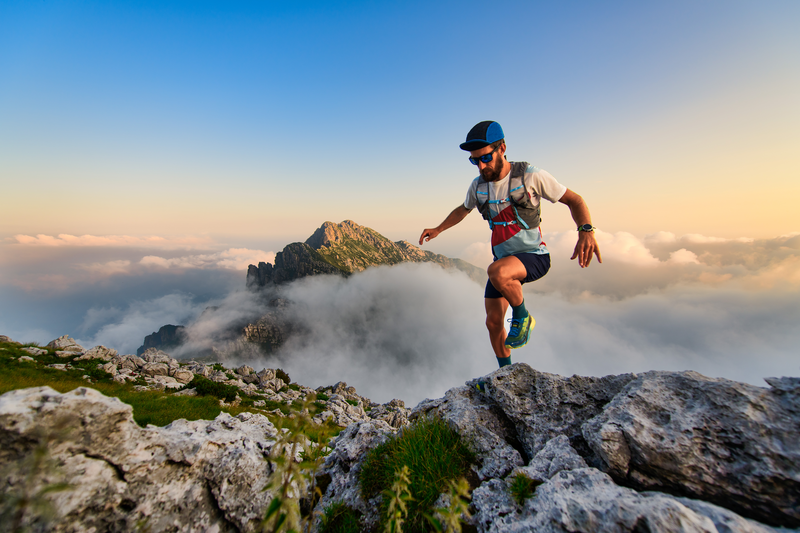
94% of researchers rate our articles as excellent or good
Learn more about the work of our research integrity team to safeguard the quality of each article we publish.
Find out more
ORIGINAL RESEARCH article
Front. Malar. , 31 January 2025
Sec. Case Management
Volume 3 - 2025 | https://doi.org/10.3389/fmala.2025.1545825
This article is part of the Research Topic Women in Malaria Research View all 13 articles
Background: Malaria remains a significant health burden, particularly in Uganda, which has one of the highest incidence rates globally. Rapid diagnostic tests (RDTs) are widely used for malaria diagnosis due to their ease of use and affordability in resource-limited settings. However, false-positive RDT results may lead to unnecessary antimalarial treatments and missed diagnoses of other febrile conditions. This study aimed to evaluate the diagnostic accuracy of RDT compared to microscopy and loop-mediated isothermal amplification (LAMP) in a malaria-endemic region of Uganda.
Methods: A prospective study was conducted with 225 febrile individuals diagnosed with malaria using RightSign Malaria Ag HRPII/Pan Plasmodium Aldolase RDT (Hangzhou Biotest Biotech Co., Ltd., China) at Iganga General Hospital, Uganda. Blood samples were analyzed using microscopy and LAMP. Demographic information, malaria history, and parasitemia levels were also recorded.
Results: Among the RDT-positive participants, 48% tested negative by microscopy, and 45% of these were also negative by LAMP, indicating that 20% of all RDT-positive individuals were likely false positives. Overall, 66% (149/225) of RDT-positive cases were confirmed as true positives.
Conclusions: This study identified a high rate of false positives with the tested RDT, risking inappropriate treatment and missed diagnoses of other illnesses. It is essential that healthcare facilities use RDTs validated and recommended by the World Health Organization (WHO) to ensure high specificity and accuracy, particularly in resource-limited settings where alternative diagnostic methods may not be available.
Malaria, a life-threatening disease caused by Plasmodium parasites and transmitted through the bite of female Anopheles mosquitoes, remains a significant global health challenge. In 2023, an estimated 263 million cases and 597 000 deaths were reported, with the majority occurring in sub-Saharan Africa (World Health Organization, 2024). Uganda, one of the most affected nations, has an annual incidence rate of 268 cases per 1000 inhabitants, reflecting the severe disease burden in the area (World Health Organization, 2024). Beyond its severe health impact, malaria imposes a substantial socioeconomic burden in endemic regions. While significant progress has been made in controlling the disease through advancements in diagnostics, treatment, and vector control, numerous challenges remain. One key issue is the need for more sensitive and specific diagnostic tools to guide appropriate treatment and reduce unnecessary antimalarial use, especially given the increasing resistance to artemisinin-based therapies (World Health Organization, 2024).
In malaria-endemic regions, WHO recommends considering malaria in individuals with a fever, or history of fever exceeding 37.5°C, when no other cause is apparent (World Health Organization, 2023). Since 2012, parasitological testing of all suspected cases has been advised using methods such as light microscopy and rapid diagnostic tests (RDTs) in endemic areas. To detect low level parasitemia, or to detect parasites in the blood in a non-endemic country where microscopy is not available 24/7, polymerase chain reaction (PCR) or loop-mediated isothermal amplification (LAMP) can be used. LAMP, a molecular technique, has a sensitivity comparable to PCR and detects as few as 1-2 parasites/µL, but offers the advantages of simplicity and speed (Cook et al., 2015; Puri et al., 2022). In contrast, microscopy and RDTs have higher detection thresholds, at 50-100 and 100 parasites/µL, respectively, limiting their sensitivity in cases of low parasite densities (Puri et al., 2022).
