- 1Department of Environmental Science and Policy, George Mason University, Fairfax, VA, USA
- 2Department of Paleobiology, National Museum of Natural History, Smithsonian Institution, Washington, DC, USA
- 3Departments of Mammalogy and Paleontology, Burke Museum of Natural History and Culture, Seattle, WA, USA
- 4College of Extended Education, California State University, Seaside, CA, USA
Baleen whales, or mysticetes, include the largest vertebrates to have ever evolved. Their gigantism, evolutionary success, and ecological diversity have been linked to filter feeding. Mysticetes filter feed using elaborate keratinous baleen plates, which grow from the palate and allow them to strain large quantities of prey out of the water. While the earliest mysticetes retained the adult, mineralized teeth present in ancestral whales, all species of living baleen whales lack teeth and instead possess baleen. The mechanism by which this evolutionary transformation took place remains unknown. We present four independent, but non-exclusive hypotheses for the origin of baleen. We evaluate the support for these hypotheses based on separate lines of evidence, including paleontological, molecular, and ontogenetic data. We suggest that the origin of baleen is decoupled from the loss of teeth, with a separate morphological and genetic basis. Moreover, we outline how new fossils and phylogenetic analyses may resolve current debates about morphological transformations in tooth loss and baleen origin across the phylogeny of stem and crown Mysticeti. Additional insights will likely arise from more detailed examination of developmental and biomechanical data, with sufficient ontogenetic and phylogenetic sampling.
Introduction
Baleen whales (crown Mysticeti) are a clade of cetaceans that have evolved to include the largest vertebrates in history. Their gigantism, evolutionary success, and ecological diversity have been linked to their ability to filter feed. Mysticetes filter feed using elaborate keratinous baleen plates, which grow from the palate, effectively filtering prey from large volumes of engulfed water (Werth, 2000; Goldbogen, 2010). All extant Mysticeti possess baleen and they are born entirely edentulous, despite being descended from ancestors that possessed teeth (Uhen, 2010). The presence of rudimentary teeth in extant mysticete fetuses (Saint-Hilaire, 1807; Karlsen, 1962) reflects this ancestry, but the developmental mechanisms responsible for tooth loss in utero remain obscure. Molecular data suggests that a single transformation to toothless adults occurred in the common ancestor of all living mysticetes (Meredith et al., 2011). This argument is broadly supported by new fossil discoveries of stem Mysticeti, although the increasing diversity of toothed and toothless states in new stem taxa points to a more complex transition from raptorial feeding to filter feeding than suggested by step-wise models (Deméré et al., 2008).
We here consolidate previous and new work into four independent, but non-exclusive hypotheses for the origin of baleen (Figure 1). The first (dental filtration hypothesis) suggests that filter feeding evolved first using elaborate dental cusps analogous to extant crabeater seals (Lobodon carcinophagus; Fordyce, 1989; Mitchell, 1989; Ichishima, 2005). The second (medial baleen hypothesis) suggests that incipient baleen evolved medial to a functional dental row (Deméré and Berta, 2008; Deméré et al., 2008; Ekdale et al., 2015). The third (posterior baleen hypothesis) suggests that functional baleen evolved posterior to vestigial adult teeth retained at the distal tip of the rostrum and dentaries, with the dentition and baleen aligned in the rostrum (Boessenecker and Fordyce, 2015a,b). A fourth (suction feeding hypothesis) proposes suction feeding as the ancestral condition, suggesting a transition first from raptorial feeding to suction feeding and then subsequently to bulk filter feeding (Marx et al., 2015, 2016b). We elaborate on this idea to raise this latter possibility to suggest that a transitional step in stem mysticetes included taxa bearing neither teeth nor baleen (Figure 1).
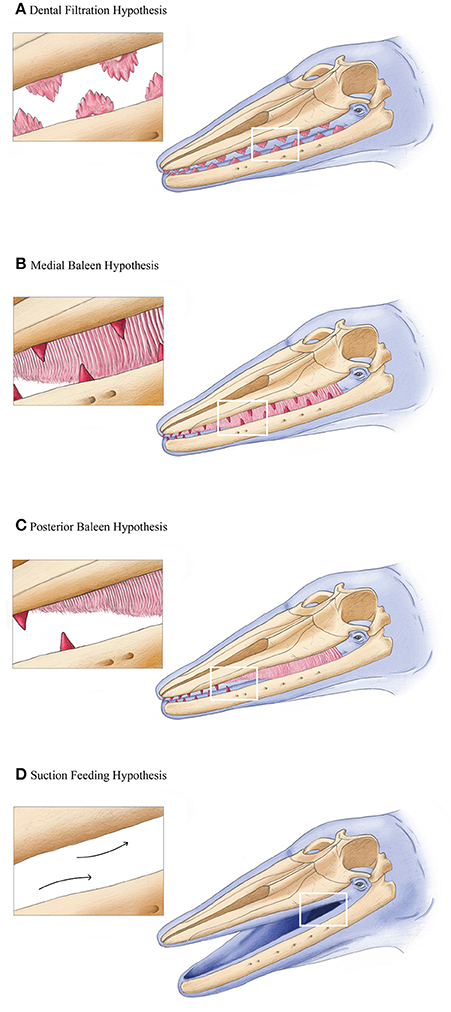
Figure 1. Four hypothetical transitional filter feeding stages in mysticetes, with oblique lateral views of a generalized stem mysticete. Hypotheses (A–D) are not mutually exclusive, nor explicitly step-wise. See text for more details. All artwork courtesy Alex Boersma (http://www.alexboersma.com).
We evaluate the support for these hypotheses based on separate lines of evidence, including ontogenetic, molecular, and paleontological data. We argue that previous hypotheses for baleen origin did not consider all the available lines of evidence, nor couched their mechanisms in a phylogenetic context. For example, a large volume of embryological data on extant mysticetes is scattered across two centuries of literature, spanning at least seven languages. This body of literature has been largely ignored because of its inconsistent or outdated anatomical terminology. While molecular data have recently illuminated enamel and tooth loss in extant mammals, including cetaceans (Meredith et al., 2009, 2011), the underpinnings of baleen development and its morphogenesis are unknown. A recent study on bowhead whale (Balaena mysticetus) ontogeny provided the first strong evidence that baleen morphogenesis recruits developmental patterning from tooth bud signaling (Thewissen et al., 2017). Pending studies that more broadly sample these traits across mysticete phylogeny, we argue that patterns of dental evolution and loss in cetaceans should not be a priori linked, neither in step-wise nor correlative models. Lastly, stem Mysticeti show at least four different lineages with markedly disparate feeding morphologies (Mitchell, 1989; Fitzgerald, 2006, 2010; Boessenecker and Fordyce, 2015a; Marx et al., 2015). The functional and ecological interpretations of these extinct morphologies are unclear and, we argue, potentially over interpreted.
Ultimately, hypotheses for baleen origin and evolution are reliant on morphological interpretations of the fossil record belonging to stem Mysticeti (Figure 2). The timing and mode of baleen origin remains obscure, but it likely occurred sometime between the latest Eocene (~34 million years ago) to the latest Oligocene (~23 million years ago). During this interval of geologic time, ocean circulation patterns, and climate changed dramatically at the Eocene-Oligocene boundary (Prothero and Ivany, 2003), suggesting an important link between environment and morphological innovation in cetaceans. In this review, we provide a new synthesis of existing data, clarifying the mechanisms by which baleen could have evolved, and point to specific research avenues to test these ideas.
