- 1Division of Life Science, Hong Kong University of Science and Technology, Hong Kong, China
- 2South China Sea Institute of Planning and Environmental Research, State Oceanic Administration, Guangzhou, China
- 3Department of Earth and Ocean Sciences, University of British Columbia, Vancouver, BC, Canada
Diatoms are often a major food source for zooplankton and contribute significantly to vertical POC flux through sinking of dead cells, aggregates and zooplankton fecal pellets. The silica content of diatoms varies among different species and within a species growing under different environmental conditions and physiological status. However, to-date there has been no investigation of the effect of diatom silica content on zooplankton grazing, growth and reproduction. We conducted a series of experiments using the diatom Thalassiosira weissflogii with different silica content achieved by growth under high and low light and these cells were fed to a copepod, Parvocalanus crassirostris. Our results show that this copepod strongly preferred cells with low silica content over high silica-containing cells, with the ingestion rate on low silica diatoms being 2–3 times higher than that on high silica diatoms. Fecal pellet production rate was significantly higher for copepods feeding on highly silicified cells. Furthermore, copepod growth rate (measured as an increase in wet weight), egg production rate and hatching success were all severely compromised under a high silica diatom diet. Females of P. crassirostris feeding on a low silica diatom diet produced an average 90 eggs during a 1 day incubation, while those fed with high silica diatoms produced only 11 eggs per day. Similarly, the hatching success during a 3-day period was 82 ± 17 and 23 ± 36% for the low and high silica diatom treatments, respectively, with zero success observed in ~65% of the females feeding on high Si diatoms. Our findings have important ecological implications for the biological pump and may alter our previous view of the role of diatoms in planktonic food webs and the role of the degree of silicification in controlling amount of POC flux to deeper waters.
Introduction
Carbon uptake by marine phytoplankton, and its export as organic matter to the interior of the ocean (i.e., the “biological pump”), lowers the partial pressure of carbon dioxide (pCO2) in the upper ocean and facilitates the downward diffusive flux of atmospheric CO2. Among different groups of phytoplankton, diatoms are one of the most important; they generate about as much organic carbon as all the terrestrial rainforests combined (Nelson et al., 1995; Field et al., 1998). However, unlike much of the carbon generated by trees, the organic carbon produced by diatoms is consumed rapidly and serves as a food and energy source for marine food webs. Diatoms dominate the export flux of carbon and contribute significantly to the long-term sequestration of atmospheric CO2 in the ocean's interior (Smetacek, 1999).
Diatoms are the most diverse group of phytoplankton, ranging in size from a few micrometers to a few millimeters and exist either as single cells or chains of cells (Kooistra et al., 2007). Diatoms tend to dominate phytoplankton communities in well-mixed coastal and upwelling regions, as well as along the sea-ice edge, where sufficient light, inorganic nitrogen, phosphorus, silicate, and trace elements are available to sustain their growth (Armbrust, 2009). They form spring blooms in the temperate and polar seas and after artificial or natural iron inputs in high nitrate low chlorophyll (HNLC) regions (Ducklow and Harris, 1993; Boyd et al., 2007). Diatom blooms are also observed in mesoscale and sub-mesoscale eddies in oligotrophic oceans, which play a very important role in bringing nutrients from nutrient-rich deep waters to the euphotic layer (Benitez-Nelson et al., 2007; Benitez-Nelson and McGillicuddy, 2008). Pigment and microscopic analyses of samples after the passing of the Hawaiian Lee cyclone revealed that the bloom within the eddy was dominated by large diatoms and they accounted for almost 85% of the total phytoplankton biomass (Brown et al., 2008; Rii et al., 2008).
All diatoms have siliceous cell walls (frustules) made of polymerized silicic acid (biogenic silica, bSi), but there is considerable variation in the Si content among different species (Parsons et al., 1961; Harrison et al., 1977; Paasche, 1980; Brzezinski, 1985; Conley et al., 1989). Within a given species, the bSi content can vary by up to an order of magnitude (Taylor, 1985; Claquin et al., 2002) and is a function of cell size (Paasche, 1973; Durbin, 1977) the cell cycle (Brzezinski et al., 1990) or sexual reproduction (Harrison et al., 1977; D'Alelio et al., 2010), as well as due to external factors, such as light, temperature, salinity, nutrients, and trace metals (Furnas, 1978; Martin-Jézéquel et al., 2000; Claquin et al., 2002; Vrieling et al., 2007). For example, Saito and Tsuda (2003) reported that diatoms in the Oyashio region off the east coast of Japan, increased their Si:N ratio (i.e., their Si content) during the spring bloom, possibly due to slow growth induced by light limitation resulting from self-shading due to high cell numbers during the bloom.
