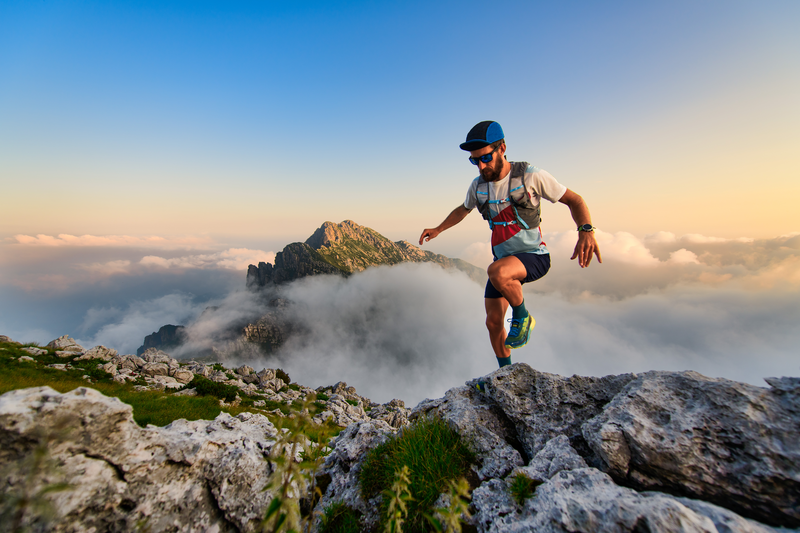
95% of researchers rate our articles as excellent or good
Learn more about the work of our research integrity team to safeguard the quality of each article we publish.
Find out more
BRIEF RESEARCH REPORT article
Front. Malar. , 24 December 2024
Sec. Vectors
Volume 2 - 2024 | https://doi.org/10.3389/fmala.2024.1507392
This article is part of the Research Topic Addressing Contemporary Threats to Global Malaria Control: New Tools and Strategies View all 8 articles
Introduction: The resistance of malaria vectors to pyrethroids has compromised the efficacy of pyrethroid insecticide-treated nets (ITNs). In response, ITNs with pyrethroids and piperonyl butoxide (PBO) synergists were developed to overcome metabolic pyrethroid resistance mechanisms. One such net is Vector Guard®. To demonstrate its potential efficacy for public health use, a comparative efficacy study was conducted to evaluate Vector Guard® relative to Olyset® Plus (a pyrethroid–PBO ITN proven to reduce malaria transmission more effectively than pyrethroid ITNs) and Royal® Sentry 2.0 (a pyrethroid-only ITN included to demonstrate the added benefit of PBO) in experimental huts in Tanzania.
Methods: An experimental hut trial using two blocks of a 7 × 7 Latin square design was conducted over seven rounds (49 experimental nights). Treatments were rotated at the end of each round, and volunteers were rotated nightly within one block. Both unwashed and 20× washed nets of each type were evaluated, alongside an untreated net used as a negative control. The primary endpoint was the proportion of Anopheles arabiensis that died within 24 h, and the secondary endpoint was the proportion of blood feeding. Data were analyzed using binomial logistic regression with fixed effects using a 7% non-inferiority margin.
Results: The pooled results showed that Vector Guard® was non-inferior and was superior to Olyset® Plus, with higher mortality for Vector Guard® [28% vs. 18%; odds ratio (OR) = 1.93, 95%CI = 1.81–2.06]. Both pyrethroid–PBO nets were superior to Royal Sentry® 2.0 (p < 0.001) in terms of mosquito mortality. For the secondary blood feeding endpoint, Vector Guard® was also found to be non-inferior to Olyset® Plus (0.6% vs. 0.2%; OR = 2.37, 95%CI = 1.77–3.17). Vector Guard® showed similar efficacy to Royal Sentry® 2.0 in reducing the proportion of mosquito blood feeding (0.6% vs. 0.6%; OR = 0.85, 95%CI = 0.68–1.07, p = 0.161).
Conclusion: Vector Guard® demonstrated superior mosquito mortality compared with both Olyset® Plus and Royal Sentry® 2.0, indicating that Vector Guard® is another promising pyrethroid–PBO ITN for the control of resistant malaria vectors. The addition of Vector Guard® to the class of pyrethroid–PBO ITNs will enable malaria control programs to select cost-effective ITNs, improving access to effective protection from malaria transmitted by resistant vectors.
Insecticide-treated nets (ITNs) with additional active ingredients (AIs), including piperonyl butoxide (PBO) synergists, are recommended for the control of malaria in areas where pyrethroid-resistant mosquitoes are predominant (WHO, 2023a). These pyrethroid–PBO nets are more effective than standard pyrethroid nets in the control of malaria in areas where mosquitoes are resistant to pyrethroids (Gleave et al., 2021). PBO acts as a synergist and increases the bioavailability of pyrethroids through the inhibition of mixed-function oxidases (Casida, 1970), the metabolic enzymes responsible for pyrethroid detoxification (Menze et al., 2022).
In 2017, the first pyrethroid–PBO net, Olyset® Plus, received a World Health Organization (WHO) policy recommendation (WHO, 2017) based on its demonstrated impact on malaria reduction in cluster randomized trials conducted in Tanzania (Protopopoff et al., 2018a) and Uganda (Staedke et al., 2020). Therefore, pyrethroid–PBO nets are recommended for the control of resistant mosquitoes, with Olyset® Plus nets recognized as the first-in-class (FIC) nets.
There is a need for more PBO nets on the market to increase price competition, which would help lower the unit cost of ITNs. This, in turn, would allow for procuring more nets, improving ITN coverage, which will ultimately result in more effective malaria control (WHO, 2023b). Other manufacturers of ITNs have produced pyrethroid–PBO nets that differ from Olyset® Plus in terms of design and specifications, including the denier, type of pyrethroids, dosage of PBO and pyrethroids, and the placement of PBO on the roof or all over the ITN. Therefore, it is crucial to understand whether products grouped within the PBO ITN class perform similarly to the FIC product that has demonstrated public health benefits.
Therefore, the WHO requires that new candidate products be compared to the FIC product in experimental hut trials (EHTs) in order to assess whether the new product is not unacceptably worse (“non-inferior”) than the FIC based on the entomological outcome measures as a correlate of protection (WHO, 2021). This comparison is performed to provide reassurance of the likely public health value of a candidate net in the same intervention class as the FIC product (WHOPES, 2013; WHO, 2023c; WHO, 2024). EHTs are a robust method for providing evidence of the efficacy of a new PBO product compared to a product with proven public health value (Sherrard-Smith et al., 2022). They use mosquito mortality as an entomological surrogate endpoint, which reliably predicts protection from malaria; in the same way, the immune response is used as a surrogate endpoint in vaccine development (Gilbert et al., 2008), allowing a rapid and low-cost evaluation of new vector control tools in multiple ecologies. This is critical to ensure that well-performing products that induce high mosquito mortality are not delayed from entering the market.