Despite its advantages, LAMP, like PCR, requires electricity and well-maintained equipment, which are often unavailable in low-resource settings. As a result, LAMP is not routinely used in clinical settings in malaria-endemic regions like Uganda and is generally limited to research. In comparison, malaria RDTs are easy to use, relatively inexpensive and widely implemented. RDTs detect malaria antigens such as histidine-rich protein 2 (HRP2), specific to Plasmodium falciparum (P. falciparum), or pan-specific markers like aldolase and Plasmodium lactate dehydrogenase (pLDH). The introduction of RDTs marked a significant breakthrough in the field of malaria diagnostics, contributing to a notable reduction in the prescription of artemisinin combination therapies (ACTs) for malaria-like febrile illnesses and improving the accuracy of reported malaria cases in the following years (Aidoo and Incardona, 2021).
However, a significant challenge associated with RDTs is the potential for false-positive results, leading to misdiagnosis that can delay appropriate care for bacterial or viral infections. Unnecessary antimalarial treatments not only increase healthcare costs but contribute to the growing threat of drug resistance. In a broader context, false-positive malaria results also impact public health surveillance, affecting statistics important for resource allocation, policy formulation, and health program management. Various factors contribute to false-positive RDT outcomes. HRP2 persistence in the bloodstream following effective malaria treatment can lead to false-positive results for several weeks (Grandesso et al., 2016). Infections such as human trypanosomiasis (Gillet et al., 2013), schistosomiasis (Leshem et al., 2011) and leishmaniasis (Unterborn et al., 2022), can occasionally lower RDT specificity by causing cross-reactivity. Furthermore, the presence of rheumatoid factor (RF) is known to influence test accuracy, with false-positive rates ranging from 2.2 to 13%, depending on the RDT type and manufacturer (Lee et al., 2014; Gatton et al., 2018).
Few studies have focused on the issue of false-positive results when evaluating RDTs in clinical settings. This study aimed to evaluate the diagnostic accuracy of a widely used malaria RDT in a district hospital in Uganda, specifically examining the potential for overdiagnosis of malaria in a real-world context. We compared the RDT with microscopy and LAMP in febrile children and adults from a malaria-endemic region. Additionally, we explored the implications of false-positive RDT results, including the overuse of antimalarial treatment and the potential for missed diagnoses of non-malarial fevers. Addressing these issues is essential for improving health outcomes and optimizing healthcare interventions in malaria-endemic settings.
This prospective study, previously detailed elsewhere (Mortazavi et al., 2024), was conducted from March to December 2022 at Iganga General Hospital, 120 kilometers east of Uganda’s capital, Kampala. The region experiences year-round malaria transmission, with peaks during the rainy seasons of April-June and September-December. P. falciparum is the predominant species in the area (Kaddumukasa et al., 2014). Participants included adults and children presenting at the Outpatient Department with acute malaria diagnosed via RDT. Inclusion and exclusion criteria were based on the parent study, which focused on antibody responses. Inclusion criteria were a history of fever within the past 24 hours, axillary temperature ≥ 37.5°C, hemoglobin > 5 g/dl, P. falciparum infection diagnosed by RDT following the manufacturer’s instructions, and informed written consent from participants or guardians for minors. Infants under 6 months were excluded due to maternal antibodies, and individuals with recent blood transfusions, and chronic conditions such as sickle cell disease, severe malnutrition, cancer, or HIV were excluded due to potential interference with immune function. After obtaining consent, demographic data, malaria history, anti-malarial treatment, and usage of insecticide-treated bed nets (ITNs) and indoor residual spraying (IRS) were recorded.
Blood samples for on-site RDT and venous blood (5 mL from adults, 2 mL from children) were collected in ethylenediaminetetraacetic acid (EDTA) tubes and transported to Makerere University Biomedical Cross-Cutting Laboratory within 4 hours for processing. Peripheral blood mononuclear cells (PBMCs) and plasma were separated, aliquoted, and stored at -80°C. To ensure sample integrity during international transport, plasma samples were kept frozen using dry ice and shipped to Sweden. Upon arrival in Sweden, the samples were immediately stored at -80°C and only thawed once prior to analysis for LAMP, preserving their quality for testing.
Fresh capillary blood was used for the RDT procedure. The RDT RightSign Malaria Ag HRPII/Pan Plasmodium Aldolase (Hangzhou Biotest Biotech Co., Ltd., China), was conducted following the manufacturer’s instructions. Microscopy of thick blood smears was carried out at the Makerere University Biomedical Cross-cutting laboratory using standard techniques. Two experienced technicians, who were blinded to the others´ results, independently assessed the slides, with a third microscopist adjudicating in cases of disagreement. Parasitemia was estimated by counting parasites per 200 white blood cells (WBCs), assuming 8000 WBC/µL. Slides were classified as negative if no Plasmodium asexual forms or gametocytes were observed after counting 500 WBCs.
