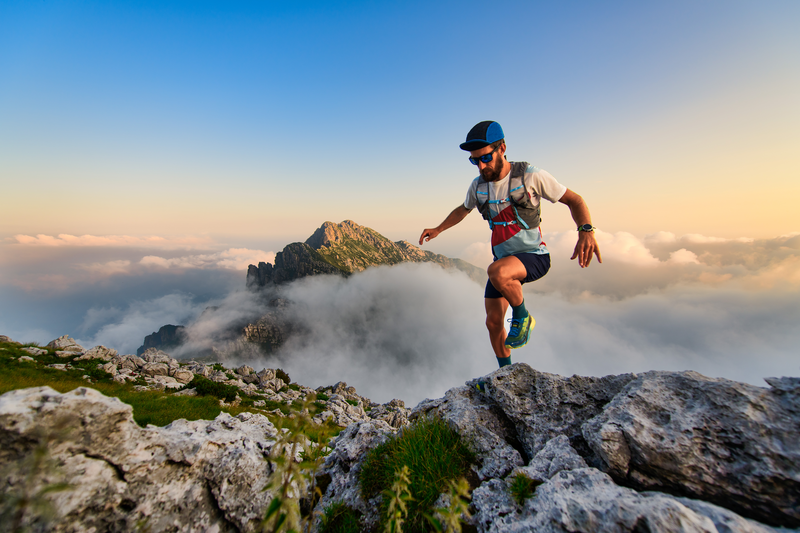
94% of researchers rate our articles as excellent or good
Learn more about the work of our research integrity team to safeguard the quality of each article we publish.
Find out more
BRIEF RESEARCH REPORT article
Front. Manuf. Technol. , 25 April 2022
Sec. Additive Processes
Volume 2 - 2022 | https://doi.org/10.3389/fmtec.2022.863593
Objective: The purpose of this study was to measure the surface roughness and compare the fit of a cobalt-chromium selective laser-melted removable partial denture framework and a titanium binder jetting removable partial denture framework.
Materials and methods: A design for a removable partial prosthesis framework was made using 3Shape software, and thereafter, one framework was additively manufactured in cobalt-chromium with selective laser melting and one in titanium using binder jetting technology. The weight of the frameworks was measured, and the surface parameters Sa, Sds, and Sdr were measured by white light interferometry. The fit of the frameworks was analyzed using visual inspection and the pressing test.
Results: The weight of the frameworks in their post-printed state was 15.66 g and 7.43 g for cobalt-chromium and titanium, respectively. After finishing and polishing, the cobalt-chromium framework’s outer surface showed a high shine and smooth surface, with lower values in the Sa and Sdr parameters. The visual inspection showed gaps in the titanium framework, and the pressing test detected movement greater than 0.5 mm for the same material.
Conclusion: The Ti framework showed higher surface roughness and was left with a lackluster finish after polishing. Also, the fit of the Ti framework was not clinically acceptable. The additive manufacturing binder jetting technology for titanium needs improvement or an adjustment of the settings to be used for clinically removable partial dentures. The cobalt-chromium framework manufactured by selective laser melting showed an acceptable clinical fit and surface roughness.
Metal frameworks for removable partial dentures (RPDs) have traditionally been fabricated using the lost-wax technique, which is a laborious, manual, and material- and time-consuming process that involves several steps where errors are prone to occur (Rudd and Rudd, 2001; Lima et al., 2014). Computer-aided design/computer-aided manufacturing (CAD/CAM) technology for the fabrication of fixed dental prostheses is well-developed and has been used in dentistry for more than 30 years (Miyazaki et al., 2009). For the fabrication of RPDs, this technology is relatively new. The development of CAD/CAM technology in the field of RPDs was, in the beginning, limited due to the lack of dedicated software that could support digital surveying and design in CAD, and by the lack of an appropriate manufacturing technique (Williams et al., 2004). In the fabrication of metal frameworks for RPDs that include components with complex shapes that must be thin, the subtractive method is not a suitable choice. It is not easy to cut the complicated shapes and/or undercut areas. Milling of hard metals requires heavy cutting forces for efficient material removal. These cutting conditions cause excessive vibration and exert thermal and mechanical stresses on the workpiece, which can contribute to dimensional distortion, especially on thin parts. Additive manufacturing (AM) has the advantage of being able to produce large objects with surface irregularities, undercuts, voids, and hollow morphology, which makes it more suitable for manufacturing metal RPD frameworks. The passive nature of the manufacturing technique, due to the lack of force application and vibration of the machine during the production of the workpiece, allows the production of delicate and thin structures. The amount of wasted raw material in this technique is also much lower than that in the subtractive technique (Abduo et al., 2014; Lima et al., 2014; Barazanchi et al., 2017; Ohkubo et al., 2017). A problem with the AM technique for the fabrication of dental metal objects is that the printed objects are left with a much rougher surface than objects manufactured by either subtractive or lost-wax techniques (Fernández et al., 2014; Ohkubo et al., 2017). The surface roughness varies between different AM processing techniques but is also influenced by processing parameters used during the build process (Hong et al., 2016; Fousová et al., 2018).
