- Medical Faculty, Centre for Infectious Diseases, Heidelberg University, University Hospital Heidelberg, Heidelberg, Germany
To date, insecticide-treated bed nets are the most effective tool in preventing malaria-related morbidity and mortality. All distributed bed nets contain pyrethroid insecticides; however, widespread resistance to this class in the malaria vectors, Anopheles spp., has led to the development of dual active ingredient bed nets, termed ‘next-generation bed nets’. These nets combine pyrethroids with a second chemistry, aimed at countering pyrethroid resistance. The World Health Organization (WHO) recently issued recommendations for three classes of next-generation bed nets. In this review, we report on key indicators across Africa for each net type. The results underscore a substantial variation in key indicators across Africa when comparing next-generation bed nets to traditional pyrethroid-only nets. Nevertheless, dual active ingredient bed nets generally outperform pyrethroid-only nets in a various settings. The results show that new nets are a formidable tool in malaria control, with superior performance compared to traditional pyrethroid-only nets. The variability in outcomes across Africa underscores the need for a region-specific approach, fostering a targeted and adaptive strategy for the deployment of these next-generation interventions.
Introduction
Malaria remains a significant global health challenge with an estimated 247 million cases and 619,000 deaths in 2021 alone (WHO, 2023). To date, long-lasting insecticidal nets (ITNs) are the most effective intervention in malaria control and are the main contributing factor to a reduction in morbidity and mortality (Bhatt et al., 2015; WHO, 2023). The most used class of insecticides are pyrethroids (PY), utilised on all bed nets distributed (Supplementary Table S1). Due to the intense selection pressure exerted on the mosquito, pyrethroid resistance is widespread; therefore, dual active ingredient (AI) bed nets have recently been recommended for use (Programme, 2021). These so-called ‘next-generation bed nets’ (ngITNs) combine pyrethroids with either a second insecticide or a synergist that restores susceptibility to pyrethroids by blocking metabolism of the insecticide (Supplementary Table S1) (Metcalf, 1967; WHO, 2012).
In March 2023, WHO issued recommendations for the three newly licensed next-generation ITNs, with a strong recommendation for the use of pyrethroid + chlorfenapyr (CFP) nets over the use of pyrethroid-only nets (pITNs) (WHO, 2023). CFP is a second insecticide that targets the mitochondria of insects (Anon, 1995), and thus, has a complementary mode of action to pyrethroids. The second class of dual active ITNs combines piperonyl butoxide (PBO), which inhibits cytochrome P450s (P450s) from detoxifying pyrethroids, and thus, restores susceptibility. Following WHO’s recommendation for the use of pyrethroid + PBO ITNs in settings with high pyrethroid resistance, almost half of the currently distributed ITNs are pyrethroids + PBO nets (WHO, 2023). Finally, WHO issued a conditional recommendation for pyrethroid + pyriproxyfen (PPF) nets (Organization, 2023). PPF, a juvenile hormone analogue, sterilises adult mosquitoes after contact and reduces their lifespan (Itoh et al., 1994; Dhadialla et al., 1998).
To best implement these recommendations, understanding of the underlying pyrethroid resistance mechanisms is needed [recently reviewed in (Ingham et al., 2023)]. Pyrethroid resistance can be due to a number of mechanisms, including well-described changes to the target site of the insecticide (kdr) (Martinez-Torres et al., 1998; Weill et al., 2004), upregulation of metabolic detoxification genes (specifically P450s) (Challenger et al., 2023), and thickening of the cuticle (Balabanidou et al., 2016).
In this review, we descriptively report on diverse key indicators (Table 1) collected in Phase I–III trials in Africa on ngITNs (Supplementary Table S1). These studies represent published data from 40 trials from dual AI vs. PY-only nets [PBO (n = 33), PPF (n = 4), CFP (n = 14)]. Included studies utilised ITNs in the field with either field-caught or laboratory-reared resistant Anopheles spp., and studies that tested ITNs in a laboratory environment using field-caught resistant Anopheles spp. (Supplementary Table S1). Phase I trials (n = 7) identified ITN-induced mortality in association with resistance mechanisms, Phase II (n = 23) studies examined ITN-specific EHT outcome measures, and Phase III (n = 10) trials investigated ITN impact on epidemiological markers. All data given as exact metrics were extracted from West (Pwalia et al., 2019), Central (WHO, 2023), and East (Challenger et al., 2023) Africa (Supplementary Table S1). Relevant papers were retrieved from Google Scholar and Web of Science using search terms described in Supplementary Table S1. Accounting for confounding effects such as phase of trial, trial design, methodology, or locality are beyond the scope of this review but have been addressed in prior statistical studies (Sherrard-Smith et al., 2022; Challenger et al., 2023).
Malaria epidemiology
Parasite and anaemia prevalence
Several trials were conducted across West Africa, an area with relatively higher levels of insecticide resistance (Hancock et al., 2022). In Burkina Faso, a randomised controlled trial (RCT) with An. gambiae s.l. and An. funestus reported a decline in prevalence and incidence with the use of PermaNet 3.0 (PBO) and IG2 (CFP) compared to pITNs, with PermaNet 3.0 showing the greatest reduction in prevalence (Gansané et al., 2023). Similarly, in Benin, IG2 significantly reduced case incidence, infection prevalence, and entomological inoculation rate (EIR) over 24 months with An. gambiae and An. funestus. In contrast, the PPF net (Royal Guard, RG) did not impact prevalence, although the EIR was reduced (Accrombessi et al., 2023). As in Burkina Faso, PermaNet 3.0 performed well in Nigeria (1-year RCT) with a greater impact on EIR and significantly larger impact on sporozoite rates with An. gambiae s.l (Awolola et al., 2014). However, in Mali (2-year RCT) PermaNet 3.0 and Olyset Plus did not perform well and provided no evidence for a difference in sporozoite rate in An. gambiae s.l., despite pre-exposure to PBO indicating partial involvement of P450s (Cisse et al., 2017). In Tanzania, a significant reduction in case incidence and prevalence was observed in IG2 compared to pITNs, whilst no reductions were reported in Olyset Plus or RG after 2 years (Mosha et al., 2022). EIR was significantly reduced in IG2 for 2 years, 1 year for Olyset Plus, and no difference for RG (Mosha et al., 2022). By the third-year, post intervention analysis revealed only IG2 had a significant decline in EIR (Mosha et al., 2023). A trial in Uganda (18-month RCT) reported that PermaNet 3.0 and Olyset Plus significantly lowered per protocol parasite prevalence in An. gambiae s.l. and An. Funestus (Staedke et al., 2020). Additionally, in Tanzania (with a 33-month study) with An. gambiae s.l. and An. funestus, Olyset Plus had a lower parasite prevalence; there was no significant difference in EIR and sporozoite rates (Protopopoff et al., 2023).
