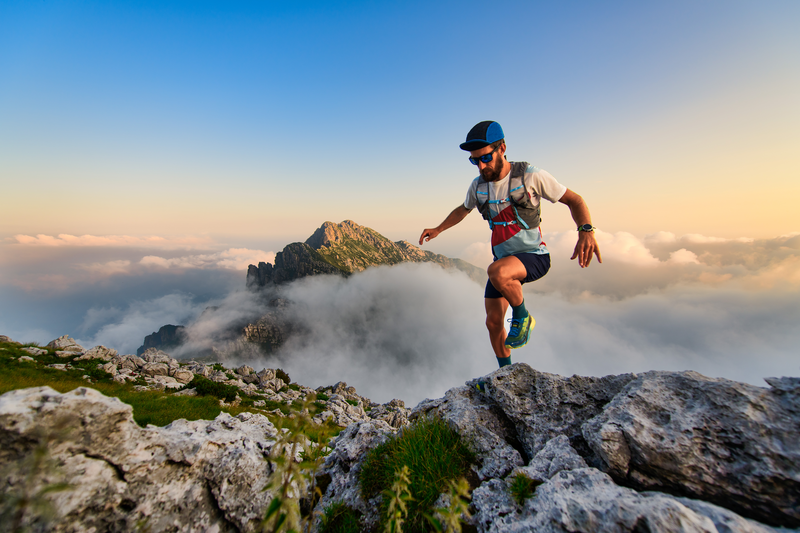
94% of researchers rate our articles as excellent or good
Learn more about the work of our research integrity team to safeguard the quality of each article we publish.
Find out more
SYSTEMATIC REVIEW article
Front. Malar. , 12 December 2024
Sec. Pathogenesis
Volume 2 - 2024 | https://doi.org/10.3389/fmala.2024.1511568
Background: India accounts for the bulk of Plasmodium vivax burden in South East Asia. Primaquine (PQ) is the only currently available drug for treating relapses in P. vivax malaria.
Methods: Here, we provide an overview of the epidemiology and clinical characteristics of P. vivax recurrent infections in India and discuss current knowledge gaps and priority research areas for further investigations, with emphasis on relapses and their radical cure with PQ.
Results and discussion: A total of 27 studies involving ~27,000 P. vivax infected patients were finally included. Recurrent infections with P. vivax malaria are common, especially in young males. The burden of P. vivax relapse greatly varies across Indian regions, with a proportion range of 1.47 to 6% based on the included studies which all used low (very low) PQ dose. There is a need for more empirical data on the effectiveness and safety of weekly administration of PQ at 0.75 mg/Kg for eight weeks in G6PD–deficiency patients in India, especially in children. Further research priorities should also be focused on the epidemiology of confounding factor, such as CQ-resistance, mixed infections, or Pv genetic diversity are needed. The clinical impact of P. vivax relapses (e.g., severe malaria, mortality) is also of valuable interest in upcoming studies. More studies addressing the above-mentioned missing links should be implemented to inform malariologists, clinicians, populations, and policy makers on real situation of P. vivax relapses and the clinical impact of PQ in India. All taken together, these would have important implications for P. vivax malaria control and elimination in endemic areas.
In 2022, malaria was responsible for ~249 million cases and ~ 608,000 deaths worldwide, which were mostly reported in sub-Saharan Africa (sSA) and South east Asia (SEA) (WHO, 2023). This infectious disease is caused by protozoan parasites of the Plasmodium genus transmitted to humans via infecting bites of female Anopheles mosquitoes (Cowman et al., 2016). Five species are currently associated with human morbidity and mortality: P. falciparum (Pf), P. ovale (Po), P. vivax (Pv), P. malariae (Pm) and P. knowlesi (Pk) (Cowman et al., 2016). Other species such as P. cynomolgi and P. brasilianum are emerging in humans and are associated with asymptomatic to mild infections (Kojom Foko et al., 2023). Pf and Pv are the main malarial species in endemic regions, though in very few countries such as Malaysia, Pk contributes largely to the malaria burden (Cooper et al., 2019; WHO, 2023).
Malaria is still a cause of concern in India despite the tremendous efforts and achievements made by the country over the last two decades. India and Indonesia accounted for ~94% of Pv malaria related total mortality seen in 2022 in the SEA region (WHO, 2023). The epidemiology of malaria in India is complex, diverse, and led by Pf and Pv while Po, Pm and Pk are reported to a lesser extent (Figure 1A) (Anvikar et al., 2016; Kojom Foko and Singh, 2023). Even though Pv is the most geographically distributed species in the world, the current literature on Pv is much fewer than that dedicated to its Pf counterpart (Gething et al., 2012; Garrido-Cardenas et al., 2019). The control of Pv is tricky owing to peculiarities intrinsically linked to its biology, namely (i) high chances for transmission to Anopheles mosquitoes due to early triggering of gametocytogenesis and efficient transmission at low parasite densities, (ii) potential to elicit severe malaria and deaths, and (iii) ability to induce relapsing infections via reactivation of dormant stages called hypnozoites (Sattabongkot et al., 2004; Bousema and Drakeley, 2011; Douglas et al., 2013; Matlani et al., 2020; Kojom Foko et al., 2021; Kojom Foko et al., 2022).
Figure 1. (A) Relative contribution of Plasmodium species to malaria infections in different states and union territories of India, 2022, and (B) Evolution of utilization of primaquine in national drug policy for radical cure of laboratory confirmed Pv malaria, India. CQ, Chloroquine; PQ, Primaquine; P. vivax, Plasmodium vivax. In (A), *Non-falciparum species are mainly due to P. vivax. The data were retrieved from official website of the National Vector Borne Disease Control Programme (https://nvbdcp.gov.in). The map was retrieved from official website of Ministry of External Affairs of the Government of India (https://mea.gov.in/india-at-glance.htm). In (B), Primaquine is contraindicated to pregnant women. The figure was generated using national data (Anvikar et al., 2014; NIMR and NVBDCP, 2014).
Reports on the contribution of Pv relapses to malaria transmission, morbidity, and mortality are increasingly published (White et al., 2014; Liu et al., 2021; Phyo et al., 2022). Relapsing Pv malaria is associated with a high proportion of recurrent infections and transmittable gametocytaemia (Douglas et al., 2013). The co-administration of schizonticidal and hypnozoiticidal drugs is adopted for the radical cure of Pv malaria and thus prevents relapses (Chu and White, 2021). Primaquine (PQ) is the only hypnozoiticidal drug currently recommended by the World Health Organization (WHO) for the radical cure of Pv infections (WHO, 2023). In the late 1950s, PQ was included in national Indian guidelines for Pv malaria treatment (Figure 1B) (Anvikar et al., 2014; NIMR and NVBDCP, 2014). Unfortunately, utilisation of PQ is greatly jeopardised by the high risk of severe haemolytic anaemia in patients with a deficiency in glucose-6-phosphate dehydrogenase (G6PD) (Peters and Van Noorden, 2009; Chu and White, 2021).
Little is known about recurrent Pv infections (i.e., recrudescence, reinfection, and relapses) in India, and filling this gap could be helpful for efficiently controlling Pv malaria in the country. In the present scoping review, we systematically summarised and analysed Indian literature on Pv malaria recurrences, especially relapses in the context of radical cure with primaquine. Practical implications, knowledge gaps, solutions, and future prospects are also discussed.