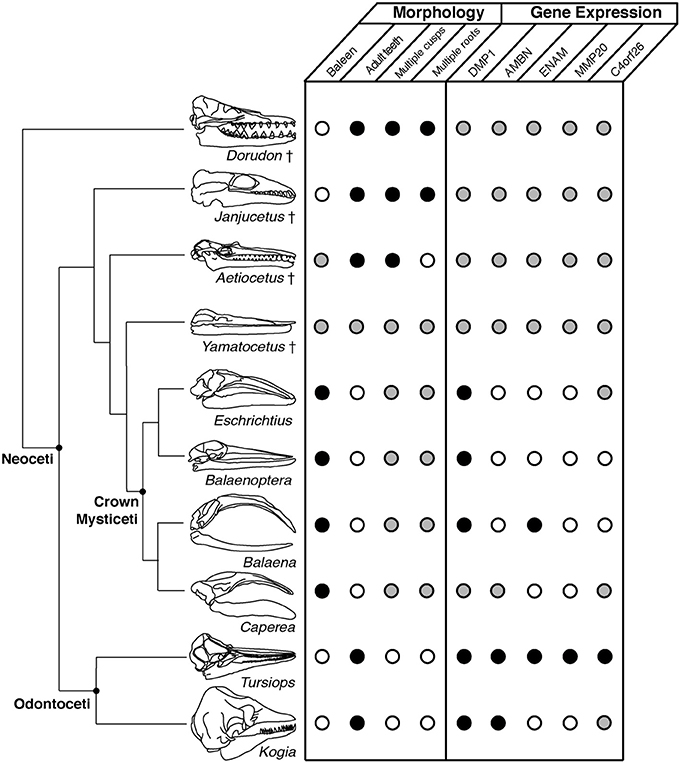
Figure 2. Phylogenetic context for the morphological and molecular traits exhibited by fossil and extant taxa (phylogeny after Marx et al., 2016a). Skull images are scaled to the same condylobasal length. Open circles indicate the absence of a trait or gene function; conversely, black circles indicate their presence. Gray circles indicate an unknown character state.
Paleontological Evidence
The earliest stem mysticete in the fossil record is Llanocetus denticrenatus from the late Eocene of Seymour Island, Antarctica (Mitchell, 1989). Llanocetus is known from an endocast and a fragment of mandible bearing a unique dentition (Figure 3), though additional material, including a skull, awaits description. Based on mandible dimensions, Llanocetus was comparable in size to an adult minke whale (Balaenoptera acutorostrata). Similarly, remains of comparatively sized, toothed cetaceans from the Oligocene of South Carolina (published informally as the “Charleston mysticetes” but otherwise unnamed and undescribed) have been attributed to stem Mysticeti, despite preserving many stem cetacean characteristics (Fitzgerald, 2010). Both Llanocetus and the “Charleston mysticetes” have been coded for phylogenetic analyses (Marx et al., 2015; Boessenecker and Fordyce, 2016), resulting in equivocal phylogenetic placements among stem Mysticeti. However, the lack of any published data on their feeding morphology limits our understanding of their role in the evolution of tooth loss.
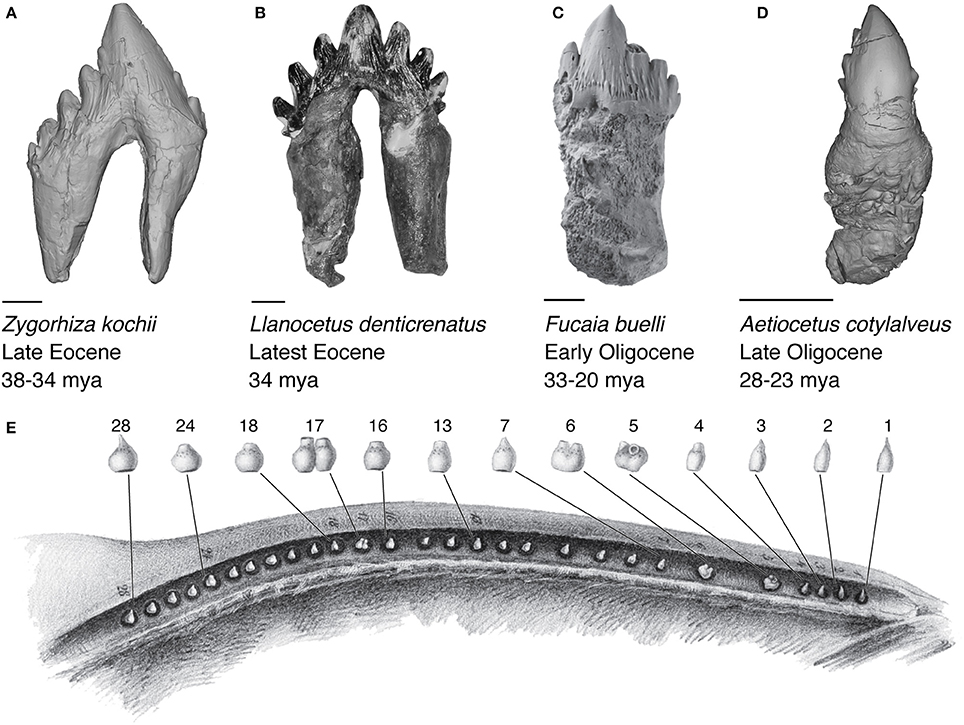
Figure 3. Cheek tooth morphology of relevant fossil and extant cetaceans. (A) 3D model of the stem cetacean Zygorhiza kochii (USNM 11962). (B) Photograph of the stem mysticete Llanocetus dentricrenatus (USNM 183022). (C) Photograph of the stem mysticete Fucaia buelli (UWBM 84024), adapted from Marx et al. (2015). (D) 3D model of the stem mysticete Aetiocetus cotylalveus (USNM 25210). Scale bars represent 10 mm (E) Embryonic tooth buds of a humpback whale (Megaptera novaeangliae) fetus ~115 cm in length, modified from Eschricht (1849).
In contrast, other stem Mysticeti from the mid-late Oligocene (latest Rupelian to Chattian marine stages) are much better known from described material, illustrating vastly different feeding morphologies for geographically and phylogenetically separate groups: The relatively small Mammalodontidae (~3 m in total length), including Janjucetus hunderi (Fitzgerald, 2006), Mammalodon colliveri (Fitzgerald, 2010), and Mammalodon hakataramea (Fordyce and Marx, 2016) from Australia and New Zealand; the equally small Aetiocetidae (~2–4 m in length), from the North Pacific Ocean (Emlong, 1966; Marx et al., 2015); and the minke-sized Eomysticetidae (~6–8 m in length), found in both Northern and Southern hemispheres, represented by several genera (Boessenecker and Fordyce, 2015a,b). Both Mammalodontidae and Aetiocetidae unequivocally possessed functional upper and lower dentitions, while Eomysticetidae either possessed severely reduced or entirely lacked dentition.
Initial ideas about feeding in stem mysticetes proposed a filter feeding mode using the denticulate cusps of their teeth as a sieve, in a similar manner to crabeater seals (L. carcinophagus; Hypothesis 1, Figure 1; Fordyce, 1989; Ichishima, 2005). Specifically, Mitchell (1989) cited the unworn cusps on the tooth crowns of Llanocetus as evidence for such a sieve, but notes that soft tissue would have been necessary given the wide spacing of the adjacent teeth. Fitzgerald (2006, 2010) argued that no stem Mysticeti actually exhibit the closely inter-locking lobate dentition of crabeater seals. Fitzgerald (2010) put forth strong evidence for M. colliveri as a highly specialized suction feeder (Fitzgerald, 2010), while positing that J. hunderi was a sarcophagous raptorial feeder (Fitzgerald, 2006). Aetiocetid teeth are notably smaller, simpler, and more widely spaced than mammalodontids, suggesting a range of feeding modes. Among aetiocetids, only Fucaia buelli preserves a dentition exhibiting occlusion, interpreted for mastication, rather than filtration, based on wear patterns (Marx et al., 2015).
