- 1Department of Clinical and Experimental Medicine, University of Pisa, Pisa, Italy
- 2Department of Surgical, Medical and Molecular Pathology and of Critical Care Medicine, University of Pisa, Pisa, Italy
- 3Department of Educational Sciences, University of Genoa, Genoa, Italy
Introduction
Due to its distinct and widely recognizable pattern of face expression, anger has always been included in the repertoire of basic emotions (Ekman, 1999). Relying on polyvagal theory, Beauchaine et al. (2007) summarized the results of three studies (Beauchaine, 2001; Mead et al., 2004; Crowell et al., 2006) evaluating autonomic nervous system functioning in children manifesting aggression and conduct problems, aged 4–18. Children with aggressive oppositional defiant disorder or conduct disorder exhibited both sympathetic hypo-arousal at baseline and sympathetic insensitivity to reward at a very early age, marking a general disinhibitory tendency. In addition to this disinhibition, PNS deficiencies were found and contributed to increased emotional lability. Using transcutaneous vagus nerve stimulation (tVNS), Steenbergen et al. (2021), investigating subjects with age ranging from 18 to 28, found that active tVNS, compared to sham stimulation, enhanced the recognition of anger but reduced the ability to recognize sadness.
According to developmental research, an actual expression of anger does not emerge until the last months of the first year of life (Sroufe, 1996). According to this, research on 5-, 12-, and 15-month-old infants has shown that an adult-like, late, non-linear pattern of cortical response to masked faces at various levels of visibility emerged as early as 5 months of age, starting around 900 ms, possibly due to the development of the right fusiform gyrus (Guy et al., 2016) and its increased sensitivity to fearful faces from 5 to 12 months (Xie et al., 2019; Chen et al., 2021). Subsequently, this late component shifted to a more sustained and faster response in older infants (~750 ms), to reach around 300 ms in adults (Kouider et al., 2013). Consequently, in infants aged 5–12 months exposed to facial expressions of happiness, fear, and anger with normal levels of visibility, the N290 event-related potential (ERP) component was found to be larger in amplitude in response to angry and happy faces than to angry ones, revealing greater cortical activation in the right fusiform face area, while the P400 and the negative-central (Nc) ERP components were found to be larger in amplitude in response to angry faces than to happy and fearful ones, revealing greater activation of the posterior cingulate cortex (PCC)/precuneus associated with the allocation of infants' attention. Interestingly, these effects emerged at 5 months, became well established at 7 months, and then disappeared at 12 months (Xie et al., 2019; Chen et al., 2021). As extensively shown for sensory development (Berardi et al., 2003; Hübener and Bonhoeffer, 2014; Ribot et al., 2021), this evidence may suggest a sensitive period for emotional development (Woodard and Pollak, 2020) related, in particular, to the learning of safety (Porges, 2015, 2022).
Hierarchical emotional responses: A polyvagal perspective on anger
It is possibly throughout the process of the learning of safety that newborns may become aware that their intentional actions can actually be blocked by internal or external factors, so a definite anger reaction may emerge (Williams, 2017). Internal factors, such as self-disgust, self-criticism (Zahn et al., 2015), and shame proneness have been found to be associated with anger suppression and conflict avoidance (Cheung and Park, 2010) as well as with subsequent anger outbursts (Alia-Klein et al., 2020). In addition, structural equation modeling showed that self-criticism predicted shame and guilt, which, in turn, predicted problematic dysfunctional outcomes such as addiction and problematic behavior (Sassover et al., 2021; Snoek et al., 2021). It has been proposed that anger may be an emotion supporting goal-directed behavior when an environmental situation prevents the desired goal to be fulfilled, causing frustration (Panksepp, 2005). In addition, anger may function as a protection from an external predatory attack. In this case, anger may emerge as a possible consequence of fear (Wilkowski and Robinson, 2010).