One important aspect in the diatom—copepod food chain that has not been investigated is whether the variation in the level of diatom silicification affects ingestion by copepods and the subsequent biological, physiological and biogeochemical implications for carbon flux to depth. While one recent study (Pondaven et al., 2007) showed a grazing-induced increase in the cell wall silicification of a diatom, which can be viewed as an adaptive reaction to high grazing pressure, there are no direct studies on the opposite effect, i.e., how do diatoms with different silica content affect copepod grazing. Smetacek (1999), and others (e.g., Raven and Waite, 2004) speculated that thick shells will reduce the number of grazers that are capable of consuming diatoms, but no direct experimental support has been reported so far.
Our objective was to test the hypothesis that the silica content of a diatom affects the feeding of copepods, and consequently their growth and reproduction. In order to exclude other factors that can affect zooplankton feeding selectivity, such as cell size, we used a single diatom species, Thalassiosira weissflogii, with high and low silica content achieved by growth under low and high light and the calanoid copepod, Parvocalanus crassirostris, to investigate how a copepod responds to a diatom with different cellular silica content.
Materials and Methods
Culturing T. weissflogii to Produce Different bSi Content
Initially, we tried to generate low and high bSi T. weissflogii cells by growing them in Si replete (f/2) and Si deplete (f/2-Si) media, but surprisingly there was no significant difference in the Si content (data not shown). We then used different light levels to produce fast and slow growing cells that generated T. weissflogii with different silica content. Cultures were maintained in f/2 medium at 23.5°C and 14:10 L:D cycle under four different light intensities (17, 66, 116, and 199 μmol photons m−2 s−1; measured with a Biospherical Instruments Inc. Model # QSL-100 light meter) achieved by using neutral density screening and adjusting distances from the light source. Cultures were acclimated to the light intensities for least 5 cell divisions before the experiment was initiated. Cell concentrations were monitored daily and cell volume was determined at the end of the experiment using a Coulter Counter (Beckman Coulter, Z2 Coulter Particle Count and Size Analyzer). Duplicate 20 ml samples were taken every day (every 2 days for the culture at the lowest light level because it grew much slower) for biogenic silica analysis. bSi was measured on day 6 after transferring to new medium for the lowest light level and on day 4 for the remaining 3 light levels. Based on the bSi data, cultures with 116 and 17 μmol photons m−2 s−1 were used for further grazing experiments and hereafter referred to as high and low light cells, respectively. Cellular carbon and nitrogen content was also measured for diatoms grown under high and low light by filtering 20–30 ml of culture onto a pre-combusted GF/C filter, and particulate organic carbon and nitrogen was determined with a Perkin Elmer model 2400 CHNS elemental analyzer.
Biogenic Silica Measurement
A modified version of Paasche (1980) was used to measure bSi. Cells were collected on a 1 μm polycarbonate filter (47 mm diameter) and washed with 10 ml autoclaved seawater and 0.01 M HCl during filtration to remove the intercellular silicate pools. The folded filter was immediately placed into a 15 ml polypropylene tube and stored at −80°C. Hydrolysis was carried out when 4 ml 0.2 M NaOH was added and digested at 98°C for 40 min. After cooling in a water bath, 1.0 ml of 1 M HCl was added to each tube to neutralize the sample and the concentration of silicic acid was analyzed colorimetrically following Grasshoff et al. (1999). bSi per surface area was calculated by assuming a spherical shape using the ESD estimated by the Coulter Counter.
Copepod Grazing Experiments
Copepods were collected by a net tow with 200 μm mesh from the pier at Port Shelter, Hong Kong. The dominant species, Parvocalanus crassirostris, was used as the grazer; females were selected under a dissecting microscope, rinsed at least three times to remove other prey and placed in freshly filtered seawater for 1 h before being transferred to the feeding experiments. Two feeding experiments were conducted. In the first experiment, 10 copepods were added to each triplicate 600 ml PC bottle (i.e., 1 copepod per 60 ml) and T. weissflogii cells with high and low bSi (i.e., high and low light cells) were added at approximately the same density (185 cells ml−1; i.e., 1 copepod with 11,100 cells). Duplicate bottles with the same density of diatoms but no copepods, were set up as the control. The second experiment was also conducted in triplicate with the same no copepods control. Thirty copepods were added to 1.2 L bottles (i.e., 1 copepod per 40 ml) and the prey concentration of high and low silica cells was approximately 385 cells ml−1 (i.e., 1 copepod with 15,400 cells). The incubation lasted for 5 h for both experiments with gentle manual stirring done hourly and concentrations of T. weissflogii were determined at the beginning and end of the incubation by a Coulter Counter (Beckman Coulter, Z2 Coulter Particle Count and Size Analyzer). Duplicate aliquots of culture were also filtered onto a 1.0 μm, 47 mm GE polycarbonate membrane filter and stored in polymethylpentene centrifuge tubes in a −80°C freezer for bSi analysis. Copepod fecal pellet production rate was only measured in the second experiment.
