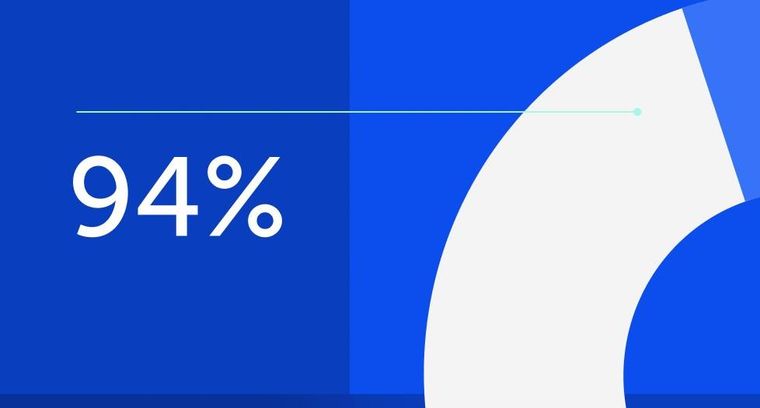
94% of researchers rate our articles as excellent or good
Learn more about the work of our research integrity team to safeguard the quality of each article we publish.
Find out more
MINI REVIEW article
Front. Mar. Sci., 19 September 2014
Sec. Marine Pollution
Volume 1 - 2014 | https://doi.org/10.3389/fmars.2014.00039
Organotin compounds (OTs) have been used as biocides in antifouling paints and agriculture. The IMO introduced a global ban on the use of OTs in antifouling systems in 2001 due to their high toxicity. However, OTs have still been detected in the environment and pose a threat to the ecosystem. Several research groups have summarized the analytical methods, environmental fate, biochemistry, reproductive toxicity and mechanisms of actions of OTs. Here, we reviewed the developmental toxicity of OTs in various organisms such as sea urchin, ascidian, mussel, and fish. The differences in sensitivity to OT exposure exist not only in different species but also at different stages in the same species. Though some hypotheses have been proposed to explain the developmental toxicity of OTs, the solid evidences are greatly in need.
Organotin compounds (OTs) have been widely used as biocides in antifouling paints and agriculture since the 1960s. Tributyltin (TBT) and triphenyltin (TPT) are most important OTs. TBT is considered the most toxic man-made and deliberately substances introduced into the environment. It can cause the imposex in gastropods—a condition in which male sex organs develop in females (Gibbs et al., 1987; Shi et al., 2005). Therefore, the International Maritime Organization (IMO) calls for a global treaty that bans the application of OTs as biocides (IMO, 2001). The unique story of TBT and imposex not only is one of the best examples of endocrine disruption in wildlife but also show us a typical story of the integration between the fields of scientific research and regulation (Sousa et al., 2013).
Despite this success, the story of OTs is far from its end. OTs are still widely used rather than in antifouling paints. OTs have been still detected in various environments in recent years (Yi et al., 2012; Ho and Leung, 2014). Thus, OTs inputs will continue, and the hazard of OTs will still exist. In recent years, several research groups have reviewed the analytical methods (Dubalska et al., 2013), environmental fate (Dubalska et al., 2013; Graceli et al., 2013; Sousa et al., 2013), reproductive toxicity (Graceli et al., 2013), and the mechanisms of actions of OTs (Pagliarani et al., 2013). The early developmental stages of organisms are highly sensitive to chemical exposure. Therefore, we reviewed the current study of developmental toxicity of OTs in the present paper.
Many studies have shown that OTs have high toxicity to embryos of various organisms at environmentally relevant concentrations (Table 1).
TBT significantly reduces the growth of sea urchin Paracentrotus lividus from post-fertilization to the pluteus stage at 0.01 μg/L (Marin et al., 2000). The length of the P. lividus pluteus somatic rods is significantly reduced by 1.5 μg/L TPT at 48 h post-fertilization. Progressive increases in skeletal anomalies are also detected. Embryos never reach the pluteus stage at 5 μg/L, and the development is blocked at the gastrula stage at 10 μg/L (Moschino and Marin, 2002).