According to the polyvagal theory, the structural organization and function of the human autonomic nervous system are hierarchically based on its phylogenetic heritage (Porges, 2007, 2009, 2022). The myelinated ventral vagal complex (VVC), whose cardioinhibitory fibers emerge from the nucleus ambiguus (NA) in the brainstem, is the source of the social engagement system (SES). Due to its myelinated fibers, the VVC is the least homeostatically disruptive, phylogenetically youngest, and fastest responding challenge-response system. The sympathetic nervous system (SNS) is phylogenetically older than the VVC, and its activation promotes increased heart rate, breathing, and mobilized behaviors in order to implement active responses to threats such as escape or defensive confrontation. The Dorsal Vagal Complex (DVC) is the phylogenetically oldest autonomic subsystem, with unmyelinated cardioinhibitory fibers originating in the dorsal motor nucleus (DMNX) of the vagus in the brainstem. It also has a vestigial immobilization function that initially emerged in early vertebrates (Porges, 2021a). The DVC innervates organs below the diaphragm and is involved in both homeostatic and threat reactions. When recruited during threat responses, this complex also disrupts digestion and conserves metabolic resources (Porges, 2007, 2009). Interestingly, the DVC is also the system that is primarily involved in post-traumatic responses after psychological trauma (Kolacz and Porges, 2018; Kolacz et al., 2019).
Polyvagal theory posits that the evolution of the mammalian social engagement system (SES) was aided by the integration of the myelinated cardiac vagal pathways with neural regulation of the face and head. Early atypical coordination of this system is a predictor of following social behavior and emotional control issues. The brain evaluation of environmental danger determines the preferential recruitability of the SES or the sequential hierarchical recruitment of the SNS or the DVC. According to polyvagal theory, the neural evaluation of risk is achieved through neuroception, a neural reflexive mechanism, which is distinct from perception and is capable of instantly shifting the physiological state and of distinguishing safe, dangerous, or life-threatening environmental and visceral features. A neuroception of safety supports the SES in safe contexts, and the autonomic state is adaptively adjusted to decrease SNS activity and protect the oxygen-dependent central nervous system, particularly the cortex, from the metabolically conservative reactions of the DVC (e.g., fainting). Alternatively, a neuroception of danger, or life threat, favors the recruitment of SNS or DVC (Porges, 2003, 2007, 2009, 2021b).
Interestingly, recent evidence investigating the maturation of cardiac autonomic nervous system activity in children and adolescents showed that the cardiac parasympathetic nervous system (PNS) and SNS activity in childhood follow different maturational trajectories. In particular, in the first months of life, an increase in heart rate variability (HRV) and in the number of myelinated fibers in the vagus nerve has been reported. Specifically, HRV increases steadily from the first months to early and middle childhood, then plateaus in late childhood/early adolescence (~age 11) and does not fluctuate or decrease during adolescence, while respiratory sinus arrhythmia (RSA) peaked between 7 and 8 years old. Regarding SNS development, it was found that the mean pre-ejection period showed a distinct maturational effect, as it increases linearly from early childhood to late adolescence, thus implying that SNS activity declines with age. Overall, these results suggest that PNS activity increases rapidly after birth, plateaus in middle childhood, and then declines at the end of adolescence, while SNS shows a steady decline over time (Harteveld et al., 2021). More specifically, the developmental pattern of myelinated and unmyelinated fibers of the human vagus nerve in term infants has long been known. A significant increase was found in the average content of myelin in myelinated fibers, indicating active myelination during the first 9 months of life without a significant change in fiber axonal properties. The density of myelinated fibers increases with age, both in terms of total quantity and in respect to unmyelinated fibers, implying a steady transition from unmyelinated to myelinated axons during the first year of life, notably during the first 3 months (Pereyra et al., 1992). Furthermore, in victims of sudden infant death syndrome (SIDS) between 1 and 9 months of age, more small and fewer large myelinated vagal fibers were detected, compared to unmyelinated fibers, in SIDS than in controls, implying an immature condition of the vagus nerve in SIDS (Becker et al., 1993).