Comparative efficacy EHTs demonstrate whether a new vector control product is not unacceptably worse than the FIC based on a predefined margin of non-inferiority (WHO, 2024). Assessments of the non-inferiority of ITNs are determined based on a 7% difference in mosquito mortality. This margin of non-inferiority was clinically justified by WHO (2024). Based on this margin, a new ITN is recommended if it is not worse than the FIC on the primary outcome of mosquito mortality. The superiority (based on the significance level) of the new pyrethroid–PBO ITN over the standard pyrethroid net on the primary outcome is also necessary to demonstrate that the ITN has been tested against mosquitoes that have their sensitivity to pyrethroids partially or fully restored by PBO. Comparative efficacy EHTs are powered for precision and are reported and interpreted in the same standardized method as clinical trials (Piaggio et al., 2012; Rehal et al., 2016).
In this context, the study presents a comparative efficacy assessment of a candidate pyrethroid–PBO ITN, i.e., Vector Guard®, to the FIC pyrethroid–PBO ITN, i.e., Olyset® Plus, and its superiority as compared with a pyrethroid-only ITN (Royal Sentry® 2.0), with an untreated net arm used to monitor the study quality. This assessment was conducted against wild pyrethroid-resistant malaria vectors in Tanzania responsive to PBO (Matowo et al., 2017).
The comparative efficacy study used 14 experimental huts in two simultaneous blocks, each following a 7 × 7 and another 7 × 7 Latin square design for seven rounds over 49 experimental nights, giving 98 observations per arm. Each net brand was allocated to a pair of two huts each night, with the unwashed net in one hut and the 20 times washed net of the same brand as its pair. After an initial random allocation, the nets were rotated sequentially at the end of each round (7 days interval) to minimize allocation errors. Sleepers were rotated nightly among the huts throughout the experiment. Data were collected for seven consecutive nights, after which the huts were aired for one night before the next ITN was introduced. In accordance with the WHO guidelines for ITN testing (WHO, 2024), a quality check (QC) of the performance of ITNs was performed before and after the EHT using bioassays.
The experimental huts used in this study were new Ifakara huts (Swai et al., 2023) (Figure 1), which are closer in size to other experimental hut types (West African and East African huts) (Massue et al., 2016). The suitability of the location and ecology of the study area has been thoroughly documented in a previous work (Swai et al., 2023). The experimental huts are located between the village of Lupiro and irrigated rice fields (GPS-8.38412, 36.67289), with an annual rainfall range of 1,200–1,800mm and temperature between 20°C and 34°C. The predominant malaria vector species is Anopheles arabiensis (Pinda et al., 2020), which is found all year round and is responsive to PBO (Matowo et al., 2017).
Figure 1. Experimental huts at Lupiro village in Ulanga District, southeastern Tanzania. (A) Schematic diagram of the Ifakara huts showing a view from above. (B) Side view of the Ifakara huts at the experimental site. (C) Technician collecting mosquitoes from the exit trap. (D) Landscape overview of the Ifakara huts at Lupiro village.
The QC and EHTs were performed in the facilities of the Vector Control Product Testing Unit (VCPTU) of the Ifakara Health Institute, accredited for Good Laboratory Practice (GLP) by the South African National Accreditation System (SANAS).
The study compared Vector Guard® ITN to the FIC Olyset® Plus ITN for a non-inferiority assessment and to Royal Sentry® 2.0 ITN and Safi (untreated net) for superiority assessment (Table 1). All nets were white and double sized and had their labels removed before the study. The nets were coded with a four-digit code for the blinding of those collecting and identifying mosquitoes to the identity of the nets. The Vector Guard® ITNs were from three production batches, while the controls (i.e., Olyset® Plus, Royal Sentry®, and Safi net) were from one production batch.
There were four ITNs per product prepared for QC and 16 ITNs (14 for the EHT and 2 spares) per product prepared for testing in the experimental huts. Seven arms were tested: 1) an unwashed Vector Guard®; 2) Vector Guard® washed 20 times (20×); 3) an unwashed Olyset® Plus; 4) 20× washed Olyset® Plus; 5) an unwashed Royal Sentry® 2.0; 6) 20× washed Royal Sentry® 2.0; and 7) an untreated Safi net (negative control).
The nets were prepared before and after the hut trial for QC to confirm the bioefficacy at critical phases of the experiment: 1) upon receipt of the ITNs before washing (four nets per product); 2) after washing (four nets per product); and 3) after the hut trial (two nets per arm). Net samples (25 cm × 25 cm) were taken from positions as suggested in the WHO Pesticide Evaluation Scheme (WHOPES) LLIN testing guideline (2013) (WHOPES, 2013). For the Vector Guard®, a “mosaic net,” four samples were taken from the side panels and three from the roof, while five samples were removed from each of the positive controls. For each net used in the QC, two adjacent samples were cut from each position—one for bioassay and the other for chemical analysis—which was conducted by an independent laboratory: Walloon Agricultural Research Centre, CRA-W, Gembloux, Belgium. The chemical analysis report was sent directly to the sponsor and the results were not included in this manuscript.
Seven nets for testing in the EHT and four nets for QC were washed in aluminum bowls containing 10 L of filtered well water with 20 g of Jamaa palm oil soap flakes using manual agitation (20 rotations per minute). The nets were dried horizontally in the shade, wrapped in aluminum foil, and stored in a plastic bag in between washes in temperature-controlled storage at 24.3°C–33.9°C temperature and 54.1%–91.9% relative humidity, where they were kept until testing.
The washing intervals were experimentally determined before the start of the study: 2 days for Vector Guard® and Olyset® Plus and 1 day for Royal Sentry® 2.0 ITNs.
For each product, the nets for testing in the experimental huts (seven unwashed and seven 20× washed) were deliberately holed by cutting a total of six holes (4 cm × 4 cm) in each net: two holes on each of the long sides of the net and one hole on each of the short sides 50 cm from the top of the net. Washing and deliberate perforation of the nets were carried out to simulate field-aged nets, as per the WHO guideline (WHOPES, 2013), in order to assess their efficacy in reducing mosquito blood feeding and mortality.