No differences were reported in anaemia prevalence amongst children across Africa when comparing ngITNs to pITNs over the entire trial period (Accrombessi et al., 2023; Mosha et al., 2023; Protopopoff et al., 2023).
Overall, PBO and CFP nets show a generalised reduction in parasite indicators across both East and West Africa [e.g., sporozoite rate pITN:median = 2% (range=1.3%–5%), CFP:0.8% (0.6%–1.2%), PBO:1.9% (0.9%–6.9%)], with only one trial in Mali showing no impact of PBO nets compared to pITNs (Figure 1A).
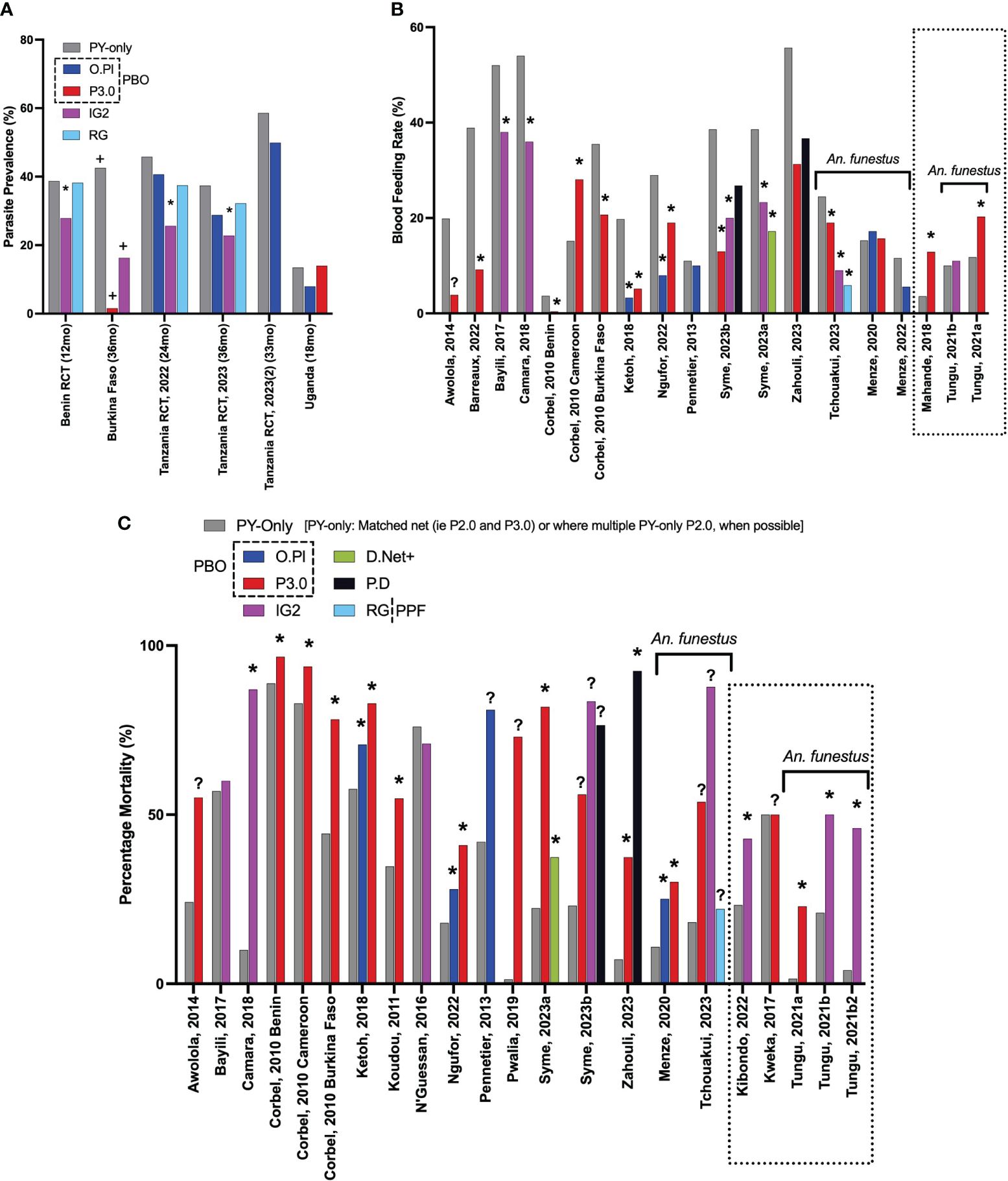
Figure 1 Key indicators from selected studies on next-generation bed net efficacy. (A) Parasite prevalence (%) indicated on the y-axis from six trials (x-axis). Trial duration is indicated next to the country, whether it was a randomised controlled trial (RCT) and the year of publication (not trial dates). * indicates significance compared to the PY-only control net as reported in the studies; + indicates significance from baseline data. (B) The blood-feeding rate (%) is given on the x-axis with study citation on the y-axis. * indicates significance compared to PY-only control nets as reported in the studies;? indicates no statistics reported. (C) This shows the percentage of mortality (%) of field mosquitoes exposed to field nets for each relevant study (y-axis). * indicates significance compared to PY-only control nets as reported in the studies;? indicates no statistics reported. Unless otherwise indicated, studies were performed with An. gambiae s.l.; studies within the dashed box took place in East Africa; those without, took place in West Africa. The types of nets are differentiated according to colour: PY-only (gray); Olyset Plus (dark blue); PermaNet 3.0 (red); Interceptor G2 (pink); DuraNet+ (green); PermaNet Dual (black), and Royal Guard (light blue). All the data are available in Supplementary Table S1.
Entomological markers
Mortality and sterility
In West Africa, PermaNet 3.0, Olyset Plus, and Veeralin nets across Côte d’Ivoire, Burkina Faso, Ghana, Togo, Benin, Nigeria, and Cameroon reported higher mortality levels compared to pITNs in An. arabiensis, An. funestus, and An. gambiae s.s./s.l (Corbel et al., 2010; Koudou et al., 2011; Pennetier et al., 2013; Awolola et al., 2014; Ketoh et al., 2018; Oumbouke et al., 2019; Pwalia et al., 2019; Tchakounte et al., 2019; Ibrahim et al., 2020; Menze et al., 2020; Barreaux et al., 2022; Menze et al., 2022; Tchouakui et al., 2023). To confirm that the success of PBO is through direct inhibition of P450s, studies provided evidence of P450-mediated resistance through several indicators: mortality in bioassays with a pre-exposure to PBO; P450 activity assays; and higher frequencies of P450 allelic markers (Awolola et al., 2014; Ketoh et al., 2018; Menze et al., 2022). Contrastingly, a transversal study conducted throughout Benin highlighted variability in mortality levels, with no clear indication of the performance of PBO nets (Allossogbe et al., 2017).