A critical period for experience-dependent development of the feelings of safety during early infancy
Taken together, the results reviewed in this work indicate that at birth, regarding PNS, an increased unmyelinated-to-myelinated fibers ratio (UMR) may be prevalent, suggesting an increased probability of DVC states. Therefore, the subsequent need to experience a neuroception of safety for the infant may be the neurophysiological underpinning of human attachment (Feldman, 2017). Experiencing safety can promote the maturation of vagus' myelinated fibers and the transition from unmyelinated to myelinated axons, decreasing UMR, during the first year of life as a critical period (Woodard and Pollak, 2020). Accordingly, considering the increased UMR of the PNS during the first 3 months of life (Pereyra et al., 1992), it could be hypothesized that it may represent a sensitive period for early environmental safety detection through the DVC, in order to possibly establish a baseline threshold for threat detection and for the emergence of fear. Possibly, contingent experience of safety may promote fiber myelination, the decrease of UMR of PNS, and the emergence of VVC, through a process of activity-dependent myelination (Fields, 2015; Noori et al., 2020) that may operate during the critical period, but also throughout life (Faria et al., 2019). On the contrary, experiencing unsafety may prevent the decrease of UMR of PNS, potentially promoting SIDS (Becker et al., 1993), and the linear age-dependent increase in PEP, which allows for a decrease in the cardiac effects of SNS activity with age (Harteveld et al., 2021). Therefore, in adulthood, after closure of the critical period, unsafety detection in the environment may preferentially promote the emergence of mobilizing sympathetic self-protective strategies related to anger to avoid the prevalence of immobilizing DVC self-protective strategies related to fear (Figure 1). Interestingly, patients with panic disorder were characterized by relative reactive sympathetic dominance in response to mental stress compared to healthy controls (Kotianova et al., 2018).
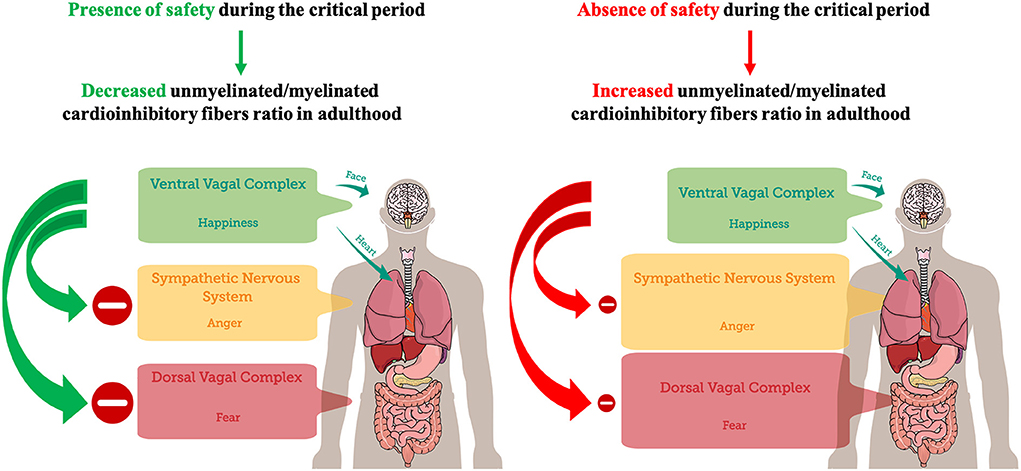
Figure 1. A postnatal critical period for the development of feelings of safety. The presence of safety during the critical period (the first year of life) may lead to a decreased unmyelinated/myelinated cardioinhibitory fibers ratio in adulthood, promoting VVC development, adaptive inhibition of SNS and DVC, and emotional self-regulation. Conversely, the absence of safety during the critical period may lead to an increased unmyelinated/myelinated cardioinhibitory fibers ratio in adulthood, dampening VVC development. Dampened VVC activity reduces the capacity of adaptive inhibition of SNS and DVC, and emotional self-regulation. Hence, environmental detection of unsafety cues may preferentially trigger SNS-mediated anger in order to avoid DVC-mediated immobilization with fear.