In recent years, research and development of specific software for the designing of RPDs together with evaluation of AM techniques for fabricating RPDs has made it possible to design and manufacture RPD frameworks by CAD/CAM (Lima et al., 2014). The design in CAD offers advantages such as automatic determination of a suggested path of insertion, almost instant elimination of unwanted undercuts, and the making of a virtual pattern in a much faster time than is achieved using the traditional lost-wax technique (Han et al., 2010).
The first metal frameworks of removable partial dentures produced by the design in CAD and AM techniques used additively manufactured epoxy-based and acrylate-based polymer sacrificial patterns. The sacrificial patterns were invested and cast (Williams et al., 2004; Eggbeer et al., 2005). It was found that stereolithography (SLA) was the most suitable choice of the additive process for the fabrication of a sacrificial epoxy-based resin pattern that produced satisfactory casting results (Eggbeer et al., 2005). Further development of CAD/CAM and additive technologies in RPD framework fabrication was achieved by omitting the casting stage. The direct manufacturing of an RPD metal framework in a cobalt-chromium alloy (CoCr) was produced directly from CAD data and selective laser melting (SLM) technology (Richard Bibb et al., 2006; Williams et al., 2006).
To evaluate the fit of RPD frameworks, visual inspection and pressing tests have been proposed and described as tests commonly used and accepted in clinical practice. For example, occlusal rests are pressured to detect whether there is movement, and the adaption of the clasp and rigid elements to the teeth are assessed visually (Eggbeer et al., 2005; Han et al., 2010; Ye et al., 2017). RPD frameworks in CoCr obtained by CAD and AM with SLA or SLM technologies have shown an acceptable clinical fit, comparable with frameworks produced using the lost-wax technique, when subjectively evaluated by visual inspection and pressing tests (Richard Bibb et al., 2006; Bibb R. J. et al., 2006; Williams et al., 2006; Ye et al., 2017).
AM with titanium (Ti) powder in the production of removable dental applications has been attempted (Tan et al., 2019). Kanazawa et al. fabricated a Ti alloy framework for a maxillary complete denture by using the SLM technique and found only small internal gaps in the palatal framework when placed on the stone master model. However, they also stated that a more precise fitting evaluation is needed (Kanazawa et al., 2014). Due to its low density, the titanium RPDs are lighter than CoCr RPDs and would therefore be more suitable for use in larger RPD frameworks to offer more comfort for the patient. Other advantages of titanium include excellent corrosion resistance and biocompatibility (Ohkubo et al., 2008; Ohkubo et al., 2017).
The surface roughness of RPD frameworks has been reported in a few studies mainly analyzing the surface of rests or clasps. In these studies, cast CoCr has been reported to have an Ra value of 9.96–10.15 μm for the clasp arm and 2.4–2.5 μm for the rest (Kajima et al., 2016; Nakata et al., 2017; Torii et al., 2018). The surface roughness of the SLM CoCr is affected by the build angle: the 90° build angle has a lower Ra value than 0° and 45°, 2.37–10.22 μm for the clasp arm (Kajima et al., 2016; Kittikundecha et al., 2019).