CFP nets induced higher mortality than pITNs encompassing pyrethroid resistant (PR) An. funestus and An. gambiae s.l. in Côte d’Ivoire, Burkina Faso, Benin, and Cameroon (Bayili et al., 2017; Camara et al., 2018; Syme et al., 2023b; Syme et al., 2023a; Tchouakui et al., 2023). Differences were evident between net manufacturers, with IG2 outperforming PBO and other CFP nets (PermaNet 3.0, DuraNet Plus, and PermaNet Dual) in Cameroon and Benin (Syme et al., 2023b; Syme et al., 2023a; Tchouakui et al., 2023), whilst in Côte d’Ivoire and Benin, the use of PermaNet 3.0 and PermaNet Dual resulted in higher mortality than RG and Olyset Plus (Ngufor et al., 2022; Tchouakui et al., 2023).
RG trialled in Cameroon with resistant field An. gambiae s.l. and An. funestus showed no increased mortality compared to pITNs (Tchouakui et al., 2023). The only other published RG study recorded that mid-low mortality levels are induced with An. gambiae s.l. from Benin; however, mosquitoes were completely sterile after exposure (Zoungbedji et al., 2023).
In East Africa, PermaNet 3.0 was compared to pITNs in Uganda and Ethiopia and results show higher mortality in An. gambiae s.s. and An. arabiensis (Yewhalaw et al., 2012; Okia et al., 2013). Conversely in Tanzania and Kenya, there was no difference between PermaNet 3.0 and PermaNet 2.0; however, in Kenya, PermaNet 3.0 induced higher mortality levels than Olyset Net (Kweka et al., 2017; Omondi et al., 2017). A single study in Central Africa was conducted in the Democratic Republic of Congo (DRC) in which the use of PermaNet 3.0 caused significantly higher rates of mortality than pITNs (Thierry Bobanga et al., 2013). Unlike West Africa, RG exposure did not result in significant sterilisation of An. funestus or An. gambiae s.l. from Tanzania (Mosha et al., 2022). Also in Tanzania, IG2 significantly reduced mortality when compared to pITNs (Tungu et al., 2021a).
Overall, CFP nets showed consistently higher mortality than pITNs in West Africa [pITN:22.4% (7.2%–76%), CFP:82.9% (60%–92.5%)], whilst PBO nets generally showed higher mortality than their pITN counterparts [pITN:26.2% (7.2%–88.8%), PBO:54.9% (25.1%–99.1%)]; however, this is not consistent across all studies in West and East Africa (Figure 1B).
Reduction in blood feeding
In West Africa, PBO nets consistently demonstrated a decrease in blood-feeding ability compared to pITNs. Studies in Burkina Faso, Cameroon, and Togo; two studies in Benin; and three studies in Côte d’Ivoire showed significantly reduced ability to take a blood meal due to exposure to a variety of PBO nets in An. gambiae s.s., An. gambiae s.l., and An. funestus (Corbel et al., 2010; Ketoh et al., 2018; Oumbouke et al., 2019; Barreaux et al., 2022; Menze et al., 2022; Ngufor et al., 2022; Syme et al., 2023a; Zahouli et al., 2023). Only one study showed an increase in blood-feeding rate after PBO net exposure in Cameroon (Corbel et al., 2010), whilst no difference was seen between PBO and pITNs in Benin, Côte d’Ivoire, and Cameroon (Koudou et al., 2011; Pennetier et al., 2013; Menze et al., 2020). Interestingly, a study in Benin demonstrated that PBO nets show significantly increased blood-feeding inhibition compared to CFP nets (Syme et al., 2023a). One study directly compared blood-feeding inhibition with Olyset Plus and PermaNet 3.0 and saw no difference between the nets (Ketoh et al., 2018). Similar observations were made for CFP-containing PermaNet Dual, with a general decrease in blood-feeding ability in Benin (Syme et al., 2023b). IG2 also reported a significantly reduced BF ability compared to PY-only nets in An. gambiae s.l. from Burkina Faso, Benin, Cameroon, and Côte d’Ivoire (Bayili et al., 2017; Camara et al., 2018; Syme et al., 2023a; Tchouakui et al., 2023). One study on RG in Cameroon shows a reduced BF rate (Tchouakui et al., 2023).
In contrast, in East Africa, no difference in blood-feeding ability was seen with IG2 in Tanzania (Tungu et al., 2021a) whilst two studies in Tanzania with An. funestus and An. arabiensis showed increased blood-feeding rates with PBO nets compared to pITNs (Mahande et al., 2018; Tungu et al., 2021a; Tungu et al., 2021b; Kibondo et al., 2022).
Generally, in West Africa, blood-feeding inhibition is increased [pITN:52.5% (12.8%–90.1%), CFP:58.4% (2%–92%), PBO:72.6% (46.1%–98.7%)], and blood-feeding rate is decreased [pITN:22.2% (3.7%–55.8%), CFP:21.7% (2.38%), PBO:11.5% (0.4%–31.3%), RG:24.5%] when mosquitoes are exposed to next-generation bed nets regardless of the manufacturer or chemistry. The studies present evidence that PBO-containing nets have the best blood-feeding deterrence. Interestingly, this contrasts completely with East Africa where studies show no increased efficacy in preventing blood feeding (Figure 1C).
Deterrence
Multiple studies across West Africa reported differing deterrence levels of next-generation nets compared to pITNs. In the bordering countries Burkina Faso and Togo, there was evidence that PBO nets (Olyset Plus and PermaNet 3.0) significantly deterred An. gambiae s.l. when trialled in experimental huts compared to pITNs (Corbel et al., 2010; Ketoh et al., 2018). PermaNet 3.0 also performed better with An. funestus in Cameroon; however, this study reported that a permethrin-PBO combination (Olyset Plus) did not provide the same improved level of deterrence (Menze et al., 2020). Similarly, An. gambiae s.l. in Cameroon and Benin were not increasingly deterred by PermaNet 3.0 (Corbel et al., 2010); however, the impact in Benin has been contradicted more recently where both PermaNet 3.0 and Olyset Plus increased deterrence compared to pITNs (Ngufor et al., 2022). To date, the only published data from Côte d’Ivoire present no difference in deterrence between PBO nets compared to pITNs (Koudou et al., 2011; Oumbouke et al., 2019). Interestingly, PermaNet Dual also had no improved effect on deterrence in Côte d’Ivoire compared to pITNs (Camara et al., 2018; Zahouli et al., 2023). IG2 also had no impact on deterrence in Benin and Burkina Faso (N’Guessan et al., 2016; Bayili et al., 2017; Syme et al., 2023a).
