- Seagrass Ecosystem Research Group, College of Science, Swansea University, Swansea, UK
There exists increasing evidence that top-down ecological processes, such as herbivory are key in controlling marine ecosystems and their community structure. Herbivory has the potential to be altered by numerous environmental and ecological factors that operate at a variety of temporal and spatial scales, one such spatial factor is the influence of the marine landscape. We know little about how ecological processes, such as herbivory change throughout the marine landscape and how the effects of these processes cascade. This is because most landscape scale studies observe species richness and abundance patterns. In terrestrial systems the landscape is well documented to influence ecological processes, but empirical evidence of this is limited in marine systems. In tropical seagrass meadows direct herbivory by parrotfish can be readily observed due to the clear hemispherical bite marks they leave on the seagrass. As with herbivory in other systems, this leaf consumption is thought to assist with leaf turnover, positively influencing leaf growth. Changes in its rate and extent are therefore likely to influence the characteristics of the plant. The faunal communities of seagrass meadows alter with respect to changes in the landscape, particularly with respect to connectivity to adjacent habitats. It might therefore be expected that a key ecological process, such as herbivory will change with respect to habitat configuration and have cascading impacts upon the status of the seagrass. In the present study we examined indirect evidence of parrotfish grazing throughout the marine landscape and assessed this relative to plant condition. Seagrasses in locations of close proximity to mangroves were found to have double the amount of parrotfish grazing than sites away from mangroves. Evidence of herbivory was also found to be strongly and significantly negatively correlated to the abundance of plant attached epicover. The decreased epicover in the presence of elevated herbivory suggests increased leaf turnover. These results indicate that seagrass may have higher levels of ecosystem resilience in the presence of mangroves. Our research highlights how ecological processes can change throughout the marine landscape with cascade impacts on the resilience of the system.
Introduction
To understand how ecosystems function and predict how communities might shift in response to anthropogenic impacts and/or management interventions, we require better knowledge of the relative influences of different biological and physical factors and processes on community structure (Gilby et al., 2016). Top-down ecological processes, such as herbivory have been shown to control ecosystems and their community structure, and there is increasing evidence that such control is much more pronounced in aquatic relative to terrestrial environments (Shurin et al., 2006). In marine systems it is estimated that herbivores graze up to 70% of global benthic primary production, and removing such species can lead to phase-shifts in tropical habitats (Poore et al., 2012; Vergés et al., 2014). Although we have increasing knowledge of how these ecological processes are critical in different ecosystems (e.g., kelp, coral reef, and seagrass), we have a limited understanding of how landscape may alter this (Olds et al., 2012). We also know little about the ecological consequences of any changes at spatial scales and what they mean in terms of the resilience of systems and their environmental management (Mumby and Hastings, 2008; Olds et al., 2012, 2016).
The composition of habitat types and their spatial arrangement within a landscape has the capacity to be a major source of influence upon ecological processes (Dunning et al., 1992). For example, pollinator visitation has been found to be substantially higher in small clover patches surrounded by bare-ground than in larger patches embedded within grass (Diekötter et al., 2007). In fragmented forests, parasitism on the forest tent caterpillar altered depending on the proportion of forested to unforested land (Roland and Taylor, 1997). The spatial arrangement of habitats across the marine seascape also profoundly alters marine faunal communities in terms of their behavior, resource use, abundance, and diversity (Nagelkerken, 2009; Olds et al., 2016). These differences can result in significant changes in floral and faunal communities and potentially alter their functional ecology (Mumby and Hastings, 2008; Pagès et al., 2014). This may be particularly the case when one habitat may be absent from the configuration in a marine seascape.
In tropical seagrass meadows herbivory is a key ecological process. High rates of herbivory have been found to confer resilience by causing a high turnover of plant tissue, resulting in reduced buildup of epiphytic material (Christianen et al., 2012). This herbivory also results in plants increasing their aboveground net production (Valentine et al., 1997; Duarte and Chiscano, 1999). Processes that influence the rate of this herbivory therefore have the potential to result in cascading feedbacks to the wider seagrass meadow (Maxwell et al., 2016). Seagrass meadows are a globally important ecosystem under significant anthropogenic threat (Waycott et al., 2009). The management of these ecosystems requires a better understanding of the functional ecological processes that ultimately confer resilience upon it (Unsworth et al., 2015) and their interactions throughout the seascape.