Copepod Growth, Egg Production, and Hatching Success
A number of active females of Parvocalanus crassirostris were collected from the same location as used in the previous feeding experiments and were sorted under a dissecting microscope soon after the tow for initial body weight measurements. They were then maintained in a 2-L flask at 24°C at two different light levels as described above. High and low silica cells were added as food at a high concentration of 5000 cells ml−1. Duplicate 10 ml samples of the diatom cultures were filtered onto a 2 μm PC membrane for initial bSi analysis. The copepods were transferred to new containers with the same prey concentration (5000 cells ml−1) and environmental conditions to ensure that the prey concentration was nearly constant during the growth experiment. To compare the body condition of P. crassirostris grown on cells with different silica content, the wet weights of P. crassirostris were measured after 2 and 3 days of incubation with high and low silica prey. A number of copepods were pooled together on a pre-weighted membrane and weighed by an electronic balance.
For copepod egg production experiment, a total of 18 females were incubated in three 6-well plates, with 1 copepods in each well, containing the same culture medium (15 ml) and diatom food concentration. The plates were placed in the same previous high and low light for 24 h and the fitness and activity of the copepods was monitored during the incubation. At the end of the incubation, the copepod's body wet weight was determined and the number of eggs and hatched egg shells in the plates were counted under an inverted microscope. In order to measure the egg hatching rate, the eggs remaining in the plates were incubated for a further 72 h.
Statistical Analysis
Statistical analyses were performed with SigmaPlot 12.5 (Systat Software). Student's t-test (2-tailed) and one-way analysis of variance (ANOVA) were performed with significance levels of p < 0.05.
Results
Diatom Cellular Silica Content Affects Copepod Grazing Rate
T. weissflogii grown at the lowest light contained nearly three times higher silica than cells grown in higher light (Figure 1). No significant difference (one-way ANOVA) in cell volume was observed among all 4 light levels (data not shown). A further comparison of cultures grown at high (116 μmol photons m−2 s−1) and low (17 μmol photons m−2 s−1) light showed no significant difference in equivalent spherical diameter, and cellular carbon and nitrogen content and the C:N molar ratio (Table 1). In contrast, the Si:C (0.19 vs. 0.07) and Si:N (2.1 vs. 0.7) molar ratios were significantly higher for cells grown under low compared to high light due to the higher Si per cell and per surface area in the low light condition (p < 0.001, Student t-test).
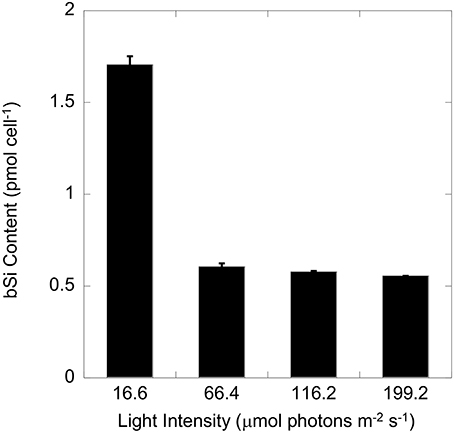
Figure 1. Cellular silica (bSi) of T. weissflogii growing exponentially under four different light intensities. Error bars show 1 SD (n = 3).

Table 1. Cell size and cellular element content of Thalassiosira weissflogii grown under low and high light.
In the two grazing experiments, the cellular silica content of T. weissflogii was significantly lower under high vs. low light conditions (Expt 1 = 291 vs. 760 fmol cell−1 and Expt 2 = 537 vs. 808 fmol cell−1, p < 0.001, Student t-test). In both grazing experiments, clearance rates and ingestion rates of P. crassirostris were significantly higher when copepods consumed low silica cells (Figure 2, p < 0.001, Student t-test). This indicates that copepods strongly preferred cells with low silica over high silica cells. The clearance rate in the first grazing experiment was higher than that in the second experiment, which could be due to the lower prey concentration in the first vs. second experiment (i.e., 1 copepod per 11,000 vs. 15,400 cells ml−1). In addition, in the second feeding experiment, fecal pellet production rate was significantly higher for copepods fed with highly silicified cells (0.46 ± 0.04 fecal pellets cop−1 h−1 compared to 0.28 ± 0.04 fecal pellets cop−1 h−1 for the low bSi diatom diet).