TBT blocks Styela plicat embryo development to the larval stage from 1 μM. Exposure to 10 μM TPT soon after fertilization hinders embryonic cleavage and at the two-cell to four-cell stage blocks further development (Cima et al., 1996). Incubation of Ciona intestinalis neurula larvae in 0.1–10 μM TBT solutions for 1–2 h provokes serious anomalies thus causes an irreversible block of embryonic cleavage (Dolcemascolo et al., 2005).
TBT increases the mortality of Crassostrea gigas embryos at 0.36 μg/L at 24 h (Tsunemasa et al., 2011), and induces malformations of Mytilus galloprovincialis embryos at 0.161 μg/L (Beiras and Bellas, 2008). TBT also induces cytogenetic damage (sister chromatid exchanges and chromosomal aberrations) in Mytilus edulis embryo (Jha et al., 2000).
TPT increases the mortality of European minnows Phoxinus phoxinus embryos at 3.9 μg/L and causes bent tails, opaque eyes and impaired swimming behavior in P. phoxinus larvae after 3 days exposure (Fent and Meier, 1994). TPT also induced skeletal and ocular deformations in other fishes at environmentally relevant concentration (Strmac and Braunbeck, 1999; Hu et al., 2009). TBT reduces the hatchability and causes dorsal curvature, severely twisted tails and pericardial edema in Sebastiscus marmoratus embryo at no less than 10 ngSn/L levels (Zhang et al., 2011).
TBT and TPT induce multiple malformations in Xenopus tropicalis embryos at environmentally relevantly concentrations (Guo et al., 2010; Yu et al., 2011). The dominant phenotypes of malformation include abnormal eyes, enlarged proctodaeum, and narrow fins (Yu et al., 2011). TPT also significantly affects survival, growth, and days to metamorphosis in Lithobates sylvaticus larvae at 0.1 μg/L (Higley et al., 2013).
DBTCl (dibutyltin chloride) leads to a significantly decrease of survival rate of fetuses at terminal cesarean sectioning in cynomolgus monkeys after 2.5 mg/kg exposure. TPT induces postimplantation embryonic loss in pregnant rats, but no results indicate teratogenic responses. The study of mammals may suggest that OTs possesses no teratogenic effect in mammals (Ema et al., 2007).
Toxicity of xenobiotics to embryos is dependent not only on type, level, and duration of exposure but also on developmental stages of exposure. In sea urchin, organotins strongly affect all stages (soon after fertilization, two to four cells, gastrula, and neurula) of Styela plicata embryo, but the most sensitive and critical stage is gastrula (Cima et al., 1996). Same sensitive stage has been reported in the Ciona intestinalis embryo (Vittoriaa et al., 2009). Gastrula is also the most sensitive stage when Lytechinus variegatus embryos is treated with TBT (Perina et al., 2011). The most susceptible developmental stage to trimethyltin (TMT) exposure is between 48 and 72 hpf in zebrafish embryo (Chen et al., 2012). The most sensitive stage is stage39/40 to stage41, followed by stage41 to stage43 in amphibian (Xenopus tropicalis) embryos after exposure to TPT (Yuan et al., 2011).
Several mechanisms of actions of OTs have been reported both in vitro and in vivo studies (Grün and Blumberg, 2006). However, the mechanism of OTs in the developmental stages is still not clear.
TBT and TPT have been proved to induce imposex in snails by binding to retinoid X receptor (RXR), as the natural ligand of RXR, 9-cis retinoic acid (9c-RA) acts (Figures 1A–C) (Nishikawa et al., 2004; Nakanishi, 2008). Many studies have suggested that TBT or TPT disturb fish and frog embryos development through RXR (Yu et al., 2011; Zhang et al., 2011; Higley et al., 2013). Since the natural ligand of RXR (i.e., 9c-RA) is a well-known teratogen by disrupting retinoic acid (RA) signal in vertebrate embryos (Minucci et al., 1996), it is reasonable to deduce that OTs induce malformations through RA signaling pathway (Hu et al., 2009). However, TPT and 9c-RA induce totally different phenotypes of malformations and have different sensitive exposure windows in frog embryos (Figures 1D–F) (Yu et al., 2011; Yuan et al., 2011). These findings suggest that RA signal is not involved in OTs-induced teratogenicity.