Recent work on aetiocetids has also suggested that at least several species may have had incipient baleen or so-called “proto-baleen” (Deméré and Berta, 2008; Deméré et al., 2008). Specifically, Deméré et al. (2008) argued that foramina on the lateral margins of the palate, medial of tooth alveoli, were homologous with baleen innervation and vascularization in extant mysticetes (Figure 4). Consequently, artistic reconstructions of select aetiocetids depict them as tooth-bearing cetaceans with a functional rack of baleen medial to the dental row (see Hypothesis 2, Figure 1; Deméré and Berta, 2008; Deméré et al., 2008; Ekdale et al., 2015). There are several reasons to question this reconstruction as an overinterpretation.
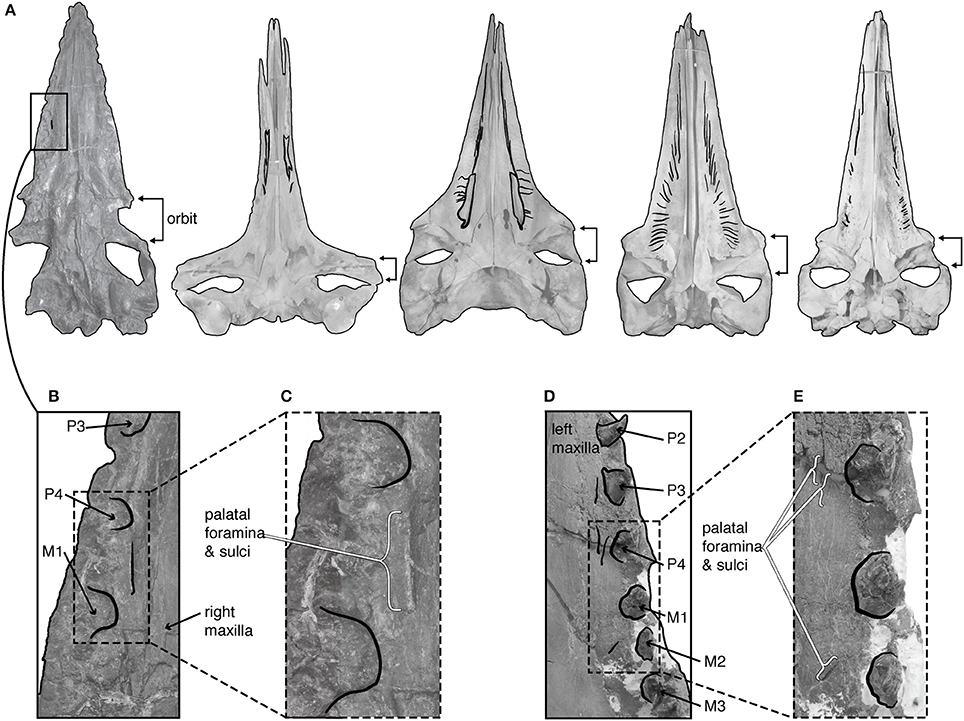
Figure 4. Palatal morphology of fossil and extant Mysticeti. (A) From left to right, the palate of Aetiocetus cotylalveus (USNM 215210), Eubalaena glacialis (USNM 301637), Caperea marginata (USNM 550146), Balaenoptera borealis (USNM 593415), and Eschrichtius robustus (USNM 364973), all scaled to the same condylobasal length. Black shading highlights palatal sulci. (B,C) Magnified view of the palate of Aetiocetus cotylalveus highlighting the single possible palatal sulcus. (D,E) Magnified view of the palate of Aetiocetus weltoni highlighting four possible palatal sulci. M, upper molar; P, upper premolar; after Deméré and Berta (2008).
First, the foramina observed in aetiocetids are patent in only three species of aetiocetids (Aetiocetus cotylalveus, A. weltoni, and Fucaia goedertorum), and the foramina are much smaller and more sparse in number than those of extant mystietes (Figure 4). While recent work suggests the baleen in extant gray whales (Eschrichtius robustus) may be vascularized via branches of the superior alveolar artery (Ekdale et al., 2015), it remains unclear how these branches could supply both teeth and baleen, as would be necessary for extant mysticetes in utero, or for aetiocetids if these possessed both types of feeding structures. Second, Deméré et al. (2008) compared aetiocetids only to species of Balaenoptera, although balaenids lack lateral foramina entirely and Eschrichtius and Caperea display very different patterns (Figure 4). Because palatal vascularization varies extensively in living Mysticeti, the absence of any comparative work verifying how this vascularization supplies baleen across extant Mysticeti makes any assertion of homology with the superficial sulci on aetiocetid palates premature. A comparative study documenting the phylogenetic patterns of vascularization among all extant and extinct taxa would provide the necessary basis for evaluating claims of homology, especially Deméré et al. (2008)'s argument that the palatal foramina of Oligocene mysticetes are direct, functional correlates for baleen.
Immediately outside crown Mysticeti are the extinct eomysticetids, a group of stem mysticetes broadly interpreted as bearing almost complete baleen racks (Figure 2). Because eomysticetids preserve putative alveoli only at the distal tip of the rostrum and mandible, they have been interpreted as lacking a functional adult dentition. The only direct evidence for teeth comes from an isolated incomplete tooth, discovered in association with a specimen referred to Tokarahia lophocephalus (Boessenecker and Fordyce, 2015b). Consequently, this morphology led Boessenecker and Fordyce (2015b) to suggest that eomysticetids possessed functional baleen along most of the rostral margin and a few vestigial teeth or alveoli remaining at the anterior tip of the rostrum (Hypothesis 3, Figure 1; Boessenecker and Fordyce, 2015a). This hypothesis is a functional intermediate between raptorial feeding and filter feeding, although it similarly lacks fossilized baleen as direct evidence, and it does not explain the biomechanics of a feeding mode with both baleen and teeth. Moreover, few eomysticetids preserve complete palates, especially rostral margins, making it difficult to confidently infer the presence of baleen. Although data from Yamatocetus canaliculatus and Waharoa ruwhenua suggest that palatal foramina may have been present, as with aetiocetids, these foramina are few and not clearly homologous to the widely variable palatal foramina observed in extant Mysticeti (Figure 4).
The suction feeding hypothesis was articulated recently by Marx et al. (2015, 2016b), who noted the presence of tooth wear indicative of suction feeding in an undescribed aetiocetid. On this basis, they suggested that suction feeding may have been the ancestral condition for the earliest mysticetes, and that stem mysticetes underwent first a transition from raptorial feeding to suction feeding and then subsequently transition again to bulk filter feeding. Considering the tenuous link between palatal foramina and baleen, this hypothesis better reflects the aetiocetid feeding condition given the available morphological data. However, Marx et al. (2016b)'s hypothesis did not perform ancestral state reconstructions with dense taxonomic sampling, limiting the power of phylogenetic inference for this feeding mode. As only one of several feeding morphologies within Aetiocetidae, suction feeding may be plesiomorphic as the basic mammalian condition for suckling (Werth, 2000). Pending rigorous tests of phylogenetic trait evolution, there is a plurality of potential feeding modes to be considered in the ancestry of baleen whale feeding.
We expand on the last hypothesis by highlighting an additional morphological pathway: Suction feeding may have resulted in the reduction and eventual loss of teeth prior to any emergence of baleen. In other words, the presence or absence of dentition was decoupled from the presence or absence of baleen, concomitant with associated feeding modes. Thus, it is possible that some stem Mysticeti were suction feeding without dentition, and prior to the innovation of baleen and filter feeding (Figure 1). As a morphological parallel, suction feeding remains a viable feeding mode among odontocetes despite the varying degrees of dental simplification or loss (Werth, 2000, 2006).