The pyrethroid-susceptible Anopheles gambiae sensu stricto (Ifakara) and the pyrethroid-resistant An. arabiensis (Kingani) laboratory colonies were used for QC. The larvae were fed with TetraMin® fish feed (Spectrum Brands Pet, LLC, Blacksburg, VA, USA). Adults were kept in cages with access to 10% glucose solution ad libitum and were provided with blood meal between 3 and 6 days after emergence for egg production. The temperature in the insectary was maintained at 27 ± 2°C, and the relative humidity was between 40% and 100%, following the MR4 guidelines (MR4. Methods in Anopheles Research Manual, 2009), with an approximately 12:12 ambient light–dark cycle. The WHO susceptibility test was conducted on the pyrethroid class of insecticides alone and with PBO to observe their efficacy against the test systems (An. gambiae and An. arabiensis). A total of 25 mosquitoes were exposed in each of four replicates of the WHO tube test using a discriminating dose of insecticide. Knockdown was recorded after 1 h, and mosquito mortality was assessed after 24 h.
The EHT was conducted from 3 March to 27 April 2022, with a 2-week interval following the completion of the 20 washes to ensure that the nets were fully regenerated. The experimental huts used in this study were Ifakara huts (Swai et al., 2023), with a fully sealed plywood dividing wall to create two new Ifakara experimental huts with dimensions of 3.25 m × 3.5 m × 2 m (length × width × height) (Swai et al., 2023) (Figure 1). The experiment had two simultaneous seven arms: 1) an unwashed Olyset® Plus; 2) 20× washed Olyset® Plus; 3) an unwashed Vector Guard®; 4) 20× washed Vector Guard®; 5) an unwashed Royal Sentry® 2.0; 6) 20× washed Royal Sentry® 2.0; and 7) an untreated net (Safi) as a negative control. The primary outcome was the proportion of 24-h mortality. Data were collected for seven nights as one experimental round, with one night spent airing between rounds to reduce residual effects before introducing the next treatment. The nets were hung in the experimental hut before 1900 hours and were removed after the collection of the mosquitoes in the morning at 0600 hours. There were 14 sleepers rotated sequentially among huts each night using a pre-prepared roster. They entered the huts at 1900 hours and slept under the ITN on a mattress until 0600 hours. Each morning of the study period, wild mosquitoes were collected into paper cups using aspiration, which were labeled by date, treatment, hut, and location (i.e., inside the net, inside the hut, or in exit traps). The collection began inside the net, followed by the floor of the hut, the walls, and then the exit traps. The collected mosquitoes were transported to the field laboratory, sorted according to the species level, and then scored into four categories: dead-fed, dead-unfed, alive-fed, and alive-unfed. They were then held with access to a 10% sugar solution in a temperature-controlled mosquito holding room (27 ± 2°C and 40%–100% relative humidity) for 24 h post-collection to assess delayed mortality.
A short questionnaire was given to all participants who slept in the experimental huts in order to record users’ perception on any perceived side effects based on the WHO guidelines.
Cone bioassays were performed according to the WHO procedures as described by Odufuwa et al (Odufuwa et al., 2024). Each sample was examined with four replicates of cones (20 mosquitoes), and mosquitoes were exposed to each sample for 3 min. Knockdown was recorded at 60 min post-exposure, and mortality was recorded 24 h after the end of the exposure period.
The WHO tunnel test was conducted on samples that did not meet the specified thresholds (≥80% mosquito mortality or ≥95% mosquito knockdown) for the cone bioefficacy as described in the WHO 2013 ITN testing guideline (WHOPES, 2013). Rabbit was used as a host in the tunnel test, and each sample was assessed once, alongside a negative control. Blood feeding and the mortality rates were assessed and recorded.
All data were collected using paper-based data forms before double entry into Microsoft Excel by two different data entry clerks. Data were cleaned and analyzed using Stata version 17 (StataCorp LLC, College Station, TX, USA) (StataCorp, 2019).
The proportion of dead and blood-fed mosquitoes was calculated from the logistic regression with a random effect for hut–night, including the specific net type only with their respective 95% confidence intervals (CIs). Inferential statistics were also performed on the outcomes from the EHTs using a binomial logistic regression for the proportions of mortality and blood feeding. Inferential analysis was based on the revised guideline (WHO, 2023d), which used fixed effects for ITN brand, hut, sleeper, and collection day. For pooled analysis, the washing status (i.e., unwashed or 20× washed) was added as a fixed effect. The non-inferiority of a candidate net was determined when the odds ratio (OR) of the lower 95%CI of Vector Guard® compared to Olyset® Plus was not lower than the delta (estimated as the OR of a 7% difference from the recorded percent mortality or blood feeding of Olyset® Plus). The primary endpoint was 24-h mortality. The results were presented for each net type and condition (unwashed, 20× washed, and combination of unwashed and 20× washed).
The sample size for the experimental hut study was calculated using a simulation-based power analysis (WHO, 2018) in R statistical software (version 3.02) with the lme4 package (R Core Team, 2021). For this evaluation, 1,000 simulations for generalized linear mixed models (GLMMs) were conducted using 25% mosquito mortality estimates, with an overall study variation of logs of 0.61 and 20 An. arabiensis mosquitoes per night per hut, which were from a previous trial conducted in the same experiment for a Latin square design of 49 nights of data collection in 14 huts, with volunteers and huts accounted for as fixed effects. The study achieved 98% power with 95%CI of simulated power (0.97–0.99) to determine that Vector Guard® was non-inferior to Olyset® Plus (WHO, 2023c) on the primary endpoint of 24-h mosquito mortality.
Post-hoc power analysis was again performed by simulation to ensure that the study was adequately powered for non-inferiority (WHO, 2023c), with treatment, volunteer, hut, and day adjusted as fixed effects using R software (R Core Team, 2021). After the experimental hut implementation and using the estimates from this study, a median nightly mosquito of 54, a nightly variation of 1, and 100% power (99.6–100) were achieved.
The experiment was conducted in Lupiro during the study period, with a median nighttime temperature and relative humidity of 27.5°C [interquartile range (IQR) = 26.7–28.7°C] and 83.1% (IQR = 60.8%–96.8%), respectively. Mosquitoes were kept in the holding room for delayed mortality of the EHT and QC at temperature and relative humidity of 27.5°C (IQR = 26.7–28.7°C) and 79.8% (IQR = 75.9%–82.4%), respectively.