This scoping review followed the Preferred Reporting Items for Systematic Reviews and Meta-Analyses extension for Scoping Reviews (PRISMA-ScR) checklist as well as general related guidance (Supplementary File 1) (Pham et al., 2014; Tricco et al., 2018). The project was registered with the Open Science Framework (https://osf.io/shku4/) (Foster and Deardoff, 2017).
The scoping review approach is considered particularly relevant and useful for analysing, identifying, and synthesising a range of complex health public concerns, such as the management of relapsing malaria (Munn et al., 2018; Peters et al., 2020). The research question examines the literature that exists on the burden and treatment of recurrent Pv malaria in India, with an emphasis on relapses. Second, identifying causes/determinants of relapses in the Indian context was also addressed. Third, we also investigated the efficacy and safety of primaquine for the radical cure of Pv malaria infections. The knowledge gaps, practical implications, solutions, and future prospects of Pv malaria treatment are also discussed.
The searches were conducted from September to December 2022 and March to April 2023 without any restriction period in six electronic databases, including PubMed, Wiley Online Library, clinicaltrials.gov, the International Clinical Trials Registry Platform, ScienceDirect, ResearchGate and Google scholar. Official websites of local journals and scientific associations were also scrutinised. Search terms were used in combination with Boolean terms (i.e., AND, OR) (Table 1). The full texts of open access publications were retrieved from each electronic database. Principal investigators and editors-in-chief of local journals were contacted by email or phone call to request a full-length paper and/or more details on the relevant studies. The papers were purchased in case of absence of feedback or refusal. Full texts were scrutinised for eligibility in the PRISMA-ScR. Also, the reference list of relevant papers was examined to find more potentially relevant papers.
Only papers published from 1913 to 2022 and written in English were included. Quantitative and interventional studies were considered of interest if they addressed any aspect of recurrent Pv malaria including type (i.e., recrudescence, reinfection, and relapse), prevalence, clinical presentation, biology, determinants, outcomes, diagnostics, prevention, and treatment. The cut-off of 1913 was chosen as the oldest Indian medical journal, i.e., the Indian Journal of Medical Research, started its first releases circa 1913.
We excluded studies i) with a qualitative-based design (narrative review, conference, letter, correspondence, comment), ii) protocol studies, iii) conducted outside India, iv) having evaluated antimalarial drug efficacy against Pv malaria without addressing Pv recurrences, v) having evaluated antimalarial drug efficacy against recurrent Pf malaria, vi) having evaluated presumptive treatments of Pf and/or Pv infections, and vii) having used animal models. Titles and abstracts of potentially relevant papers were evaluated independently by the authors. Some publications were excluded at this step of the screening strategy, while duplicates were removed.
First, one author (L.P.K.F.) initially extracted the relevant information from eligible studies using an Excel standardised form. The data charting process mapped study findings according to the attributes which could be categorised into five groups namely i) study details (first author’s name, year of publication, paper title, collection area, malaria endemicity, state/territory union and year of data collection); ii) design details (setting, study design, study population, parasitological diagnosis method, definition used to identify Pv relapses); iii) drug details (number of treatment arms, number of patient in each treatment arms, number of anti-relapse regimens, comparative control regimen, nature of blood schizonticidal and liver anti-relapse drugs, dose of blood schizonticidal drug, total dose of liver anti-relapse drug, daily dose and duration of anti-relapse treatment, G6PD profile, method used to determine blood level of anti-relapse drug); iv) follow-up details (type, frequency and duration), and v) outcomes (number of recurrent parasitaemia events in each treatment arms, number of recurrent events in each treatment arms, month of recurrent/relapse event in each treatment arms, sociodemographic and clinical characteristics of patients with recurrent event, number of haemolytic anaemia event). The recurrent event included relapse, recrudescence, and reinfection. The design of this extraction form was piloted and refined by all authors.
Data were explored and summarised in congruence with scoping review objectives, along with narrative analysis. The selected evidence based on the source, study characteristics, and major findings were mapped and presented in tabular and/or graphical forms. For instance, the geographical distribution of studies was mapped by Indian states/union territories. Also, pie charts were used to present the distribution of included studies by setting (research institute, health facility, and community), study design (case report, randomised clinical trial – RCT, and prospective study), and study population (children, adults, and general population). The term “not specified” was used if details on one of the above-mentioned items were not mentioned in the included studies. PQ total dose was classified as very low (≤ 2.5 mg/Kg), low (> 2.5 – < 5 mg/Kg) and high (≥ 5 mg/Kg) as described earlier (John et al., 2012). The multiplication of blood stages of early relapses is suppressed by slowly eliminated drugs, and thus, relapses become microscopically patent 3 weeks after QN or artesunate, 3 – 6 weeks after artemether + lumefantrine, and 5 – 7 weeks after CQ treatment (White, 2011; Chu and White, 2021). In this context, we categorised Pv malaria recurrences and/or relapses as frequent (~3 – 7 weeks) and long (~8 – 9 months).
We used the approach proposed by Commons and colleagues to appraise the proportion of Pv recurrences caused by relapse, with slight modifications (Commons et al., 2020). In this approach, the primary outcome was the incidence rate of Pv recurrences over 365 days following a given treatment. The incidence rate ratio (IRR) was calculated as the ratio of the incidence rate of Pv recurrences in the treatment arm with PQ to the ratio of incidence rate of Pv recurrences in the treatment arm without PQ. The minimum proportion of recurrences due to relapse was calculated by subtracting the IRR from 1 (Commons et al., 2020). This approach assumes that PQ has an anti-relapse preventive efficacy of 100% and does not improve the killing effect of asexual blood stage parasites (Commons et al., 2020).
In this section, only RCTs that included PQ in one of the regimen arms, followed up patients actively regardless of duration of follow-up, supervised PQ administration daily, and had an extractable incidence rate were included to assess Pv relapse burden. We used these stringent criteria to reduce any ambiguity about the distinction between reinfection, recrudescence, and relapses and thus give a consistent estimate of the proportion of Pv recurrence attributable to relapse in the Indian context. Also, Pv relapse estimates as calculated in each individual study were extracted and stratified by definition used for relapse, time of follow-up, and PQ dosing.
Two reviewers (L.P.KF. and V.S.) independently assessed the bias risk of the RCTs included to determine Pv relapse burden. The methodological quality of RCTs was evaluated using Joanna Briggs Institute - JBI critical appraisal tools designed for RCTs (https://jbi.global/critical-appraisal-tools). This tool consists of 13 questions on four types of methodology-related bias viz. i) selection, ii) performance, iii) attrition, and iv) reporting. A choice between four answers (“Yes”, “No”, “Unclear” and “Not applicable”) was proposed for each question as per the JBI tool. Thus, the risk of bias was categorised as “low” if the answer was “Yes”, “high” if the answer was “No” and “unclear” if the answer was “Unclear or Not applicable” (Arya et al., 2021). Disagreements between reviewers were resolved through discussion and consensus.
The data used in this review was retrieved from publicly accessible databases. Thus, ethics committee approval was not requested.