Less data are available about Anopheline deterrence rates in Eastern Africa. Data from Tanzania concluded that PermaNet 3.0 (Kweka et al., 2017; Tungu et al., 2021b) and Veeralin (Tungu et al., 2021b) increased deterrence compared to pITNs. Contrastingly, IG2 in Tanzania showed no increase in deterrence with An. gambiae s.l (Tungu et al., 2021a).
Both West and East African studies reported mixed results on deterrence with PBO-treated bed nets compared to pITNs, whilst CFP nets did not seem to increase deterrence.
Exophily
The impact on An. gambiae s.l. was investigated in West African hut trials finding increased exophily in PBO nets: PermaNet 3.0 (Cote d’Ivoire), Olyset Plus (Togo), and DuraNet Plus (Benin) compared to pITNs (Ketoh et al., 2018; Syme et al., 2023b; Syme et al., 2023a; Zahouli et al., 2023). In contrast, in other areas, PermaNet 3.0 (Benin, Burkina Faso, Cameroon, and Côte d’Ivoire), Olyset Plus (Benin), and Veeralin (Côte d’Ivoire) induced no impact on exophily in An. gambiae s.l (Corbel et al., 2010; Koudou et al., 2011; Oumbouke et al., 2019). nor in An. funestus in Cameroon (Menze et al., 2020; Tchouakui et al., 2023). Similarly heterogenous findings were seen for exophily, following exposure to IG2 across West Africa. An increase in exophily compared to pITNs was observed in An. gambiae s.l. populations from Benin and Cote d’Ivoire (Camara et al., 2018; Syme et al., 2023a). In contrast, no difference in exophily was reported in An. gambiae s.l. in Burkina Faso and Benin (N’Guessan et al., 2016; Bayili et al., 2017). When two CFP-containing nets were compared, there was no difference in exophily (Syme et al., 2023a).
In East Africa, no difference in exophily was reported from PBO ITNs, including PermaNet 3.0, in An. arabiensis and An. funestus and Veeralin in An. funestus in Tanzania (Kweka et al., 2017; Tungu et al., 2021b). Similarly, a single study reported no differences in exophily in one trial and increased exophily in a second trial following IG2 exposure at the same site with An. funestus (Tungu et al., 2021a).
Several studies attempted to discern the impact of the chlorfenapyr component of next-generation nets, through using a chlorfenapyr-only net (CTN). Some studies showed CTN significantly decreased exophily, suggesting that the alpha-cypermethrin component of IG2 may be responsible for induced exophily (N’Guessan et al., 2016; Bayili et al., 2017; Tungu et al., 2021a); however, a study from Cote d’Ivoire contradicted these findings, reporting that CTN had higher exophily than pITNs (Camara et al., 2018).
As with deterrence, the data for exophily are mixed across East and West Africa with little indication that PBO or CFP contribute to changes in exophily.
Direct ITN measures
Durability
Across West Africa, PBO nets show a significant reduction in active ingredient (AI) at the ‘end of life’ point as defined by WHO. All studies on PermaNet 3.0 following 20 washes showed a significant reduction in AI, or a reduced mortality in An. gambiae s.s. and An. gambiae s.l (Corbel et al., 2010; Koudou et al., 2011; Thierry Bobanga et al., 2013; Ngufor et al., 2022). Similarly, Olyset Plus was reported to retain only around 75% PY and 25%–50% PBO content, which translated to a reduced mortality (Pennetier et al., 2013; Ketoh et al., 2018). However, the Veeralin PBO net appears not to lose a significant amount of active ingredient after 20 washes, though mortality was reduced in PR An. gambiae s.l (Oumbouke et al., 2019).
Contrastingly, CFP nets exhibited good insecticide retention in West Africa (Syme et al., 2023a), with PermaNet Dual retaining more AI than IG2 post-washing (Syme et al., 2023b), and with better PY content retention than PermaNet 2.0 and 3.0 (Zahouli et al., 2023). CFP nets also resulted in high mortality, outperforming pITNs after washing in Cameroon (N’Guessan et al., 2016; Barreaux et al., 2022; Tchouakui et al., 2023) and Côte d’Ivoire (Zahouli et al., 2023). In experimental hut trials, mortality with IG2 nets did not decrease significantly throughout the studies (Bayili et al., 2017; Camara et al., 2018).
In East Africa, similar observations have been made. Several studies reported reduced AI in nets, often consistent with a reduction in mortality. Both PBO nets PermaNet 3.0 and Olyset Plus showed significant reductions in AI content (Awolola et al., 2014; Gichuki et al., 2021; Mechan et al., 2022; Mosha et al., 2022). Interestingly, the pITN Olyset Net appeared to retain PY better than Olyset Plus (Gichuki et al., 2021). Furthermore, trials that compared net efficacy after 12–36 months found significant reductions in both permethrin and PBO content in PermaNet 3.0 and Olyset Plus; however, one study showed no impact on the mortality of a susceptible An. gambiae lab strain (Staedke et al., 2020; Protopopoff et al., 2023). Despite the low retention of AI on Olyset Plus (7% PBO) and RG (28% PPF), the parasite indicators were not significantly impacted (Mosha et al., 2023). The durability of the Olyset Net and Olyset Plus nets in terms of development of holes was also comparable, with both nets in good condition after a 36-month phase III trial (Gichuki et al., 2021).
Unlike in West Africa, IG2 was reported to experience an insecticidal content reduction in both alpha-cypermethrin and CFP after 20 washes, though mortality was not impacted (Tungu et al., 2021a). The final year analysis of hut trials (36 months) reported that IG2 had a chlorfenapyr retention of 8%, yet still induced a significant impact on parasite prevalence and EIR (Staedke et al., 2020; Protopopoff et al., 2023).
Overall, nets maintained good condition as defined by WHO over a 12-month period, with a decline after 25 months, exhibiting comparable levels between various net types (Staedke et al., 2020; Mechan et al., 2022).