To build the RPD framework with an SLM machine, adequate supports are needed to support the build material and prevent it from collapsing. In addition, the support acts as a heat conductor as the material melts and solidifies during the build process. Inadequate support can result in incomplete parts or heat-induced curls, which may result in the build failure. The supports are either manually or automatically designed and fabricated along with the object. When the build process is finished, the support structures are removed with cutting tools. To avoid the rough finish created by the support structures or the risk of damaging the fitting surface of the RPD framework during the removal of the support, the framework is oriented such that the support avoids the fitting surface (Richard Bibb et al., 2006; Williams et al., 2006; Han et al., 2010). Despite the promising benefit of a much faster “digital workflow” with the CAD/CAM AM technique than with the lost-wax technique in the making of RPDs, the removal of the support structures are time-consuming (Alharbi et al., 2016; Campbell et al., 2017). The purpose of this study was to measure the surface roughness and compare the fit of a CoCr SLM RPD framework and a Ti binder jetting RPD framework.
A type IV dental stone model of a partially edentulous maxilla with the anterior teeth 24, 17, and 18 remaining was created from a silicon mold. An experienced dental technician scanned the stone model using a laboratory scanner (D700 3shape Copenhagen, Denmark), and an appropriate framework of an RPD to fit the stone model was designed using CAD (Dental manager, 3shape, Copenhagen Denmark). Virtual surveying was performed, a suggestion of an ideal path of insertion was offered, unwanted undercuts were blocked, appropriate components of the RPD framework were designed, and the components were combined to form the RPD framework. Extra material was manually applied to the minor connectors to achieve a smooth transition between the different components. The design data of the RPD framework were saved as an STL file. The file was then sent to a production center (Forstec Open mill, Malmö, Sweden) for the fabrication of a CoCr metal framework by using the SLM technique (CoCr Mediloy S-Co. Co 63.9%, Cr 24.7, W 5.4%, Mo 5.0%, Si 1.0%; grain size 10–45 μm. Bego, Bremen, Germany), and to Digital Metal (Höganäs, Sweden) for the fabrication of a Ti RPD framework with Digital Metal P2500 binder jetting technology (Ti alloy Digital Metal ISO 22068. Al 6%, V 4%, oxygen < 0.30%, carbon < 0.20%).
The two frameworks were weighed on a digital scale (Sauter precision balance RE 2012) and test-fitted to the stone model in their post-printed state. The two frameworks were then analyzed for surface roughness and evaluated for fit to the stone model. Prior to surface analysis and evaluation of fit, the intaglio surfaces of the frameworks were carefully processed with a small round bur for the removal of the post-printed surface layer. When fresh metal subjectively could be seen during the removal of the surface layer, no further treatment was performed in that area. The outer surfaces of the frameworks were finished and polished using conventional methods (Lima et al., 2014): removal of the post-printed rough surface by grinding with tungsten carbide millers (Rudd and Rudd, 2001) and polishing with fine abrasive rubber wheels (for the removal of scratches from the previous grinding procedure) (Miyazaki et al., 2009), followed by the use of finer polishing rubber wheels, brushes, and polishing paste for final polishing. When no further improvement in surface smoothness could be observed subjectively, the finishing and polishing were terminated. The mesh bases of the frameworks were left untouched.
The surface roughness was examined utilizing white light interferometry (Smart WLI-extended optical 3D surface Measuring system GBS, mbH, Ilmenau, Germany), and software was used to perform the surface measurements and evaluate the measurements (smartVIS 3D/Mountains MAP, Digital surf, France). Four arbitrary areas were randomly selected: areas on the surfaces of the prepared intaglio, outer surfaces of the frameworks, and the intaglio and outer surfaces of the mesh bases. Each measurement had a size of 360 × 226 μm. To numerically describe the surface, parameters Sa, Sds, and Sdr were selected (ISO 25178). Sa (μm) is the arithmetic mean roughness of a surface. Sds (1/μm2) describes the density of peaks per unit of area. Sdr (%) represents the developed surface area ratio.
The finished and polished frameworks were test-fitted to the stone model. If needed, any necessary adjustments were made using tungsten carbide burs to fit the frameworks to the stone model. Visual inspection and pressing tests were used to assess the fit of the frameworks, methods which have been used to analyze the fit of RPD frameworks to stone models in previous studies (Eggbeer et al., 2005; Han et al., 2010; Ye et al., 2017). Based on a method for the clinical evaluation of RPDs proposed by Frank et al. (2000), two modified categories were used in this study to assess the fit of the frameworks (Lima et al., 2014): visual inspection, whether all rigid elements touch the teeth (Rudd and Rudd, 2001), and the degree of the base support, observing the extent of movement when applying pressure to the most anterior point of contact between the framework and stone teeth. The base support was divided into less or more than 0.5 mm of movement. The fit of the frameworks to the stone model was evaluated by an experienced dental technician with more than 20 years of work experience in the fabrication of removable dentures.