A total of 2,106 potentially relevant records were retrieved from electronic databases. Of these records, 1504 were retained after removing duplicates. We excluded 1478 records after analysing titles and abstracts based on the above mentioned exclusion criteria: i) studies conducted outside India (n = 154), ii) studies on recurrent Pf malaria (n = 96), iii) anti-relapse drugs were not tested (n = 7), iv) reviews (n = 5), v) Pv relapses were not addressed (n = 5), vi) studies on presumptive malaria treatment (n = 4), vii) protocols (n = 3), viii) correspondence (n = 1), ix) animal-based studies (n = 1), full-text not found (n = 1), and xi) irrelevant studies (n = 1201) (Figure 2). Twenty-six publications were evaluated for eligibility. We identified three more publications through the bibliographic references listed in the 26 publications, thereby giving a total of 29 potentially eligible publications. Of these, two records were excluded because of evidence of duplicate (i.e., same findings published in different journals). Thus, 27 studies involving ~27,000 Pv-infected patients treated with PQ-based treatment regimens were finally included to analyse Pv recurrences (Figure 2) (Singh et al., 1953; Basavaraj, 1960; Sharma et al., 1973; Roy et al., 1977; Roy et al., 1979; Appavoo et al., 1984; Sinha et al., 1989; Sharma et al., 1990; Prasad et al., 1991; Srivastava et al., 1996; Gogtay et al., 1998; Gogtay et al., 1999; Adak et al., 2001; Dua and Sharma, 2001; Yadav and Ghosh, 2002; Rajgor et al., 2003; Imwong et al., 2007; Saifi et al., 2010; Kim et al., 2012; Rajgor et al., 2014; Pareek et al., 2015; Savargaonkar et al., 2015; Kumar et al., 2016; Savargaonkar et al., 2017; Saravu et al., 2018; Kishore et al., 2020; Gandrala et al., 2022). In order to determine the proportion of Pv recurrences due to relapse, 24 studies were excluded due to three reasons: 21 studies were not designed as RCTs, 2 studies evaluated PQ-based treatment regimens, and recurrence data were not extractable in one study (Figure 2).
Figure 2. PRISMA flow diagram depicting the selection process of studies. Pf, Plasmodium falciparum; Pv, Plasmodium vivax; PQ, Primaquine.
Details of the included studies are presented in Figure 3 and Supplementary File 2. Studies included were published over changes in PQ drug policies in India, with 23.1% of them published from 1960 – 1990, during which Pv infections were treated using CQ given as a single dose followed by PQ at a dose of 0.25 mg/Kg for five days (Figure 3A). More than one third (38.5%) of the studies were published from 2010 – present. Most studies were conducted in Maharashtra (n = 6), Delhi (n = 6), Uttar Pradesh (n = 4), and Karnataka (n = 4) (Figure 3B). The bulk of studies were conducted in a health facility setting (55.5%), designed as prospective studies (66.4%), and included patients of all ages (57.7%) (Figures 3C–E).
Figure 3. Characteristics of studies on P. vivax recurrences in India. In (A), proportion of studies with respect to changes in PQ related policies in India. In (B), international codes for Indian areas were used (DL, Delhi; GJ, Gujarat; KA, Karnataka; MH, Maharashtra; MP, Madhya Pradesh; OR, Orissa; TN, Tamil Nadu; UP, Uttar Pradesh) Number of studies conducted in areas is presented. The distribution of studies as per setting (C), study design (D), and study population (E) is also depicted.
CQ was the main blood-stage antimalarial drug associated with PQ regimens, while some studies used artemisinin-based combination therapies (ACT), amodiaquine (AQ), quinine (QN), and pyrimethamine (PYR). The majority of studies (n = 22, 81.5%) evaluated CQ + PQ to prevent Pv recurrences. Other studies associated PQ with other 8-aminoquinolines such as pentaquine, pamaquine and AQ while one study evaluated the ACT + PQ association to prevent Pv recurrences (Table 2). Two studies evaluated the anti-recurrence potential of different PQ monotherapies (i.e., standard and sustained release) (Singh et al., 1953; Pareek et al., 2015). Another study evaluated the anti-relapse effect of bulaquine, a PQ analogue, in patients from Delhi (Adak et al., 2001).
Table 2. Characteristics of therapeutic regimens evaluated to prevent recurrent P. vivax parasitaemia.
PQ posology greatly varied between studies, with the majority of studies testing very low PQ dose regimens (total dose < 2.5 mg/Kg). PQ was administered daily mostly for 5 days (n = 16, 59.3%), followed by daily administration for 14 days in 10 studies (37%), and 7 days in 3 studies (11.5%) (Table 2). Patients were either followed up actively or passively for durations ranging from 180 to 1460 months. Regarding active follow-up, patients were followed up either fortnightly, weekly or every 4/6/8 weeks.
Light microscopy (LM) was invariably used for Pv detection in patients, while some studies coupled it with polymerase chain reaction (PCR), rapid diagnostic test (RDT), and quantitative buffy coat (QBC). While if G6PD status of patients was not specified or evaluated in the bulk of the study, we noted that recent studies either excluded G6PD-d patients or evaluated G6PD status (Table 2). In addition, two studies determined the blood level of PQ using liquid chromatography – mass spectrometry (LC-MS) and reverse high-pressure liquid chromatography (HPLC) while the technique was not specified in one study (Rajgor et al., 2003; Saravu et al., 2018; Gandrala et al., 2022).
The approach used to analyse recurrent infections was either not specified or inexistent in 34.6% and 26.9% of the included studies, respectively. For the remaining studies, six types of approaches were proposed, and based on patterns of recurrences following the primary infection, genotyping, parasitological evidence, clinical symptoms, and mathematical modelling (Table 3).
Table 3. Approaches used to discriminate the different types of Plasmodium vivax recurrences (relapse, reinfection, recrudescence).
A genotyping-based approach relied on molecular amplification and/or sequencing of genetic markers (genes, microsatellites) in order to distinguish between relapse (heterologous infections) and recrudescence (homologous infections). The assumption behind this approach is that recurrent infections are classified as relapses if they are genetically distinct from primary infections. One study from Karnataka used molecular markers such as microsatellites (MS7 and MS10) to analyse Pv recurrence in adults treated with CQ + PQ (Saravu et al., 2018). In an RCT conducted in Maharashtra, genetic markers were associated with a linear regression-based mathematical model to determine the attributable fraction of Pv recurrence caused by relapse in patients treated with CQ alone or in association with PQ for 5 and 14 days (Table 3) (Kim et al., 2012).
Before the advent of molecular methods, earlier studies identified relapses using an approach based on patterns of recurrences, clinical symptoms, and parasitological evidence. In Uttar Pradesh, one study defined relapses as follows: “Patient became positive again, a few weeks after clearance of parasitaemia and in spite of a full course of CQ and PQ, associated with the analysis of periodicity to distinguish relapses from fresh infections” (Sinha et al., 1989).
Few studies analysed demographic characteristics of Pv recurrences and only data was extracted from five community-based studies and one case report conducted in Karnataka, Uttar Pradesh, Gujarat, Delhi, and Orissa states (Basavaraj, 1960; Sinha et al., 1989; Prasad et al., 1991; Srivastava et al., 1996; Yadav and Ghosh, 2002; Savargaonkar et al., 2017), even though several attempts were made to contact the corresponding and supervising authors of unavailable studies. Most Pv recurrences were found in males and those aged > 14 years old. In Karnataka, the proportion of Pv recurrences was higher in males (71.8%) and patients aged > 14 years (53.8%) compared to their female and younger counterparts, respectively (Basavaraj, 1960). Likewise, two studies from Uttar Pradesh reported a higher proportion of males (≥ 66%) and aged > 14 years (≥ 60%) (Sinha et al., 1989; Prasad et al., 1991). In contrast, Yadav and Ghosh found no statistically significant difference in Pv recurrence proportion between males and females in Orissa (Yadav and Ghosh, 2002).
