marpolbul.2010.07.020
Morishige, C., Donohue, M. J., Flint, E., Swenson, C., and Woolaway, C. (2007). Factors affecting marine debris deposition at French Frigate Shoals, Northwestern Hawaiian Islands Marine National Monument, 1990-2006. Mar. Pollut. Bull. 54, 1162–1169. doi: 10.1016/j.marpolbul.2007.04.014
Morris, A. W., and Hamilton, E. I. (1974). Polystyrene spherules in the Bristol Channel. Mar. Pollut. Bull. 5, 26–27. doi: 10.1016/0025-326X(74)90311-7
Naidoo, T., Glassom, D., and Smit, A. J. (2015). Plastic pollution in five urban estuaries of KwaZulu-Natal, South Africa. Mar. Pollut. Bull. 101, 473–480. doi: 10.1016/j.marpolbul.2015.09.044
Pollard, R. T., Grifftths, M. J., Cunningham, S. A., Read, J. F., Pérez, F. F., and Ríos, A. F. (1996). Vivaldi 1991–A study of the formation, circulation and ventilation of Eastern North Atlantic Central Water. Prog. Oceanogr. 37, 167–192. doi: 10.1016/S0079-6611(96)00008-0
Rech, S., Macaya-Caquilpán, V., Pantoja, J. F., Rivadeneira, M. M., Jofre Madariaga, D., and Thiel, M. (2014). Rivers as a source of marine litter–a study from the SE Pacific. Mar. Pollut. Bull. 82, 66–75. doi: 10.1016/j.marpolbul.2014.03.019
Reisser, J., Slat, B., Noble, K., Du Plessis, K., Epp, M., Proietti, M., et al. (2015). The vertical distribution of buoyant plastics at sea: an observational study in the North Atlantic Gyre. Biogeosciences 12, 1249–1256. doi: 10.5194/bg-12-1249-2015
Rios Mendoza, L. M., and Jones, P. R. (2015). Characterisation of microplastics and toxic chemicals extracted from microplastic samples from the North Pacific Gyre. Environ. Chem. 12, 611–617. doi: 10.1071/EN14236
Ryan, P. G., Moore, C. J., van Franeker, J. A., and Moloney, C. L. (2009). Monitoring the abundance of plastic debris in the marine environment. Philos. Trans. R. Soc. Lond. B Biol. Sci. 364, 1999–2012. doi: 10.1098/rstb.2008.0207
Schratzberger, M., and Jennings, S. (2002). Impacts of chronic trawling disturbance on meiofaunal communities. Mar. Biol. 141, 991–1000. doi: 10.1007/s00227-002-0895-5
Strand, J., Lassen, P., Shashoua, Y., and Andersen, J. (2013). “Microplastic particles in sediments from Danish waters,” in ICES Annual Science Conference (ASC). Available online at: http://www.forskningsdatabasen.dk/en/catalog/2282206747 (Accessed January 31, 2017).
Sundt, P., Schultze, P.-E., and Syversen, F. (2014). Sources of Microplastic Pollution to the Marine Environment. Mepex Norwegian Environment Agency. 1–108. Report number: M-321/2015. Available online at: http://www.miljodirektoratet.no/Documents/publikasjoner/M321/M321.pdf
Thiel, M., Hinojosa, I. A., Joschko, T., and Gutow, L. (2011). Spatio-temporal distribution of floating objects in the German Bight (North Sea). J. Sea Res. 65, 368–379. doi: 10.1016/j.seares.2011.03.002
Thompson, R. C., Olsen, Y., Mitchell, R. P., Davis, A., Rowland, S. J., John, A. W., et al. (2004). Lost at sea: where is all the plastic? Science 304, 838. doi: 10.1126/science.1094559
Underwood, A. J., Chapman, M. G., Browne, M. A., Hobbs, G., Lednev, I. K., Kurtz, S. M., et al. (2017). Some problems and practicalities in design and interpretation of samples of microplastic waste. Anal. Methods 9, 1332–1345. doi: 10.1039/C6AY02641A
Van Cauwenberghe, L., Vanreusel, A., Mees, J., and Janssen, C. R. (2013). Microplastic pollution in deep-sea sediments. Environ. Pollut. 182, 495–499. doi: 10.1016/j.envpol.2013.08.013
van der Meulen, M. D., Devriese, L. I., Maes, T., Van Dalfsen, J. A., Huvet, A., Soudant, P., et al. (2015). Socio-Economic Impact of Microplastics in the 2 Seas, Channel and France Manche Region. Deltares. Available online at: http://archimer.ifremer.fr/doc/00287/39834/38359.pdf
van der Wal, M., Van der Meulen, M., Tweehuijsen, G., Peterlin, M., Palatinus, A., Kovač Viršek, M., et al. (2015). Identification and Assessment of Riverine Input of (Marine) Litter. JRC. Available online at: http://mcc.jrc.ec.europa.eu/document.py?code=201606244356&title=Final%20Report%20on%20Identification%20and%20Assessment%20of%20Riverine%20Input%20of%20(Marine)%20Litter
van Sebille, E., Wilcox, C., Lebreton, L., Maximenko, N., Hardesty, B. D., van Franeker, J. A., et al. (2015). A global inventory of small floating plastic debris. Environ. Res. Lett. 10:124006. doi: 10.1088/1748-9326/10/12/124006
Vethaak, A. D., and Leslie, H. A. (2016). Plastic Debris is a human health issue. Environ. Sci. Technol. 50, 6825–6826. doi: 10.1021/acs.est.6b02569
Wentworth, C. K. (1922). A scale of grade and class terms for clastic sediments. J. Geol. 30, 377–392. doi: 10.1086/622910
Woodall, L. C., Sanchez-Vidal, A., Canals, M., Paterson, G. L. J., Coppock, R., Sleight, V., et al. (2014). The deep sea is a major sink for microplastic debris. R. Soc. Open Sci. 1, 140317–140317. doi: 10.1098/rsos.140317
Keywords: microplastics, marine litter, floating debris, sediment, Marine Strategy Framework Directive (MSFD), baseline
Citation: Maes T, Van der Meulen MD, Devriese LI, Leslie HA, Huvet A, Frère L, Robbens J and Vethaak AD (2017) Microplastics Baseline Surveys at the Water Surface and in Sediments of the North-East Atlantic. Front. Mar. Sci. 4:135. doi: 10.3389/fmars.2017.00135
Received: 01 February 2017; Accepted: 24 April 2017;
Published: 26 May 2017.
Edited by:
Julia Reisser, The Ocean Cleanup, NetherlandsReviewed by:
Christos Ioakeimidis, United Nations Environment Programme Mediterranean Action Plan (UNEP/MAP), GreeceAndré Ricardo de Araújo Lima, Federal University of Pernambuco, Brazil
Copyright © 2017 Maes, Van der Meulen, Devriese, Leslie, Huvet, Frère, Robbens and Vethaak. This is an open-access article distributed under the terms of the Creative Commons Attribution License (CC BY). The use, distribution or reproduction in other forums is permitted, provided the original author(s) or licensor are credited and that the original publication in this journal is cited, in accordance with accepted academic practice. No use, distribution or reproduction is permitted which does not comply with these terms.
*Correspondence: Thomas Maes, thomas.maes@cefas.co.uk