Moreover, the separation of these evolutionary events is an important consideration: Baleen likely did not evolve its morphological complexity (Pinto and Shadwick, 2013) in a single saltational event. The very first keratinous plates in fossil mysticetes may not have acted as a filter, nor necessarily worked as one. By analog, the first feathers on theropod skin potentially had multiple roles prior to their use as airfoils (Prum, 2005). Darwin (1872) presaged this argument in pointing to the comb-like lamellae that many ducks possess for straining food from water. In the sixth edition of Origin, Darwin (1872) argued that such lamellae were an analogous starting point for how baleen may have originated, and then evolved ever more complexity, culminating in the longest baleen plates, observed in bowhead whales. These remarks highlight the need for explicit models of baleen evolution similar to those presented for other complex integumentary systems, such as feathers (Prum, 2005).
Molecular Evidence
Molecular data concerning the origin of baleen are entirely restricted to studies in the twenty-first century. More crucially, most studies center on the genetics of tooth loss in cetaceans; the genetic underpinnings of baleen morphogenesis has only recently become a topic of study (Thewissen et al., 2017). Primarily, one gene family is responsible for proper dental development in mammals (SCPP, coding for secretory calcium-binding phosphoprotein; Kawasaki and Weiss, 2003; Huq et al., 2005). This gene family includes DMP1 (dentin matrix acidic phosphoprotein), known to affect the development of dentine, cartilage, and bone (Feng et al., 2003; Ye et al., 2005), and the AMBN (amenoblastin) and ENAM (enamelin) genes, which both play roles in enamel development (Hu and Yamakoshi, 2003; Kim et al., 2005).
Building on this work, Deméré et al. (2008) demonstrated that SCPP genes are present, but not functional in mysticetes, suggesting that the loss of functional teeth had resulted in the SCPP gene family being released from selective pressures. All frameshift mutations occurred in two enamel related genes, AMBN and ENAM; no frame shifts were observed in the multi-functional DMP1 gene (Deméré et al., 2008). Meredith et al. (2009) expanded on these results by sequencing nearly 80% of the protein coding region for ENAM in all placental mammals that either lack enamel or lack teeth entirely. Frameshift or stop codons occurred in 19 of the 20 taxa that are enamel-less or edentulous (and all five of the mysticetes studied), despite no mutations occurring in enamel-bearing placentals. While all mysticetes exhibited either a frameshift or a stop codon mutation, no specific mutation in enamel-related genes united all Mysticeti (Meredith et al., 2009). Later, Meredith et al. (2011) presented the first evidence for pseudogenization of a tooth gene in the common ancestor of all living mysticetes, discovered not in the SCPP family of genes, but in the enamelysin gene of the matrix metalloproteinase 20 (MMP20) family. MMP20 processes structural proteins secreted during enamel formation; mutations in MMP20 lead to thin, hypomineralized enamel (Caterina et al., 2002). Meredith et al. (2011) identified a CHR-2 SINE retroposon insertion in exon 2 of MMP20 present in the eight mysticetes studied (representative of all extant genera), suggesting that this mutation arose in the shared common ancestor of crown Mysticeti, prior to reported mutations in ENAM and AMBN (Meredith et al., 2009). Pseudogenization can occur either from neutral evolution or positive selection, thus it is unclear if the SINE insertion on MMP20 happened as release from or under active selection (Meredith et al., 2011). However, MMP20 also appears to be a pseudogene in pygmy sperm whales (Kogia breviceps) and Hoffman's two-toed sloths (Choloepus hoffmanni), which both have teeth without enamel, implying that MMP20 is critical exclusively to enamel production. Springer et al. (2015) identified inactivating mutations of a different gene C4orf26, which occurs only in toothless, but not enamel-less taxa. This pattern implies that C4orf26, unlike the other genes mentioned above, is tooth-specific but not enamel-specific; moreover, it reinforces a decoupling between the processes responsible for tooth and enamel production. Recently, Thewissen et al. (2017) provided the first study to shed light on the genetic underpinnings of baleen morphogenesis. This study examined the development of teeth and baleen in bowhead whales and demonstrated that the fibroglast growth factor family (specifically FGF4) is critical in the development of baleen. FGF4 is a protein typically associated with the development of teeth, not with those of hair or palatal rugae. Thewissen et al. (2017) thus suggested that the genetic underpinnings of baleen morphogenesis may be more closely tied to those of tooth development than morphological studies may suggest. In sum, the evolution of tooth loss is a more complex process than indicated by morphological observations alone and it remains unclear how it relates to the origin of baleen. We hope that additional endeavors to understand baleen-associated genes and morphogenesis in early ontogeny will continue to bear significantly on these issues.
Embryological and Histological Evidence
The occurrence of teeth in mysticete embryos was first reported in the early nineteenth century by Saint-Hilaire (1807) in the caption of a figure comparing bird skulls. Saint-Hilaire provided few details about mysticete anatomy, but hypothesized that rapid ossification of the rostrum was responsible for damaging and stifling mysticete dentition before it fully formed. Subsequent authors (see references in subsections below) provided anatomical descriptions of the tooth buds at varying stages of development in mysticetes, yet disagreed over morphological observations and implied homology (e.g., heterodonty vs. homodonty, deciduous vs. permanent dentition). It should be noted that terminology in this section follows that of the original authors (teeth vs. tooth buds); unless otherwise noted, both terms refer to the dentition developing in utero rather than to fully formed, mineralized, and erupted dentition, as seen in other mammals. It also should be noted that, although beyond the scope of this paper, much of this literature and the citations therein contain valuable data to understand the developmental basis for evolutionary changes in the dentition of odontocetes and Mammalia as a whole. They may serve as vital starting points for future studies on such topics.
Shape of the Dentition
Eschricht (1846) initially described the teeth of mysticete embryos as pointed, likening them to those of Tursiops (Figure 3), though he later categorized the overall dentition as heterodont (Eschricht, 1849). Julin (1880) identified and described three forms of teeth in mysticetes: Pointed anteriorly; forked in the middle of the incipient tooth row; and three-pronged posteriorly. Pouchet and Chabry (1882) echoed this standpoint, describing anterior pointed and posterior tricuspid teeth. Kükenthal, however, disagreed with his predecessors, and considered the dentition unicusped and entirely homodont (Kükenthal, 1891, 1893). Kükenthal attributed the appearance of multicusped teeth to damage from resorption. Ridewood (1923) noted the presence of accessory cusps on the cheek teeth, but considered them insignificant and judged the entire dentition as homodont. In 1954, Van Dissel-Scherft and Vervoort followed the scheme provided by Leche (1895) to provide the first descriptions of teeth with reference to standard mammalian stages of tooth development, concluding that Kükenthal's descriptions were accurate, although incorrectly interpreted as homodont. Van Dissel-Scherft and Vervoort (1954a,b) instead argued that both Eschricht (1849) and Julin (1880) correctly interpreted the dentition of living mysticetes as heterodont. Notably, the recent work by Thewissen et al. (2017) suggests that this condition may not be consistent between the upper and lower dentition. Thewissen et al. (2017) indicate that, at least in bowhead whales, the upper dentition is homodont while the lower is heterodont.