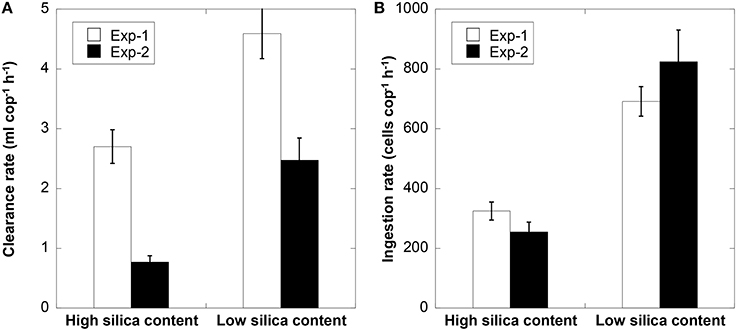
Figure 2. Clearance rate (A), and ingestion rate (B), of Parvocalanus crassirostris feeding on T. weissflogii cells with high and low silica content for two feeding experiments. Error bars are 1 SD (n = 3).
Copepod Growth, Egg Production, and Hatching Success under High and Low bSi Diatom Diets
Copepods fed with low bSi diatoms had a higher body weight than those fed with high bSi cells. The average wet weight of P. crassirostris increased from 7.2 to 10.8 μg ind−1 after 48 h when fed on low bSi cells, while those fed on high bSi diatoms remained nearly unchanged (7.4 μg ind−1). On day 3 of the incubation, the wet weight of copepods in both treatments decreased, but the difference between low and high bSi diets was still apparent (8.5 vs. 6.5 μg ind−1). Egg production rate and the percent hatching success were also significantly higher for copepods on the low bSi diatom diet compared to those fed high bSi cells (t-test, t = 9.956, df = 37, p < 0.001 for egg production rate; t = 5.383, df = 37, p < 0.001 for egg hatching success). Egg hatching data were arcsin-transformed for normality. The average egg production for 20 females of P. crassirostris fed with low bSi cells was 90 eggs female−1 d−1 (SD = 34), while 19 females of P. crassirostris on the high bSi diatom diet only produced an average of 11 eggs per day (SD = 8) (Figure 3). The percent egg hatching success for the low Si diatom diet was 82 ± 17% (1 SD), whereas the hatching success rate for those fed with high bSi diatoms was low (23 ± 36%) and highly variable with 12 out of 19 individuals yielded 0% hatching success. Therefore, highly silicified diatoms were detrimental to copepod growth, egg reproduction, and hatching success.
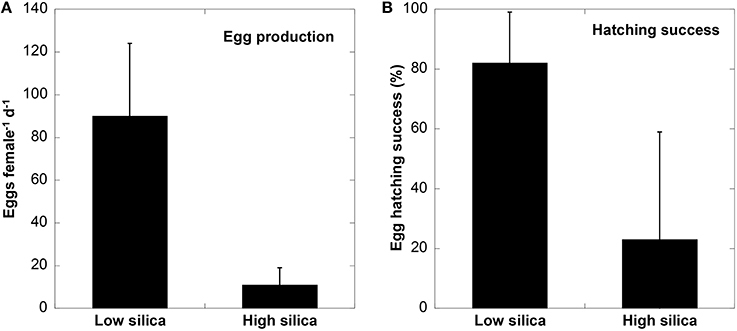
Figure 3. Egg production rate (A), and % hatching success (B), of P. crassirostris growing on low and high bSi diatom diets. Error bars indicate 1 SD and n = 19 for high Si and 20 for low Si treatments, respectively.
Discussion
Growth rate determined the bSi content of T. weissflogii, as the growth rate of T. weissflogii (0.32 d−1) grown at the lowest light level of 17 μmol m−2 s−1 was significantly lower than at higher light levels (0.81–0.98 d−1; data not shown). Using light, N, and P-limited continuous cultures of Thalassiosira pseudonana, Claquin et al. (2002) demonstrated that a decrease in growth rate resulted in a significant increase in the time spent in the period of cell wall synthesis and mitosis (G2+M), when Si uptake is usually high. Martin-Jézéquel et al. (2000) confirmed that both silicate availability and the prolongation of the cell cycle phase during which cell wall synthesis occurs, led to increased incorporation of silica into the cell wall. Claquin et al. (2002) also found that regardless of the type of limitation, biogenic silica (bSi) increased per cell and per cell surface area with decreasing growth rate, indicating that silicification was not coupled to the metabolism of N or P. Nevertheless, the cellular quota of C, N, and Si (both per cell and per surface area), as well as the C:N, Si:C, and Si:N ratios obtained in our experiments (Table 1) fell within the range reported for diatoms in general and for T. weissflogii specifically (Brzezinski, 1985; Strzepek and Price, 2000; Claquin et al., 2002).