Plasma samples from microscopy-negative individuals underwent further testing using the LAMP-test HumaTurb C + A Loopamp™ Malaria Pan Detection Kit (Human Diagnostics Worldwide, Wiesbaden, Germany), conducted at the Clinical Microbiology Laboratory of Region Skåne according to the manufacturer’s instructions and as previously described (Ivarsson et al., 2023). Due to logistical constraints, plasma was used for all LAMP testing in this study, as it was the available sample type for analysis. While whole blood is typically used for malaria diagnostics, previous studies indicate that using plasma does not significantly affect the sensitivity, as both plasma and whole blood contain detectable levels of Plasmodium antigen (Kifude et al., 2008).
True positives were defined as RDT-positive cases confirmed by microscopy or LAMP. False positives were defined as RDT-positive cases negative by both microscopy and LAMP.
Continuous data were reported as mean ± standard deviation (SD) for normally distributed variables and median with interquartile range (IQR) for non-normal distributions. Group comparisons were performed using one-way ANOVA with multiple comparisons for parametric data and the Kruskal-Wallis test for non-parametric data. Two-sided P-values were calculated for all tests, with significance set at P < 0.05. All statistical analyses were conducted using GraphPad Prism version 10 (GraphPad Software Inc., San Diego, CA, USA).
225 participants with complete RDT and expert microscopy results were included in the analysis, representing all available samples from the original study cohort (Figure 1). Of these, 61% (n=137) were female, and the median age was 13.5 years (IQR: 6-22), ranging from 1 to 81 years. Children under 5 years constituted 12% (n=28) of the participants. The mean time since the last diagnosed and treated malaria episode was 3.6 months (SD: 1.9). ITN usage was reported by 82% of participants, though not all responded to this question, so the actual percentage could be higher.
Figure 1. Overview of the number of participants included and analyses performed. 31 of the microscopy negative samples were not analyzed by LAMP due to insufficient sample volume.
Half of the participants (52%, n=118) exhibited at least one positive blood smear based on microscopy analysis (Figure 1). The median parasite density was 1360 parasites/µL (IQR: 128 – 13,820), with parasitemia levels ranging from 16 to 189 304 parasites/µL. Among the study participants, 40 individuals (39%) had low-level parasite densities below 500 parasites/µL, while 57 (30%) surpassed 1000 parasites/µL. 55% (n=65) of those with positive microscopy were children under the age of 15. There was no significant difference in median parasitemia levels between adults (1160 parasites/µL, IQR: 144 – 4900) and children under the age of 15 (3336 parasites/µL, IQR: 104 – 28190) (p = 0.06).
Out of the 107 samples that were microscopy-negative, 76 were analyzed with LAMP; the remaining samples could not be tested due to insufficient volumes. Among these, LAMP identified 31 (41%) as positive, while 45 (59%) tested negative. A large proportion of LAMP-positive samples (68%) came from children under the age of 15. There was no significant difference in LAMP positivity rates between children (21 positive, 23 negative) and adults (10 positive, 22 negative) (p = 0.16) (Figure 2).
Figure 2. Distribution of individuals across different groups, illustrating the proportion of adults versus children under 15 years of age. No significant differences were observed between the groups.
In this study population, the malaria prevalence, as determined by positive results from either light microscopy or LAMP, was 66% (149 out of 225 participants). Among the 225 participants, 48% were negative in microscopy, 14% were microscopy-negative but LAMP-positive, and 20% were negative in both microscopy and LAMP. The remaining 14% of microscopy-negative cases could not be tested with LAMP due to limited plasma. No significant differences were observed among the three groups regarding age, time since the last confirmed and treated malaria infection or ITN usage (Table 1).
In our study, we observed that 20% of febrile individuals treated for malaria based on positive RDT results were likely not infected, as confirmed by microscopy and LAMP. These individuals may have been suffering from other undiagnosed conditions. It is important to note that due to sample volume limitations, not all microscopy-negative samples were re-tested with LAMP; therefore, the actual percentage of false positives could be even higher.
The introduction of RDTs marked a major milestone in malaria diagnosis, providing rapid and accessible results that have significantly contributed to disease control efforts. However, while they have been invaluable in increasing access to diagnostic methods, the high rate of false positives remains a critical concern. RDTs have made malaria diagnosis quicker and easier, but they do not always provide accurate results. When clinicians rely solely on these results for diagnosis and treatment, patients may receive inappropriate care, leading to the mismanagement of other serious conditions. In 2017, Uganda adopted national guidelines based on WHO recommendations, aiming to test over 75% of suspected malaria cases with a parasitological test before initiating treatment (Kigozi et al., 2021). A recent study showed significant progress, with the percentage of health facilities testing over 75% of suspected malaria patients increasing from 59% in 2014 to 86% in 2017 (Kigozi et al., 2021). While microscopy is still considered the gold standard for malaria diagnosis, it is often impractical in many health facilities due to the need for technical expertise, specialized equipment and reliable power supply. Therefore, in many areas, particularly in Sub-Saharan Africa, RDTs are the only available option for malaria diagnostics (Boyce and O’Meara, 2017).