Double Teeth
Many of these aforementioned authors also noted so-called “double teeth” in mysticetes, which appeared as individual, separate, and tightly appressed teeth, though others considered them indicative of complex tooth development. Double teeth were first reported by Owen (1845), though he credited their discovery to Eschricht, without providing a citation. Whether from his own observations, or from conversation with Eschricht, Owen argued that double teeth were the result of spontaneous fission from a single tooth germ. Julin (1880) later confirmed the presence of double teeth, but did not comment on them. Kükenthal (1891) described double teeth in detail, noting that they are more abundant in younger fetuses and that they can occur anywhere along the dental row, not only posteriorly, implying that they are not homologous with multicusped molars. Later, Kükenthal further concluded that their increased abundance in younger fetuses indicates that double teeth are the ancestral condition in mysticetes, and that the increased number of teeth in crown Cetacea is the result of ancestral double teeth fission (Kükenthal, 1893). Thus, he argued that only the youngest fetuses are heterodont (with true double teeth), prior to achieving homodonty later in ontogeny through fission (with single teeth, modified by resorption, unzipping from the center, giving the impression of two distinct tooth buds). While Ridewood (1923) also noted the presence of double teeth and their high frequency in younger embryos, he mentioned little in the way of homodonty or heterodonty. Van Dissel-Scherft and Vervoot largely agreed with Kükenthal (Van Dissel-Scherft and Vervoort, 1954a,b), noting little evidence of double teeth, attributing the occasional double or even triple tooth observed in large embryos to resorption altering the shape of the tooth. Thus, overall, the issue of a homodont or heterodont dentition in mysticete embryos largely hinges on the interpretation of cheek teeth as either being multicusped molars or merely double teeth resulting from fission.
Karlsen (1962) provided thorough comparative descriptions of rorqual embryos ranging from 2 to 148 cm in length (Kükenthal's smallest had been 43 cm; Kükenthal, 1891, 1893). Karlsen suggested that the youngest specimens all have single teeth and that the double teeth observed by previous authors were the result of fusion (not fission) occurring very early in embryonic development (at lengths smaller than Kükenthal's data). Karlsen (1962) also noted that the division of teeth in later embryos described by Kükenthal had never actually been observed, only inferred, and that there is no mechanism to explain the division of dentine, which already covers the teeth at fetal lengths of 55 cm. Karlsen (1962) therefore concluded double teeth are the result of fusion, and that no fission occurs, as described by Kükenthal.
Which Dentition?
Along with tooth morphology, it remains unclear whether the tooth buds observed in mysticete embryos represent the first, deciduous dentition, or the second, permanent dentition. Kükenthal (1891) first commented that the tooth buds likely represent the first dentition because an additional set of tooth buds appear to erupt lingual to the latter row. Later, Kükenthal (1893) revised this description to suggest a third set of tooth buds labial to the main dental row. Thus, Kükenthal (1891) interpreted as many as three sets of dentitions, and because he presumed that baleen was homologous with the permanent dentition, he argued that the “tooth germ” (Zahnanlagen) was the site of origin for the emerging baleen. Van Dissel-Scherft and Vervoot found no additional dental material except in the largest of embryos, which they argued were the end result of resorption dissociating individual zahnanlagen (Van Dissel-Scherft and Vervoort, 1954a,b). Karlsen (1962) agreed with this argument, and concluded that only a single dentition is present throughout the entire embryonic development and that it is homologous to the first, deciduous dentition.
More recently, histological studies have attempted to understand the developmental patterns for tooth buds and baleen origin in the context of growth factors and molecular signaling (Ishikawa et al., 1999). While these authors commented neither on homodonty nor double teeth, they argued that resorption mirrors the mechanism of tooth shedding in diphyodont mammals. However, they noted that, in diphyodont mammals, only the root of the deciduous tooth undergoes resorption, whereas in mysticete embryos the entire tooth is resorbed (Ishikawa et al., 1999). Clearly, further investigations should focus on more in-depth histological work about the homology of the tooth buds, their morphology, and the differences in these traits across a wider ontogenetic series, along with interspecific comparisons across mysticete species. In parallel with questions about tooth shape in early ontogeny, Thewissen et al. (2017) pointed to a mismatch in the resorption patterns between the upper and lower dentitions of fetal bowhead whales, with the lower dentition exhibiting some evidence of replacement, while the upper dentition not.
Discussion
Based on the available range of evidence, the origin and evolution of baleen in mysticetes defies simple explanations. However, one conclusion is that the origin of baleen is not necessarily coupled with tooth loss. These two evolutionary processes may be connected in one or more ways; we argue, however, that they should not be linked as an a priori condition simply because both are oral morphological systems related to feeding. Similarly, any models for baleen origins need to also address tooth loss, and outline explicit transformations and expected transitional stages. Equally, the hypotheses presented herein are not mutually exclusive, although they do depend on different types of data that have not always been assembled for testing. All four hypotheses rely heavily on morphological evidence from paleontological data, yet they would also each benefit from better integration with modern anatomical, molecular, histological, and biomechanical studies. Berta et al. (2016) also highlighted the value of geochemical data from fossil data. Discriminating among the available hypotheses for baleen origins will require integrating the full range of emerging datasets. Below, we review the viability of each hypothesis given current evidence and discuss ways that they may be further tested.
The dental filtration hypothesis argues that filter feeding evolved first using denticulate cheek teeth, and that baleen later evolved as a secondary structure to increase feeding efficiency. While this hypothesis addresses the issue of how filter feeding can occur without baleen, it does not address the timing or pattern of tooth loss, nor the origin of baleen. While fossil mysticetes do possess denticulate teeth (Figure 3), none exhibit the occlusion observed in filter feeding crabeater or leopard seals (Hocking et al., 2013). This hypothesis requires detailed biomechanical modeling, either using fluid dynamics or experimental physical models to test its viability in stem mysticetes, as has been done for baleen in extant mysticetes (Werth, 2004, 2013). In particular, such work should focus on the distinction between dental filtration and bulk filtration, and whether the former can effectively lead to the latter.
The medial baleen hypothesis proposes that proto-baleen evolved medial to an existing and functional dental row, perhaps allowing some lineages (e.g., aetiocetids) to alternate between filter feeding and raptorial feeding. While this hypothesis notes the presence of palatal sulci in select aetiocetids, it fails to provide a developmental explanation for the presence of both feeding structures concurrently, and it fails to provide an anatomical explanation for how both teeth and baleen can be innervated and vascularized simultaneously. While rorqual palates show some similarities to the palatal foramina of aetiocetids (Deméré et al., 2008), other extant Mysticeti (Balaena, Eubalaena, Caperea) do not show sulci, but rather fenestrae (Figure 4), which limits the argument for palatal foramina in aetiocetids as direct, functional correlates for baleen. The biomechanical implications of possessing both structures simultaneously remains unclear given the known challenges of modeling fluid flow in the oral cavity of living mysticetes (Werth, 2001). Embryological data suggest that baleen develops directly ventral to the resorbing tooth buds, making it inconsistent with the medial baleen hypothesis. The medial baleen hypothesis would benefit from further embryological studies, perhaps employing immunohistochemistry techniques, to document the position of development of baleen with respect to the resorbing tooth buds.
The posterior baleen hypothesis suggests that stem mysticetes such as the eomysticetids possessed functional baleen racks along most of the rostrum in the same sagittal plane, with a few vestigial teeth at the anterior most tip of the rostrum. This hypothesis is consistent with the observation that baleen develops ventral to the existing tooth buds in embryos, and it is bolstered by fossil taxa (e.g., Waharoa and Tokarahia; Boessenecker and Fordyce, 2015a) that exhibit alveoli only at the distal tip of the rostrum. However, only the holotype specimen of Y. canaliculatus (Figure 2) preserves a complete palatal margin, leaving the morphology of other eomysticetids unknown. Moreover, the mandibles of extant Mysticeti preserve extensive foramina along their dorsomedial margin all throughout ontogeny (Peredo et al., 2016); thus, the mere presence of a foramen on the mandibles is not necessarily indicative of a true alveolus (only a single possible tooth root has been attributed to an eomysticetid, thus far). The posterior baleen hypothesis would benefit from histological studies of embryos documenting whether baleen emerges all at once, or beginning at the posterior end and moving anteriorly.