The CoCr frameworks were delivered from the production centers with the support structures removed; therefore, no extra laboratory time was needed in the removal. Finishing and polishing of both the CoCr and titanium frameworks could begin in the state they were delivered. The CoCr framework had a weight of 15.66 g, and the Ti framework had a weight of 7.43 g in their post-printed states.
Visually, the post-printed surface of the titanium framework had a fine homogeneous grain-like texture, while the surface of the CoCr framework appeared rougher with small pores and nabs distributed over the surface (Figure 1). However, the post-printed surface of the CoCr framework was easier and faster to remove during grinding than that of the titanium framework. Both frameworks displayed a line-like distribution of pores along the external borders of the outer surfaces. The removal of these required extra grinding and polishing in both cases, especially in the titanium specimen. After finishing and polishing, the CoCr framework’s outer surface showed a high shine and smooth surface. This desired surface finish was not achieved with the titanium framework. Although extensive polishing of the outer surface of the titanium framework was executed, the surface was left with a lackluster finish. The roughness values of the two frameworks obtained by white light interferometry are listed in Table 1.
TABLE 1. Mean values ± standard deviations of surface roughness at four different areas of the frameworks.
The CoCr framework was easily fit to the stone model, and after removal of the post-printed surface, no further adjustments were needed. The titanium framework did not fit to the stone model, and adjustments with tungsten carbide burs had to be done on the minor connectors, dental strap, and reciprocal arm before it could be fit.
The results of the visual inspection and pressing test of the CoCr and the Ti frameworks are, respectively, presented in Table 2. The fit of the CoCr framework was rated as clinically acceptable. The fit of the Ti framework was rated as not clinically acceptable (Figure 2).
The purpose of this study was to investigate if an additive manufacturing technique to produce an RPD metal framework in titanium could be compared to a CoCr framework manufactured using the SLM technique. The titanium framework was 8 g lighter than the CoCr framework, most likely due to the lower density of titanium. Both frameworks were delivered without support structures, but more time was needed in making adjustments of the fit and polishing of the Ti framework, and the fit still did not meet the clinical acceptance and the outer surface was left with a lackluster finish.
These subjective findings were also consistent with the results from the surface analysis. The outer surface of the CoCr framework had lower Sa and Sdr mean values than the outer surface of the Ti framework, while the Sds mean value was higher. This indicates that the surface roughness of the CoCr framework was lower than that of the Ti framework. The lower Sdr value of the CoCr outer surface points to the fact that although the number of peaks per unit area was higher than the number of peaks per unit area of the Ti framework, the peaks and valleys did not deviate as much from the mean surface plane. The CoCr intaglio surface also presented lower roughness values than the intaglio surface of the Ti framework. The roughness values of the Ti mesh base outer and intaglio surfaces also implied that the surfaces of the framework had higher roughness in their post-printed states.
Owing to the subjective nature of the evaluation method for assessing the fit of the frameworks in the present study, the results should not be directly compared with the results obtained in other studies. Other studies using similar subjective evaluation methods for assessing the accuracy of fit as the method used in the present study have found the fit of CoCr RPD frameworks fabricated using CAD/CAM SLM techniques to have a good or satisfactory fit (Eggbeer et al., 2005; Han et al., 2010). Ye et al. (2017) evaluated the clinical fit of CoCr RPD frameworks by visual inspection and pressing tests and used a silicon impression material for the quantitative evaluation of occlusal rest seat adaption. They found that although the average gap between the occlusal rest and rest seat of the SLM RPDs was significantly larger than that of the investment casting group, the SLM RPDs met the clinical needs when evaluated by visual inspection and pressing tests. Using light microscopy, Arnold et al. (2018) evaluated the fit of RPD clasps fabricated by means of five different methods: SLA (castable), SLM (direct), lost-wax technique, and two types of subtractive (direct and castable) methods. Before measurement, the frameworks were rated subjectively for design and stability. The frameworks in the direct and indirect AM groups showed reduced stability to transverse and sagittal movements. Also, the retentive clasp fabricated by AM techniques exhibited the highest discrepancies. During the fabrication process, the build orientation, layer thickness, and other processing parameters affected the accuracy of the printed parts (Kulkarni et al., 2000; Alharbi et al., 2016; Maamoun et al., 2018). The settings of these parameters used during the manufacturing process could be the reason for the reduced stability found with the AM-produced framework.