The number of Pv recurrences with regard to treatment arm and latency type is presented in Figure 4. Recurrences were observed for all arm treatments analysed (CQ, PQ, AQ + PQ, QN + Pentaquine, CQ + PYR, and CQ + PQ). Most Pv recurrences had intermediate and long latency. In patients treated with PQ monotherapy, Pv recurrences had frequent latency, while Pv recurrences had intermediate and long latency in those treated with CQ + PYR (Figure 4).
Figure 4. Latency patterns of P. vivax recurrences in treatment arms. AQ, Amodiaquine; CQ, Chloroquine; PQ, Primaquine; PYR, Pyrimethamine; QN, Quinine. Points depicted as star represent the number of relapses from studies with passive follow up of P. vivax-infected patients treated with CQ (purple star) or CQ + PQ (brown star). Points depicted as round, diamond and triangle represent the number of relapses from studies with active follow up of P. vivax-infected patients. Latency period of P. vivax recurrences was defined as frequent and long as proposed earlier (White, 2011; Chu and White, 2021). **These recurrences were subjectively termed “intermediate” by authors of the included studies. All treatment arms with PQ were very low dose (i.e. PQ total dose ≤ 2.5 mg/Kg).
As stated previously, three studies met inclusion criteria to determine the fraction of Pv recurrences caused by relapse (Rajgor et al., 2003; Kim et al., 2012; Rajgor et al., 2014). Although similarities between the three RCTs were noted for a place (Maharashtra), design (open label RCT), control arm (CQ) and follow-up (active), there were some differences found for population, number of treatment arms, PQ total dosing evaluated, duration of follow-up, parasitological method, and determination of PQ blood level (Supplementary File 3). For instance, patients were followed-up for 180 days in two studies and 450 days in the remaining study. Only one study determined the blood level of PQ in its design. Overall, the risk of bias was low for all evaluation components, with the exception of concealment and blinding to drug treatment (Supplementary File 4).
In the included studies, estimates of the proportion of Pv malaria recurrences that relapsed were based on different approaches (genotyping, mathematical model, temporal pattern of recurrence). Using the Commons and colleagues-based adapted approach, the proportion estimates was ~0 – 60% across the three RCTs included. These estimates were higher than those found based on approaches defined by the authors of each individual study. Using two PCR-based approaches, Rajgor et al. found Pv relapses at proportions of 6% and 2 – 5% in CQ + PQ-treated adults from Maharashtra (Table 4) (Rajgor et al., 2003; Rajgor et al., 2014).
Table 4. Comparative analysis of estimates of Pv relapses based on approaches used by authors of individual studies and adapted from the Commons and colleagues’ study.
In one study from Delhi, PQ was administered at 0.75 mg/Kg/week for eight weeks in a 23-year-old male with G6PD-d who had presented three episodes of relapses 4, 5 and 6 months after the primary Pv infection. No haemolytic anaemia event was reported in the patient (Savargaonkar et al., 2017).
It is evident that recurrent malaria parasitaemia is an important impediment to malaria control strategies and that accurately determining the burden and patterns of relapsing Plasmodium infections is a key determinant to evaluating the clinical impact of Pv malaria and informing policy makers on the utility of PQ-based national strategies in India. However, there are several missing links and solutions presented below and in Figure 5, that should be addressed in the future.
Figure 5. Current challenges to appraise and control Pv recurrences caused by relapses, and some proposed solutions. CQ, Chloroquine; G6PD, Glucose-6-Phosphate dehydrogenase; G6PD-d, Glucose-6-Phosphate dehydrogenase deficiency; PQ, Primaquine; Pf, Plasmodium falciparum; Pv, Plasmodium vivax; RCT, Randomised controlled trial.
Various approaches, such as genotyping, patterns of recurrence and statistical models, were used in individual studies, and this increases uncertainty over the true epidemiology of relapse burden and patterns in India. Distinguishing relapses and reinfections using genotyping is still problematic given that relapse–associated genotypes can be identical, closely similar, or different from those of the primary infection (Imwong et al., 2007; White, 2011; Imwong et al., 2012; Popovici et al., 2018; Chu and White, 2021). In Thailand, Imwong et al. showed that the first relapses of life in babies are genetically similar to the primary infections (Imwong et al., 2012). Using denoting protocols, Popovici and colleagues showed that most Pv relapses were polyclonal and closely related genotypes that caused acute infection in Cambodian individuals (Popovici et al., 2018). More recently, Xu and colleagues showed the possible utility of the circumsporozoite protein gene for tracing the origin of Pv relapse among Chinese patients (Xu et al., 2023). In community settings, the utilisation of genotyping methods is challenging and thus requires the development of point-of-care testing for the detection of relapse risk as recently proposed by the WHO (2024).
On analysis of the periodicity of Pv recurrence events, it was observed that these showed frequent and long latency phenotypes. It is plausible that some Pv recurrences observed in Indian studies were relapses. Both frequent and long latency types coexist in India (White, 2011). PQ has a narrow therapeutic window and a short half-life (~4 – 7 hours) which requires daily administration for up to two weeks (Pareek et al., 2015). Thus, this may result in poor adherence and explain some recurrent infections. In some included studies, recurrent infections appeared within less than 28 days of follow-up, thereby suspecting a possible CQ-resistance of the Pv parasite and/or suboptimal blood levels of the PQ active metabolite.
Recurrences of Pv malaria were mostly seen in young males, and this is consistent with previous studies in Brazil (Daher et al., 2019), but contradictory to other investigations (Cuong et al., 2006; Vieira et al., 2020). In addition to the male sex, Douglas and colleagues found that young age, Pf/Pv mixed infections at enrolment, lower haematocrit, higher Pf asexual parasite density, Pf gametocytaemia at enrolment, and drug partner in the ACTs were risk factors for Pv recurrence after treatment of acute Pf malaria in individuals from the Thai-Myanmar border (Hossain et al., 2020). The role of age on the risk of Pv recurrence is a bit less elusive than that of gender. Higher rates of Pv recurrences observed in young individuals are likely due to immunity levels that are known to increase with age with the lowest levels during infancy and the highest during adulthood, thereby reducing the number of Pv recurrences in increasing age groups (White, 2011). Regarding gender, its relationship with Pv recurrences is still elusive, and thus, further studies are necessary to understand how this variable could modulate Pv recurrence.