Tropical seagrass meadows exist in connectivity with reef and mangrove systems, which results in the movement of diverse communities of animals at different ontogenetic life stages throughout this seascape (Nagelkerken, 2009). The relative availability of mangrove and reef environments will therefore potentially impact upon the value of seagrass meadows in providing this corridor habitat for fish and invertebrates species of different ages that utilize these diverse habitats (Skilleter et al., 2005; Unsworth et al., 2008). Knowledge of this is of particular value for managing functionally important species, such as herbivores.
The present research examined the relationship between seagrass herbivory by Parrotfish (Scarids) (a key ecological process) and the spatial habitat arrangement within the tropical marine seascape. The present study had the following a priori null hypotheses: (1) Evidence of seagrass herbivory does not vary with respect to proximity to mangroves, (2) Herbivory does not reduce the build-up of attached epiphytic material on seagrass.
Materials and Methods
Data on seagrass and associated Scarid herbivory was collected at six seagrass meadows in La Parguera Bay, Puerto Rico in January 2016. Seagrass meadows at San Cristobal, Laurel Cay and White Pole were all on coral cays containing no mangrove whereas seagrass sites at Collado, Majimo and Enrique Cay were all on coral cays containing abundant mangroves (see Figure 1). All sites were of a consistent depth and dominated by the seagrass Thalassia testudinum.
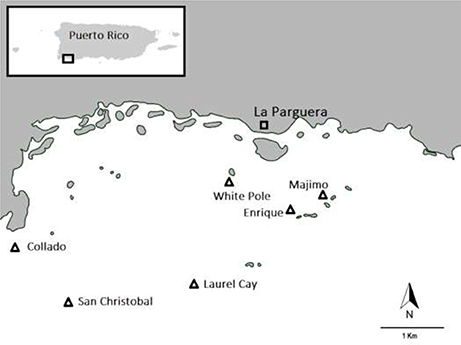
Figure 1. Location of seagrass sites in La Parguera Bay, Puerto Rico that were examined in order to quantify scarid herbivory.
Seagrass data was collected along three 25 m transects that were laid out at each site, perpendicular to the coral cay or mangrove (15 m distance from the island or mangrove). Six 0.5 m2 quadrats were placed along each transect starting at 0 m. Within each quadrat seagrass was assessed for percentage cover, species composition, percentage epicover, percentage algal cover and canopy height using standard seagrass protocols (McKenzie et al., 2001). Our study design resulted in the collection of data from 108 quadrats spread over 18 independent transects across 6 independent sites.
To assess herbivory, the percentage of shoots within each quadrat containing scarid bite marks was estimated. Scarid bite marks are easily distinguished on seagrass leaves due to their hemispherical shape (Kirsch et al., 2002; Unsworth et al., 2007). The size of scarid bites was determined in all quadrats by measuring the width (using calipers) of the two bites closest to the bottom left hand corner of the quadrat, nearest the transect tape.
Statistical Analysis
A two-way nested ANOVA was conducted to assess the effect of seascape and site (site nested in seascape) on the percentage of leaves bitten, percentage seagrass cover, and percentage epicover. All data (expect bite size) was found to initially fail a key assumption of ANOVA (homogeneity of variance). Data was therefore transformed in order to obtain homogeneity of variance. Some data was ArcSin transformed (% seagrass cover, % algae cover and % leaves bitten). Data on epicover was square root transformed, whilst canopy height was transformed using a natural log.
In order to examine the potential influence of herbivory on attached epicover a Partial Least Squares Regression (PLS) model was developed in Minitab (version 17) (Carrascal et al., 2009; Haapkylä et al., 2011). PLS was used to investigate which of the observed seagrass meadow variables correlated most with epicover. PLS regression is particularly suited to incidences when there is multi co-linearity among variables (Carrascal et al., 2009). The study had variables that were co-linear. This technique has commonly been used to analyse a range of ecological datasets (Rasheed and Unsworth, 2011).
The % epicover on the seagrass was analyzed against five variables (% seagrass cover, % algal cover, seagrass canopy, bite size, % shoots bitten). PLS was conducted in a step-wise manner that allowed for the successive removal of variables that did not contribute to the model, enabling the strongest possible PLS model to be created. The PLS analysis also calculated a predicted residual sum of squares (PRESS) following cross-validation.