In addition to the fact that each AM technique has its own inherent processing accuracy (Alharbi et al., 2017), variations in the use of processing parameters between the two manufacturing techniques examined in the present study could also explain the difference in the fit and stability found between the two frameworks. Also, during the AM build process, model features like overhang or undercuts need to be supported either by the build material itself or by support structures to prevent the build material from collapsing (Liu et al., 2006). Inadequate support may also lead to the deformation of parts due to heat-induced stress, causing the unsupported parts to warp (Mumtaz et al., 2011). Inadequate support may be another reason for the inferior fit observed with the Ti framework in the present study. Also, residual stresses within the material could lead to warping post-build. The ease of processing the build material varies between different metals, and the processing of CoCr has been reported as easier to control in comparison with Ti (Kruth et al., 2005; Vandenbroucke and Kruth, 2007). Therefore, a CoCr framework produced by the AM technique (Digital Metal P2500 binder jetting technology) using no support structures and examined as in the present study may have shown a better fit than the Ti framework.
Support structures have been reported to increase the surface roughness at the point of contact with the part being manufactured and prolong the time needed for finishing and polishing (Kulkarni et al., 2000; Alharbi et al., 2016). Still, lower roughness was observed with the CoCr framework, and the time needed for finishing and polishing was shorter than that for the Ti framework. The surface quality of AM-fabricated parts has been observed to be influenced by the build orientation, layer thickness, and process parameter settings (Vandenbroucke and Kruth, 2007; Hong et al., 2016) and by different AM-processing techniques (Fousová et al., 2018). As discussed previously, the use of different processing techniques and variations in the process parameter settings could offer an explanation for the difference in the roughness observed between the two frameworks. Using CoCr and Ti metal powders to produce two SLM-fabricated objects, Vandenbroucke and Kruth (2007) found that the Ti samples showed higher roughness than the CoCr samples even though a lower particle size was used. This was explained by the difference in physical properties between the two materials giving more stable melt pools for CoCr. Antanasova et al. (2018) also found higher surface roughness of the Ti samples fabricated by SLM than the CoCr samples, but the difference was not significant. A higher surface roughness observed for the Ti frameworks in the present study may be due to the use of different manufacturing techniques, parameter settings, or the material itself.
The RPD frameworks in the present study were not electropolished, a procedure normally used in the finishing and polishing part of RPD frameworks (Brudvik and Reimers, 1992). If electropolished, the Ti framework could have obtained a smoother surface. On the other hand, Kevser Aydin (1991) found that the achieved smoothness of the electropolished object was dependent on the surface finish prior to the procedure, which indicates that the CoCr framework would still obtain higher smoothness. The loss of metal due to electropolishing could further have worsened the fit of the titanium framework (Brudvik and Reimers, 1992). The finishing and polishing of the frameworks were performed with separate tools but with similar patterns of blade distribution to produce as comparable effects on the surfaces as possible. The finishing and polishing procedures were performed by one operator using the same protocol. Still, variations in the applied cutting force and magnitude could have affected the distribution and depth of the scratches and cuts, affecting the surface roughness of the frameworks differently.
The RPD frameworks in the present study were designed without any occlusal rests or active clasps. This might have caused excess movements during the pressing test due to the loss of stability that these RPD parts may have contributed to. Still, the CoCr framework achieved an acceptable clinical fit, indicating that SLM as a manufacturing technique could produce frameworks in CoCr with sufficient fit. The results from this study suggest that different methods of AM using different metals for an RPD framework may produce different levels of accuracy of fit and surface roughness.
The Ti framework showed higher surface roughness and was left with a lackluster finish after polishing. Also, the fit of the Ti framework was not clinically acceptable. The AM binder jetting technology for titanium needs improvement or an adjustment of the settings to be used for clinically removable partial dentures. The CoCr framework manufactured by selective laser melting showed an acceptable clinical fit and surface roughness.
The original contributions presented in the study are included in the article/Supplementary Material, further inquiries can be directed to the corresponding author.
All authors listed have made a substantial, direct, and intellectual contribution to the work and approved it for publication.
The study was supported by the Wilhelm and Martina Lundgren Science Foundation 2017-1616.