Figure 1. Comparison of toxic effects of triphenyltin (TPT) and 9-cis retinoic acid (9c-RA) on snail (Thais clavigera) (A–C) and frog (Xenopus tropicalis) (D–F). Photographs of (A–C) were cited from Nishikawa et al. (2004) with permission. cg, capsule gland; ov, ovary; p, penis; vd, vas deferens; e, eye; f, fin; bt, bent tail; te, turbid lens of eyes; nf, narrow fin; pe, protruding eye.
In zebrafish (Danio rerio) embryo, 5 μM TMT exposure significantly promots apoptosis in the tail, this enhanced apoptosis in the tail may partly explain the attenuated tail touch response in the TMT treated embryo (Chen et al., 2011). Similar localized apoptosis in the tail is caused by TBT in Sebastiscus marmoratus embryo, which may be the reason of TBT induced twisted tails abnormality (Zhang et al., 2011). TMT actives the caspase 8/caspase 3 pathway for nuclear translocation of DNases in the primary cultured cortical neurons from embryonic mice, suggesting that TMT neurotoxicity is initially caused by activating this apoptosis pathway (Kuramoto et al., 2011).
Calcium ion is a key signaling molecule in many developmental processes and plays a critical role in chemical-induced toxic cell killing and apoptosis. In the eggs of the sea urchin Paracentrotus lividus, TBT inhibits intracellular sequestration of Ca2+ into the reticular compartment at low concentrations and inhibits the ion flow during skeletal deposition (Moschino and Marin, 2002).
TBT exposure results in a significant decrease of Na+/K+-ATPase activity in S. marmoratus brains and induces changes in the total pattern of phosphotyrosine and in the phosphorylation levels of ERK 1/2 in Ciona intestinali embryos (Zhang et al., 2008; Damiani et al., 2009). Another subfamily of MAPKs, c-Jun N-terminal kinase (JNK), is reported to be involved in part of the neuronal degeneration induced by TMT in cortical neurons of mice (Shuto et al., 2009).
A lot of researchers have suggested that OTs show a high toxicity to many species, especially at their early life stages. The differences in sensitivity to OT exposure exist not only in different species but also at different stages in the same species. Though some hypotheses have been proposed to explain the developmental toxicity of OTs, the solid evidences are greatly in need. Further studies may help to fill gaps and to improve knowledge of developmental toxicity of OTs.
The Associate Editor, Dr. Wan-Xi Yang, declares that despite being affiliated to the same institution as authors Zhenhuang Chen and Xiao Huang, as well as Reviewer Dan-dan Ma, the review process was handled objectively and no conflict of interest exists. The authors declare that the research was conducted in the absence of any commercial or financial relationships that could be construed as a potential conflict of interest.
This work was supported by grants from the Natural Science Foundation of China No.31271560 (attributed to Xiao Huang) and No.21277049 (attributed to Huahong Shi).
Beiras, R., and Bellas, J. (2008). Inhibition of embryo development of the Mytilus galloprovincialis marine mussel by organic pollutants; assessment of risk for its extensive culture in the Galician Rias. Aquaculture 277, 208–212. doi: 10.1016/j.aquaculture.2008.03.002
Chen, J. F., Huang, C. J., Truong, L., Du, J. L., Tilton, S. C., Waters, K. M., et al. (2012). Early life stage trimethyltin exposure induces ADP-ribosylation factor expression and perturbs the vascular system in zebrafish. Toxicology 302, 129–139. doi: 10.1016/j.tox.2012.09.004
Chen, J. F., Huang, C. J., Zheng, L. D., Simonich, M., Bai, C. L., Tanguay, R., et al. (2011). Trimethyltin chloride (TMT) neurobehavioral toxicity in embryonic zebrafish. Neurotoxicol. Teratol. 33, 721–726. doi: 10.1016/j.ntt.2011.09.003
Cima, F., Ballarin, L., Bressa, G., Martinucci, G., and Burighel, P. (1996). Toxicity of organotin compounds on embryos of a marine invertebrate (Styela plicata; tunicata). Ecotoxicol. Environ. Saf. 35, 174–182. doi: 10.1006/eesa.1996.0097
Damiani, F., Gianguzza, M., and Dolcemascolo, G. (2009). Effects of tributyltin chloride in ascidian embryos: modulation of kinase-mediated signalling pathways. Invert. Surviv. J. 6, S87–S94.