The proportion of Pv recurrences that relapse in India varied from 0 to 60% using the adapted Commons and colleagues’ approach, but from 1.47 to 6% using the methods described in the included studies. Relapse burden was estimated earlier to be minimally at 66%, using the Commons and colleagues’ approach, across Pv malaria endemic regions (Afghanistan, Ethiopia, Indonesia, Nepal, Pakistan, Thailand, and Vietnam) (Commons et al., 2020). This is not surprising as the approach proposed by Commons and colleagues has some limitations as outlined by them previously (Commons et al., 2020). For instance, the approach assumes that the risk of recrudescence is similar between individuals treated with or without PQ. It is known that PQ has a schizonticidal activity against early recurrent infection that likely derivate from relapse and/or recrudescence (White, 2008). In areas where Pv isolates have reduced CQ susceptibility, it is probable to observe lower rates of recurrent infections in individuals treated with PQ, and in this scenario, the proportions of recurrences that relapses can be overestimated (Commons et al., 2020). Furthermore, as we adapted the Commons’ approach (for instance, we included all studies regardless of the duration of follow-up), this inevitably introduced another bias in the estimation of relapse rates, thus explaining the differences observed. Also, it is evident that between-study differences may explain this large range of estimates, even though the studies included for analysis were all conducted in Maharashtra, and this limits our estimates to this area and not at the country level. Huber and colleagues recently pointed out that differences in the conditions under which clinical trials are conducted may explain difficulties in comparative analyses of efficacy estimates between clinical trials and underestimate the effect of radical cure on Pv hypnozoites (Huber et al., 2021). More importantly, it should be important to mention that this large variation in the prevalence of relapse is likely due to the fact that PQ was given at a low dose in the included studies. In general, if proper treatment is given, relapse rates may be lower than 5%, as is evidenced by their studies using genotyping techniques. Also, some of the included studies did not follow the Indian Government guidelines for the treatment of Pv malaria which in itself is cause for concern. India adopted the WHO guidelines for dealing with Pv relapses which consists of administering PQ for 14 days. Very low doses of PQ will not eliminate the hypnozoites, which are dormant and are in the liver cells. This is why the PQ doses should be standardised and would help eliminate dormant stages so that no relapse occurs. Adequate PQ dosing is a key factor to reduce the chances of relapses. Several studies reported that the chances of relapses were decreased with increasing PQ doses (Galappaththy et al., 2013; Verma et al., 2023). Some of the current questions are about the duration of the PQ regimen (e.g., 3 days, 7 days, 14 days) and the impact of adjustment variables (e.g., age, weight, G6PD activity level, G6PD-d) of the PQ doses (Taylor et al., 2021; Moore et al., 2023; Pukrittayakamee et al., 2024; Rajasekhar et al., 2024). Besides, the included studies did not use the same definitions for Pv relapses, and this has also introduced bias in comparative analysis (Xu et al., 2023).
Besides, the drug resistance profile of infecting Pv strains is also an important confounder in the ability to distinguish the different types of recurrent infections. Drug resistance profile is particularly important in areas where high rates of drug-resistant Pv parasites, especially CQ-resistant parasites, are documented. CQ is the drug of choice for treating Pv infections in India. Even though, there are no validated molecular markers of Pv-related CQ-resistance till now, clinical efficacy studies may reveal valuable help to appreciate the burden of both CQ-resistance and Pv relapse (Popovici et al., 2019; Twohig et al., 2019; Kojom Foko et al., 2024). Unfortunately, this aspect was not addressed in the included studies that evaluate the burden of Pv relapses. Also, measuring the G6PD activity of individuals is crucial to evaluating both severe haemolysis risk and the effectiveness and efficacy of PQ against relapse. Again, these aspects were not evaluated in the included studies and should be investigated in future. A recent study by Rajasekhar and colleagues concluded the safety of PQ radical cure at doses 0.25–0.5 mg/kg in patients with G6PD activity of 30% or higher, and 0.25–1 mg/kg per day regimens in patients with G6PD activity of 70% or higher, in comparison with patients treated without PQ (Rajasekhar et al., 2024). Finally, host-related factors such as pharmacogenetics should also investigated in future. Indeed, three main genetic polymorphisms, i.e., cytochrome P450 2D6 (CYP2D6), monoamine oxidase (MAO), and cytochrome P450 NADPH: oxidoreductase, can affect the PQ metabolism and its conversion to active form, and thereby possibly impairing its efficacy or effectiveness and increasing the chances of relapses (Nain et al., 2022). Thus, it would be crucial to conduct proper clinical studies, combining correct doses of PQ, sensitive genotyping techniques, and evaluation of G6PD activity, clinical CQ resistance, and host-related genetic factors to better understand the real burden of Pv recurrences that relapse in India.
The original contributions presented in the study are included in the article/Supplementary Material. Further inquiries can be directed to the corresponding author.
LK: Conceptualization, Data curation, Formal analysis, Investigation, Methodology, Software, Writing – original draft. VS: Conceptualization, Project administration, Resources, Supervision, Validation, Visualization, Writing – review & editing.
The author(s) declare that no financial support was received for the research, authorship, and/or publication of this article.
The authors acknowledge Department of Biotechnology (DBT), New Delhi, Government of India; and The World Academy of Sciences (TWAS), Trieste, Italy to first author a Postgraduate Fellowship programme (DBT-TWAS Postgraduate Fellowship - 2017). We also thank Mr. Rashid Perwez (Assistant Librarian & Information Officer, ICMR-NIMR, India) for sharing full-texts of some relevant papers included in this scoping review. Finally, we thank ICMR-NIMR for infrastructure support towards providing a working environment.
The authors declare that the research was conducted in the absence of any commercial or financial relationships that could be construed as a potential conflict of interest.
The author(s) declare that no Generative AI was used in the creation of this manuscript.
All claims expressed in this article are solely those of the authors and do not necessarily represent those of their affiliated organizations, or those of the publisher, the editors and the reviewers. Any product that may be evaluated in this article, or claim that may be made by its manufacturer, is not guaranteed or endorsed by the publisher.
The Supplementary Material for this article can be found online at: https://www.frontiersin.org/articles/10.3389/fmala.2024.1511568/full#supplementary-material
ACT, Artemisinin-based combination therapy; AQ, Amodiaquine; CQ, Chloroquine; csp, Circumsporozoite gene; G6PD, Glucose-6-phosphate dehydrogenase; G6PD-d, Glucose-6-phosphate dehydrogenase deficiency; HPLC, High pressure liquid chromatography; IRR, Incidence rate ratio; JBI, Joanna Briggs Institute; LC-MS, Liquid chromatography coupled with mass spectrometry; LM, Light microscopy; MS, Microsatellites; msp1, Merozoite surface protein 1 gene; msp3-α, Merozoite surface protein 3 alpha gene; n.a, Not available; NVBDCP, National Vector Borne Disease Control Programme; PCR – SSCP, Polymerase chain reaction – Single strand conformational polymorphism; PCR – RFLP, Polymerase chain reaction – Restriction fragment length polymorphism; PRISMA-ScR, The Preferred Reporting Items for Systematic Reviews and Meta-Analyses extension for Scoping Reviews; PQ, Primaquine; Pf, Plasmodium falciparum; Pk, Plasmodium knowlesi; Pm, Plasmodium malariae; Po, Plasmodium ovale; Pv, Plasmodium vivax; Pvcrt-o, Plasmodium vivax chloroquine carrier transporter orthologue; PYR, Pyrimethamine; QBC, Quantitative buffy coat; QN, Quinine; RDT, Rapid diagnostic test; SEA, South East Asia; sSA, sub-Saharan Africa; WHO, World Health Organization.
Adak T., Valecha N., Sharma V. P. (2001). Plasmodium vivax polymorphism in a clinical drug trial. Clin. Diagn. Lab. Immunol. 8, 891–894. doi: 10.1128/CDLI.8.5.891-894.2001
Anvikar A. R., Arora U., Sonal G. S., Mishra N., Shahi B., Savargaonkar D., et al. (2014). Antimalarial drug policy in India: Past, present & future. Indian J. Med. Res. 139, 205–215.