While RDTs have made diagnostic advancements, maintaining high specificity and sensitivity is essential as more facilities depend on them for diagnosing or ruling out malaria in febrile patients. Accurate testing is crucial, as almost all patients who test positive for malaria receive anti-malarial treatment, regardless of the actual cause of their fever (Kabaghe et al., 2016). In sub-Saharan Africa, only one-third of febrile children with a positive malaria test actually have malaria, while the remaining two-thirds most likely have other causes of fever (Dalrymple et al., 2017). This highlights the need for precise diagnosis to ensure appropriate treatment. WHO recommends RDTs with a false-positive rate below 10% for procurement (World Health Organization, 2018a). WHO evaluations conducted between 2008 and 2018 assessed RDTs using microscopy as the standard reference method, analyzing geographically diverse clinical samples of P. falciparum and P. vivax at both high and low parasite densities, as well as a parasite-negative panel. These evaluations included the RightSign Malaria Ag HRPII/Pan Plasmodium Aldolase test (Hangzhou Biotest Biotech Co., Ltd., China), which is the same RDT tested in our study and commonly used at Iganga General Hospital, and found it had a false-positive rate of 14% (World Health Organization, 2018b). In our study, this RDT demonstrated an even higher false-positive rate of 20%. Importantly, the issue of high false-positive rates is not limited to this specific brand; similar results have been observed in Tanzania (Ishengoma et al., 2011), Guinea Bissau (Nag et al., 2019) and Kenya (Robinson et al., 2019), where RDTs from other manufacturers also showed inaccuracies. These studies confirmed malaria positivity using PCR, sometimes from dried blood samples collected from used RDTs or filter papers stored for years. Prolonged storage can compromise sample quality, leading to inaccuracies. In contrast, we analyzed plasma samples stored for no longer than 1.5 years at -80°C, minimizing concerns about DNA degradation and improving result reliability. Testing plasma instead of whole blood in LAMP could theoretically miss cases with very low parasite levels. However, previous studies have demonstrated that analyzing DNA in plasma is a sensitive method for detecting parasites (Gal et al., 2001; Imwong et al., 2015), and our LAMP method includes six targets, which can improve sensitivity. If an individual is truly ill with malaria, their plasma is expected to test positive. A negative plasma result suggests a very low parasite load, indicating that the fever is likely caused by another condition.
Several factors contribute to false-positive results in RDTs. One of the main causes is the persistence of circulating antigens after successful anti-malarial treatment. Studies show that HRP2-based RDTs can yield positive results for up to 61 days post-treatment, due to the slow degradation of HRP2 after parasite clearance (Dalrymple et al., 2018). This persistence is linked to initial parasite density; higher parasite levels are associated with longer persistence (Huong et al., 2002; Dalrymple et al., 2018). This phenomenon is often more pronounced in children, who tend to have higher initial parasitemia and less-developed immunity, leading to longer antigen persistence than in adults (Doolan et al., 2009). In our study, we compared three groups: those who tested positive by microscopy (true positives), LAMP (true positives) and those who tested negative by both methods (false positives). We specifically examined factors such as age, sex, time since the last treated malaria episode and usage of ITNs. There were no significant differences between the groups, suggesting that recent treatment did not appear to be a primary factor contributing to the false positives results observed in this study.
Among the 14% of individuals who were microscopy negative but tested positive by LAMP, a larger proportion were children under 15 years. LAMP is more sensitive than microscopy in detecting low levels of Plasmodium spp (Picot et al., 2020)., suggesting that some positive cases may represent asymptomatic parasitemia rather than symptomatic malaria. This is particularly relevant in Uganda, where children aged 5-15 are significant contributors to malaria transmission and are more likely to carry asymptomatic infections compared to younger children and adults (Andolina et al., 2021). The persistence of positive LAMP results also adds complexity to their interpretation. LAMP can detect Plasmodium DNA for several weeks after successful treatment (Ponce et al., 2017), likely reflecting residual circulating DNA rather than active infection. Similar results have been observed with PCR, where positivity may persist for up to 6-7 weeks post-treatment (Haanshuus and Mørch, 2020). These factors suggest that some study participants who tested positive for malaria via LAMP may not have been symptomatic, but instead had low-level asymptomatic parasitemia or residual DNA from recent infections.