Over 49 nights of data collection, a total of 46,835 wild An. arabiensis were collected, with a median of 54 mosquitoes (IQR = 29–93) captured per hut. The median numbers of mosquitoes captured in huts with different types of nets were as follows: 40 (IQR = 23–67) for the Vector Guard® net, 55 (IQR = 30–91) for Olyset® Plus, 65 (IQR = 37–120) for Royal Sentry®2.0, and 64 (IQR = 30–104) for the untreated Safi net. There were differences in the number of mosquitoes captured in the unwashed and 20× washed nets (Figure 2; Table 2).
Figure 2. Violin plot showing the distribution of the numbers of female Anopheles arabiensis mosquitoes collected per net type and hut–night.
Table 2. Superiority evaluation of piperonyl butoxide (PBO) insecticide-treated nets (ITNs) in comparison to Royal Sentry® 2.0 (Pyrethroid only net) against wild pyrethroid-resistant Anopheles arabiensis in Tanzania.
Against wild, free-flying An. arabiensis, Vector Guard® showed higher 24-h mortality than Olyset® Plus. The mortality of wild, free-flying An. arabiensis in the untreated (Safi) net was 0.7 (Figure 3).
Figure 3. Percentage mean 24-h mortality of wild free-flying Anopheles arabiensis in experimental huts.
Vector Guard® was non-inferior and was superior to Olyset® Plus for the primary endpoint of 24-h mortality based on the analysis conducted on the pooled data for the unwashed and the 20× washed nets, with estimates in the direction of higher mortality for Vector Guard® (28% vs. 18%; OR = 1.93, 95%CI = 1.81–2.06) as its lower CI remained higher than the delta (0.56). The unwashed Vector Guard® was non-inferior and was superior to the unwashed Olyset® Plus in the direction of higher mortality for Vector Guard® (40% vs. 22%; OR = 2.33, 95%CI = 2.10–2.58). The same result was seen for the 20× washed Vector Guard® relative to the 20× washed Olyset® Plus (20% vs. 15%; OR = 1.73, 95%CI = 1.58–1.89) (Figure 4; Table 3).
Figure 4. Non-inferiority of Vector Guard® to Olyset® Plus on the mortality of female Anopheles arabiensis mosquitoes at 24 h (95%) after collection. Circle indicates unwashed, hollow circle represents washed, and triangle is a combination of unwashed and 20 times washed.
Table 3. Non-inferiority efficacy of Vector Guard® insecticide-treated nets (ITNs) in comparison to Olyset® Plus against wild free-flying pyrethroid-resistant Anopheles arabiensis.
The superiority evaluation of pyrethroid–PBO ITNs compared with the pyrethroid-only net (Royal Sentry® 2.0) is presented in Table 2. For the pooled analysis of the unwashed and 20× washed arms using the 24-h mortality endpoint, Vector Guard® was found superior to Royal Sentry® 2.0 (28% vs. 15%; OR = 2.33, 95%CI = 2.19–2.49, p < 0.001). Olyset® Plus was also superior to Royal Sentry® 2.0 (18% vs. 15%; OR = 1.21, 95%CI = 1.13–1.29, p < 0.001). It should be noted, however, that when the total number of mosquitoes that died was considered, more mosquitoes in total were killed in the Royal Sentry® 2.0 arm (n = 2,588) than in the Olyset® Plus arm (n = 2,480).
The proportions of mosquitoes that blood-fed were extremely low in all arms (Figure 5; Table 2), with 553/7,654 (6.4%) An. arabiensis blood-fed in the negative control arm and blood feeding <1% in the treated net arms.
Higher proportions of mosquito blood feeding were recorded in Vector Guard® than in Olyset® Plus. Although Vector Guard® had higher blood feeding overall (both unwashed and washed) compared with Olyset® Plus (0.6% vs. 0.2%; OR = 2.37, 95%CI = 1.77–3.17), it was found to be non-inferior to Olyset® Plus on the proportion of blood-fed as its upper CI remained lower than the delta (8.61) (Table 3). Higher proportions of mosquito blood feeding were recorded in the unwashed Vector Guard® vs. the unwashed Olyset® Plus (0.7% vs. 0.1%; OR = 4.57, 95%CI = 2.67–7.82) and in the washed 20× Vector Guard® nets vs. the 20× washed Olyset® Plus (0.5% vs. 0.4%; OR = 1.78, 95%CI = 1.26–2.53), with the upper CI of the estimated OR remaining lower than the delta (8.61), indicating non-inferiority (Table 3).
The superiority evaluation of PBO ITNs on blood feeding in comparison to the pyrethroid-only net (Royal Sentry® 2.0) is presented in Table 2. Vector Guard® was not significantly different from Royal Sentry® 2.0 in the pooled analysis of the unwashed and washed 20× nets (0.6% vs. 0.6%; OR = 0.85, 95%CI = 0.68–1.07, p = 0.161) on the blood feeding success endpoint. This was also the same for the unwashed net, with Vector Guard® similar to Royal Sentry® 2.0 (0.7% vs. 0.6%; OR = 1.44, 95%CI = 0.99–2.09, p = 0.058). On the other hand, for the 20× washed nets, Vector Guard® had fewer blood-fed mosquitoes and was superior to the Royal Sentry® 2.0 net (0.5% vs. 0.7%; OR = 0.65, 95%CI = 0.49–0.87, p = 0.004). Olyset® Plus was superior to Royal Sentry® 2.0 (0.2% vs. 0.6%; OR = 0.36, 95%CI = 0.28–0.47, p < 0.001) in the pooled analysis of the unwashed and 20× washed condition nets.
There were no adverse events reported.
The results of the WHO susceptibility test showed that An. gambiae s.s. was susceptible to permethrin, deltamethrin, and alpha-cypermethrin. Both laboratory-reared (Kingani strain) and wild (Lupiro strain) pyrethroid-resistant An. arabiensis mosquitoes had less than 25% mortality after exposure to these insecticides, which were restored with the addition of PBO (Supplementary Table S1).
All unwashed ITNs (Vector Guard® and Royal Sentry® 2.0) met the 2013 threshold for cone bioefficacy (WHOPES, 2013). Before and after the hut trial, the unwashed ITNs killed >80% of fully susceptible An. gambiae s.s. (Ifakara). After washing 20 times, all ITNs (Vector Guard® and Royal Sentry® 2.0) killed >95% of An. gambiae s.s., with the exception of Olyset® Plus, which killed 32.0% (95%CI = 25.0–39.0) before the experimental hut and 6.0% (95%CI = 2.8–9.2) of susceptible An. gambiae s.s. after EHT. Olyset® Plus induced >70% mortality and 95% feeding inhibition of the susceptible An. gambiae s.s. in the WHO tunnel test (Supplementary Table S2).