Anvikar A. R., Shah N., Dhariwal A. C., Sonal G. S., Pradhan M. M., Ghosh S. K., et al. (2016). Epidemiology of Plasmodium vivax malaria in India. Am. J. Trop. Med. Hyg. 95, 108–120. doi: 10.4269/ajtmh.16-0163
Appavoo N., Roy R., Kapal V. (1984). Results of 3-day radical treatment of Plasmodium vivax in North Arcot and South Arcot districts of Tamil Nadu. Indian J. Malariol. 21, 21–24.
Arya A., Kojom Foko L. P., Chaudhry S., Sharma A., Singh V. (2021). Artemisinin-based combination therapy (ACT) and drug resistance molecular markers: A systematic review of clinical studies from two malaria endemic regions – India and sub-Saharan Africa. Int. J. Parasitol. Drugs Drug Resist. 15, 43–56. doi: 10.1016/j.ijpddr.2020.11.006
Basavaraj H. (1960). Observations on the treatment of 678 malaria cases with primaquine in an area free from malaria transmission in Mysore state, India. Indian J. Malariol. 14, 269–281.
Bousema T., Drakeley C. (2011). Epidemiology and infectivity of Plasmodium falciparum and Plasmodium vivax gametocytes in relation to malaria control and elimination. Clin. Microbiol. Rev. 24, 377–410. doi: 10.1128/CMR.00051-10
Chu C. S., White N. J. (2021). The prevention and treatment of Plasmodium vivax malaria. PloS Med. 18, e1003561. doi: 10.1371/journal.pmed.1003561
Commons R. J., Simpson J. A., Watson J., White N. J., Price R. N. (2020). Estimating the proportion of Plasmodium vivax recurrences caused by relapse: A systematic review and meta-analysis. Am. J. Trop. Med. Hyg. 103, 1094–1099. doi: 10.4269/ajtmh.20-0186
Cooper D. J., Rajahram G. S., William T., Jelip J., Mohammad R., Benedict J., et al. (2019). Plasmodium knowlesi malaria in Sabah, Malaysia, 2015–2017: Ongoing increase in incidence despite near-elimination of the human-only Plasmodium species. Clin. Infect. Dis. 70, 361–367. doi: 10.1093/cid/ciz237
Cowman A. F., Healer J., Marapana D., Marsh K. (2016). Malaria: biology and disease. Cell 167, 610–624. doi: 10.1016/j.cell.2016.07.055
Cuong B. T., Binh V. Q., Dai B., Duy D. N., Lovell C. M., Rieckmann K. H., et al. (2006). Does gender, food or grapefruit juice alter the pharmacokinetics of primaquine in healthy subjects? Br. J. Clin. Pharmacol. 61, 682–689. doi: 10.1111/j.1365-2125.2006.02601.x
Daher A., Silva J. C. A. L., Stevens A., Marchesini P., Fontes C. J., Ter Kuile F. O., et al. (2019). Evaluation of Plasmodium vivax malaria recurrence in Brazil. Malar. J. 18, 18. doi: 10.1186/s12936-019-2644-y
Douglas N. M., Simpson J. A., Phyo A. P., Siswantoro H., Hasugian A. R., Kenangalem E., et al. (2013). Gametocyte dynamics and the role of drugs in reducing the transmission potential of Plasmodium vivax. J. Infect. Dis. 208, 801–812. doi: 10.1093/infdis/jit261
Dua V. K., Sharma V. P. (2001). Plasmodium vivax relapses after 5 days of primaquine treatment, in some industrial complexes of India. Ann. Trop. Med. Parasitol. 95, 655–659. doi: 10.1080/00034983.2001.11813682
Foster E. D., Deardoff A. (2017). Open science framework (OSF). J. Med. Libr. Assoc. 107, 203–206. doi: 10.5195/jmla.2017.88
Galappaththy G. N. L., Tharyan P., Kirubakaran R. (2013). Primaquine for preventing relapse in people with Plasmodium vivax malaria treated with chloroquine. Cochrane Database Syst. Rev. 2013, CD004389. doi: 10.1002/14651858.CD004389.pub3
Gandrala D., Gupta N., Lavu A., Nallapati V. T., Guddattu V. (2022). Recurrence in Plasmodium vivax malaria: A prospective cohort study with long follow-up from a coastal region in South-West India. F1000Research 11, 1–16. doi: 10.12688/f1000research
Garrido-Cardenas J. A., Cebrián-Carmona J., González-Cerón L., Manzano-Agugliaro F., Mesa-Valle C. (2019). Analysis of global research on malaria and Plasmodium vivax. Int. J. Env. Res. Public Health 16, 1928. doi: 10.3390/ijerph16111928
Gething P. W., Elyazar I. R. F., Moyes C. L., Smith D. L., Battle K. E., Guerra C. A., et al. (2012). A long neglected world malaria map: Plasmodium vivax endemicity in 2010. PloS Negl. Trop. Dis. 6, e1814. doi: 10.1371/journal.pntd.0001814
Gogtay N., Desai S., Kamtekar K., Kadam V., Dalvi S., Kshirsagar N. (1999). Efficacies of 5-and-14-day primaquine regimens in the prevention of relapses in Plasmodium vivax infections. Ann. Trop. Med. Parasitol. 93, 809–812. doi: 10.1080/00034989957790
Gogtay N., Garg M., Kadam V., Kamtekar K., Kshirsagar N. A. (1998). A 5 days primaquine regimen as antirelapse therapy for Plasmodium vivax. Trans. R Soc. Trop. Med. Hyg. 92, 341. doi: 10.1016/S0035-9203(98)91034-3
Hossain M. S., Commons R. J., Douglas N. M., Thriemer K., Alemayehu B. H., Amaratunga C., et al. (2020). The risk of Plasmodium vivax parasitaemia after P. falciparum malaria: An individual patient data meta-analysis from the WorldWide Antimalarial Resistance Network. PloS Med. 17, e1003393. doi: 10.1371/journal.pmed.1003393
Huber J. H., Koepfli C., España G., Nekkab N., White M. T., Alex Perkins T. (2021). How radical is radical cure? Site-specific biases in clinical trials underestimate the effect of radical cure on Plasmodium vivax hypnozoites. Malar. J. 20, 479. doi: 10.1186/s12936-021-04017-1
Imwong M., Boel M. E., Pagornrat W., Pimanpanarak M., McGready R., Day N. P.J., et al. (2012). The first Plasmodium vivax relapses of life are usually genetically homologous. J. Infect. Dis. 205, 680–683. doi: 10.1093/infdis/jir806
Imwong M., Snounou G., Pukrittayakamee S., Tanomsing N., Kim J. R., Nandy A., et al. (2007). Relapses of Plasmodium vivax infection usually result from activation of heterologous hypnozoites. J. Infect. Dis. 195, 927–933. doi: 10.1086/512241
John G. K., Douglas N. M., Von Seidlein L., Nosten F., Baird J. K., White N. J., et al. (2012). Primaquine radical cure of Plasmodium vivax: A critical review of the literature. Malar. J. 11, 280. doi: 10.1186/1475-2875-11-280
Kim J. R., Nandy A., Maji A. K., Addy M., Dondorp A. M., Day N. P.J., et al. (2012). Genotyping of Plasmodium vivax reveals both short and long latency relapse patterns in Kolkata. PloS One 7, e39645. doi: 10.1371/journal.pone.0039645