Results
Seagrass percentage cover ranged from 1% cover to 100% cover and was highly variable across the 108 quadrats with mean % cover of 57.2 ± 27.3 (Table 1). Although the mean seagrass cover near to mangrove was higher (77.6 ± 18.4) than far from mangrove (37.0 ± 18.3) the high variability meant there was no significant influence of mangrove proximity [F(1, 107) = 2.1, p = 0.22] (Table 2). This pattern was consistent across sites (Table 1). The seagrass had a taller canopy [F(1, 107) = 30.3, p = 0.005] at sites closer to mangrove, creating more habitat (see Table 1), however there were significant [F(1, 107) = 4.0, p = 0.005] inter-site interactions. Seagrass at sites close to mangrove had significantly [F(1, 107) = 153.7, p = 0.000] lower levels of epicover (phytic and biont) (13.3 ± 11.8%) than sites away from mangrove (40.7 ± 17.8%). This pattern was consistent across sites (Table 1).
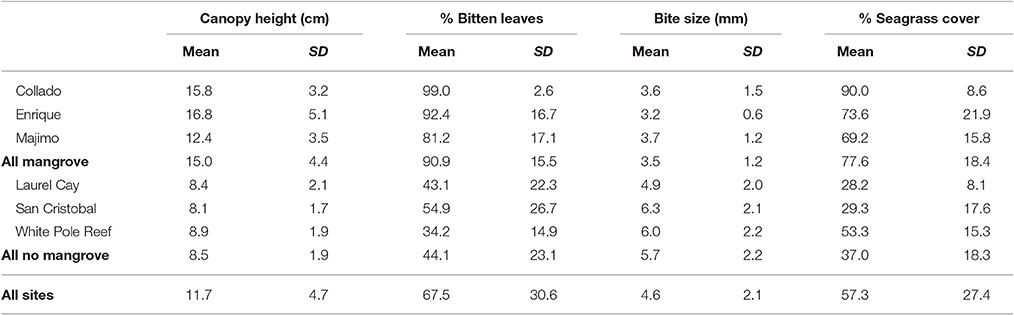
Table 1. Seagrass (Thalassia testudinum) metrics (mean ± SD) sampled in quadrats at six sites of varying proximity to mangroves in La Parguera Bay, Puerto Rico.
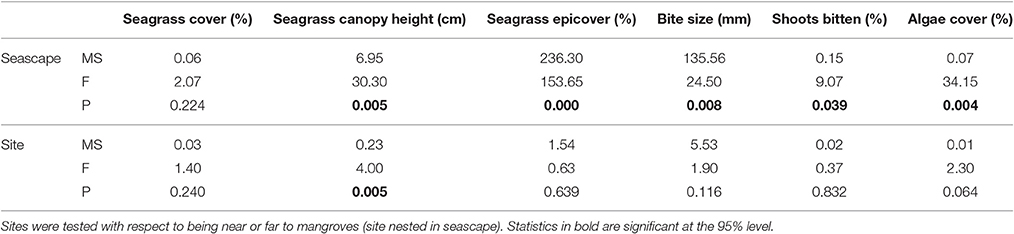
Table 2. Analysis of variance (nested) statistics for seagrass (Thalassia testudinum) metrics sampled in quadrats at six sites of varying proximity to mangroves (seascape) in La Parguera Bay, Puerto Rico.
At sites near to mangrove, seagrass contained a significantly [F(1, 107) = 9.1, p = 0.039] higher proportion of shoots displaying signs of scarid bite marks than shoots at sites far from mangrove. The size of these were significantly smaller [F(1, 107) = 24.5, p = 0.008] (Table 2) at seagrass sites near to mangrove (3.5 ± 1.2 mm) relative to those on seagrass further from a mangrove forest (5.7 ± 2.2 mm). This pattern was also consistent across sites (Table 1). Bite sizes varied between 1 and 13 mm at sites far from mangrove and 1–9 mm at sites close to mangroves.
The percentage of seagrass shoots showing signs of herbivory was found to significantly (P < 0.001, Pearson's coefficient = −0.787) and negatively correlate with the percentage of attached epicover (Figure 2). Given the close colinearity of the potential alternative correlates of epicover (Table 3) a Partial Least Squares (PLS) model was developed to further examine this correlation. 54% of the seagrass epicover variability was explained in PLS by the % of bitten seagrass shoots (correlation coefficient = −0.63) and canopy height (correlation coefficient = −0.15). After PLS cross validation (i.e., randomly removing 25 data points at a time then re-running the PLS analysis) this correlation remained significant and explained variability reduced only slightly to 52% (pred R-Sq), statistically indicating that the relationship was not driven exclusively by a small proportion of data points.