In both West and East Africa, PBO nets experienced a large reduction in AI retention [pyr:73% (21%–96%), PBO:51% (4%–105%)], which often but not always translated to a reduction in mortality [e.g., wash mortality pITN:23.7% (10.3%–70.7%), PBO:43.8% (14.2%–77.9%)]. In contrast, CFP-containing nets appeared to maintain insecticidal content in West Africa [pyr:90% (80%–100%), CFP:66% (63%–90%)] whilst exhibiting a decrease in East Africa [pyr:31% (22%–59%), CFP:18% (6%–32%)]; nevertheless, the efficacy of the nets did not seem to decrease [e.g., wash mortality pITN:13% (11%–14.2%), CFP:65% (65%–74.9%)].
Personal protection
Across West Africa, amongst An. gambiae s.l. PBO ITNs, DuraNet Plus (Benin), Veeralin (Côte d’Ivoire), PermaNet 3.0 (Togo, Cameroon), and Olyset Plus (Togo), provided greater personal protection (PP) than pITNs (Ketoh et al., 2018; Oumbouke et al., 2019; Menze et al., 2020; Syme et al., 2023a). However, no differences in PP were reported by Olyset Plus in An. funestus from Cameroon (Menze et al., 2020) and An. gambiae s.l. in Benin and Burkina Faso (Corbel et al., 2010). In contrast, PermaNet 3.0 provided less PP than pITNs in An. gambiae s.l. from Cameroon (Corbel et al., 2010). For CFP ITNs, greater PP was provided with IG2 than pITNs in An. gambiae s.l. in Benin (Syme et al., 2023a), Cote d’Ivoire, and Burkina Faso (Bayili et al., 2017; Camara et al., 2018). In Benin and Burkina Faso, lower PP was reported for CTN nets (N’Guessan et al., 2016; Bayili et al., 2017) implying that the alpha-cypermethrin component may provide some protection. When PY-PBO (DuraNet Plus) and IG2 were compared in Benin, IG2 provided superior PP (Syme et al., 2023a). PermaNet Dual was non-inferior to IG2 in providing personal protection (Syme et al., 2023b).
In Tanzania, PermaNet 3.0 (Tanzania) offered similar levels of PP (100%) to PY-only with An. arabiensis populations (Kweka et al., 2017). The only other study showed contradicting PP data with IG2 and pITNs across two trials (Tungu et al., 2021a).
Overall, PP results are heterogenous for both CFP and PBO-containing nets, with increased, no difference, and decreased levels of PP compared to pITNs [pITN:70% (24%–100%), CFP:59% (31%–88%), and PBO:85% (53%–100%)].
Discussion
This review outlines the substantial variability in indicators across different African settings in the comparison of ngITNs compared to pITNs, with no single metric reported across independent studies (Supplementary Table S1). Deducing statistically relevant outputs for ngITNs and pITNs is also challenging as untreated nets are regularly used for reference. Alternating experimental design adds further complexities to performing broad-scale analyses of ngITNs compared to pITNs, notably for testing net durability (Supplementary Table S1). Furthermore, this review has highlighted the distinct lack of studies investigating PPF nets, particularly with appropriate assays for sterility. The variability in findings even in neighbouring countries raises intriguing questions and underscores the complexity of malaria control on the continent. The diverse performance outcomes suggest that the efficacy of these interventions is influenced by a multitude of factors, including local vector species, ecological conditions, pre-existing levels of pyrethroid resistance, and with housing style also important in East vs. West Africa.
Despite the variation in outcomes, this review highlights that ngITNs consistently outperformed pITNs; this not only underlines the importance of the strategic shift toward dual active ingredient formulations but also emphasises the urgent need for their widespread adoption. The enhanced efficacy of these nets may be attributed to the additive action of the second AI, countering the widespread pyrethroid resistance observed in mosquito populations. A cautionary note on the use of these chemistries is highlighted in (Menze et al., 2022) with a negative association between mortality and the presence of the kdr-w mutation with chlorfenapyr, potentially indicating reduced efficacy in certain areas of increased pyrethroid resistance. This study highlights the necessity to consistently monitor the efficacy of these tools in different settings, with a specific emphasis on tracking resistance to new chemistries. The availability of multiple tools in vector control and the emergence of insecticide resistance underline the importance of utilising integrated vector control as a form of resistance mitigation.
Author contributions
VI: Writing – review & editing, Writing – original draft, Visualization, Validation, Supervision, Project administration, Funding acquisition, Conceptualization. AB: Writing – review & editing, Writing – original draft, Methodology, Formal analysis, Data curation. RL: Writing – review & editing, Writing – original draft, Methodology, Investigation, Formal analysis, Data curation. NP: Writing – review & editing, Writing – original draft, Methodology, Investigation, Formal analysis, Data curation. JH: Writing – review & editing, Writing – original draft, Investigation.
Funding
The author(s) declare financial support was received for the research, authorship, and/or publication of this article. This review was funded by the Deutsches Zentrum für Infektionsforschung (DZIF, TTU03.705), the Deutsche Forschungsgemeinschaft (DFG, German Research Foundation) with project number 240245660-SFB 1129, the ERC Starting Grant (101075634, ReMVeC), and the Bill & Melinda Gates Foundation (INV-050587). For the publication fee, we acknowledge financial support by the Deutsche Forschungsgemeinschaft within the funding programme “Open Access Publikationskosten” and by Heidelberg University.
Conflict of interest
The authors declare that the research was conducted in the absence of any commercial or financial relationships that could be construed as a potential conflict of interest.
Publisher’s note
All claims expressed in this article are solely those of the authors and do not necessarily represent those of their affiliated organizations, or those of the publisher, the editors and the reviewers. Any product that may be evaluated in this article, or claim that may be made by its manufacturer, is not guaranteed or endorsed by the publisher.
Supplementary material
The Supplementary Material for this article can be found online at: https://www.frontiersin.org/articles/10.3389/fmala.2024.1337572/full#supplementary-material
References
Accrombessi M., Cook J., Dangbenon E., Yovogan B., Akpovi H., Sovi A., et al. (2023). Efficacy of pyriproxyfen-pyrethroid long-lasting insecticidal nets (LLINs) and chlorfenapyr-pyrethroid LLINs compared with pyrethroid-only LLINs for malaria control in Benin: a cluster-randomised, superiority trial. Lancet. 401, 435–446. doi: 10.1016/S0140-6736(22)02319-4
Allossogbe M., Gnanguenon V., Yovogan B., Akinro B., Anagonou R., Agossa F., et al. (2017). WHO cone bio-assays of classical and new-generation long-lasting insecticidal nets call for innovative insecticides targeting the knock-down resistance mechanism in Benin. Malar J. 16, 77. doi: 10.1186/s12936-017-1727-x
Anon (1995). AC 303,630 insecticide–miticide (American Cyanamid Company), 1–20. Available at: https://archive.epa.gov/pesticides/chemicalsearch/chemical/foia/web/pdf/129093/129093-006.pdf.