Kishore R., Dhakad S., Arif N., Dar L., Mirdha B. R., Aggarwal R., et al. (2020). COVID-19: Possible cause of induction of relapse of Plasmodium vivax infection. Indian J. Pediatr. 87, 751–752. doi: 10.1007/s12098-020-03441-6
Kojom Foko L. P., Arya A., Sharma A., Singh V. (2021). Epidemiology and clinical outcomes of severe Plasmodium vivax malaria in India. J. Infect. 82, 231–246. doi: 10.1016/j.jinf.2021.03.028
Kojom Foko L. P., Jakhan J., Tamang S., Hawadak J., Kouemo Motse F. D., Singh V. (2024). First insight into drug resistance genetic markers, glucose−6−phosphate dehydrogenase and phylogenetic patterns of misdiagnosed Plasmodium vivax malaria in Far North region, Cameroon. Curr. Microbiol. 81, 9. doi: 10.1007/s00284-023-03522-7
Kojom Foko L. P., Kumar A., Hawadak J., Singh V. (2023). Plasmodium cynomolgi in humans: current knowledge and future directions of an emerging zoonotic malaria parasite. Infection 51, 623–640. doi: 10.1007/s15010-022-01952-2
Kojom Foko L. P., Narang G., Tamang S., Hawadak J., Jakhan J., Sharma A., et al. (2022). The spectrum of clinical biomarkers in severe malaria and new avenues for exploration. Virulence 13, 634–654. doi: 10.1080/21505594.2022.2056966
Kojom Foko L. P., Singh V. (2023). Malaria in pregnancy in India: A 50-year bird’s eye. Front. Public Health 11, 1150466. doi: 10.3389/fpubh.2023.1150466
Kumar R., Guddattu V., Saravu K. (2016). Therapeutic assessment of primaquine for radical cure of Plasmodium vivax malaria at primary and tertiary care centres in Southwestern India. Korean J. Parasitol. 54, 733–742. doi: 10.3347/kjp.2016.54.6.733
Liu P., Shen L., Wang S., Qin P., Si Y., Pan M., et al. (2021). Increasing proportions of relapsing parasite species among imported malaria in China’s Guangxi Province from Western and Central Africa. Travel Med. Infect. Dis. 43, 102130. doi: 10.1016/j.tmaid.2021.102130
Matlani M., Kojom L. P., Mishra N., Dogra V., Singh V. (2020). Severe vivax malaria trends in the last two years: a study from a tertiary care centre, Delhi, India. Ann. Clin. Microbiol. Antimicrob. 19, 49. doi: 10.1186/s12941-020-00393-9
Moore B. R., Salman S., Tobe R., Benjamin J., Yadi G., Kasian B., et al. (2023). Short-course, high-dose primaquine regimens for the treatment of liver-stage vivax malaria in children. Int. J. Infect. Dis. 134, 114–122. doi: 10.1016/j.ijid.2023.05.063
Munn Z., Peters M. D. J., Stern C., Tufanaru C., McArthur A., Aromataris E. (2018). Systematic review or scoping review? Guidance for authors when choosing between a systematic or scoping review approach. BMC Med. Res. Methodol. 18, 143. doi: 10.1186/s12874-018-0611-x
Nain M., Mohan M., Sharma A. (2022). Effects of host genetic polymorphisms on the efficacy of the radical cure malaria drug primaquine. Am. J. Trop. Med. Hyg. 106, 764–767. doi: 10.4269/ajtmh.21-1115
NIMR and NVBDCP (2014). Guidelines on diagnosis and treatment of malaria in India, 2014. Third Ed (New-Delhi, India: NIMR and NVBDCP).
Pareek A., Chandurkar N., Gogtay N., Deshpande A., Kakrani A., Kaneria M., et al. (2015). Sustained release formulation of primaquine for prevention of relapse of Plasmodium vivax malaria: A randomized, double-blind, comparative, multicentric study. Malar. Res. Treat. 2015, 579864. doi: 10.1155/2015/579864
Peters M. D. J., Marnie C., Tricco A. C., Pollock D., Munn Z., Alexander L., et al. (2020). Updated methodological guidance for the conduct of scoping reviews. JBI Evid. Synth. 18, 2119–2126. doi: 10.11124/JBIES-20-00167
Peters A. L., Van Noorden C. J. F. (2009). Glucose-6-phosphate dehydrogenase deficiency and malaria: Cytochemical detection of heterozygous G6PD deficiency in women. J. Histochem. Cytochem. 57, 1003–1011. doi: 10.1369/jhc.2009.953828
Pham M. T., Rajić A., Greig J. D., Sargeant J. M., Papadopoulos A., Mcewen S. A. (2014). A scoping review of scoping reviews: Advancing the approach and enhancing the consistency. Res. Synth. Methods 5, 371–385. doi: 10.1002/jrsm.1123
Phyo A. P., Dahal P., Mayxay M., Ashley E. A. (2022). Clinical impact of vivax malaria: A collection review. PloS Med. 19, e1003890. doi: 10.1371/journal.pmed.1003890
Popovici J., Friedrich L. R., Kim S., Bin S., Run V., Lek D., et al. (2018). Genomic analyses reveal the common occurrence and complexity of Plasmodium vivax relapses in Cambodia. MBio 9, E018886–17. doi: 10.1128/mBio.01888-17
Popovici J., Pierce-Friedrich L., Kim S., Bin S., Run V., Lek D., et al. (2019). Recrudescence, reinfection, or Relapse? A more rigorous framework to assess chloroquine efficacy for Plasmodium vivax malaria. J. Infect. Dis. 219, 315–322. doi: 10.1093/infdis/jiy484
Prasad R. N., Virk K. J., Sharma V. P. (1991). Relapse/reinfection patterns of Plasmodium vivax infection: a four year study. Southeast Asian J. Trop. Med. Public Health 22, 499–503.
Pukrittayakamee S., Jittamala P., Watson J. A., Hanboonkunupakarn B., Leungsinsiri P., Poovorawan K., et al. (2024). Primaquine in glucose-6-phosphate dehydrogenase deficiency: an adaptive pharmacometric assessment of ascending dose regimens in healthy volunteers. Elife 13, RP87318. doi: 10.7554/eLife.87318.3.sa2
Rajasekhar M., Simpson J. A., Ley B., Edler P., Chu C. S., Abreha T., et al. (2024). Primaquine dose and the risk of haemolysis in patients with uncomplicated Plasmodium vivax malaria: a systematic review and individual patient data meta-analysis. Lancet Infect. Dis. 24, 184–195. doi: 10.1016/S1473-3099(23)00431-0
Rajgor D. D., Gogtay N. J., Kadam V. S., Kamtekar K. D., Dalvi S. S., Chogle A. R., et al. (2003). Efficacy of a 14-day primaquine regimen in preventing relapses in patients with Plasmodium vivax malaria in Mumbai, India. Trans. R Soc. Trop. Med. Hyg. 97, 438–440. doi: 10.1016/S0035-9203(03)90082-4
Rajgor D. D., Gogtay N. J., Kadam V. S., Kocharekar M. M., Parulekar M. S., Dalvi S. S., et al. (2014). Antirelapse efficacy of various primaquine regimens for Plasmodium vivax. Malar. Res. Treat 2014, 347018. doi: 10.1155/2014/347018
Roy R., Chakrapani K., Dhinagaran D., Sitaraman N., Ghosh R. (1977). Efficacy of 5-day radical treatment of Plasmodium vivax infection in Tamil Nadu. Indian J. Med. Res. 65, 652–656.