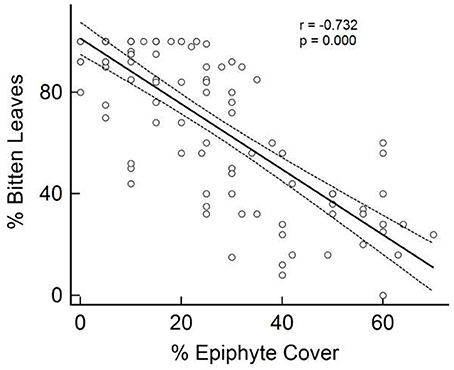
Figure 2. Percentage of seagrass (Thalassia testudinum) leaves observed to show signs of parrotfish bites in relation to observed coverage in epiphytic material. Seagrass sampled in quadrats at six sites of varying proximity to mangroves in La Parguera Bay, Puerto Rico.
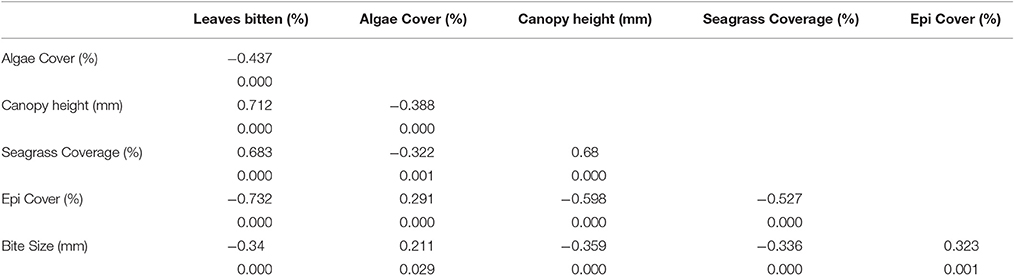
Table 3. Correlation coefficients and probability values between metrics of seagrass meadows sampled in quadrats at six sites of varying proximity to mangroves (seascape) in La Parguera Bay, Puerto Rico.
Discussion
Tropical marine landscapes are characterized by highly variable habitat configurations (Nagelkerken, 2009). This variability is further enhanced by anthropogenic loss of habitats across the tropical seas (Orth et al., 2006; Duke et al., 2007). In the present study we provide evidence that the position in this habitat configuration influences an important ecological process, in this case herbivory, leading to potential cascading impacts upon the epiphytic and epibiont growth. Such growth may reduce the resilience of the seagrass to stressors.
Seagrasses in locations of close proximity to mangroves had approximately double the evidence of scarid grazing compared to sites away from mangroves. This finding mirrors data from other areas of the Caribbean where increased scarid abundance and species richness has been recorded in seagrass adjacent to mangroves relative to seagrass without (Nagelkerken et al., 2001).
Evidence of enhanced herbivory at sites close to mangroves is proposed to be the result of an increased presence of parrotfish using seagrass as a nursery habitat. Our finding that bite marks on seagrass close to mangroves were smaller in size supports this premise, presumably as a result of the higher abundance of juvenile fish. Previous studies on tropical seagrasses find the mangrove-seagrass continuum to provide a corridor for diel and tidal feeding migrations of juvenile fish in shallow sheltered habitats (Nagelkerken et al., 2001; Unsworth et al., 2009). Many fish typically spend time in mangroves during the day and move into nearby seagrass meadows at night to feed upon leaves (Igulu et al., 2015).
The present study found strong correlative evidence that increasing presence of herbivory is associated with reduced epicover suggesting increased leaf turnover and higher resultant levels of resistance to potential impacts (e.g., poor water quality) (Unsworth et al., 2015). Attached flora or fauna on seagrass leaves has the potential to reduce photosynthetic rate due to shading (Oh et al., 2009). The present study recorded that such attached material can cover over 60% of the leaf area. This shading reduces the capacity of the plant to resist the effect of any further shading from problems, such as periods of poor water quality (Unsworth et al., 2015). We recorded a significant negative correlation between the percentage of leaves bitten and total epiphyte and epibiont coverage. We suggest that the increased removal rate of leaf material may allow quicker turnover rates of plant biomass, leaving less time for epiphytes to colonize the leaf surface (Christianen et al., 2012). This would be due to a compensatory growth response of the plants to herbivory. Such a response has been recorded in many plant species (McNaughton, 1983). Studies on seagrass fish herbivory have previously shown increased seagrass shoot density after herbivory (Valentine et al., 1997; Heck and Valentine, 2006). Research on Green Turtle grazing has found the presence of epiphytic material on seagrass leaves to be associated with high nutrient loads that could cover seagrass meadows if grazers are not present (Christianen et al., 2012).