In our cohort, 20% of individuals tested negative by both microscopy and LAMP, suggesting that other factors may contribute to the false-positive RDT results. Several infections have been linked to RDT cross-reactivity, including acute typhoid fever (Meatherall et al., 2014), schistosomiasis (Leshem et al., 2011), human African trypanosomiasis (Gillet et al., 2013), Mycoplasma pneumoniae (Haberichter Kristle et al., 2017), dengue, and leishmaniasis (World Health Organization, 2018b), some of which are prevalent in our study setting. Autoantibodies, particularly RF, are another well-established cause of false-positive RDT results. RF, commonly associated with autoimmune diseases like rheumatoid arthritis, can produce false-positive rates of 2.2 – 13% (Lee et al., 2014; Gatton et al., 2018), by interacting with specific antibodies on RDT strips (Lee et al., 2014). However, RF is not exclusive to autoimmune diseases and is also found in parasitic, viral, and bacterial infections, such as hepatitis, HIV, leprosy and tuberculosis (Iqbal et al., 2000). Notably, RF is present in approximately 5-21% of the healthy population, depending on genetic, environmental, and age-related factors (Ingegnoli et al., 2013; Kumar et al., 2022; Satiş et al., 2024). In tropical regions, RF seroprevalence may be higher due to exposure to chronic infections (Iqbal et al., 2000). Although we did not test for RF specifically, it is possible that some false-positive results in our cohort were caused by circulating RF.
Our study has a few limitations that should be acknowledged. While LAMP offers greater sensitivity than microscopy for detecting low-level parasitemia, its ability to detect residual Plasmodium DNA weeks after treatment could have led to misclassification of individuals with non-malarial febrile illnesses as true positives. This may have resulted in an overestimation of malaria prevalence in our cohort. Furthermore, the use of plasma rather than whole blood for LAMP testing may have reduced sensitivity for detecting very low parasite densities. However, since this is a study of clinical cases of malaria, we would expect both plasma and blood to be positive if there were enough parasites to cause symptoms. Due to sample volume limitations, not all microscopy-negative samples were re-tested with LAMP, which means the actual percentage of false-positive RDT cases could be even higher than our findings suggest. While we performed LAMP testing on most microscopy-negative samples, testing the entire cohort would have provided a more comprehensive analysis and potentially identified additional false-positive RDT-cases. Furthermore, the single-cohort design limits the generalizability of our findings to regions with different transmission dynamics, co-infection rates, or diagnostic practices. Finally, data on prior malaria episodes and treatments were self-reported, introducing the possibility of recall bias that might impact the reliability of these variables.
Given the high rate of false-positive RDTs, improving their specificity, is a key step forward to align with WHO’s ongoing efforts to improve diagnostic accuracy. Using more sensitive tests, like LAMP, alongside RDTs could provide a more reliable way to diagnose malaria in these regions. However, for LAMP to be more practical in field settings, further work is needed to make the technology more accessible and affordable. This could include reducing the need for expensive equipment, improving the portability of LAMP kits, and simplifying the testing process so that it can be used by health workers with less training. Furthermore, our findings address the need for further research into the causes of febrile illnesses in malaria-endemic regions, to improve the diagnosis and treatment of non-malarial fevers. In northwest and central Uganda, arboviral, rickettsial and typhoid infections have been identified as common causes (Kigozi et al., 2023), while other studies from different regions have reported brucellosis and leptospirosis (Migisha et al., 2018). This underscores the importance of investigating the etiology of febrile illnesses in different regions, as pathogens can vary based on demographics and environmental factors. In the future, cost-effective multiplex point-of-care diagnostic tools could aid in differentiating causes of fever. Additionally, it is crucial that hospitals use RDTs that are recommended by the WHO. These RDTs meet high standards for sensitivity and specificity, ensuring more accurate malaria diagnoses.
In conclusion, our study shows that 20% of individuals treated for malaria based on positive RDT results were negative by microscopy and LAMP, indicating false positives. This highlights the important issue of misdiagnosis, which can lead to overuse of antimalarials and delays in diagnosing other serious conditions, impacting treatment effectiveness and public health. These findings emphasize the need for more accurate diagnostic tools and contribute to global efforts aimed at addressing challenges in malaria control, such as false positives and factors like co-infections and residual parasitemia. While our study did not compare multiple RDT brands, the concern about false positives persists regardless of brand. We recommend using only validated, WHO-recommended RDTs in settings without access to microscopy. In the future, as methods like LAMP become more feasible, integrating them with RDTs could improve diagnostic accuracy and strengthen malaria control efforts.
The raw data supporting the conclusions of this article will be made available by the authors, without undue reservation.
The studies involving humans were approved by the School of Biomedical Sciences Research and Ethics Committee of Makerere University, the Uganda National Council of Science and Technology (approval SBS-REC-765 and HS1267ES) and by Regionala Etikprövningsnämnden, Lund (Dnr 2015/7) and Etikprövningsmyndigheten in Stockholm, Sweden (204/478-32 and 2022-03160-01). The studies were conducted in accordance with the local legislation and institutional requirements. Written informed consent for participation in this study was provided by the participants’ legal guardians/next of kin.