Awolola S. T., Adeogun A. O., Olojede J. B., Oduola A. O., Oyewole I. O., Amajoh C. N. (2014). Impact of PermaNet 3.0 on entomological indices in an area of pyrethroid resistant Anopheles Gambiae in south-western Nigeria. Parasites Vectors 7, 236. doi: 10.1186/1756-3305-7-236
Balabanidou V., Kampouraki A., MacLean M., Blomquist G. J., Tittiger C., Juárez M. P., et al. (2016). Cytochrome P450 associated with insecticide resistance catalyzes cuticular hydrocarbon production in Anopheles Gambiae. Proc. Natl. Acad. Sci. U S A 113, 9268–9273. doi: 10.1073/pnas.1608295113
Barreaux P., Koella J. C., N’Guessan R., Thomas M. B. (2022). Use of novel lab assays to examine the effect of pyrethroid-treated bed nets on blood-feeding success and longevity of highly insecticide-resistant Anopheles Gambiae s.l. mosquitoes. Parasit Vectors 15, 111. doi: 10.1186/s13071-022-05220-y
Bayili K., N’do S., Namountougou M., Sanou R., Ouattara A., Dabiré R. K., et al. (2017). Evaluation of efficacy of Interceptor® G2, a long-lasting insecticide net coated with a mixture of chlorfenapyr and alpha-cypermethrin, against pyrethroid resistant Anopheles Gambiae s.l. in Burkina Faso. Malaria J. 16, 190. doi: 10.1186/s12936-017-1846-4
Bhatt S., Weiss D. J., Cameron E., Bisanzio D., Mappin B., Dalrymple U., et al. (2015). The effect of malaria control on Plasmodium falciparum in Africa between 2000 and 2015. Nature 526, 7572, 207–211. doi: 10.1038/nature15535
Camara S., Ahoua Alou L. P., Koffi A. A., Clegban Y. C. M., Kabran J. P., Koffi F. M., et al. (2018). Efficacy of Interceptor(®) G2, a new long-lasting insecticidal net against wild pyrethroid-resistant Anopheles Gambiae s.s. from Côte d’Ivoire: a semi-field trial. Parasite 25, 42. doi: 10.1051/parasite/2018042
Challenger J. D., Nash R. K., Ngufor C., Sanou A., Toe K. H., Moore S., et al. (2023). Assessing the variability in experimental hut trials evaluating insecticide-treated nets against malaria vectors. Curr. Res. Parasitol. Vector Borne Dis. 3, 100115. doi: 10.1016/j.crpvbd.2023.100115
Cisse M. B. M., Sangare D., Oxborough R. M., Dicko A., Dengela D., Sadou A., et al. (2017). A village level cluster-randomized entomological evaluation of combination long-lasting insecticidal nets containing pyrethroid plus PBO synergist in Southern Mali. Malar J. 16, 477. doi: 10.1186/s12936-017-2124-1
Corbel V., Chabi J., Dabiré R. K., Etang J., Nwane P., Pigeon O., et al. (2010). Field efficacy of a new mosaic long-lasting mosquito net (PermaNet® 3.0) against pyrethroid-resistant malaria vectors: a multi centre study in Western and Central Africa. Malaria J. 9, 113. doi: 10.1186/1475-2875-9-113
Dhadialla T. S., Carlson G. R., Le D. P. (1998). New insecticides with ecdysteroidal and juvenile hormone activity. Annu. Rev. Entomol. 43, 545–569. doi: 10.1146/annurev.ento.43.1.545
Gansané A., Gogue C., Guelbeogo W. M., Debe S., Kagone M., Kinda R., et al. (2023). The effectiveness of dual-active ingredient insecticide treated bed nets at reducing malaria transmission in Burkina Faso: Results from the New Nets Project pilot evaluation observational analyses (Research Square). doi: 10.21203/rs.3.rs-3256536/v1
Gichuki P. M., Kamau L., Njagi K., Karoki S., Muigai N., Matoke-Muhia D., et al. (2021). Bioefficacy and durability of Olyset® Plus, a permethrin and piperonyl butoxide-treated insecticidal net in a 3-year long trial in Kenya. Infect. Dis. Poverty 10. doi: 10.1186/s40249-021-00916-2
Hancock P. A., Lynd A., Wiebe A., Devine M., Essandoh J., Wat’Senga F., et al. (2022). Modelling spatiotemporal trends in the frequency of genetic mutations conferring insecticide target-site resistance in African mosquito malaria vector species. BMC Biol. 20. doi: 10.1186/s12915-022-01242-1
Ibrahim S. S., Mukhtar M. M., Irving H., Riveron J. M., Fadel A. N., Tchapga W., et al. (2020). Exploring the mechanisms of multiple insecticide resistance in a highly plasmodium-infected malaria vector anopheles funestus sensu stricto from sahel of northern Nigeria. Genes (Basel) 11. doi: 10.3390/genes11040454
Ingham V. A., Grigoraki L., Ranson H. (2023). Pyrethroid resistance mechanisms in the major malaria vector species complex. Entomologia Generalis. 43, 515–526. doi: 10.1127/entomologia/2023/1880
Itoh T., Kawada H., Abe A., Eshita Y., Rongsriyam Y., Igarashi A. (1994). Utilization of bloodfed females of Aedes aEgypti as a vehicle for the transfer of the insect growth regulator pyriproxyfen to larval habitats. J. Am. Mosq. Control Assoc. 10, 344–347.