Dimitriou, P., Castritsi-Catharios, J., and Miliou, H. (2003). Acute toxicity effects of tributyltin chloride and triphenyltin chloride on gilthead seabream, Sparus aurata L., embryos. Ecotoxicolo. Environ. Saf. 54, 30–35. doi: 10.1016/S0147-6513(02)00008-8
Dolcemascolo, G., Gianguzza, P., Pellerito, C., and Gianguzza, M. (2005). Effects of tri-n-butyltin (IV) chloride on neurulation of Ciona intestinalis (Tunicata, Ascidiacea): an ultrastructural study. Appl. Organometal. Chem. 19, 11–22. doi: 10.1002/aoc.764
Dubalska, K., Rutkowska, M., Bajger-Nowak, G., Konieczkaa, P., and Namieśnika, J. (2013). Organotin compounds: environmental fate and analytics. Crit. Rev. Anal. Chem. 43, 35–54. doi: 10.1080/10408347.2012.743846
Ema, M., Fukunishi, K., Matsumoto, M., Hirose, A., Kamata, E., and Ihara, T. (2007). Developmental toxicity of dibutyltin dichloride in cynomolgus monkeys. Reprod. Toxicol. 32, 150–157. doi: 10.1016/j.reprotox.2006.09.003
Fent, K., and Meier, W. (1994). Effects of triphenyltin on fish early life stages. Arch. Environ. Contam. Toxicol. 27, 224–231. doi: 10.1007/BF00214266
Gibbs, P. E., Bryan, G. W., Pascoe, P. L., and Burt, G. R. (1987). The use of the dog- whelk, Nucella lapillus, as an indicator of tributyltin (TBT) contamination. J. Mar. Biol. Assoc. 67, 507–523. doi: 10.1017/S0025315400027260
Graceli, J. B., Sena, G. C., and Iguatemy Lopes, P. F. (2013). Organotins: a review of their reproductive toxicity, biochemistry, and environmental fate. Reprod. Toxicol. 36, 40–52. doi: 10.1016/j.reprotox.2012.11.008
Grün, F., and Blumberg, B. (2006). Environmental obesogens: organotins and endocrine disruption via nuclear receptor signaling. Endocrinology 147, S50–S55. doi: 10.1210/en.2005-1129
Guo, S. Z., Qian, L. J., Shi, H. H., Barry, T., Cao, Q. Z., and Liu, J. Q. (2010). Effects of tributyltin (TBT) on Xenopus tropicalis embryos at environmentally relevant concentrations. Chemosphere 79, 529–533. doi: 10.1016/j.chemosphere.2010.02.021
Higley, E., Tompsett, A. R., Giesy, J. P., Hecker, M., and Wiseman, S. (2013). Effects of triphenyltin on growth and development of the wood frog (Lithobates sylvaticus). Aquat. Toxicol. 144–145, 155–161. doi: 10.1016/j.aquatox.2013.09.029
Ho, K. K. Y., and Leung, K. M. Y. (2014). Organotin contamination in seafood and its implication for human health risk in Hong Kong. Mar. Pollut. Bull. 85, 634–640. doi: 10.1016/j.marpolbul.2013.12.039
Hu, J. Y., Zhang, Z. B., Wei, Q. W., Zhen, H. J., Zhao, Y. B., Peng, H., et al. (2009). Malformations of the endangered Chinese sturgeon, Acipenser sinensis, and its causal agent. Proc. Natl. Acad. Sci. U.S.A. 106, 9339–9344. doi: 10.1073/pnas.0809434106
International Marine Organisation (IMO). (2001). International convention on the control of harmful anti-fouling systems on ships. Available online at: http://www.imo.org/About/Conventions/ListOfConventions/Pages/International-Convention-on-the-Control-of-Harmful-Anti-fouling-Systems-on-Ships-(AFS).aspx (Accessed 2014).