The authors declare that the research was conducted in the absence of any commercial or financial relationships that could be construed as a potential conflict of interest.
All claims expressed in this article are solely those of the authors and do not necessarily represent those of their affiliated organizations, or those of the publisher, the editors, and the reviewers. Any product that may be evaluated in this article, or claim that may be made by its manufacturer, is not guaranteed or endorsed by the publisher.
The authors would like to thank dental technician Johan Oxby from TVG Dentalteknik (Lysekil, Sweden) for help with scanning and design of the prosthesis and dental technician Hans Pehrson of Svea Dentallab (Gothenburg, Sweden) for assistance in fit evaluation. Also, Ann Wennerberg is thanked for guidance in surface measurements. Forstec Open Mill (Malmö, Sweden) performed the build of the CoCr framework and Höganäs Digital Metal (Höganäs, Sweden) the titanium framework.
Abduo, J., Lyons, K., and Bennamoun, M. (2014). Trends in Computer-Aided Manufacturing in Prosthodontics: a Review of the Available Streams. Int. J. Dent. 2014, 783948. doi:10.1155/2014/783948
Alharbi, N., Osman, R., and Wismeijer, D. (2016). Factors Influencing the Dimensional Accuracy of 3D-Printed Full-Coverage Dental Restorations Using Stereolithography Technology. Int. J. Prosthodont. 29 (5), 503–510. doi:10.11607/ijp.4835
Alharbi, N., Wismeijer, D., and Osman, R. (2017). Additive Manufacturing Techniques in Prosthodontics: Where Do We Currently Stand? A Critical Review. Int. J. Prosthodont. 30 (5), 474–484. doi:10.11607/ijp.5079
Antanasova, M., Kocjan, A., Kovač, J., Žužek, B., and Jevnikar, P. (2018). Influence of Thermo-Mechanical Cycling on Porcelain Bonding to Cobalt-Chromium and Titanium Dental Alloys Fabricated by Casting, Milling, and Selective Laser Melting. J. Prosthodontic Res. 62 (2), 184–194. doi:10.1016/j.jpor.2017.08.007
Arnold, C., Hey, J., Schweyen, R., and Setz, J. M. (2018). Accuracy of CAD-CAM-Fabricated Removable Partial Dentures. J. Prosthet. Dent. 119 (4), 586–592. doi:10.1016/j.prosdent.2017.04.017
Barazanchi, A., Li, K. C., Al-Amleh, B., Lyons, K., and Waddell, J. N. (2017). Additive Technology: Update on Current Materials and Applications in Dentistry. J. Prosthodont. 26 (2), 156–163. doi:10.1111/jopr.12510
Bibb, R. J., Eggbeer, D., Williams, R. J., and Woodward, A. (2006). Trial Fitting of a Removable Partial Denture Framework Made Using Computer-Aided Design and Rapid Prototyping Techniques. Proc. Inst. Mech. Eng. H 220 (7), 793–797. doi:10.1243/09544119jeim62
Bibb, R., Eggbeer, D., and Williams, R. (2006). Rapid Manufacture of Removable Partial Denture Frameworks. Rapid Prototyping J. 12 (2), 95–99. doi:10.1108/13552540610652438
Brudvik, J. S., and Reimers, D. (1992). The Tooth-Removable Partial Denture Interface. J. Prosthet. Dent. 68 (6), 924–927. doi:10.1016/0022-3913(92)90552-l
Campbell, S. D., Cooper, L., Craddock, H., Hyde, T. P., Nattress, B., Pavitt, S. H., et al. (2017). Removable Partial Dentures: The Clinical Need for Innovation. J. Prosthet. Dent. 118 (3), 273–280. doi:10.1016/j.prosdent.2017.01.008
Eggbeer, D., Bibb, R., and Williams, R. (2005). The Computer-Aided Design and Rapid Prototyping Fabrication of Removable Partial Denture Frameworks. Proc. Inst. Mech. Eng. H 219 (3), 195–202. doi:10.1243/095441105x9372
Fernández, M., Delgado, L., Molmeneu, M., García, D., and Rodríguez, D. (2014). Analysis of the Misfit of Dental Implant-Supported Prostheses Made with Three Manufacturing Processes. J. Prosthet. Dent. 111 (2), 116–123. doi:10.1016/j.prosdent.2013.09.006
Fousová, M., Vojtěch, D., Doubrava, K., Daniel, M., and Lin, C-F. (2018). Influence of Inherent Surface and Internal Defects on Mechanical Properties of Additively Manufactured Ti6Al4V alloy: Comparison between Selective Laser Melting and Electron Beam Melting. J. Mater. 11 (4), 537. doi:10.3390/ma11040537
Frank, R. P., Brudvik, J. S., Leroux, B., Milgrom, P., and Hawkins, N. (2000). Relationship between the Standards of Removable Partial Denture Construction, Clinical Acceptability, and Patient Satisfaction. J. Prosthet. Dent. 83 (5), 521–527. doi:10.1016/s0022-3913(00)70008-4
Han, J., Wang, Y., and Lü, P. (2010). A Preliminary Report of Designing Removable Partial Denture Frameworks Using a Specifically Developed Software Package. Int. J. Prosthodont. 23 (4), 370–375.