Lastly, the suction feeding hypothesis suggests that stem mysticetes enhanced their suction capabilities, resulting in a transition to filter feeding that was mediated by suction. This hypothesis is bolstered by evidence for suction in select stem mysticetes based on wear in Mammalodon and an unnamed aetiocetid (Fitzgerald, 2010; Marx et al., 2016b). However, the presence of tooth wear is probably auxillary evidence for suction feeding, at best. Suction feeding is the basal condition for all marine mammals, and this mode was already considered the likely feeding mechanism for Mammalodon (Fitzgerald, 2010); even raptorial feeders employ some degree of suction during the feeding cycle (Werth, 2000). Moreover, the wide variability in dental morphology across mammalodontids and aetiocetids hints at many distinct feeding strategies, including raptorial feeding with mastication (Marx et al., 2015), without mastication (Barnes et al., 1994), and suction feeding (Marx et al., 2016b). To better understand the evolution of feeding modes among stem Mysticeti, we propose clear categorization or quantification of known suction-related traits (e.g., palate shape, hyoid proportions, and tooth wear) with clear methods for phylogenetic reconstruction of ancestral traits. Such a framework would permit different phylogenetic tree topologies of stem mysticete relationships as multiple working hypotheses, and a strong basis for making evolutionary statements about trait transformation.
We hypothesize that efficient suction feeding in stem mysticetes would have resulted first in tooth loss, prior to the origin of baleen. The potential decoupling of enamel loss and tooth loss as separate genetic events provides a blueprint by which this hypothesis, involving tooth loss and baleen development as separate events, may be tested. While suction feeding without the aid of a feeding apparatus would seem unlikely for a zooplankton-based diet, it is consistent with the known record of piscivory, which has been documented in Eocene basilosaurids (Uhen, 2004), Miocene mysticetes (Collareta et al., 2015), and extant mysticetes, all of which likely employ some degree of suction during the gape cycle (Werth, 2000). Thus, suction feeding as a basal feeding mode for stem mysticetes best incorporates histological and molecular datasets by proposing a fundamental decoupling between the loss of teeth and the emergence of baleen.
All four hypotheses can be tested by additional contributions from the fossil record. For example, the discovery of Oligocene mysticetes with soft tissue preservation (as in Miocene mysticetes) would provide direct evidence for the presence of baleen. Currently, the oldest record of fossil baleen dates to the late Miocene (Esperante et al., 2008), which implies a stratigraphic gap between these direct records and the inferred presence of baleen in Oligocene toothless mysticetes. Nevertheless, fossil baleen from the late Miocene permits basic morphological comparisons to the wide range of baleen types among extant mysticetes (Woodward et al., 2006).
While phylogenetic bracketing strengthens the inference for baleen presence in any extinct taxon within crown Mysticeti, we strongly caution against assuming the presence of baleen in any toothless taxa outside crown Mysticeti, on the basis that direct osteological correlates for baleen are poorly supported. For example, Peredo and Uhen (2016) interpreted the stem mysticete Sitsqwayk cornishorum as edentulous because it lacks any evidence of teeth or alveoli in the dentary. However, the type and only known specimen of this taxon preserves merely a fragmentary palate. Moreover, because Sitsqwayk is phylogenetically placed on the stem outside of crown Mysticeti, there is a weak basis for inferring baleen based on phylogeny. Despite its incompleteness, its phylogenetic position implies that the sequence of tooth loss in basal mysticete evolution was not a straightforward or even stepwise pattern.
Challenges and Unresolved Questions
In some vertebrate clades, integrative approaches have been successful in illuminating the evolutionary origin of novel integumentary and tissue systems (e.g., feathers in archosaurs, headgear in ruminants; Prum, 2005; Davis et al., 2011). For the origin of baleen in mysticetes, this problem remains one of the more difficult questions to answer in cetacean macroevolution for reasons relating to the challenges of studying living mysticetes. Their broad habitat ranges and large body size imposes serious logistical and methodological constraints (Pyenson, 2011), while their conservation status and the legal framework protecting them depends on geopolitical context, making it extremely difficult to collect fresh vouchers for specimen-based research, especially from fetal material crucial to understanding embryonic tooth development. Examination of the Yamato and Pyenson (2015) dataset failed to identify tooth buds in mysticete embryos between 16 and 500 cm in total length, suggesting they are too small to appear in conventional medical computed tomography (CT) scanning techniques, though potentially could be visible with enhancement (e.g., iodine-based contrast-enhanced methods).
While genomic studies have begun to shed light on the molecular underpinnings of tooth loss, progress on understanding the genetics of baleen growth and development has been limited, with Thewissen et al. (2017) as a notable exception. The morphological structure of baleen lacks any analog among living and extinct vertebrates (i.e., there is no similar keratinous filter feeding structure). However, given that close artiodactyl relatives of cetaceans also exhibit rugose keratinized palates, we suggest looking for homologous gene expression patterns in these taxa with potential structural analogs of precursor states for early baleen. Alternatively, examining the genetics of hair loss may also yield insights about the expression of keratin in mysticetes. Most crucially, any study on baleen morphogenesis requires a thorough dissection of its growth pattern and timing relative to tooth development and resorption, as these two systems are possibly decoupled in mysticete evolution.
We argue that a complete understanding of the origin of baleen must arise from interdisciplinary efforts and all available datasets (Berta et al., 2016). Museum collections that preserve embryonic and fetal mysticete material should be investigated in more detail, especially with sophisticated non-invasive imaging techniques, to better resolve in situ tooth bud morphology and its anatomical and ontogenetic contexts. Among other questions, these studies should endeavor to determine whether mysticetes possess only a single dentition, identify whether it represents the deciduous or permanent dentition, if it is heterodont or homodont, and whether enamel develops. It is also essential that paleontological efforts continue to refine a phylogenetic context. While it is clear that stem mysticetes represent many ancestral conditions for crown Mysticeti, the exact phylogenetic relationships of these basal branching taxa are unresolved (Marx et al., 2016a), and it remains unclear how additional fossil material may impact the phylogeny. Lastly, future studies need to explain the specific biomechanics of transitional morphology in any incipient filter feeding structures among fossil taxa.
Author Contributions
All authors were involved in study design, data analysis, preparation of figures, and review of final drafts.
Funding
Research was funded by the Smithsonian Institution, its Remington Kellogg Fund, and with support from the Basis Foundation. The funders had no role in study design, data collection and analysis, decision to publish, or preparation of the manuscript.
Conflict of Interest Statement
The authors declare that the research was conducted in the absence of any commercial or financial relationships that could be construed as a potential conflict of interest.
Acknowledgments
We thank David Bohaska, Kristofer Helgen, John Ososky, and Darrin Lunde for access to USNM specimens. Thanks to Chesapeake Testing (Belcamp, Maryland), C. Peitsch, R. Peitsch, and C. Schueler for providing access to resources for scanning and modeling specimens. We also thank Cristina Robinson for assistance photographing specimens and the Biodiversity Heritage Library and Smithsonian Libraries for facilitating access to numerous references. Finally, we thank Hans-Dieter Sues for valuable assistance translating German literature.