SM: Conceptualization, Data curation, Formal analysis, Funding acquisition, Investigation, Methodology, Project administration, Software, Validation, Visualization, Writing – original draft, Writing – review & editing. AL: Conceptualization, Data curation, Investigation, Methodology, Project administration, Resources, Software, Supervision, Validation, Visualization, Writing – original draft, Writing – review & editing. A-CI: Investigation, Methodology, Writing – original draft, Writing – review & editing. SK: Investigation, Methodology, Writing – original draft, Writing – review & editing. HN: Conceptualization, Methodology, Resources, Supervision, Validation, Visualization, Writing – original draft, Writing – review & editing. KP: Conceptualization, Data curation, Formal analysis, Funding acquisition, Investigation, Methodology, Project administration, Resources, Software, Supervision, Validation, Visualization, Writing – original draft, Writing – review & editing.
The author(s) declare financial support was received for the research, authorship, and/or publication of this article. Funding was provided from ALF (Region Skåne/Lund University) and Alfred Österlunds Stiftelse. The funders had no role in study design, data collection and analysis, decision to publish, or preparation of the manuscript. Open access funding provided by Lund University.
We are grateful to all study participants for kindly donating blood samples. We also thank the clinical and administrative staff of Iganga General Hospital for assistance in the study.
The authors declare that the research was conducted in the absence of any commercial or financial relationships that could be construed as a potential conflict of interest.
The author(s) declare that no Generative AI was used in the creation of this manuscript.
All claims expressed in this article are solely those of the authors and do not necessarily represent those of their affiliated organizations, or those of the publisher, the editors and the reviewers. Any product that may be evaluated in this article, or claim that may be made by its manufacturer, is not guaranteed or endorsed by the publisher.
Aidoo M., Incardona S. (2021). Ten years of universal testing: how the rapid diagnostic test became a game changer for malaria case management and improved disease reporting. Am. J. Trop. Med. Hyg. 106, 29–32. doi: 10.4269/ajtmh.21-0643
Andolina C., Rek J. C., Briggs J., Okoth J., Musiime A., Ramjith J., et al. (2021). Sources of persistent malaria transmission in a setting with effective malaria control in eastern Uganda: a longitudinal, observational cohort study. Lancet Infect. Dis. 21, 1568–1578. doi: 10.1016/S1473-3099(21)00072-4
Boyce M. R., O’Meara W. P. (2017). Use of malaria RDTs in various health contexts across sub-Saharan Africa: a systematic review. BMC Public Health 17, 470. doi: 10.1186/s12889-017-4398-1
Cook J., Aydin-Schmidt B., González I. J., Bell D., Edlund E., Nassor M. H., et al. (2015). Loop-mediated isothermal amplification (LAMP) for point-of-care detection of asymptomatic low-density malaria parasite carriers in Zanzibar. Malar J. 14, 43. doi: 10.1186/s12936-015-0573-y
Dalrymple U., Arambepola R., Gething P. W., Cameron E. (2018). How long do rapid diagnostic tests remain positive after anti-malarial treatment? Malar J. 17, 228. doi: 10.1186/s12936-018-2371-9
Dalrymple U., Cameron E., Bhatt S., Weiss D. J., Gupta S., Gething P. W. (2017). Quantifying the contribution of Plasmodium falciparum malaria to febrile illness amongst African children. eLife 6, e29198. doi: 10.7554/eLife.29198
Doolan D. L., Dobaño C., Baird J. K. (2009). Acquired immunity to malaria. Clin. Microbiol. Rev. 22, 13–36. doi: 10.1128/CMR.00025-08
Gal S., Fidler C., Turner S., Lo Y. M., Roberts D. J., Wainscoat J. S. (2001). Detection of Plasmodium falciparum DNA in plasma. Ann. N Y Acad. Sci. 945, 234–238. doi: 10.1111/j.1749-6632.2001.tb03891.x
Gatton M. L., Ciketic S., Barnwell J. W., Cheng Q., Chiodini P. L., Incardona S., et al. (2018). An assessment of false positive rates for malaria rapid diagnostic tests caused by non-Plasmodium infectious agents and immunological factors. PloS One 13, e0197395. doi: 10.1371/journal.pone.0197395
Gillet P., Mumba Ngoyi D., Lukuka A., Kande V., Atua B., van Griensven J., et al. (2013). False positivity of non-targeted infections in malaria rapid diagnostic tests: the case of human african trypanosomiasis. PloS Negl. Trop. Dis. 7, e2180. doi: 10.1371/journal.pntd.0002180