Hong, M.-H., Min, B., and Kwon, T.-Y. (2016). The Influence of Process Parameters on the Surface Roughness of a 3D-Printed Co-cr Dental Alloy Produced via Selective Laser Melting. Appl. Sci. 6 (12), 401. doi:10.3390/app6120401
Kajima, Y., Takaichi, A., Nakamoto, T., Kimura, T., Yogo, Y., Ashida, M., et al. (2016). Fatigue Strength of Co-cr-Mo alloy Clasps Prepared by Selective Laser Melting. J. Mech. Behav. Biomed. Mater. 59, 446–458. doi:10.1016/j.jmbbm.2016.02.032
Kanazawa, M., Iwaki, M., Minakuchi, S., and Nomura, N. (2014). Fabrication of Titanium alloy Frameworks for Complete Dentures by Selective Laser Melting. J. Prosthet. Dent. 112 (6), 1441–1447. doi:10.1016/j.prosdent.2014.06.017
Kevser Aydin, A. (1991). Evaluation of Finishing and Polishing Techniques on Surface Roughness of Chromium-Cobalt Castings. J. Prosthet. Dent. 65 (6), 763–767. doi:10.1016/s0022-3913(05)80008-3
Kittikundecha, N., Kajima, Y., Takaichi, A., Wai Cho, H. H., Htat, H. L., Doi, H., et al. (2019). Fatigue Properties of Removable Partial Denture Clasps Fabricated by Selective Laser Melting Followed by Heat Treatment. J. Mech. Behav. Biomed. Mater. 98, 79–89. doi:10.1016/j.jmbbm.2019.06.010
Kruth, J. P., Mercelis, P., Van Vaerenbergh, J., Froyen, L., and Rombouts, M. (2005). Binding Mechanisms in Selective Laser Sintering and Selective Laser Melting. Rapid Prototyping J. 11 (1), 26–36. doi:10.1108/13552540510573365
Kulkarni, P., Marsan, A., and Dutta, D. (2000). A Review of Process Planning Techniques in Layered Manufacturing. Rapid Prototyping J. 6 (1), 18–35. doi:10.1108/13552540010309859
Lima, J. M. C., Anami, L. C., Araujo, R. M., and Pavanelli, C. A. (2014). Removable Partial Dentures: Use of Rapid Prototyping. J. Prosthodont. 23 (7), 588–591. doi:10.1111/jopr.12154
Liu, Q., Leu, M. C., and Schmitt, S. M. (2006). Rapid Prototyping in Dentistry: Technology and Application. Int. J. Adv. Manufact Tech. 29 (3), 317–335. doi:10.1007/s00170-005-2523-2
Maamoun, A. H., Xue, Y. F., Elbestawi, M. A., and Veldhuis, S. C. (2018). Effect of Selective Laser Melting Process Parameters on the Quality of Al Alloy Parts: Powder Characterization, Density, Surface Roughness, and Dimensional Accuracy. Materials (Basel) 11 (12), 2343. doi:10.3390/ma11122343
Miyazaki, T., Hotta, Y., Kunii, J., Kuriyama, S., and Tamaki, Y. (2009). A Review of Dental CAD/CAM: Current Status and Future Perspectives from 20 Years of Experience. Dent. Mater. J. 28 (1), 44–56. doi:10.4012/dmj.28.44
Mumtaz, K., Vora, P., and Hopkinson, N. (2011). “A Method to Eliminate Anchors/supports from Directly Laser Melted Metal Powder Bed Processes,” in Proc Solid Freeform Fabrication Symposium, Austin, Texas, August 8–10, 2011. Sheffield.