Jha, A. N., Hagger, J. A., and Hill, S. J. (2000). Tributyltin induces cytogenetic damage in the early life stages of the marine mussel, Mytilus edulis. Environ. Mol. Mutagen. 35, 343–350. doi: 10.1002/1098-2280(2000)35:4<343::AID-EM9>3.0.CO;2-5
Kuramoto, N., Seko, K., Sugiyama, C., Shuto, M., and Ogita, K. (2011). Trimethyltin initially activates the caspase 8/caspase 3 pathway for damaging the primary cultured cortical neurons derived from embryonic mice. J. Neurosci. Res. 89, 552–561. doi: 10.1002/jnr.22588
Marin, M. G., Moschino, V., Cima, F., and Celli, C. (2000). Embryotoxicity of butyltin compounds to the sea urchin Paracentrotus lividus. Mar. Environ. Res. 50, 231–235. doi: 10.1016/S0141-1136(00)00072-6
Minucci, S., Saint-Jeannet, J. P., Toyama, R., Scita, G., DeLuc, L. M., Tiara, M., et al. (1996). Retinoid X receptor-selective ligands produce malformations in Xenopus embryos. Proc. Natl. Acad. Sci. U.S.A. 93, 1803–1807. doi: 10.1073/pnas.93.5.1803
Moschino, V., and Marin, M. G. (2002). Spermiotoxicity and embryotoxicity of triphenyltin in the sea urchin Paracentrotus lividus Lmk. Appl. Organometal. Chem. 16, 175–181. doi: 10.1002/aoc.285
Nakanishi, T. (2008). Endocrine disruption induced by organotin compounds; organotins function as a powerful agonist for nuclear receptors rather than an aromatase inhibitor. J. Toxicol. Sci. 33, 269–276. doi: 10.2131/jts.33.269
Nishikawa, J., Mamiya, S., and Kanayama, T. (2004). Involvement of the retinoid X receptor in the development of imposex caused by organotins in gastropods. Environ. Sci. Technol. 38, 6271–6276. doi: 10.1021/es049593u
Pagliarani, A., Nesci, S., and Ventrella, V. (2013). Toxicity of organotin compounds: shared and unshared biochemical targets and mechanisms in animal cells. Toxicol. In. Vitro 27, 978–990. doi: 10.1016/j.tiv.2012.12.002
Perina, F. C., Abessa, D. M., Pinho, G. L., and Fillmann, G. (2011). Comparative toxicity of antifouling compounds on the development of sea urchin. Ecotoxicology 20, 1870–1880. doi: 10.1007/s10646-011-0725-y
Shi, H. H., Huang, C. J., Zhu, S. X., Yu, X. J., and Xie, W. Y. (2005). Generalized system of imposex and reproductive failure in female gastropods of coastal waters of mainland China. Mar. Ecol. Prog. Ser. 304, 179–189. doi: 10.3354/meps304179
Shuto, M., Seko, K., Kuramoto, N., Sugiyama, C., Kawada, K., Yoneyama, M., et al. (2009). Activation of c-Jun N-terminal kinase cascades is involved in part of the neuronal degeneration induced by trimethyltin in cortical neurons of mice. J. Pharmacol. Sci. 109, 60–70. doi: 10.1254/jphs.08211FP
Sousa, A. C. A., Pastorinho, M. R., and Takahashi, S. (2013). History on organotin compounds, from snails to humans. Environ. Chem. Lett. 12, 117–137. doi: 10.1007/s10311-013-0449-8
Strmac, M., and Braunbeck, T. (1999). Effects of triphenyltin acetate on survival, hatching success, and liver ultrastructure of early life stages of zebrafish (Danio rerio). Ecotoxicol. Environ. Saf. 44, 25–39. doi: 10.1006/eesa.1999.1781
Tsunemasa, N., Tsuboi, A., and Okamura, H. (2011). Effects of organotin alternative antifoulants on oyster. Arch. Environ. Contam. Toxicol. 61, 128–134. doi: 10.1007/s00244-010-9598-y