Grandesso F., Nabasumba C., Nyehangane D., Page A. L., Bastard M., De Smet M., et al. (2016). Performance and time to become negative after treatment of three malaria rapid diagnostic tests in low and high malaria transmission settings. Malar J. 15, 496. doi: 10.1186/s12936-016-1529-6
Haanshuus C. G., Mørch K. (2020). Detection of remaining Plasmodium DNA and gametocytes during follow up after curative malaria treatment among returned travellers in Norway. Malar J. 19, 296. doi: 10.1186/s12936-020-03367-6
Haberichter Kristle L., Johnson Paul C., Chittick Paul J., Millward P., Robinson-Dunn B., Boyanton Bobby L. (2017). The brief case: false-positive rapid malaria antigen test result in a returned traveler. J. Clin. Microbiol. 55, 2294–2297. doi: 10.1128/JCM.02347-16
Huong N. M., Davis T. M., Hewitt S., Huong N. V., Uyen T. T., Nhan D. H., et al. (2002). Comparison of three antigen detection methods for diagnosis and therapeutic monitoring of malaria: a field study from southern Vietnam. Trop. Med. Int. Health 7, 304–308. doi: 10.1046/j.1365-3156.2002.00869.x
Imwong M., Woodrow C. J., Hendriksen I. C., Veenemans J., Verhoef H., Faiz M. A., et al. (2015). Plasma concentration of parasite DNA as a measure of disease severity in falciparum malaria. J. Infect. Dis. 211, 1128–1133. doi: 10.1093/infdis/jiu590
Ingegnoli F., Castelli R., Gualtierotti R. (2013). Rheumatoid factors: clinical applications. Dis. Markers 35, 727–734. doi: 10.1155/2013/726598
Iqbal J., Sher A., Rab A. (2000). Plasmodium falciparum histidine-rich protein 2-based immunocapture diagnostic assay for malaria: cross-reactivity with rheumatoid factors. J. Clin. Microbiol. 38, 1184–1186. doi: 10.1128/JCM.38.3.1184-1186.2000
Ishengoma D. S., Lwitiho S., Madebe R. A., Nyagonde N., Persson O., Vestergaard L. S., et al. (2011). Using rapid diagnostic tests as source of malaria parasite DNA for molecular analyses in the era of declining malaria prevalence. Malar J. 10, 6. doi: 10.1186/1475-2875-10-6
Ivarsson A.-C., Fransén E., Broumou I., Färnert A., Persson K. E. M., Söbirk S. K. (2023). Head-to-head comparison of two loop-mediated isothermal amplification (LAMP) kits for diagnosis of malaria in a non-endemic setting. Malaria J. 22, 377. doi: 10.1186/s12936-023-04809-7
Kabaghe A. N., Visser B. J., Spijker R., Phiri K. S., Grobusch M. P., van Vugt M. (2016). Health workers’ compliance to rapid diagnostic tests (RDTs) to guide malaria treatment: a systematic review and meta-analysis. Malar J. 15, 163. doi: 10.1186/s12936-016-1218-5
Kaddumukasa M., Buwembo W., Sekikubo M., Naiwumbwe H., Namusoke F., Kiwuwa S., et al. (2014). Malariometric indices from Iganga, Uganda: baseline characterization in preparation of GMZ2 vaccine trial. BMC Res. Notes 7, 793. doi: 10.1186/1756-0500-7-793
Kifude C. M., Rajasekariah H. G., Sullivan D. J. Jr., Stewart V. A., Angov E., Martin S. K., et al. (2008). Enzyme-linked immunosorbent assay for detection of Plasmodium falciparum histidine-rich protein 2 in blood, plasma, and serum. Clin. Vaccine Immunol. 15, 1012–1018. doi: 10.1128/CVI.00385-07
Kigozi R. N., Bwanika J., Goodwin E., Thomas P., Bukoma P., Nabyonga P., et al. (2021). Determinants of malaria testing at health facilities: the case of Uganda. Malar J. 20, 456. doi: 10.1186/s12936-021-03992-9
Kigozi B. K., Kharod G. A., BuKenya H., Shadomy S. V., Haberling D. L., Stoddard R. A., et al. (2023). Investigating the etiology of acute febrile illness: a prospective clinic-based study in Uganda. BMC Infect. Dis. 23, 411. doi: 10.1186/s12879-023-08335-4
Kumar A., Vasdev V., Patnaik S. K., Bhatt S., Singh R., Bhayana A., et al. (2022). The diagnostic utility of rheumatoid factor and anticitrullinated protein antibody for rheumatoid arthritis in the Indian population. Med. J. Armed Forces India 78, S69–S74. doi: 10.1016/j.mjafi.2020.04.007
Lee J. H., Jang J. W., Cho C. H., Kim J. Y., Han E. T., Yun S. G., et al. (2014). False-positive results for rapid diagnostic tests for malaria in patients with rheumatoid factor. J. Clin. Microbiol. 52, 3784–3787. doi: 10.1128/JCM.01797-14
Leshem E., Keller N., Guthman D., Grossman T., Solomon M., Marva E., et al. (2011). False-positive Plasmodium falciparum histidine-rich protein 2 immunocapture assay results for acute schistosomiasis caused by Schistosoma mekongi. J. Clin. Microbiol. 49, 2331–2332. doi: 10.1128/JCM.00307-11
Meatherall B., Preston K., Pillai D. R. (2014). False positive malaria rapid diagnostic test in returning traveler with typhoid fever. BMC Infect. Dis. 14, 377. doi: 10.1186/1471-2334-14-377
Migisha R., Dan N., Boum Y., Page A. L., Zúñiga-Ripa A., Conde-Álvarez R., et al. (2018). Prevalence and risk factors of brucellosis among febrile patients attending a community hospital in south western Uganda. Sci. Rep. 8, 15465. doi: 10.1038/s41598-018-33915-9