Our results indicate that copepods strongly preferred diatoms with low silica content over high silica-containing cells. It is not clear what trigged the low ingestion rate on highly silicified cells, but the thicker frustule most probably reduced the digestibility of the cells (Raven and Waite, 2004). The significantly higher Si:C and Si:N ratios (i.e., the highest silica content for low light cells) may be one reason for the reduced feeding activity, however other chemical composition changes may also be associated with the growth on low light. It is well known that zooplankton feeding may also be reduced by the morphology or physical structure of their prey. Van Donk et al. (1997) reported that cells of the wall-deficient mutant of Chlamydomonas were more easily ingested and digested than normal cells, and cells with a thicker cell wall that were produced under nutrient limitation were not digested by Daphnia. We suspect that a copepod needs a longer time to digest and evacuate the highly silicified diatoms through its gut which would lead to a reduction in the ingestion rate. Bienfang (1980) found that fecal pellets from copepods feeding on diatoms were similar in volume but denser and subsequently having a higher sinking rate than fecal pellets from copepods feeding on flagellates. Therefore, the silica frustule in the fecal pellet enhances carbon export.
The fecal pellet production rate was significantly higher for copepods that were fed highly silicified cells. Low ingestion and high fecal production would result in malnutrition, which would lead to slow growth, low egg production and hatching success and ultimately a decline in a copepod population. Therefore, it is reasonable to predict that copepods during the early stage of a diatom bloom (i.e., with relatively low silicification due to sufficient silicic acid and high growth rate) will be generally healthier than copepods feeding during the declining phase of the bloom (i.e., high silicification due to the exhaustion of SiO4 and slow growth), or those feeding in iron-limited waters as iron limitation also increases Si uptake relative to N and thus a higher cellular Si:N ratio (Takeda, 1998; Marchetti and Harrison, 2007). This may partially explain why mesozooplankton abundance is often low in waters with a large diatom bloom (e.g., Liu and Dagg, 2003).
Several studies have reported grazing selectivity against diatoms (Kleppel et al., 1991; Strom and Welschmeyer, 1991; Liu et al., 2005, 2010). It has been proposed that some diatoms are of inferior nutritional quality because they lack some essential lipids (Kleppel, 1993; Jónasdóttir and Kiørboe, 1996), while others have claimed the nutritional superiority of diatoms (Brown et al., 1997; Chen et al., 2012). Some studies have suggested detrimental effects of diatoms on copepod reproduction, probably due to toxic aldehydes (e.g., Miralto et al., 1999), but other studies have shown no negative effect of a diatom-dominated diet (e.g., Irigoien et al., 2002). However, no study has directly tested the effect of the level of diatom silicification on copepods. In a mesocosm study, Van Nieuwerburgh et al. (2004) reported that a heavily silicified diatom bloom hampered copepod growth. They further suggested that copepod populations can be strongly regulated by ambient Si:N ratios since high Si:N ratios led to larger diatom species and heavier silicification, providing diatoms with the possibility to escape from grazing, leading to the accumulation of algal biomass without a transfer to higher trophic levels. Our study is the first controlled laboratory study to demonstrate that diatoms with different cellular silica content can influence copepod clearance and ingestion rates since lightly silicified cells were preferred over heavily silicified cells. Furthermore, diatoms with a thick frustule may have a negative impact on copepod growth, egg production and hatching rates as observed in this study (Figure 3).
Observational and modeling studies usually relate high export of carbon with diatom blooms (Honjo and Manganini, 1993; Allen et al., 2005). By studying the physiology and reproductive biology of copepods growing on a diet of diatoms with different silica content, we are able to better understand the copepod population dynamics during the spring diatom bloom and post-bloom environments. Based on both the low ingestion rate and compromised growth and reproduction of copepods grown with highly silicified diatoms as their sole food source, it can be predicted that a rapid population growth of copepods may not occur as the diatom bloom progresses and consumption of the bloom by copepods may be limited. Therefore, the growth environment of diatoms may determine the fate of the bloom and the scale and proportion of the carbon export through the sinking of ungrazed dead diatom cells and aggregates compared to faster the transport of silica to depth via zooplankton fecal pellets.