Ketoh G. K., Ahadji-Dabla K. M., Chabi J., Amoudji A. D., Apetogbo G. Y., Awokou F., et al. (2018). Efficacy of two PBO long lasting insecticidal nets against natural populations of Anopheles Gambiae s.l. in experimental huts, Kolokope, Togo. PloS One 13, e0192492. doi: 10.1371/journal.pone.0192492
Kibondo U. A., Odufuwa O. G., Ngonyani S. H., Mpelepele A. B., Matanilla I., Ngonyani H., et al. (2022). Influence of testing modality on bioefficacy for the evaluation of Interceptor. Parasit Vectors 15, 124. doi: 10.1186/s13071-022-05207-9
Koudou B. G., Koffi A. A., Malone D., Hemingway J. (2011). Efficacy of PermaNet® 2.0 and PermaNet® 3.0 against insecticide-resistant Anopheles Gambiae in experimental huts in Côte d’Ivoire. Malaria J. 10, 172. doi: 10.1186/1475-2875-10-172
Kweka E. J., Lyaruu L. J., Mahande A. M. (2017). Efficacy of PermaNet(R) 3.0 and PermaNet(R) 2.0 nets against laboratory-reared and wild Anopheles Gambiae sensu lato populations in northern Tanzania. Infect. Dis. Poverty 6, 11. doi: 10.1186/s40249-016-0220-z
Mahande A. M., Msangi S., Lyaruu L. J., Kweka E. J. (2018). Bio-efficacy of DuraNet(R) long-lasting insecticidal nets against wild populations of Anopheles arabiensis in experimental huts. Trop. Med. Health 46, 36. doi: 10.1186/s41182-018-0118-5
Martinez-Torres D., Chandre F., Williamson M. S., Darriet F., Bergé J. B., Devonshire A. L., et al. (1998). Molecular characterization of pyrethroid knockdown resistance (kdr) in the major malaria vector Anopheles Gambiae s.s. Insect Mol. Biol. 7, 179–184. doi: 10.1046/j.1365-2583.1998.72062.x
Mechan F., Katureebe A., Tuhaise V., Mugote M., Oruni A., Onyige I., et al. (2022). LLIN evaluation in Uganda project (LLINEUP): The fabric integrity, chemical content and bioefficacy of long-lasting insecticidal nets treated with and without piperonyl butoxide across two years of operational use in Uganda. Curr. Res. Parasitol. Vector Borne Dis. 2, 100092. doi: 10.1016/j.crpvbd.2022.100092
Menze B. D., Kouamo M. F., Wondji M. J., Tchapga W., Tchoupo M., Kusimo M. O., et al. (2020). An Experimental Hut Evaluation of PBO-Based and Pyrethroid-Only Nets against the Malaria Vector Anopheles funestus Reveals a Loss of Bed Nets Efficacy Associated with GSTe2 Metabolic Resistance. Genes (Basel) 11. doi: 10.3390/genes11020143
Menze B. D., Mugenzi L. M. J., Tchouakui M., Wondji M. J., Tchoupo M., Wondji C. S. (2022). Experimental Hut Trials Reveal That CYP6P9a/b P450 Alleles Are Reducing the Efficacy of Pyrethroid-Only Olyset Net against the Malaria Vector Anopheles funestus but PBO-Based Olyset Plus Net Remains Effective. Pathogens. 11. doi: 10.3390/pathogens11060638
Metcalf R. L. (1967). Mode of action of insecticide synergists. Annu. Rev. Entomol. 12, 229–256. doi: 10.1146/annurev.en.12.010167.001305
Mosha J. F., Kulkarni M. A., Lukole E., Matowo N. S., Pitt C., Messenger L. A., et al. (2022). Effectiveness and cost-effectiveness against malaria of three types of dual-active-ingredient long-lasting insecticidal nets (LLINs) compared with pyrethroid-only LLINs in Tanzania: a four-arm, cluster-randomised trial. Lancet. 399, 1227–1241. doi: 10.1016/S0140-6736(21)02499-5
Mosha J. F., Matowo N. S., Kulkarni M. A., Messenger L. A., Lukole E., Mallya E., et al. (2023). Effectiveness of long-lasting insecticidal nets with pyriproxyfen-pyrethroid, chlorfenapyr-pyrethroid, or piperonyl butoxide-pyrethroid versus pyrethroid only against malaria in Tanzania: final-year results of a four-arm, single-blind, cluster-randomised trial. Lancet Infect. Dis. 24, 87–97. doi: 10.1016/S1473-3099(23)00420-6
N’Guessan R., Odjo A., Ngufor C., Malone D., Rowland M. (2016). A chlorfenapyr mixture net interceptor® G2 shows high efficacy and wash durability against resistant mosquitoes in west africa. PloS One 11, e0165925. doi: 10.1371/journal.pone.0165925
Ngufor C., Fagbohoun J., Agbevo A., Ismail H., Challenger J. D., Churcher T. S., et al. (2022). Comparative efficacy of two pyrethroid-piperonyl butoxide nets (Olyset Plus and PermaNet 3.0) against pyrethroid resistant malaria vectors: a non-inferiority assessment. Malaria J. 21. doi: 10.1186/s12936-022-04041-9
Okia M., Ndyomugyenyi R., Kirunda J., Byaruhanga A., Adibaku S., Lwamafa D. K., et al. (2013). Bioefficacy of long-lasting insecticidal nets against pyrethroid-resistant populations of Anopheles Gambiae s.s. from different malaria transmission zones in Uganda. Parasites Vectors 6, 130. doi: 10.1186/1756-3305-6-130
Omondi S., Mukabana W. R., Ochomo E., Muchoki M., Kemei B., Mbogo C., et al. (2017). Quantifying the intensity of permethrin insecticide resistance in Anopheles mosquitoes in western Kenya. Parasit Vectors 10, 548. doi: 10.1186/s13071-017-2489-6
World Health Organization (2023). WHO guidelines for malaria, 16 October 2023 (Geneva: World Health Organization).
Oumbouke W. A., Rowland M., Koffi A. A., Alou L. P. A., Camara S., N’Guessan R. (2019). Evaluation of an alpha-cypermethrin + PBO mixture long-lasting insecticidal net VEERALIN(R) LN against pyrethroid resistant Anopheles Gambiae s.s.: an experimental hut trial in M’be, central Cote d’Ivoire. Parasit Vectors 12, 544. doi: 10.1186/s13071-019-3796-x
Pennetier C., Bouraima A., Chandre F., Piameu M., Etang J., Rossignol M., et al. (2013). Efficacy of Olyset(R) Plus, a new long-lasting insecticidal net incorporating permethrin and piperonyl-butoxide against multi-resistant malaria vectors [corrected]. PloS One 8, e75134. doi: 10.1371/annotation/bed4305a-d665-4150-a682-a20d9cf9b79f
Global Malaria Programme (GMP) (2021). Insecticide-treated nets for malaria transmission control in areas with insecticide-resistant mosquito populations. Available at: https://www.who.int/publications/i/item/9789240018730
Protopopoff N., Mosha J. F., Messenger L. A., Lukole E., Charlwood J. D., Wright A., et al. (2023). Effectiveness of piperonyl butoxide and pyrethroid-treated long-lasting insecticidal nets (LLINs) versus pyrethroid-only LLINs with and without indoor residual spray against malaria infection: third year results of a cluster, randomised controlled, two-by. Malaria J. 22. doi: 10.1186/s12936-023-04727-8
Pwalia R., Joannides J., Iddrisu A., Addae C., Acquah-Baidoo D., Obuobi D., et al. (2019). High insecticide resistance intensity of Anopheles Gambiae (s.l.) and low efficacy of pyrethroid LLINs in Accra, Ghana. Parasit Vectors 12, 299.