Mortazavi S. E., Lugaajju A., Danielsson L., Wu B., Norrgren H., Persson K. E. M. (2024). Dynamics of osteopontin levels and correlation with parasitemia in acute malaria in Uganda and Sweden. BMC Infect. Dis. 24, 1164. doi: 10.1186/s12879-024-10076-x
Nag S., Ursing J., Rodrigues A., Crespo M., Krogsgaard C., Lund O., et al. (2019). Proof of concept: used malaria rapid diagnostic tests applied for parallel sequencing for surveillance of molecular markers of anti-malarial resistance in Bissau, Guinea-Bissau during 2014-2017. Malar J. 18, 252. doi: 10.1186/s12936-019-2894-8
Picot S., Cucherat M., Bienvenu A. L. (2020). Systematic review and meta-analysis of diagnostic accuracy of loop-mediated isothermal amplification (LAMP) methods compared with microscopy, polymerase chain reaction and rapid diagnostic tests for malaria diagnosis. Int. J. Infect. Dis. 98, 408–419. doi: 10.1016/j.ijid.2020.07.009
Ponce C., Kaczorowski F., Perpoint T., Miailhes P., Sigal A., Javouhey E., et al. (2017). Diagnostic accuracy of loop-mediated isothermal amplification (LAMP) for screening patients with imported malaria in a non-endemic setting. Parasite 24, 53. doi: 10.1051/parasite/2017054
Puri M., Kaur Brar H., Madan E., Srinivasan R., Rawat K., Gorthi S. S., et al. (2022). Rapid diagnosis of Plasmodium falciparum malaria using a point-of-care loop-mediated isothermal amplification device. Front. Cell Infect. Microbiol. 12, 961832. doi: 10.3389/fcimb.2022.961832
Robinson A., Busula A. O., Muwanguzi J. K., Powers S. J., Masiga D. K., Bousema T., et al. (2019). Molecular quantification of Plasmodium parasite density from the blood retained in used RDTs. Sci. Rep. 9, 5107. doi: 10.1038/s41598-019-41438-0
Satiş H., Erden A., Bilgin E., Ayan G., Armağan B., Tecer D., et al. (2024). Nationwide study on the prevalence of rheumatoid factor and anticitrullinated peptide positivity and their contribution to rheumatoid arthritis diagnosis. Turk J. Med. Sci. 54, 949–955. doi: 10.55730/1300-0144.5872
Unterborn R., Henao-Cordero J., Kousari A., Ramanan P., Franco-Paredes C., Madinger N. (2022). False-positive rapid diagnostic test for malaria in new world cutaneous leishmaniasis: a tale of two travelers. Ther. Adv. Infect. Dis. 9, 20499361221097791. doi: 10.1177/20499361221097791
World Health Organization. (2024). World malaria report 2024: addressing inequity in the global malaria response (Geneva: World Health Organization).
World Health Organization. (2023). WHO guidelines for malaria, 16 October 2023 (Geneva: World Health Organization).
World Health Organization. (2018a). Recommended selection criteria for procurement of malaria rapid diagnostic tests (Geneva: World Health Organization). Available at: https://iris.who.int/handle/10665/259870 (Accessed October 10, 2024).
Keywords: malaria, Plasmodium, false positive, rapid diagnostic test, microscopy, LAMP, RDT
Citation: Mortazavi SE, Lugaajju A, Ivarsson A-C, Karlsson Söbirk S, Norrgren H and Persson KEM (2025) High rate of false positive malaria rapid diagnostic tests in a district hospital in Uganda. Front. Malar. 3:1545825. doi: 10.3389/fmala.2025.1545825
Received: 15 December 2024; Accepted: 17 January 2025;
Published: 31 January 2025.
Edited by:
Alena Pance, University of Hertfordshire, United KingdomReviewed by:
Emily Ciccone, University of North Carolina at Chapel Hill, United StatesCopyright © 2025 Mortazavi, Lugaajju, Ivarsson, Karlsson Söbirk, Norrgren and Persson. This is an open-access article distributed under the terms of the Creative Commons Attribution License (CC BY). The use, distribution or reproduction in other forums is permitted, provided the original author(s) and the copyright owner(s) are credited and that the original publication in this journal is cited, in accordance with accepted academic practice. No use, distribution or reproduction is permitted which does not comply with these terms.
*Correspondence: Susanne E. Mortazavi, c3VzYW5uZS5tb3J0YXphdmlAbWVkLmx1LnNl
Disclaimer: All claims expressed in this article are solely those of the authors and do not necessarily represent those of their affiliated organizations, or those of the publisher, the editors and the reviewers. Any product that may be evaluated in this article or claim that may be made by its manufacturer is not guaranteed or endorsed by the publisher.
Research integrity at Frontiers
Learn more about the work of our research integrity team to safeguard the quality of each article we publish.