Consequently, the right fusiform face area, related to the N290 ERP component, found to be larger in amplitude in response to fearful and happy faces than to angry faces (Xie et al., 2019), has also been found to be associated with HRV and PNS (Critchley et al., 2003). Therefore, if safety is detected, the activity of myelinated VVC and the emotion of happiness can be promoted; conversely, if unsafety is detected the activity of unmyelinated DVC and the emotion of fear can be promoted. In order to avoid the immediate predominance of immobilizing DVC self-protective strategies, anger may be recruited right after unsafety detection. Consistent with this, the PCC/precuneus were related to the P400 and the Nc ERP components, and found to be larger in response to angry faces than to happy and fearful faces (Xie et al., 2019). Furthermore, PCC/precuneus, which are brain areas belonging to the default mode network that is typically associated with parasympathetic functions (Beissner et al., 2013), were found to be significantly involved in SNS regulation (Taylor et al., 2016). This finding may explain the evidence related to the reciprocally coupled activation of PNS and SNS during both rest and acute stress (Weissman and Mendes, 2021). Interestingly, individuals with higher baseline RSA, an index of VVC, showed more reciprocal coupling than those with lower baseline RSA, reflecting greater coordination of physiological response (Weissman and Mendes, 2021). Furthermore, the disappearance of these effects at 12 months (Xie et al., 2019), possibly upon completion of UMR increase (Pereyra et al., 1992), may implement what has been learnt during the critical period for safety learning in the instinctively coupled activation of PNS and SNS. In accordance with these observations, regarding interpersonal violence it was found that perpetrators of intimate partner violence showed higher heart rate and skin conductance levels, shorter PEP, and lower RSA values during recovery from stress, as well as more anger and worse mood after stress (Romero-Martínez et al., 2021). Furthermore, individuals who exhibited longer cardiac PEP at rest and reduced PEP reactivity scored higher on measures of behavioral problems and aggression, while individuals who exhibited lower baseline RSA and greater RSA withdrawal scored lower on prosocial behavior and emotion regulation (Beauchaine et al., 2013). Consequently, RSA has been proposed as a transdiagnostic biomarker of emotion regulation and psychopathology (Beauchaine, 2015; Beauchaine and Bell, 2020).
Neurophysiologically-informed psychometric tools to assess perceived safety and future directions
Since traumatic symptoms arise from unregulated threat preoccupation, when self-regulation is not available (Motsan et al., 2021), assessing safety through the use of psychometric tools that show sound psychometric properties, and that have been rooted in the organization of the autonomic nervous system, is recommended. Considering the importance of safety evaluation within a therapeutic context, recent research has developed new tools to assess feelings of safety (see, e.g., Morton et al., 2021; Poli et al., 2021.
Future research should focus on interventions promoting safety. Maternal-newborn skin-to-skin contact may be an early essential factor needed to promote the development of the feelings of safety and the organization of the infant's physiological systems, including stress reactivity, autonomic functioning, and sleep patterns (Feldman et al., 2002). Skin-to-skin contact was able to increase autonomic functioning (measured by RSA) and enhanced child cognitive development and executive functions from 6 months to 10 years. By 10 years of age, children receiving skin-to-skin contact showed attenuated stress response, improved RSA, organized sleep, and better cognitive control (Feldman et al., 2014). In accordance with this, regarding medial prefrontal cortex (mPFC), in mice it has been shown that chronic maternal separation (MS) during the first 2 weeks of postnatal life led to a depletion of the oligodendrocyte progenitor pool in MS adults that was associated to pro-depressive effects and short-term memory impairment. Conversely, chemogenetic neuronal activation normalized oligodendrocyte progenitor pool in MS animals prevented the pro-depressive effects and short-term memory impairment of MS, identifying neuronal activity as a critical intervention for early-life stress and to promote mPFC-related behaviors (Teissier et al., 2020).
Author contributions
AP: conceptualization and writing—original draft preparation. AP and MM: methodology, validation, investigation, resources, data curation, supervision, and project administration. AP, AG, CC, and MM: writing—review and editing. All authors have read and agreed to the published version of the manuscript.
Conflict of interest
The authors declare that the research was conducted in the absence of any commercial or financial relationships that could be construed as a potential conflict of interest.
Publisher's note
All claims expressed in this article are solely those of the authors and do not necessarily represent those of their affiliated organizations, or those of the publisher, the editors and the reviewers. Any product that may be evaluated in this article, or claim that may be made by its manufacturer, is not guaranteed or endorsed by the publisher.