Although the present study shows marked differences between the amounts of evidence of herbivory at sites of differing distance from mangrove, the evidence is indirect and temporally restricted, therefore we provide a word of caution with respect to extrapolating the findings wider. The findings of this study need to be further examined by quantifying the herbivory directly in terms of the rate of seagrass consumption relative to growth by conducting tethering experiments and cage manipulations.
The present study demonstrates a strong case for greater integration of spatial ecology into ecosystem-based management (Foley et al., 2010; Massol et al., 2011; Olds et al., 2016) and suggests that by explicitly incorporating habitat configuration into conservation planning, we may be better placed to ensure the long-term resilience of such tropical ecosystems (Olds et al., 2012).
In conclusion, we find seagrass meadows in proximity to mangroves have double the amount of evidence of herbivory than those meadows far from mangrove. We use correlative evidence to hypothesize that mangroves play a significant functional role in decreasing levels of attached flora and fauna on seagrass leaves enhancing ecosystem resilience. Our research highlights how ecological processes can vary throughout the marine landscape with potential cascade impacts. This study also highlights the need for spatial planning to consider how the configuration of habitats influences ecosystem resilience.
Author Contributions
All authors contributed equally to the planning and project development. KS, RM, WB, and NH conducted all field work. All authors contributed equally to analysis and manuscript preparation.
Funding
The study was self funded.
Conflict of Interest Statement
The authors declare that the research was conducted in the absence of any commercial or financial relationships that could be construed as a potential conflict of interest.
References
Carrascal, L. M., Galvan, I., and Gordo, O. (2009). Partial least squares regression as an alternative to current regression methods used in ecology. Oikos 118, 681–690. doi: 10.1111/j.1600-0706.2008.16881.x
Christianen, M. J. A., Govers, L. L., Bouma, T. J., Kiswara, W., Roelofs, J. G. M., Lamers, L. P. M., et al. (2012). Marine megaherbivore grazing may increase seagrass tolerance to high nutrient loads. J. Ecol. 100, 546–560. doi: 10.1111/j.1365-2745.2011.01900.x
Diekötter, T., Haynes, K. J., Mazeffa, D., and Crist, T. O. (2007). Direct and indirect effects of habitat area and matrix composition on species interactions among flower-visiting insects. Oikos 116, 1588–1598. doi: 10.1111/j.0030-1299.2007.15963.x
Duarte, C. M., and Chiscano, C. L. (1999). Seagrass biomass and production: a reassessment. Aquat. Bot. 65, 159–174. doi: 10.1016/S0304-3770(99)00038-8
Duke, N. C., Meynecke, J.-O., Dittmann, S., Ellison, A. M., Anger, K., Berger, U., et al. (2007). A world without mangroves? Science 317, 41–42. doi: 10.1126/science.317.5834.41b
Dunning, J. B., Danielson, B. J., and Pulliam, H. R. (1992). Ecological processes that affect populations in complex landscapes. Oikos 65, 169–175. doi: 10.2307/3544901
Foley, M. M., Halpern, B. S., Micheli, F., Armsby, M. H., Caldwell, M. R., Crainb, C. M., et al. (2010). Guiding ecological principles for marine spatial planning. Mar. Policy 34, 955–966. doi: 10.1016/j.marpol.2010.02.001
Gilby, B. L., Tibbetts, I. R., Olds, A. D., Maxwell, P. S., and Stevens, T. (2016). Seascape context and predators override water quality effects on inshore coral reef fish communities. Coral Reefs 35, 979–990. doi: 10.1007/s00338-016-1449-5