Roy R. G., Shanmugham C. A. K., Chakrapani K. P., Ganesan A. V. (1979). Results of 5-day course of radical treatment of Plasmodium vivax in six districts of Tamil Nadu. Indian J. Med. Res. 69, 939–943.
Saifi M. A., Wajihullah, Siddiqui M. I., Al-Khalifa M. S. (2010). Malaria: Patterns of relapse and resistance. J. King Saud Univ. Sci. 22, 31–36. doi: 10.1016/j.jksus.2009.12.005
Saravu K., Tellapragada C., Kulavalli S., Xavier W., Umakanth S., Brahmarouphu G., et al. (2018). A pilot randomized controlled trial to compare the effectiveness of two 14-day primaquine regimens for the radical cure of vivax malaria in South India. Malar. J. 17, 321. doi: 10.1186/s12936-018-2472-5
Sattabongkot J., Tsuboi T., Zollner G. E., Sirichaisinthop J., Cui L. (2004). Plasmodium vivax transmission: Chances for control? Trends Parasitol. 20, 192–198. doi: 10.1016/j.pt.2004.02.001
Savargaonkar D., Ahmed M. Z., Anvikar A. R., Valecha N. (2017). Safety of weekly primaquine in G6PD deficient patient with relapsing vivax malaria: A case report. J. Vector Borne Dis. 54, 287–290. doi: 10.4103/0972-9062.217622
Savargaonkar D., Nagpal B. N., Srivastava B., Anvikar A. R., Valecha N. (2015). The footprints of relapsing malaria in Southwest Delhi, India. J. Vector Borne Dis. 52, 287–292. doi: 10.4103/0972-9062.172807
Sharma R., Gautam A., Orlov V., Sharma V. (1990). Relapse pattern of Plasmodium vivax in Kheda district, Gujarat. Indian J. Malariol. 27, 95–99.
Sharma M., Sehgal P., Vaid B., Dubey R., Nagendra S., Paithne P., et al. (1973). Effectiveness of drug schedule being followed under the national malaria eradication programme, India, for radical cure of vivax malaria cases. J. Commun. Dis. 5, 167–174.
Singh J., Ray A., Basu P., Nair C., Misra B. (1953). Preliminary studies on 8-aminoquinolines. Indian J. Malariol. 7, 289–294.
Sinha S., Dua V., Sharma V. (1989). Efficacy of 5 day radical treatment of primaquine in Plasmodium vivax cases at the BHEL industrial complex, Hardwar (U.P.). Indian J. Malariol. 26, 83–86.
Srivastava H., Sharma S., Bhatt S., Sharma V. (1996). Studies on Plasmodium vivax relapse pattern in Kheda district, Gujarat. Indian J. Malariol. 33, 173–179.
Taylor W. R., Hoglund R. M., Peerawaranun P., Nguyen T. N., Hien T. T., Tarantola A., et al. (2021). Development of weight and age-based dosing of daily primaquine for radical cure of vivax malaria. Malar. J. 20, 366. doi: 10.1186/s12936-021-03886-w
Tricco A. C., Lillie E., Zarin W., O’Brien K. K., Colquhoun H., Levac D., et al. (2018). PRISMA extension for scoping reviews (PRISMA-ScR): Checklist and explanation. Ann. Intern. Med. 169, 467–473. doi: 10.7326/M18-0850
Twohig K. A., Pfeffer D. A., Baird J. K., Price R. N., Zimmerman P. A., Hay S. I., et al. (2019). Growing evidence of Plasmodium vivax across malaria-endemic Africa. PloS Negl. Trop. Dis. 13, e007140. doi: 10.1371/journal.pntd.0007140
Verma R., Commons R. J., Gupta A., Rahi M., Bharti P. K., Thriemer K., et al. (2023). Safety and efficacy of primaquine in patients with Plasmodium vivax malaria from South Asia: a systematic review and individual patient data meta- analysis. BMJ Glob. Health 8, e012675. doi: 10.1136/bmjgh-2023-012675
Vieira M. V. D. F., Mello A. G. C. N., de Sena L. W. P., de Vieira J. L.F. (2020). Absence of gender influence on the pharmacokinetics of chloroquine combined with primaquine in malaria vivax patients. Rev. Inst. Med. Trop. Sao Paulo 62, e83. doi: 10.1590/s1678-9946202062083
White N. J. (2008). The role of anti-malarial drugs in eliminating malaria. Malar. J. 7, S8. doi: 10.1186/1475-2875-7-S1-S8
White N. J. (2011). Determinants of relapse periodicity in Plasmodium vivax malaria. Malar. J. 10, 297. doi: 10.1186/1475-2875-10-297
White M. T., Karl S., Battle K. E., Hay S. I., Mueller I., Ghani A. C. (2014). Modelling the contribution of the hypnozoite reservoir to Plasmodium vivax transmission. Elife 3, e04692. doi: 10.7554/eLife.04692.021
WHO (2024). Diagnostic tests for detecting the risk of Plasmodium vivax relapse (Geneva: World Health Organization). Available at: https://iris.who.int/bitstream/handle/10665/376457/9789240089846-eng.pdf?sequence=1. (Accessed on April 15, 2024).
Xu Y., Dong Y., Deng Y., Huang H., Chen M., Liu Y., et al. (2023). Molecular identification of vivax malaria relapse patients in the Yunnan Province based on homology analysis of the Plasmodium vivax circumsporozoite protein gene. Parasitol. Res. 122, 85–96. doi: 10.1007/s00436-022-07700-7
Keywords: epidemiology, India, Plasmodium vivax, primaquine, recurrent infections
Citation: Kojom Foko LP and Singh V (2024) Burden and clinical characteristics of recurrent Plasmodium vivax infections, and impact of primaquine for radical cure: a systematic scoping review in India. Front. Malar. 2:1511568. doi: 10.3389/fmala.2024.1511568
Received: 15 October 2024; Accepted: 26 November 2024;
Published: 12 December 2024.
Edited by:
Yang Cheng, Jiangnan University, ChinaReviewed by:
Cyprian Onyeji, University of Nigeria, NigeriaCopyright © 2024 Kojom Foko and Singh. This is an open-access article distributed under the terms of the Creative Commons Attribution License (CC BY). The use, distribution or reproduction in other forums is permitted, provided the original author(s) and the copyright owner(s) are credited and that the original publication in this journal is cited, in accordance with accepted academic practice. No use, distribution or reproduction is permitted which does not comply with these terms.
*Correspondence: Vineeta Singh, dmluZWV0YXNfMjAwMEB5YWhvby5jb20=
Disclaimer: All claims expressed in this article are solely those of the authors and do not necessarily represent those of their affiliated organizations, or those of the publisher, the editors and the reviewers. Any product that may be evaluated in this article or claim that may be made by its manufacturer is not guaranteed or endorsed by the publisher.
Research integrity at Frontiers
Learn more about the work of our research integrity team to safeguard the quality of each article we publish.