All unwashed (Vector Guard® and Royal Sentry® 2.0) ITNs before and after the hut trial killed >80% of pyrethroid-resistant An. arabiensis (Kingani). After 20 washes, Vector Guard® killed <40% of An. arabiensis before the experimental hut and >80% after experimental hut. Vector Guard® killed 71.3% (95%CI = 68.0–74.6) and induced 100% blood feeding inhibition of An. arabiensis in the WHO tunnel test. Olyset® Plus killed 32.0% (95%CI = 25.0–39.0) before the experimental hut and 6.0% (95%CI = 2.8–9.2) of An. arabiensis after EHT. In the WHO tunnel test, Olyset® Plus induced approximately >70% mortality and >95% feeding inhibition of An. arabiensis (Supplementary Table S2).
The comparative efficacy study of Vector Guard® net vs. the FIC Olyset® Plus nets in the experimental huts in Tanzania showed that Vector Guard® was superior to Olyset® Plus, inducing higher mortality against free-flying pyrethroid-resistant mosquitoes. This finding is consistent with a study conducted in Benin, where Olyset® Plus nets had a lower proportion of mosquito mortality compared with other PBO ITNs, including PermaNet 3.0 (deltamethrin and PBO) (Ngufor et al., 2022). This study is the first to demonstrate that an alpha-cypermethrin–PBO ITN could also be effective in controlling pyrethroid-resistant malaria vectors using a comparative efficacy methodology (WHO, 2024). It also adds to the large body of evidence that pyrethroid–PBO ITNs are more efficacious than pyrethroid-only ITNs against mosquitoes with resistance mediated by mixed-function oxidases (Gleave et al., 2021).
The superior efficacy of PermaNet® 3.0 and Vector® Guard could potentially be attributed to the active ingredient used. Vector Guard® contains the fast-acting, contact toxicant insecticide alpha-cypermethrin (Hougard et al., 2003), which might explain the higher observed mortality compared with Olyset® Plus that is treated with permethrin, an irritant insecticide that is highly effective at reducing bites, but that may reduce their contact time with the treated net, resulting in lower mortality (Achee et al., 2009). In addition, the difference between the pyrethroid–PBO ITNs could also be attributed to the specification of the ITN themselves: Olyset® Plus has permethrin and PBO on all five panels, while Vector Guard® has alpha-cypermethrin on all five panels and has PBO only on the roof. Some studies have confirmed that mosquitoes contact the roof panels first due to the odor plume produced by the host, and then move to the side panels (Parker et al., 2015). Placing PBO on the roof panel only is advantageous as it can reduce the cost of net production. The higher concentration of PBO in Vector Guard® than in Olyset® Plus might have also contributed to the higher mosquito mortality in the Vector Guard® nets than in the Olyset® Plus nets.
Pooled Vector Guard® and Olyset® Plus nets induced higher mortality compared with Royal Sentry® 2.0, a pyrethroid-only net, against pyrethroid-resistant mosquitoes. These results are consistent with those of other EHTs in Benin (Pennetier et al., 2013; Ngufor et al., 2022) and Tanzania (Kweka et al., 2017) demonstrating the superior effect of pyrethroid–PBO nets over pyrethroid-only nets on the mortality endpoint. These findings add to the existing knowledge of the additional benefit of the PBO incorporated into the nets (Gleave et al., 2021) compared with pyrethroid-only nets in areas with metabolically resistant mosquitoes, such as those in the study settings. Furthermore, the superior efficacy of pyrethroid–PBO over pyrethroid-only nets has been confirmed in large community randomized control trials (cRCTs) in Tanzania and Uganda, where the prevalence of malaria infection was lower in the PBO arms compared with the standard pyrethroid-only nets after 2 years since distribution (Protopopoff et al., 2018b; Maiteki-Sebuguzi et al., 2023). Therefore, it is cost effective to deploy pyrethroid–PBO nets specifically in areas where mosquito resistance to pyrethroids is predominant, ensuring that the investment in these enhanced nets translates to a meaningful improvement in malaria control. However, monitoring the durability of ITNs remains a critical concern to ensure that long-lasting, value-for-money products are selected (Lorenz et al., 2020), especially after a recent trial showing that Olyset® Plus ITNs were not more effective than Interceptor (alpha-cypermethrin-only) ITNs after 1 year of use (Mosha et al., 2022) and that much of the loss of community effect was attributed to rapid damage to Olyset ® Plus, leading users to discard them after 1 year (Martin et al., 2024).
The data showed different modes of action for the three net types, highlighting that ITNs are not homogeneous (Skovmand et al., 2021). The different mode of action of pyrethroids was observed as Olyset® Plus (permethrin) was superior at inhibiting mosquito blood feeding compared with the Vector Guard® and Royal Sentry® 2.0 nets, both incorporated with alpha-cypermethrin. Vector Guard® and Royal Sentry® 2.0 prevented a similar amount of mosquito blood feeding. Importantly, the performance of Royal Sentry® 2.0 in killing a greater number of mosquitoes overall compared with Olyset® Plus suggests that pyrethroid-only nets are still effective and can provide continued strong personal protection and some community protection even in areas with predominantly pyrethroid-resistant mosquitoes. Hence, the use of pyrethroid nets in resource-limited countries is still a useful malaria control tool (Briët et al., 2013) that should always be used in preference to untreated nets in order to maximize protection for humans (Pryce et al., 2018). Thus, mosquito mortality is the most important mode of action for ITNs to enhance a community-wide effect (Hawley et al., 2003), protecting even those who do not use ITNs by significantly reducing the mosquito populations, given that ITN access is only roughly around 50% (WHO, 2023b).
The susceptibility test confirmed the high resistance level in the vector population against pyrethroids in Tanzania. These findings indicate widespread resistance in the An. arabiensis population, consistent with other studies conducted in southeastern Tanzania (Matowo et al., 2017). The presence of insecticide resistance may be partly attributed to long-term exposure and the similarities of the chemicals used in agricultural pest control (Matowo et al., 2020; Urio et al., 2022). However, the susceptibility to standard insecticides was restored post-exposure to PBO, similar to findings from other studies in Tanzania (Tungu et al., 2023), suggesting that the synergist restores susceptibility to pyrethroids through inhibiting the metabolic insecticide detoxification of resistant mosquitoes (Allossogbe et al., 2017).