Haapkylä, J., Unsworth, R. K. F., Flavell, M., Bourne, D. G., Schaffelke, B., and Willis, B. L. (2011). Seasonal rainfall and runoff promote coral disease on an inshore reef. PLoS ONE 6:e16893. doi: 10.1371/journal.pone.0016893
Heck, K. L., and Valentine, J. F. (2006). Plant-herbivore interactions in seagrass meadows. J. Exp. Mar. Biol. Ecol. 330, 420–436. doi: 10.1016/j.jembe.2005.12.044
Igulu, M. M., Nagelkerken, I., Dorenbosch, M., Grol, M. G. G., Harborne, A. R., Kimirei, I. A., et al. (2015). Mangrove habitat use by juvenile reef fish: meta-analysis reveals that tidal regime matters more than biogeographic region. PLoS ONE 9:e114715. doi: 10.1371/journal.pone.0114715
Kirsch, K. D., Valentine, J. F., and Heck, K. L. (2002). Parrotfish grazing on turtlegrass Thalassia testudinum: evidence for the importance of seagrass consumption in food web dynamics of the Florida Keys National Marine Sanctuary. Mar. Ecol. Prog. Ser. 227, 71–85. doi: 10.3354/meps227071
Massol, F., Gravel, D., Mouquet, N., Cadotte, M. W., Fukami, T., and Leibold, M. A. (2011). Linking community and ecosystem dynamics through spatial ecology. Ecol. Lett. 14, 313–323. doi: 10.1111/j.1461-0248.2011.01588.x
Maxwell, P. S., Eklöf, J. S., van Katwijk, M. M., O'Brien, K. R., de la Torre-Castro, M., Boström, C., et al. (2016). The fundamental role of ecological feedback mechanisms for the adaptive management of seagrass ecosystems–a review. Biol. Rev. Camb. Philos. Soc. doi: 10.1111/brv.12294. [Epub ahead of print].
McKenzie, L. J., Campbell, S. J., and Roder, C. A. (2001). Seagrass-Watch: Manual for Mapping & Monitoring Seagrass Resources by Community (Citizen) Volunteers. Cairns: Queensland Fisheries Service, Department of Primary Industries.
McNaughton, S. J. (1983). Compensatory plant-growth as a response to herbivory. Oikos 40, 329–336. doi: 10.2307/3544305
Mumby, P. J., and Hastings, A. (2008). The impact of ecosystem connectivity on coral reef resilience. J. Appl. Ecol. 45, 854–862. doi: 10.1111/j.1365-2664.2008.01459.x
Nagelkerken, I. (ed.). (2009). Ecological Connectivity amoung Tropical Coastal Ecosystems. Dordrecht: Springer.
Nagelkerken, I., Kleijnen, S., Klop, T., van den Brand, R. A. C. J., de la Moriniere, E. C., and van der Velde, G. (2001). Dependence of Caribbean reef fishes on mangroves and seagrass beds as nursery habitats: a comparison of fish faunas between bays with and without mangroves/seagrass beds. Mar. Ecol. Prog. Ser. 214, 225–235. doi: 10.3354/meps214225
Oh, M.-H., Kang, D. W., Kim, T. H., Moon, Y.-H., Moon, B. Y., Chung, I. K., et al. (2009). Effects of epiphytic load on the photosynthetic performance of a seagrass, Zostera marina, monitored in vivo by chlorophyll fluorescence imaging. J. Plant Biol. 52, 171–175. doi: 10.1007/s12374-009-9010-5
Olds, A. D., Connolly, R. M., Pitt, K. A., Pittman, S. J., Maxwell, P. S., Huijbers, C. M., et al. (2016). Quantifying the conservation value of seascape connectivity: a global synthesis. Glob. Ecol. Biogeogr. 25, 3–15. doi: 10.1111/geb.12388
Olds, A. D., Pitt, K. A., Maxwell, P. S., and Connolly, R. M. (2012). Synergistic effects of reserves and connectivity on ecological resilience. J. Appl. Ecol. 49, 1195–1203. doi: 10.1111/jpe.12002
Orth, R. J., Carruthers, T. J. B., Dennison, W. C., Duarte, C. M., Fourqurean, J. W., Heck, K. L. Jr., et al. (2006). A global crisis for seagrass ecosystems. Bioscience 56, 987–996. doi: 10.1641/0006-3568(2006)56[987:AGCFSE]2.0.CO;2
Pagès, J. F., Gera, A., Romero, J., and Alcoverro, T. (2014). Matrix composition and patch edges influence plant–herbivore interactions in marine landscapes. Funct. Ecol. 28, 1440–1448. doi: 10.1111/1365-2435.12286