Author Contributions
HL designed the experiments; HL, MC, and FZ carried out the experiments; HL, ZF wrote the manuscript; HL, PH revised and improved the manuscript; HL provided funding support.
Conflict of Interest Statement
The authors declare that the research was conducted in the absence of any commercial or financial relationships that could be construed as a potential conflict of interest.
Acknowledgments
Financial support for this study was from the University Grants Council of Hong Kong AoE project (AoE/P-04/04), the Research Grant Council of Hong Kong (661809, 661610, and 661911) and the TUYF Charitable Trust (TUYF10SC08). Additional support from Natural Science Foundation of China (41330961) is also acknowledged.
References
Allen, J. T., Brown, L., Sanders, R., Moore, C. M., Mustard, A., Fielding, S., et al. (2005). Diatom carbon export enhanced by silicate upwelling in the northeast Atlantic. Nature 437, 728–732. doi: 10.1038/nature03948
Armbrust, E. V. (2009). The life of diatoms in the world's oceans. Nature 459, 185–192. doi: 10.1038/nature08057
Benitez-Nelson, C. R., Bidigare, R. R., Dickey, T. D., Landry, M. R., Leonard, C. L., Brown, S. L., et al. (2007). Mesoscale eddies drive increased silica export in the subtropical Pacific Ocean. Science 316, 1017–1021. doi: 10.1126/science.1136221
Benitez-Nelson, C., and McGillicuddy, D. J. (2008). Mesoscale physical–biological–biogeochemical linkages in the open ocean: an introduction to the results of the E-Flux and EDDIES programs. Deep Sea Res. II 55, 1133–1138. doi: 10.1016/j.dsr2.2008.03.001
Bienfang, P. K. (1980). Herbivore diet affects fecal pellet sinking. Can. J. Fish. Aquat. Sci. 37, 1352–1357. doi: 10.1139/f80-173
Boyd, P. W., Jickells, T., Law, C. S., Blain, S., Boyle, E. A., Buesseler, K. O., et al. (2007). Mesoscale iron enrichment experiments 1993-2005: synthesis and future directions. Science 315, 612–617. doi: 10.1126/science.1131669
Brown, M. R., Jeffrey, S. W., Volkman, J. K., and Dunstan, G. A. (1997). Nutritional properties of microalgae for mariculture. Aquaculture 151, 315–331. doi: 10.1016/S0044-8486(96)01501-3
Brown, S. L., Landry, M. R., and Selph, K. E. (2008). Diatoms in the desert. Deep-Sea Res. II 55, 1321–1333. doi: 10.1016/j.dsr2.2008.02.012
Brzezinski, M. A. (1985). The Si:C:N ratio of marine diatoms: interspecific variability and the effect of some environmental variables. J. Phycol. 21, 347–357. doi: 10.1111/j.0022-3646.1985.00347.x
Brzezinski, M. A., Olson, R. J., and Chisholm, S. W. (1990). Silicon availability and cell-cycle progression in marine diatoms. Mar. Ecol. Prog. Ser. 67, 83–96. doi: 10.3354/meps067083
Chen, M., Liu, H., and Chen, B. (2012). Effects of dietary essential fatty acids on reproduction rates of a subtropical calanoid copepod, Acartia erythraea. Mar. Ecol. Prog. Ser. 455, 95–110. doi: 10.3354/meps09685
Claquin, P., Martin-Jézéquel, V., Kromkamp, J. C., Veldhuis, M. J. W., and Kraay, G. W. (2002). Uncoupling of silicon compared with carbon and nitrogen metabolisms and the role of the cell cycle in continuous cultures to Thalassiosira pseudonana (Bacillariophyceae) under light, nitrogen, and phosphorus control. J. Phycol. 38, 922–930. doi: 10.1046/j.1529-8817.2002.t01-1-01220.x
Conley, D. J., Kilham, S. S., and Theriot, E. (1989). Differences in silica content between marine and freshwater diatoms. Limnol. Oceanogr. 34, 205–213. doi: 10.4319/lo.1989.34.1.0205
D'Alelio, D., d'Alicalia, M. R., Dubroca, L., Sarno, D., Zingone, A., and Montresor, M. (2010). The time for sex: a biennial life cycle in a marine planktonic diatom. Limnol. Oceanogr. 55, 106–114. doi: 10.4319/lo.2010.55.1.0106
Ducklow, H. W., and Harris, R. P. (1993). Introduction to the JGOFS North Atlantic Bloom Experiment. Deep-Sea Res. II 40, 1–8. doi: 10.1016/0967-0645(93)90003-6
Durbin, E. G. (1977). Studies on the autecology of the marine diatom Thalassiosira nordenskioeldii. 2. The influence of cell size on growth rate, and carbon, nitrogen, chlorophyll a and silica content. J. Phycol. 13, 150–155.