Nakata, T., Shimpo, H., and Ohkubo, C. (2017). Clasp Fabrication Using One-Process Molding by Repeated Laser Sintering and High-Speed Milling. J. Prosthodontic Res. 61 (3), 276–282. doi:10.1016/j.jpor.2016.10.002
Ohkubo, C., Hanatani, S., and Hosoi, T. (2008). Present Status of Titanium Removable Dentures - a Review of the Literature*. J. Oral Rehabil. 35 (9), 706–714. doi:10.1111/j.1365-2842.2007.01821.x
Ohkubo, C., Sato, Y., Nishiyama, Y., and Suzuki, Y. (2017). Titanium Removable Denture Based on a One-Metal Rehabilitation Concept. Dent. Mater. J. 36 (5), 517–523. doi:10.4012/dmj.2017-137
Rudd, R. W., and Rudd, K. D. (2001). A Review of 243 Errors Possible during the Fabrication of a Removable Partial Denture: Part I. J. Prosthet. Dent. 86 (3), 251–261. doi:10.1067/mpr.2001.118021
Tan, F.-B., Song, J.-L., Wang, C., Fan, Y.-B., and Dai, H.-W. (2019). Titanium Clasp Fabricated by Selective Laser Melting, CNC Milling, and Conventional Casting: a Comparative In Vitro Study. J. Prosthodontic Res. 63 (1), 58–65. doi:10.1016/j.jpor.2018.08.002
Torii, M., Nakata, T., Takahashi, K., Kawamura, N., Shimpo, H., and Ohkubo, C. (2018). Fitness and Retentive Force of Cobalt-Chromium alloy Clasps Fabricated with Repeated Laser Sintering and Milling. J. Prosthodontic Res. 62 (3), 342–346. doi:10.1016/j.jpor.2018.01.001
Vandenbroucke, B., and Kruth, J. P. (2007). Selective Laser Melting of Biocompatible Metals for Rapid Manufacturing of Medical Parts. Rapid Prototyping J. 13 (4), 196–203. doi:10.1108/13552540710776142
Williams, R. J., Bibb, R., and Rafik, T. (2004). A Technique for Fabricating Patterns for Removable Partial Denture Frameworks Using Digitized Casts and Electronic Surveying. J. Prosthet. Dent. 91 (1), 85–88. doi:10.1016/j.prosdent.2003.10.002
Williams, R. J., Bibb, R., Eggbeer, D., and Collis, J. (2006). Use of CAD/CAM Technology to Fabricate a Removable Partial Denture Framework. J. Prosthet. Dent. 96 (2), 96–99. doi:10.1016/j.prosdent.2006.05.029
Keywords: denture, partial, removable, titanium, cobalt, chromium. additive manufacturing
Citation: Hagman C and Svanborg P (2022) Additive Manufacturing of a Removable Partial Prosthesis in Titanium Using Binder Jetting Technology: A Brief Research Report. Front. Manuf. Technol. 2:863593. doi: 10.3389/fmtec.2022.863593
Received: 27 January 2022; Accepted: 24 March 2022;
Published: 25 April 2022.
Edited by:
Panagiotis Kyratsis, University of Western Macedonia, GreeceReviewed by:
Nikolaos Tapoglou, The University of Sheffield, United KingdomCopyright © 2022 Hagman and Svanborg. This is an open-access article distributed under the terms of the Creative Commons Attribution License (CC BY). The use, distribution or reproduction in other forums is permitted, provided the original author(s) and the copyright owner(s) are credited and that the original publication in this journal is cited, in accordance with accepted academic practice. No use, distribution or reproduction is permitted which does not comply with these terms.
*Correspondence: Per Svanborg, cGVyLnN2YW5ib3JnQGd1LnNl
†These authors have contributed equally to this work and share first authorship
Disclaimer: All claims expressed in this article are solely those of the authors and do not necessarily represent those of their affiliated organizations, or those of the publisher, the editors and the reviewers. Any product that may be evaluated in this article or claim that may be made by its manufacturer is not guaranteed or endorsed by the publisher.
Research integrity at Frontiers
Learn more about the work of our research integrity team to safeguard the quality of each article we publish.