Poore, A. G. B., Campbell, A. H., Coleman, R. A., Edgar, G. J., Jormalainen, V., Reynolds, P. L., et al. (2012). Global patterns in the impact of marine herbivores on benthic primary producers. Ecol. Lett. 15, 912–922. doi: 10.1111/j.1461-0248.2012.01804.x
Rasheed, M. A., and Unsworth, R. K. F. (2011). Long-term climate associated dynamics of a tropical seagrass meadow: implications for the future. Mar. Ecol. Prog. Ser. 422, 93–103. doi: 10.3354/meps08925
Roland, J., and Taylor, P. D. (1997). Insect parasitoid species respond to forest structure at different spatial scales. Nature 386, 710–713. doi: 10.1038/386710a0
Shurin, J. B., Gruner, D. S., and Hillebrand, H. (2006). All wet or dried up? Real differences between aquatic and terrestrial food webs. Proc. R. Soc. Lond. B Biol. Sci. 273, 1–9. doi: 10.1098/rspb.2005.3377
Skilleter, G. A., Olds, A., Loneragan, N. R., and Zharikov, Y. (2005). The value of patches of intertidal seagrass to prawns depends on their proximity to mangroves. Mar. Biol. 147, 353–365. doi: 10.1007/s00227-005-1580-2
Unsworth, R. K. F., Collier, C. J., Waycott, M., McKenzie, L. J., and Cullen-Unsworth, L. C. (2015). A framework for the resilience of seagrass ecosystems. Mar. Pollut. Bull. 100, 34–46. doi: 10.1016/j.marpolbul.2015.08.016
Unsworth, R. K. F., De León, P. S., Garrard, S. L., Jompa, J., Smith, D. J., and Bell, J. J. (2008). High connectivity of Indo-Pacific seagrass fish assemblages with mangrove and coral reef habitats. Mar. Ecol. Prog. Ser. 353, 213–224. doi: 10.3354/meps07199
Unsworth, R. K. F., Garrard, S. L., De León, P. S., Cullen, L. C., Smith, D. J., Sloman, K. A., et al. (2009). Structuring of Indo-Pacific fish assemblages along the mangrove-seagrass continuum. Aquat. Biol. 5, 85–95. doi: 10.3354/ab00139
Unsworth, R. K. F., Taylor, J. D., Powell, A., Bell, J. J., and Smith, D. J. (2007). The contribution of parrotfish (scarid) herbivory to seagrass ecosystem dynamics in the Indo-Pacific. Estuar. Coast. Shelf Sci. 74, 53–62. doi: 10.1016/j.ecss.2007.04.001
Valentine, J. F., Heck, K. L., Busby, J., and Webb, D. (1997). Experimental evidence that herbivory increases shoot density and productivity in a subtropical turtlegrass (Thalassia testudinum) meadow. Oecologia 112, 193–200. doi: 10.1007/s004420050300
Vergés, A., Steinberg, P. D., Hay, M. E., Poore, A. G. B., Campbell, A. H., Ballesteros, E., et al. (2014). The tropicalization of temperate marine ecosystems: climate-mediated changes in herbivory and community phase shifts. Proc. R. Soc. Lond. B Biol. Sci. 281:20140846. doi: 10.1098/rspb.2014.0846
Keywords: resilience, grazing, feedbacks, herbivory, seagrass, parrotfish, Thalassia
Citation: Swindells KL, Murdoch RJ, Bazen WD, Harman NW and Unsworth RKF (2017) Habitat Configuration Alters Herbivory across the Tropical Seascape. Front. Mar. Sci. 4:48. doi: 10.3389/fmars.2017.00048
Received: 17 July 2016; Accepted: 10 February 2017;
Published: 28 February 2017.
Edited by:
Xabier Irigoien, King Abdullah University of Science and Technology, Saudi ArabiaReviewed by:
Rafael Perez-Dominguez, Office for National Statistics, UKGuillem Chust, AZTI-Tecnalia, Spain
Copyright © 2017 Swindells, Murdoch, Bazen, Harman and Unsworth. This is an open-access article distributed under the terms of the Creative Commons Attribution License (CC BY). The use, distribution or reproduction in other forums is permitted, provided the original author(s) or licensor are credited and that the original publication in this journal is cited, in accordance with accepted academic practice. No use, distribution or reproduction is permitted which does not comply with these terms.
*Correspondence: Richard K. F. Unsworth, r.k.f.unsworth@swansea.ac.uk