The mortality observed in the bioassay after washing differed before and after the EHT. It is plausible that the nets continued to regenerate during the trial, while the mortality rate for Olyset® Plus decreased post-trial, possibly due to the loss of AIs over time through evaporative loss. This study did not measure whether the AIs (alpha-cypermethrin and PBO) were washed off more quickly after washing or whether they regenerated during the hut trial. Further research is recommended to assessthe chemical content of ITNs before and after hut trials and under user conditions to better understand this process.
This study highlights that ITN products perform differently. Despite the previous threshold and performance of the Olyset® Plus net on documented public health value (Skovmand et al., 2021), Vector Guard® is a net that demonstrated higher comparative efficacy over the FIC Olyset® Plus and the standard pyrethroid-only net, Royal Sentry® 2.0. This study evaluated nets that were washed 20 times; however, it may not be comparable to real-life settings. Thus, further studies are recommended to monitor the operational performance of the ITNs after 1, 2, or 3 years of field use. Moreover, this study was conducted in Tanzania, and the results may vary in different ecological settings; therefore, additional studies are required to improve the generalizability of the findings.
Vector Guard® may be considered a suitable tool for protection against pyrethroid-resistant malaria vectors, with the potential to reduce malaria transmission based on its non-inferiority to the FIC ITN, Olyset® Plus. Olyset® Plus has demonstrated epidemiological impact and superior efficacy compared with Royal Sentry® 2.0, a pyrethroid-only net, on the 24-h mosquito mortality endpoint. Therefore, Vector Guard® should be added to the pool of PBO ITNs, providing more options for malaria programs and donors when making decisions to purchase optimal products based on demonstrated efficacy. However, further studies are recommended to evaluate the field durability of Vector Guard® under real-life conditions.
The original contributions presented in the study are included in the article/Supplementary Material. Further inquiries can be directed to the corresponding authors.
The studies involving humans were approved by The National Institute of Medical Research (NIMR) (NIMR/HQ/R.8a/Vol.IX/3559) and the Institute Review Board of Ifakara Health Institute (IHI/IRB/No:48-2020). The studies were conducted in accordance with the local legislation and institutional requirements. The participants provided their written informed consent to participate in this study.
JM: Writing – original draft, Writing – review & editing. EM: Conceptualization, Data curation, Investigation, Methodology, Project administration, Supervision, Writing – original draft, Writing – review & editing. SI: Conceptualization, Writing – original draft, Writing – review & editing. JS: Investigation, Methodology, Supervision, Writing – review & editing. WN: Data curation, Supervision, Writing – review & editing. NM: Data curation, Writing – review & editing. SN: Data curation, Investigation, Supervision, Writing – review & editing. AM: Investigation, Supervision, Writing – review & editing. OO: Formal analysis, Visualization, Writing – review & editing. UK: Formal analysis, Visualization, Writing – review & editing. SM: Conceptualization, Funding acquisition, Investigation, Methodology, Project administration, Resources, Supervision, Validation, Writing – review & editing.
The author(s) declare financial support was received for the research, authorship, and/or publication of this article. This work was supported, in whole or in part, by the Bill & Melinda Gates Foundation Award INV-022988. Under the grant conditions of the Foundation, a Creative Commons Attribution 4.0 Generic License has already been assigned to the Author Accepted Manuscript version that might arise from this submission. The funder played no role in the design of the study or interpretation of the results.
We would like to extend our gratitude to the people of Lupiro community for allowing us to perform the experimental hut trial. Additionally, we appreciate the participation of the sleepers and mosquito collectors in this study. We thank all the members of the VCPTU for their contribution. Our thanks go to Dr. Amanda Ross for insightful conversations about the data analysis.
JM, EM, JS, OO, WN, SN, AM, UK, SM conduct product evaluations for a number of companies including DCT.
The remaining authors declare that the research was conducted in the absence of any commercial or financial relationships that could be construed as a potential conflict of interest.
The author(s) declared that they were an editorial board member of Frontiers, at the time of submission. This had no impact on the peer review process and the final decision.
The author(s) declare that no Generative AI was used in the creation of this manuscript.
All claims expressed in this article are solely those of the authors and do not necessarily represent those of their affiliated organizations, or those of the publisher, the editors and the reviewers. Any product that may be evaluated in this article, or claim that may be made by its manufacturer, is not guaranteed or endorsed by the publisher.
The Supplementary Material for this article can be found online at: https://www.frontiersin.org/articles/10.3389/fmala.2024.1507392/full#supplementary-material
AI, Active ingredient; DCT, Disease control technologies; FIC, First-in-class; GLP, Good Laboratory Practice; HDPE, High-density polyethylene; IHI, Ifakara Health Institute; IHI, Ifakara Health Institute; ITN, Insecticide-treated net; LLIN, Long-lasting insecticide nets; PBO, Piperonyl butoxide; QC, Quality check; RCT, Randomized controlled trial; SANAS, South African National Accreditation System; VCPTU, Vector Control Product Testing Unit; WHO, World Health Organization; WHOPES, World Health Organization Pesticide Evaluation Scheme.
Achee N. L., Sardelis M. R., Dusfour I., Chauhan K. R., Grieco J. P. (2009). Characterization of spatial repellent, contact irritant, and toxicant chemical actions of standard vector control compounds. J. Am. Mosq. Control Assoc. 25, 156–167. doi: 10.2987/08-5831.1
Allossogbe M., Gnanguenon V., Yovogan B., Akinro B., Anagonou R., Agossa F., et al. (2017). WHO cone bio-assays of classical and new-generation long-lasting insecticidal nets call for innovative insecticides targeting the knock-down resistance mechanism in Benin. Malar J. 16, 77. doi: 10.1186/s12936-017-1727-x
(2009). MR4. Methods in Anopheles Research Manual. Available online at: https://www.beiresources.org/Publications/MethodsinAnophelesResearch.aspx (Accessed September 20, 2023).