Sherrard-Smith E., Ngufor C., Sanou A., Guelbeogo M. W., N’Guessan R., Elobolobo E., et al. (2022). Inferring the epidemiological benefit of indoor vector control interventions against malaria from mosquito data. Nat. Commun. 13, 3862. doi: 10.1038/s41467-022-30700-1
Staedke S. G., Gonahasa S., Dorsey G., Kamya M. R., Maiteki-Sebuguzi C., Lynd A., et al. (2020). Effect of long-lasting insecticidal nets with and without piperonyl butoxide on malaria indicators in Uganda (LLINEUP): a pragmatic, cluster-randomised trial embedded in a national LLIN distribution campaign. Lancet. 395, 1292–1303. doi: 10.1016/S0140-6736(20)30214-2
Syme T., N’Dombidje B., Gbegbo M., Todjinou D., Ariori V., De Vos P., et al. (2023b). PermaNet Dual, a new deltamethrin-chlorfenapyr mixture net, shows improved efficacy against pyrethroid-resistant Anopheles Gambiae sensu lato in southern Benin. Sci. Rep. 13, 12232. doi: 10.1038/s41598-023-39140-3
Syme T., Nounagnon J., N’Dombidjé B., Gbegbo M., Agbevo A., Ahoga J., et al. (2023a). Can the performance of pyrethroid-chlorfenapyr nets be reduced when combined with pyrethroid-piperonyl butoxide (PBO) nets? Malaria J. 22. doi: 10.1186/s12936-023-04648-6
Tchakounte A., Tchouakui M., Mu-Chun C., Tchapga W., Kopia E., Soh P. T., et al. (2019). Exposure to the insecticide-treated bednet PermaNet 2.0 reduces the longevity of the wild African malaria vector Anopheles funestus but GSTe2-resistant mosquitoes live longer. PloS One 14, e0213949.
Tchouakui M., Thiomela R. F., Nchoutpouen E., Menze B. D., Ndo C., Achu D., et al. (2023). High efficacy of chlorfenapyr-based net Interceptor((R)) G2 against pyrethroid-resistant malaria vectors from Cameroon. Infect. Dis. Poverty 12, 81. doi: 10.1186/s40249-023-01132-w
Thierry Bobanga W. A., Zanga M., Umesumbu S., Landela A., Fataki O., Mandoko A. S., et al. (2013). Field efficacy and acceptability of PermaNet® 3.0 and OlysetNet® in Kinshasa, Democratic Republic of the Congo. J. Vector Borne Diseases 50, 206–214.
Tungu P. K., Michael E., Sudi W., Kisinza W. W., Rowland M. (2021a). Efficacy of interceptor® G2, a long-lasting insecticide mixture net treated with chlorfenapyr and alpha-cypermethrin against Anopheles funestus: experimental hut trials in north-eastern Tanzania. Malar J. 20, 180. doi: 10.1186/s12936-021-03716-z
Tungu P. K., Waweru J., Karthi S., Wangai J., Kweka E. J. (2021b). Field evaluation of Veeralin, an alpha-cypermethrin + PBO long-lasting insecticidal net, against natural populations of Anopheles funestus in experimental huts in Muheza, Tanzania. Curr. Res. Parasitol. Vector Borne Dis. 1, 100030. doi: 10.1016/j.crpvbd.2021.100030
Weill M., Malcolm C., Chandre F., Mogensen K., Berthomieu A., Marquine M., et al. (2004). The unique mutation in ace-1 giving high insecticide resistance is easily detectable in mosquito vectors. Insect Mol. Biol. 13, 1–7. doi: 10.1111/j.1365-2583.2004.00452.x
Yewhalaw D., Asale A., Tushune K., Getachew Y., Duchateau L., Speybroeck N. (2012). Bio-efficacy of selected long-lasting insecticidal nets against pyrethroid resistant Anopheles arabiensis from South-Western Ethiopia. Parasites Vectors 5, 159. doi: 10.1186/1756-3305-5-159
Zahouli J. Z. B., Edi C. A. V., Yao L. A., Lisro E. G., Adou M., Kone I., et al. (2023). Small-scale field evaluation of PermaNet((R)) Dual (a long-lasting net coated with a mixture of chlorfenapyr and deltamethrin) against pyrethroid-resistant Anopheles Gambiae mosquitoes from Tiassale, Cote d’Ivoire. Malar J. 22, 36. doi: 10.1186/s12936-023-04455-z
Zoungbedji D. M., Padonou G. G., Konkon A. K., Hougbe S., Sagbohan H., Kpanou C., et al. (2023). Assessing the susceptibility and efficacy of traditional neurotoxic (pyrethroid) and new-generation insecticides (chlorfenapyr, clothianidin, and pyriproxyfen), on wild pyrethroid-resistant populations of Anopheles Gambiae from southern Benin. Malar J. 22, 245. doi: 10.1186/s12936-023-04664-6
Keywords: insecticides, bed nets, chlorfenapyr, pyriproxyfen, piperonyl butoxide, pyrethroid, malaria, Anopheles
Citation: Böhmert AL, Logan RAE, Portwood NM, Hartke J and Ingham VA (2024) A descriptive review of next-generation insecticide-treated bed nets for malaria control. Front. Malar. 2:1337572. doi: 10.3389/fmala.2024.1337572
Received: 13 November 2023; Accepted: 26 April 2024;
Published: 28 May 2024.
Edited by:
Sarah Reece, University of Edinburgh, United KingdomReviewed by:
Rosemary Lees, Liverpool School of Tropical Medicine, United KingdomEllie Sherrard-Smith, Imperial College London, United Kingdom
Copyright © 2024 Böhmert, Logan, Portwood, Hartke and Ingham. This is an open-access article distributed under the terms of the Creative Commons Attribution License (CC BY). The use, distribution or reproduction in other forums is permitted, provided the original author(s) and the copyright owner(s) are credited and that the original publication in this journal is cited, in accordance with accepted academic practice. No use, distribution or reproduction is permitted which does not comply with these terms.
*Correspondence: Victoria A. Ingham, Victoria.ingham@uni-heidelberg.de
†These authors have contributed equally to this work and share first authorship