References
Barnes, L. G., Kimura, M., Furusawa, H., and Sawamura, H. (1994). Classification and distribution of Oligocene Aetiocetidae (Mammalia; Cetacea; Mysticeti) from western North America and Japan. Island Arc. 3, 392–431. doi: 10.1111/j.1440-1738.1994.tb00122.x
Berta, A., Lanzetti, A., Ekdale, E. G., and Deméré, T. A. (2016). From teeth to baleen and raptorial to bulk filter feeding in mysticete cetaceans: the role of paleontological, genetic, and geochemical data in feeding evolution and ecology. Integr. Comp. Biol. 56, 1271–1284. doi: 10.1093/icb/icw128
Boessenecker, R. W., and Fordyce, R. E. (2015a). Anatomy, feeding ecology, and ontogeny of a transitional baleen whale: a new genus and species of Eomysticetidae (Mammalia: Cetacea) from the oligocene of New Zealand. PeerJ 3, 1–69. doi: 10.7717/peerj.1129
Boessenecker, R. W., and Fordyce, R. E. (2015b). A new genus and species of eomysticetid (Cetacea: Mysticeti) and a reinterpretation of “Mauicetus” lophocephalus Marples, 1956: transitional baleen whales from the upper oligocene of New Zealand. Zool. J. Linn. Soc. 175, 607–660. doi: 10.1111/zoj.12297
Boessenecker, R. W., and Fordyce, R. E. (2016). A new eomysticetid from the Oligocene Kokoamu Greensand of New Zealand and a review of the Eomysticetidae (Mammalia, Cetacea). J. Syst. Palaeontol. doi: 10.1080/14772019.2016.1191045. [Epub ahead of print].
Caterina, J. J., Skobe, Z., Shi, J., Ding, Y., Simmer, J. P., Birkedal-Hansen, H., et al. (2002). Enamelysin (matrix metalloproteinase 20)-deficient mice display an amelogenesis imperfecta phenotype. J. Biol. Chem. 277, 49598–49604. doi: 10.1074/jbc.M209100200
Collareta, A., Landini, W., Lambert, O., Post, K., Tinelli, C., Di Celma, C., et al. (2015). Piscivory in a miocene cetotheriidae of Peru: first record of fossilized stomach content for an extinct baleen-bearing whale. Naturwissenschaften 102, 70. doi: 10.1007/s00114-015-1319-y
Darwin, C. (1872). The Origin of the Species by Means of Natura Selection or the Preservation of Favoured Races in the Struggle for Life. London: John Murray.
Davis, E. B., Brakora, K. A., and Lee, A. H. (2011). Evolution of ruminant headgear: a review. Proc. R. Soc. B 278, 2857–2865. doi: 10.1098/rspb.2011.0938
Deméré, T. A., and Berta, A. (2008). Skull anatomy of the Oligocene toothed mysticete Aetioceus weltoni (Mammalia; Cetacea): implications for mysticete evolution and functional anatomy. Zool. J. Linn. Soc. 154, 308–352. doi: 10.1111/j.1096-3642.2008.00414.x
Deméré, T. A., McGowen, M. R., Berta, A., and Gatesy, J. (2008). Morphological and molecular evidence for a stepwise evolutionary transition from teeth to baleen in mysticete whales. Syst. Biol. 57, 15–37. doi: 10.1080/10635150701884632
Ekdale, E. G., Deméré, T. A., and Berta, A. (2015). Vascularization of the Gray Whale palate (Cetacea, Mysticeti, Eschrichtius robustus): soft tissue evidence for an alveolar source of blood to baleen. Anat. Rec. 298, 691–702. doi: 10.1002/ar.23119
Emlong, D. R. (1966). A new archaic cetacean from the Oligocene of Northwest Oregon. Bull. Ore. Uni. Mus. Nat. Hist. 3, 1–51.
Eschricht, D. F. (1849). Zoologisch-Anatomisch-Physiologische Untersuchungen Über die Nordischen Wallthiere. Leipzig: Verlag von Leopold Voss.
Esperante, R., Brand, L., Nick, K. E., Poma, O., and Urbina, M. (2008). Exceptional occurrence of fossil baleen in shallow marine sediments of the Neogene Pisco Formation, Southern Peru. Palaeogeogr. Palaeoclimatol. Palaeoecol. 257, 344–360. doi: 10.1016/j.palaeo.2007.11.001
Feng, J. Q., Huang, H., Lu, Y., Ye, L., Xie, Y., Tsutsui, T. W., et al., (2003). The dentin matrix protein 1 (Dmp1) is specifically expressed in mineralized, but not soft, tissues during development. J. Dent. Res. 82, 776–780. doi: 10.1177/154405910308201003
Fitzgerald, E. M. G. (2006). A bizarre new toothed mysticete (Cetacea) from Australia and the early evolution of baleen whales. Proc. R. Soc. B 273, 2955–2963. doi: 10.1098/rspb.2006.3664
Fitzgerald, E. M. G. (2010). The morphology and systematics of Mammalodon colliveri (Cetacea: Mysticeti), a toothed mysticete from the oligocene of Australia. Zool. J. Linn. Soc. 158, 367–476. doi: 10.1111/j.1096-3642.2009.00572.x
Fordyce, R. E. (1989). Origins and evolution of Antarctic marine mammals. Geol. Soc. Spec. Publ. 47, 269–281. doi: 10.1144/GSL.SP.1989.047.01.20
Fordyce, R. E., and Marx, F. (2016). Mysticetes baring their teeth: a new fossil whale, Mammalodon hakataramea, from the Southwest Pacific. Mem. Mus. Victoria 74, 107–116.
Goldbogen, J. A. (2010). The ultimate mouthful: lunge feeding in rorqual whales. Am. Sci. 98, 124–131. doi: 10.1511/2010.83.124
Hocking, D. P., Evans, A. R., and Fitzgerald, E. M. G. (2013). Leopard seals (Hydrurga leptonyx) use suction and filter feeding when hunting small prey underwater. Polar Biol. 36, 211–222. doi: 10.1007/s00300-012-1253-9
Hu, J. C.-C., and Yamakoshi, Y. (2003). Enamelin and autosomal-dominant amelogenesis imperfecta. Crit. Rev. Oral Biol. Med. 14, 387–398. doi: 10.1177/154411130301400602
Huq, N. L., Cross, K. J., Ung, M., and Reynolds, E. C. (2005). A review of protein structure and gene organisation for proteins associated with mineralised tissue and calcium phosphate stabilisation encoded on human chromosome 4. Arch. Oral Biol. 50, 599–609. doi: 10.1016/j.archoralbio.2004.12.009
Ichishima, H. (2005). Notes on the phyletic relationships of the Aetiocetidae and the feeding ecology of toothed mysticetes. Bull. Ashoro Mus. Paleontol. 3, 111–117.
Ishikawa, H., Amasaki, H., Dohguchi, H., Furuya, A., and Suzuki, K. (1999). Immunohistological distributions of fibronectin, tenascin, type I, III and IV collagens, and laminin during tooth development and degeneration in fetuses of minke whale, Balaenoptera acutorostrata. J. Vet. Med. Sci. 61, 227–232. doi: 10.1292/jvms.61.227
Julin, C. (1880). Recherches sur l'ossification du maxillaire inférieur et sur la constitution du système dentaire chez le foetus de la Balaenoptera rostrata. Arch. Biol. (Liege). 1, 75–136.
Karlsen, K. (1962). Development of tooth germs and adjacent structures in the whalebone whale (Balaenoptera physalus (L.)). Hvalrådets Skrifter 45, 1–56.
Kawasaki, K., and Weiss, K. M. (2003). Mineralized tissue and vertebrate evolution: the secretory calcium-binding phosphoprotein gene cluster. Proc. Natl. Acad. Sci. U.S.A. 100, 4060–4065. doi: 10.1073/pnas.0638023100
Kim, J.-W., Seymen, F., Lin, B. P.-J., Kiziltan, B., Gencay, K., Simmer, J. P., et al. (2005). ENAM mutations in autosomal-dominant amelogenesis imperfecta. J. Dent. Res. 84, 278–282. doi: 10.1177/154405910508400314
Kükenthal, W. G. (1893). Vergleichend-anatomische und entwicklungsgeschichtliche Untersuchungen an Waltieren. Denkschr. Med.-Naturwiss Ges. Jena 3, 1–448.
Leche, W. (1895). Zur Entwicklungsgeschichte des Zahnsystems der Säugethiere: zugleich ein Beitrag zur Stammesgeschichte dieser Thiergruppe. Ontogenie. Stuttgart: E. Nägele.