Field, C. B., Behrenfeld, M. J., Randerson, J. T., and Falkowski, P. (1998). Primary production of the biosphere: integrating terrestrial and oceanic components. Science 281, 237–240. doi: 10.1126/science.281.5374.237
Furnas, M. (1978). Influence of temperature and cell size on the division rate and chemical content of the diatom Chaetoceros vurvisetum Cleve. J. Exp. Mar. Biol. Ecol. 34, 97–109.
Grasshoff, K., Kremling, K., and Ehrhardt, M. (1999). Methods of Seawater Analysis, 3rd Edn. Weinheim; Berlin: Viley-VCH.
Harrison, P. J., Conway, H. L., Holmes, R. W., and Davis, C. O. (1977). Marine diatoms in chemostats under silicate or ammonium limitation. 3. Cellular chemical composition and morphology of Chaetoceros debilis, Skeletonema costatum, and Thalassiosira gravida. Mar. Biol. 43, 19–31.
Honjo, S., and Manganini, S. J. (1993). Annual biogenic particle fluxes to the interior of the North Atlantic Ocean studied at 348 N 218 W and 488 N 218 W. Deep Sea Res. II 40, 587–607.
Irigoien, X., Harris, R. P., Verheye, H. M., Joly, P., Runge, J., Starr, M. et al. (2002). Copepod hatching success in marine ecosystems with high diatom concentrations. Nature 419, 387–389. doi: 10.1038/nature01055
Jónasdóttir, S. H., and Kiørboe, T. (1996). Copepod recruitment and food composition: do diatoms affect hatching success? Mar. Biol. 125, 743–750.
Kleppel, G. S. (1993). On the diets of calanoid copepods. Mar. Ecol. Prog. Ser. 99, 183–195. doi: 10.3354/meps099183
Kleppel, G. S., Holliday, D. V., and Pieper, R. E. (1991). Trophic interactions between copepods and microplankton: a question about the role of diatoms. Limnol. Oceanogr. 36, 172–178. doi: 10.4319/lo.1991.36.1.0172
Kooistra, W. H. C. F., Gersonde, R., Medlin, L. K., and Mann, D. G. (2007). “The origin and evolution of the diatoms: their adaptation to a planktonic existence,” in Evolution of Primary Producers in the Sea, eds P. G. Falkowski and A. H. Knoll (London, UK: Elsevier Academic Press), 207–249.
Liu, H., Chen, M., Suzuki, K., Wong, C. K., and Chen, B. (2010). Mesozooplankton selective feeding in subtropical coastal waters revealed by HPLC pigment analysis. Mar. Ecol. Prog. Ser. 407, 111–123. doi: 10.3354/meps08550
Liu, H., and Dagg, M. J. (2003). Interactions between nutrients, phytoplankton growth, and micro- and mesozooplankton grazing in the plume of the Mississippi River. Mar. Ecol. Prog. Ser. 258, 31–42. doi: 10.3354/meps258031
Liu, H., Dagg, M. J., Wu, C.-J., and Chiang, K.-P. (2005). Mesozooplankton consumption of microplankton in the Mississippi River plume, with special emphasis on planktonic ciliates. Mar. Ecol. Prog. Ser. 286, 133–144. doi: 10.3354/meps286133
Marchetti, A., and Harrison, P. J. (2007). Coupled change in the cell morphology and the elemental composition (C, N, Si) composition of the pennate diatom Pseudo-nitzschia due to iron deficiency. Limnol. Oceanogr. 52, 2220–22284. doi: 10.4319/lo.2007.52.5.2270
Martin-Jézéquel, V., Hildebrand, M., and Brzezinski, M. A. (2000). Silicon metabolism in diatoms: implications for growth. J. Phycol. 36, 821–840. doi: 10.1046/j.1529-8817.2000.00019.x
Miralto, A., Barone, G., Romano, G., Poulet, S. A., Ianora, A., Russo, G. L., et al. (1999). The insidious effect of diatoms on copepod reproduction. Nature 402, 173–176. doi: 10.1038/46023
Nelson, D. M., Treguer, P., Brzezinski, M. A., Leynaert, A., and Queguiner, B. (1995). Production and dissolution of biogenic silica in the ocean: revised global estimates, comparison with regional data and relationship to biogenic sedimentation. Glob. Biogeochem. Cycles 9, 359–372. doi: 10.1029/95gb01070
Paasche, E. (1973). The influence of cell size on growth rate, silica content, and some other properties of four marine diatom species. Norw. J. Bot. 20, 197–204.