Briët O. J., Penny M. A., Hardy D., Awolola T. S., Van Bortel W., Corbel V., et al. (2013). Effects of pyrethroid resistance on the cost effectiveness of a mass distribution of long-lasting insecticidal nets: a modelling study. Malaria J. 12, 77. doi: 10.1186/1475-2875-12-77
Casida J. E. (1970). Mixed-function oxidase involvement in the biochemistry of insecticide synergists. J. Agric. Food Chem. 18, 753–772. doi: 10.1021/jf60171a013
Gilbert P. B., Qin L., Self S. G. (2008). Evaluating a surrogate endpoint at three levels, with application to vaccine development. Stat. Med. 27, 4758–4778. doi: 10.1002/sim.v27:23
Gleave K., Lissenden N., Chaplin M., Choi L., Ranson H. (2021). Piperonyl butoxide (PBO) combined with pyrethroids in insecticide-treated nets to prevent malaria in Africa. Cochrane Database Syst. Rev. 5, CD012776. doi: 10.1002/14651858.CD012776.pub3
Hawley W. A., Phillips-Howard P. A., Ter Kuile F. O., Terlouw D. J., Vulule J. M., Ombok M., et al. (2003). Community-wide effects of permethrin-treated bed nets on child mortality and malaria morbidity in Western Kenya. Am. J. Trop. Med. Hygiene Am. J. Trop. Med. Hyg 68, 121–127. doi: 10.4269/ajtmh.2003.68.121
Hougard J.-M., Duchon S., Darriet F., Zaim M., Rogier C., Guillet P. (2003). Comparative performances, under laboratory conditions, of seven pyrethroid insecticides used for impregnation of mosquito nets. Bull World Health Organisation 81 (5), 324–333.
Kweka E. J., Lyaruu L. J., Mahande A. M. (2017). Efficacy of PermaNet® 3.0 and PermaNet® 2.0 nets against laboratory-reared and wild Anopheles Gambiae sensu lato populations in northern Tanzania. Infect. Dis. Poverty 6, 11. doi: 10.1186/s40249-016-0220-z
Lorenz L. M., Bradley J., Yukich J., Massue D. J., Mageni Mboma Z., Pigeon O., et al. (2020). Comparative functional survival and equivalent annual cost of 3 long-lasting insecticidal net (LLIN) products in Tanzania: A randomised trial with 3-year follow up. PloS Med. 17, e1003248. doi: 10.1371/journal.pmed.1003248
Maiteki-Sebuguzi C., Gonahasa S., Kamya M. R., Katureebe A., Bagala I., Lynd A., et al. (2023). Effect of long-lasting insecticidal nets with and without piperonyl butoxide on malaria indicators in Uganda (LLINEUP): final results of a cluster-randomised trial embedded in a national distribution campaign. Lancet Infect. Dis. 23, 247–258. doi: 10.1016/S1473-3099(22)00469-8
Martin J., Lukole E., Messenger L. A., Aziz T., Mallya E., Bernard E., et al. (2024). Monitoring of fabric integrity and attrition rate of dual-active ingredient long-lasting insecticidal nets in Tanzania: A prospective cohort study nested in a cluster randomized controlled trial. Insects 15, 108. doi: 10.3390/insects15020108
Massue D. J., Kisinza W. N., Malongo B. B., Mgaya C. S., Bradley J., Moore J. D., et al. (2016). Comparative performance of three experimental hut designs for measuring malaria vector responses to insecticides in Tanzania. Malar J. 15, 165. doi: 10.1186/s12936-016-1221-x
Matowo N. S., Munhenga G., Tanner M., Coetzee M., Feringa W. F., Ngowo H. S., et al. (2017). Fine-scale spatial and temporal heterogeneities in insecticide resistance profiles of the malaria vector, Anopheles arabiensis in rural south-eastern Tanzania. Wellcome Open Res. 2, 96. doi: 10.12688/wellcomeopenres
Matowo N. S., Tanner M., Munhenga G., Mapua S. A., Finda M., Utzinger J., et al. (2020). Patterns of pesticide usage in agriculture in rural Tanzania call for integrating agricultural and public health practices in managing insecticide-resistance in malaria vectors. Malar J. 19, 257. doi: 10.1186/s12936-020-03331-4
Menze B. D., Mugenzi L. M. J., Tchouakui M., Wondji M. J., Tchoupo M., Wondji C. S. (2022). Experimental Hut Trials Reveal That CYP6P9a/b P450 Alleles Are Reducing the Efficacy of Pyrethroid-Only Olyset Net against the Malaria Vector Anopheles funestus but PBO-Based Olyset Plus Net Remains Effective. Pathogens 11(6), 638. doi: 10.3390/pathogens11060638
Mosha J. F., Kulkarni M. A., Lukole E., Matowo N. S., Pitt C., Messenger L. A., et al. (2022). Effectiveness and cost-effectiveness against malaria of three types of dual-active-ingredient long-lasting insecticidal nets (LLINs) compared with pyrethroid-only LLINs in Tanzania: a four-arm, cluster-randomised trial. Lancet 399, 1227–1241. doi: 10.1016/S0140-6736(21)02499-5
Ngufor C., Fagbohoun J., Agbevo A., Ismail H., Challenger J. D., Churcher T. S., et al. (2022). Comparative efficacy of two pyrethroid-piperonyl butoxide nets (Olyset Plus and PermaNet 3.0) against pyrethroid resistant malaria vectors: a non-inferiority assessment. Malar J. 21, 20. doi: 10.1186/s12936-022-04041-9
Odufuwa O. G., Bradley J., Ngonyani S., Mpelepele A. B., Matanila I., Muganga J. B., et al. (2024). Time of exposure and assessment influence the mortality induced by insecticides against metabolic resistant mosquitoes. Parasit Vectors 17, 103. doi: 10.1186/s13071-024-06190-z
Parker J. E., Angarita-Jaimes N., Abe M., Towers C. E., Towers D., McCall P. J. (2015). Infrared video tracking of Anopheles Gambiae at insecticide-treated bed nets reveals rapid decisive impact after brief localised net contact. Sci. Rep. 5, 13392. doi: 10.1038/srep13392
Pennetier C., Bouraima A., Chandre F., Piameu M., Etang J., Rossignol M., et al. (2013). Efficacy of Olyset(R) Plus, a new long-lasting insecticidal net incorporating permethrin and piperonyl-butoxide against multi-resistant malaria vectors [corrected. PloS One 8, e75134. doi: 10.1371/annotation/bed4305a-d665-4150-a682-a20d9cf9b79f
Piaggio G., Elbourne D. R., Pocock S. J., Evans S. J., Altman D. G. (2012). Reporting of noninferiority and equivalence randomized trials: extension of the CONSORT 2010 statement. JAMA 308, 2594–2604. doi: 10.1001/jama.2012.87802
Pinda P. G., Eichenberger C., Ngowo H. S., Msaky D. S., Abbasi S., Kihonda J., et al. (2020). Comparative assessment of insecticide resistance phenotypes in two major malaria vectors, Anopheles funestus and Anopheles arabiensis in south-eastern Tanzania. Malaria J. 19, 408. doi: 10.1186/s12936-020-03483-3
Protopopoff N., Mosha J. F., Lukole E., Charlwood J. D., Wright A., Mwalimu C. D., et al. (2018a). Effectiveness of a long-lasting piperonyl butoxide-treated insecticidal net and indoor residual spray interventions, separately and together, against malaria transmitted by pyrethroid-resistant mosquitoes: a cluster, randomised controlled, two-by-two factorial design trial. Lancet 391, 1577–1588. doi: 10.1016/S0140-6736(18)30427-6
Protopopoff N., Mosha J. F., Lukole E., Charlwood J. D., Wright A., Mwalimu C. D., et al. (2018b). Effectiveness of a long-lasting piperonyl butoxide-treated insecticidal net and indoor residual spray interventions, separately and together, against malaria transmitted by pyrethroid-resistant mosquitoes: a cluster, randomised controlled, two-by-two fact. Lancet 391, 1577–1588. doi: 10.1016/S0140-6736(18)30427-6
Pryce J., Richardson M., Lengeler C. (2018). Insecticide-treated nets for preventing malaria. Cochrane Database Syst. Rev. 11, CD000363. doi: 10.1002/14651858.CD000363.pub3
R Core Team. (2021). R: A Language and Environment for Statistical Computing (Vienna, Austria: R Foundation for Statistical Computing).