Marx, F. G., Hocking, D. P., Park, T., Ziegler, T., Evans, A. R., and Fitzgerald, E. M. G. (2016b). Suction feeding preceded filtering in baleen whale evolution. Mem. Mus. Vic. 75, 71–82.
Marx, F. G., Tsai, C.-H., and Fordyce, R. E. (2015). A new Early Oligocene toothed “baleen” whale (Mysticeti: Aetiocetidae) from western North America: one of the oldest and the smallest. R. Soc. Open Sci. 2, 1–35. doi: 10.1098/rsos.150476
Meredith, R. W., Gatesy, J., Cheng, J., and Springer, M. S. (2011). Pseudogenization of the tooth gene enamelysin (MMP20) in the common ancestor of extant baleen whales. Proc. R. Soc. B 278, 993–1002. doi: 10.1098/rspb.2010.1280
Meredith, R. W., Gatesy, J., Murphy, W. J., Ryder, O. A., and Springer, M. S. (2009). Molecular decay of the tooth gene Enamelin (ENAM) mirrors the loss of enamel in the fossil record of placental mammals. PLoS Genet. 5:e1000634. doi: 10.1371/journal.pgen.1000634
Mitchell, E. D. (1989). A new cetacean from the late eocene la meseta formation, Seymour island, Antarctic Peninsula. Can. J. Fish. Aquat. Sci. 46, 2219–2235. doi: 10.1139/f89-273
Owen, R. (1845). Odontography, OR, a Treatise on the Comparative Anatomy of the Teeth, Their Physiological Relations, Mode of Developement, and Microscipic Structure, in the Vertebrate Animals. London: Hippolyte Bailierre.
Peredo, C. M., Pyenson, N. D., and Uhen, M. D. (2016). “Morphological consequences of tooth loss: a comparison of the course of the mandibular canal in mysticete cetaceans using 3D models,” in International Congress of Vertebrate Morphology (Washington DC).
Peredo, C. M., and Uhen, M. D. (2016). A new basal chaeomysticete (Mammalia: Cetacea) from the Late Oligocene Pysht Formation of Washington, USA. Pap. Palaeontol. 2, 533–554. doi: 10.1002/spp2.1051
Pinto, S. J. D., and Shadwick, R. E. (2013). Material and structural properties of fin whale (Balaenoptera physalus) Zwischensubstanz. J. Morphol. 274, 947–955. doi: 10.1002/jmor.20154
Pouchet, G., and Chabry, M. (1882). Sur l'evolution des dents de Balaenides. C. R. 'Acad. Sci. 94, 540–542.
Prothero, D. R., and Ivany, L. C. (2003). From Greenhouse to Icehouse: The Marine Eocene-Oligocene Transition. New York, NY: Columbia University Press.
Prum, R. O. (2005). Evolution of the morphological innovations of feathers. J. Exp. Zool. B Mol. Dev. Evol. 304, 570–579. doi: 10.1002/jez.b.21073
Pyenson, N. D. (2011). The high fidelity of the cetacean stranding record: insights into measuring diversity by integrating taphonomy and macroecology. Proc. Biol. Sci. 278, 3608–3616. doi: 10.1098/rspb.2011.0441
Ridewood, W. G. (1923). Observations on the skull in foetal specimens of whales of the genera Megaptera and Balaenoptera. Philos. Trans. R. Soc. B 211, 209–272. doi: 10.1098/rstb.1923.0005
Saint-Hilaire, G. (1807). Considérations sur les pièces de la tête osseuse des animaux vertébrés, et particulièrement sur celles du crâne des oiseaux. Ann. Mus. Hist. Nat. 10, 342–365.
Springer, M. S., Starrett, J., Morin, P. A., Lanzetti, A., Hayashi, C., and Gatesy, J. (2015). Inactivation of C4orf26 in toothless placental mammals. Mol. Phylogenet. Evol. 95, 34–45. doi: 10.1016/j.ympev.2015.11.002
Thewissen, J. G., Hieronymus, T. L., George, J. C., Suydam, R., Stimmelmayr, R., and McBurney, D. (2017). Evolutionary aspects of the development of teeth and baleen in the bowhead whale. J. Anat. doi: 10.1111/joa.12579. [Epub ahead of print].
Uhen, M. D. (2004). Form, function, and anatomy of Dorudon atrox (Mammalia, Cetacea): an archaeocete from the middle to late eocene of Egypt. Univ. Mich. Mus. Paleontol. Pap. Paleontol. 34, 111–222.
Uhen, M. D. (2010). The origin(s) of whales. Ann. Rev. Earth Planet. Sci. 38, 189–219. doi: 10.1146/annurev-earth-040809-152453
Van Dissel-Scherft, M. C., and Vervoort, W. (1954a). Development of the teeth in fetal Balaenoptera physalus (L.) (Cetacea, Mysticeti) - part, I. Proc. Ser. C Biol. Med. Sci. 57, 196–202.
Van Dissel-Scherft, M. C., and Vervoort, W. (1954b). Development of the teeth in fetal Balaenoptera physalus (L.) (Cetacea, Mysticeti) - part, II. Proc. Ser. C Biol. Med. Sci. 57, 203–210.
Werth, A. J. (2000). “Feeding in marine mammals,” in Feeding: Form, Function and Evolution in Tetrapod Vertebrates, ed K. Schwenk (New York, NY: Academic Press), 475–514.
Werth, A. J. (2001). How do mysticetes remove prey trapped in baleen. Bull. Mus. Comp. Zool. 156, 189–203.
Werth, A. J. (2004). Models of hydrodynamic flow in the bowhead whale filter feeding apparatus. J. Exp. Biol. 207, 3569–3580. doi: 10.1242/jeb.01202
Werth, A. J. (2006). Mandibular and dental variation and the evolution of suction feeding in Odontoceti. J. Mammal. 87, 579–588. doi: 10.1644/05-MAMM-A-279R1.1
Werth, A. J. (2013). Flow-dependent porosity and other biomechanical properties of mysticete baleen. J. Exp. Biol. 216, 1152–1159. doi: 10.1242/jeb.078931
Woodward, B. L., Winn, J. P., and Fish, F. E. (2006). Morphological specializations of baleen whales associated with hydrodynamic performance and ecological niche. J. Morphol. 267, 1284–1294. doi: 10.1002/jmor.10474
Yamato, M., and Pyenson, N. D. (2015). Early development and orientation of the acoustic funnel provides insight into the evolution of sound reception pathways in cetaceans. PLoS ONE 10:e0118582. doi: 10.1371/journal.pone.0118582
Keywords: Cetacea, dentition, filter feeding, fossils, Mammalia, Mysticeti, tooth loss
Citation: Peredo CM, Pyenson ND and Boersma AT (2017) Decoupling Tooth Loss from the Evolution of Baleen in Whales. Front. Mar. Sci. 4:67. doi: 10.3389/fmars.2017.00067
Received: 15 December 2016; Accepted: 24 February 2017;
Published: 13 March 2017.
Edited by:
Lars Bejder, Murdoch University, AustraliaReviewed by:
Alexander J. Werth, Hampden–Sydney College, USANuno Queiroz, CIBIO/InBIO, University of Porto, Portugal
Copyright © 2017 Peredo, Pyenson and Boersma. This is an open-access article distributed under the terms of the Creative Commons Attribution License (CC BY). The use, distribution or reproduction in other forums is permitted, provided the original author(s) or licensor are credited and that the original publication in this journal is cited, in accordance with accepted academic practice. No use, distribution or reproduction is permitted which does not comply with these terms.
*Correspondence: Carlos Mauricio Peredo, Y3BlcmVkb0BtYXNvbmxpdmUuZ211LmVkdQ==