Paasche, E. (1980). Silicon content of five marine plankton diatom species measured with a rapid filter method. Limnol. Oceanogr. 25, 474–480.
Parsons, T. R., Stephens, K., and Strickland, J. D. (1961). On the chemical composition of eleven species of marine phytoplankters. J. Fish. Res. Bd. Can. 18, 1001–1016. doi: 10.1139/f61-063
Pondaven, P., Gallinari, M., Chollet, S., Bucciarelli, E., Sarthou, G., Schultes, S., et al. (2007). Grazing induced changes in cell wall silicification in a marine diatom. Protist 158, 21–28. doi: 10.1016/j.protis.2006.09.002
Raven, J. A., and Waite, A. M. (2004). The evolution of silicification in diatoms: inescapable sinking and sinking as escape? New Phytol. 162, 45–61. doi: 10.1111/j.1469-8137.2004.01022.x
Rii, Y. M., Brown, S. L., Nencioli, F., Kuwahara, V. S., Dickey, T. D., Karl, D. M., et al. (2008). The transient oasis: nutrient-phytoplankton dynamics and particle export in Hawaiian lee cyclones, Deep Sea Res. II 55, 1275–1290. doi: 10.1016/j.dsr2.2008.01.013
Saito, H., and Tsuda, A. (2003). Influence of light intensity on diatom physiology and nutrient dynamics in the Oyashio region. Prog. Oceanogr. 57, 251–263. doi: 10.1016/s0079-6611(03)00100-9
Smetacek, V. (1999). Diatoms and the ocean carbon cycle. Protist 150, 25–32. doi: 10.1016/S1434-4610(99)70006-4
Strom, S. L., and Welschmeyer, N. A. (1991). Pigment-specific rates of phytoplankton growth and microzooplankton grazing in the open subarctic Pacific Ocean. Limnol. Oceanogr. 36, 50–63. doi: 10.4319/lo.1991.36.1.0050
Strzepek, R. F., and Price, N. M. (2000). Influence of irradiance and temperature on the iron content of the marine diatom Thalassiosira weissflogii (Bacillariophyceae). Mar. Ecol. Prog. Ser. 206, 107–117. doi: 10.3354/meps206107
Takeda, S. (1998). Influence of iron availability on nutrient ratios of diatoms in oceanic waters. Nature 393, 774–777. doi: 10.1038/31674
Taylor, N. J. (1985). Silica incorporation in the diatom Coscinodiscus granii as affected by light intensity. Br. Phycol. J. 20, 365–374.
Van Donk, E., Lürling, M., Hessen, D. O., and Lokhorst, G. M. (1997). Altered cell wall morphology in nutrient-deficient phytoplankton and its impact on grazers. Limnol. Oceanogr. 42, 357–364. doi: 10.4319/lo.1997.42.2.0357
Van Nieuwerburgh, L., Wänstrand, I., and Snoeijs, P. (2004). Growth and C:N:P ratios in copepods grazing on N- or Si-limited phytoplankton blooms. Hydrobiologia 514, 57–72. doi: 10.1023/B:hydr.0000018206.02271.2b
Keywords: diatom silica content, copepod feeding, copepod egg production, hatching success, feeding selectivity
Citation: Liu H, Chen M, Zhu F and Harrison PJ (2016) Effect of Diatom Silica Content on Copepod Grazing, Growth and Reproduction. Front. Mar. Sci. 3:89. doi: 10.3389/fmars.2016.00089
Received: 21 February 2016; Accepted: 20 May 2016;
Published: 02 June 2016.
Edited by:
Maeve Carroll Lohan, University of Southampton, UKReviewed by:
Alex James Poulton, National Oceanography Centre, UKEdward Buskey, University of Texas at Austin, USA
Copyright © 2016 Liu, Chen, Zhu and Harrison. This is an open-access article distributed under the terms of the Creative Commons Attribution License (CC BY). The use, distribution or reproduction in other forums is permitted, provided the original author(s) or licensor are credited and that the original publication in this journal is cited, in accordance with accepted academic practice. No use, distribution or reproduction is permitted which does not comply with these terms.
*Correspondence: Hongbin Liu, liuhb@ust.hk