Vittoriaa, C. M., Mansuetoa, V., Puccia, E., Corsi, I., Bonacci, S., Focardi, S., et al. (2009). A biochemical study of the effects of tributyltin on unfertilized eggs, embryos and larvae of the sea squirt Ciona intestinalis. Caryologia 62, 309–315. doi: 10.1080/00087114.2004.10589696
Yi, A. X., Leung, K. M., Lam, M. H., Lee, M. S., and Giesy, J. P. (2012). Review of measured concentrations of triphenyltin compounds in marine ecosystems and meta-analys is of their risks to humans and the environment. Chemosphere 89, 1015–1025. doi: 10.1016/j.chemosphere.2012.05.080
Yu, L., Zhang, X. L., Yuan, J., Cao, Q. Z., Liu, J. Q., Zhu, P., et al. (2011). Teratogenic effects of triphenyltin on embryos of amphibian (Xenopus tropicalis): a phenotypic comparison with the retinoid X and retinoic acid receptor ligands. J. Hazad. Mater. 192, 1860–1888. doi: 10.1016/j.jhazmat.2011.07.027
Yuan, J., Zhang, X. L., Yu, L., Sun, Z., Zhu, P., Wang, X., et al. (2011). Stage-specific malformations and phenotypic changes induced in embryos of amphibian (Xenopus tropicalis) by triphenyltin. Ecotoxicol. Environ. Saf. 74, 1960–1966. doi: 10.1016/j.ecoenv.2011.07.020
Zhang, J. L., Zuo, Z. H., Wang, Y. Q., Yu, A., Chen, Y. X., and Wang, C. G. (2011). Tributyltin chloride results in dorsal curvature in embryo development of Sebastiscus marmoratus via apoptosis pathway. Chemosphere 82, 437–442. doi: 10.1016/j.chemosphere.2010.09.057
Keywords: organotin, developmental toxicity, stage sensitivity, embryo, RXR
Citation: Wu L, Chen Z, Zhu J, Hu L, Huang X and Shi H (2014) Developmental toxicity of organotin compounds in animals. Front. Mar. Sci. 1:39. doi: 10.3389/fmars.2014.00039
Received: 21 July 2014; Paper pending published: 16 August 2014;
Accepted: 26 August 2014; Published online: 19 September 2014.
Edited by:
Wan-Xi Yang, Zhejiang University, ChinaReviewed by:
Jongseong Ryu, Anyang University, South KoreaCopyright © 2014 Wu, Chen, Zhu, Hu, Huang and Shi. This is an open-access article distributed under the terms of the Creative Commons Attribution License (CC BY). The use, distribution or reproduction in other forums is permitted, provided the original author(s) or licensor are credited and that the original publication in this journal is cited, in accordance with accepted academic practice. No use, distribution or reproduction is permitted which does not comply with these terms.
*Correspondence: Xiao Huang, Institute of Cellular and Developmental Biology, College of Life Sciences, Zhejiang University, 866 Yuhangtang Road, Hangzhou, Zhejiang 310058, China e-mail:eGgyMjBAemp1LmVkdS5jbg==;
Huahong Shi, State Key Laboratory of Estuarine and Coastal Research, East China Normal University, 3663 Zhongshan Road (N), Shanghai 200062, China e-mail:aGhzaGlAZGVzLmVjbnUuZWR1LmNu
Disclaimer: All claims expressed in this article are solely those of the authors and do not necessarily represent those of their affiliated organizations, or those of the publisher, the editors and the reviewers. Any product that may be evaluated in this article or claim that may be made by its manufacturer is not guaranteed or endorsed by the publisher.
Research integrity at Frontiers
Learn more about the work of our research integrity team to safeguard the quality of each article we publish.