Rehal S., Morris T. P., Fielding K., Carpenter J. R., Phillips P. P. (2016). Non-inferiority trials: are they inferior? A systematic review of reporting in major medical journals. BMJ Open 6, e012594. doi: 10.1136/bmjopen-2016-012594
Sherrard-Smith E., Winskill P., Hamlet A., Ngufor C., N’Guessan R., Guelbeogo M. W., et al. (2022). Optimising the deployment of vector control tools against malaria: a data-informed modelling study. Lancet Planetary Health 6, e100–e1e9. doi: 10.1016/S2542-5196(21)00296-5
Skovmand O., Dang D. M., Tran T. Q., Bossellman R., Moore S. J. (2021). From the factory to the field: considerations of product characteristics for insecticide-treated net (ITN) bioefficacy testing. Malaria J. 20, 363. doi: 10.1186/s12936-021-03897-7
Staedke S. G., Gonahasa S., Dorsey G., Kamya M., Maiteki-Sebuguzi C., Lynd A., et al. (2020). LLIN Evaluation in Uganda Project (LLINEUP) – Effect of long-lasting insecticidal nets (LLINs) with, and without, piperonyl butoxide on malaria indicators in Uganda: a cluster-randomised trial embedded in a national LLIN distribution campaign. Lancet 395, 1292–1303. doi: 10.1016/S0140-6736(20)30214-2
Swai J. K., Soto A. C., Ntabaliba W. S., Kibondo U. A., Ngonyani H. A., Mseka A. P., et al. (2023). Efficacy of the spatial repellent product Mosquito Shield against wild pyrethroid-resistant Anopheles arabiensis in south-eastern Tanzania. Malar J. 22, 249. doi: 10.1186/s12936-023-04674-4
Tungu P., Kabula B., Nkya T., Machafuko P., Sambu E., Batengana B., et al. (2023). Trends of insecticide resistance monitoring in mainland Tanzania, 2004-2020. Malar J. 22, 100. doi: 10.1186/s12936-023-04508-3
Urio N. H., Pinda P. G., Ngonzi A. J., Muyaga L. L., Msugupakulya B. J., Finda M., et al. (2022). Effects of agricultural pesticides on the susceptibility and fitness of malaria vectors in rural south-eastern Tanzania. Parasit Vectors 15, 213. doi: 10.1186/s13071-022-05318-3
WHO. (2017). Conditions for Deployment of Mosquito Nets Treated With a Pyrethroid and Piperonyl Butoxide (Geneva: World Health Organisation Global Malaria Program).
WHO. (2018). Determining Non-Inferiority of Insecticide-Treated Nets and Indoor Residual Spray Products Within an Established Product Class – Evidence Review Group Meeting Report (Geneva: World Health Organization).
WHO. (2021). Technical Consultation on Determining Non-inferiority of Vector Control Products Within an Established Class. (Geneva: World Health Organization).
WHO. (2023c). Data Requirements and Protocol for Determining Comparative Efficacy of Vector Control Products, With a Focus on Insecticidetreated Nets and Indoor Residual Spraying Products, 2nd ed. (Geneva: World Health Organization)
WHO. (2023d). Technical Consultation to Assess Comparative Efficacy of Vector Control Products: Meeting Report, 5 and 9 June 2023 (Geneva: World Health Organization).
WHO. (2024). Data Requirements and Protocol for Determining Comparative Efficacy of Vector Control Products (Geneva: World Health Organization).
Keywords: insecticide-treated net, Vector Guard®, pyrethroids, resistance, piperonyl butoxide, experimental huts, Anopheles arabiensis, Tanzania
Citation: Machange JJ, Mbuba E, Irish SR, Swai JK, Ntabaliba W, Makungwa NO, Ngonyani S, Mpelepele AB, Kibondo UA, Odufuwa OG and Moore SJ (2024) Comparative efficacy of Vector Guard® to Olyset® Plus insecticide-treated nets against strongly pyrethroid-resistant Anopheles arabiensis in experimental huts in Tanzania. Front. Malar. 2:1507392. doi: 10.3389/fmala.2024.1507392
Received: 07 October 2024; Accepted: 28 November 2024;
Published: 24 December 2024.
Edited by:
Nancy Stephen Matowo, University of London, United KingdomReviewed by:
Adilson José DePina, CCS-SIDA/MoH, Cabo VerdeCopyright © 2024 Machange, Mbuba, Irish, Swai, Ntabaliba, Makungwa, Ngonyani, Mpelepele, Kibondo, Odufuwa and Moore. This is an open-access article distributed under the terms of the Creative Commons Attribution License (CC BY). The use, distribution or reproduction in other forums is permitted, provided the original author(s) and the copyright owner(s) are credited and that the original publication in this journal is cited, in accordance with accepted academic practice. No use, distribution or reproduction is permitted which does not comply with these terms.
*Correspondence: Jane Johnson Machange, am1hY2hhbmdlQGloaS5vci50eg==; Emmanuel Mbuba, ZW1idWJhQGloaS5vci50eg==
Disclaimer: All claims expressed in this article are solely those of the authors and do not necessarily represent those of their affiliated organizations, or those of the publisher, the editors and the reviewers. Any product that may be evaluated in this article or claim that may be made by its manufacturer is not guaranteed or endorsed by the publisher.
Research integrity at Frontiers
Learn more about the work of our research integrity team to safeguard the quality of each article we publish.