References
Alia-Klein, N., Gan, G., Gilam, G., Bezek, J., Bruno, A., Denson, T. F., et al. (2020). The feeling of anger: from brain networks to linguistic expressions. Neurosci. Biobehav. Rev. 108, 480–497. doi: 10.1016/j.neubiorev.2019.12.002
Beauchaine, T. (2001). Vagal tone, development, and Gray's motivational theory: toward an integratedmodel of autonomic nervous system functioning in psychopathology. Dev. Psychopathol. 13, 183–214. doi: 10.1017/S0954579401002012
Beauchaine, T. P. (2015). Respiratory sinus arrhythmia: a transdiagnostic biomarker of emotion dysregulation and psychopathology. Curr. Opin. Psychol. 3, 43–47. doi: 10.1016/j.copsyc.2015.01.017
Beauchaine, T. P., and Bell, Z. E. (2020). “Respiratory sinus arrhythmia as a transdiagnostic biomarker of emotion dysregulation,” in The Oxford Handbook of Emotion Dysregulation, eds T. P. Beauchaine and S. E. Crowell (New York, NY: Oxford University Press), 152–165.
Beauchaine, T. P., Gatzke-Kopp, L., and Mead, H. K. (2007). Polyvagal theory and developmental psychopathology: emotion dysregulation and conduct problems from preschool to adolescence. Biol. Psychol. 74, 174–184. doi: 10.1016/j.biopsycho.2005.08.008
Beauchaine, T. P., Gatzke-Kopp, L., Neuhaus, E., Chipman, J., Reid, M. J., and Webster-Stratton, C. (2013). Sympathetic- and parasympathetic-linked cardiac function and prediction of externalizing behavior, emotion regulation, and prosocial behavior among preschoolers treated for ADHD. J. Consult. Clin. Psychol. 81, 481–493. doi: 10.1037/a0032302
Becker, L. E., Zhang, W., and Pereyra, P. M. (1993). Delayed maturation of the vagus nerve in sudden infant death syndrome. Acta Neuropathol. 86, 617–622. doi: 10.1007/BF00294301
Beissner, F., Meissner, K., Bar, K.-J., and Napadow, V. (2013). The autonomic brain: an activation likelihood estimation meta-analysis for central processing of autonomic function. J. Neurosci. 33, 10503–10511. doi: 10.1523/JNEUROSCI.1103-13.2013
Berardi, N., Pizzorusso, T., Ratto, G. M., and Maffei, L. (2003). Molecular basis of plasticity in the visual cortex. Trends Neurosci. 26, 369–378. doi: 10.1016/S0166-2236(03)00168-1
Chen, Y., Slinger, M., Edgar, J. C., Bloy, L., Kuschner, E. S., Kim, M., et al. (2021). Maturation of hemispheric specialization for face encoding during infancy and toddlerhood. Dev. Cogn. Neurosci. 48, 100918. doi: 10.1016/j.dcn.2021.100918
Cheung, R. Y. M., and Park, I. J. K. (2010). Anger suppression, interdependent self-construal, and depression among Asian American and European American college students. Cult. Divers. Ethnic Minor. Psychol. 16, 517–525. doi: 10.1037/a0020655
Critchley, H. D., Mathias, C. J., Josephs, O., O'Doherty, J., Zanini, S., Dewar, B., et al. (2003). Human cingulate cortex and autonomic control: converging neuroimaging and clinical evidence. Brain 126, 2139–2152. doi: 10.1093/brain/awg216
Crowell, S. E., Beauchaine, T. P., Gatzke-Kopp, L., Sylvers, P., Mead, H., and Chipman-Chacon, J. (2006). Autonomic correlates of attention-deficit/hyperactivity disorder and oppositional defiant disorder in preschool children. J. Abnorm. Psychol. 115, 174–178. doi: 10.1037/0021-843X.115.1.174
Ekman, P. (1999). “Basic emotions,” in Handbook of Cognition and Emotion, eds T. Dalgleish and M. J. Power (Chichester: John Wiley & Sons, Ltd) 45–60.
Faria, O., Gonsalvez, D. G., Nicholson, M., and Xiao, J. (2019). Activity-dependent central nervous system myelination throughout life. J. Neurochem. 148, 447–461. doi: 10.1111/jnc.14592
Feldman, R. (2017). The neurobiology of human attachments. Trends Cogn. Sci. 21, 80–99. doi: 10.1016/j.tics.2016.11.007
Feldman, R., Eidelman, A. I., Sirota, L., and Weller, A. (2002). Comparison of skin-to-skin (kangaroo) and traditional care: parenting outcomes and preterm infant development. Pediatrics 110, 16–26. doi: 10.1542/peds.110.1.16
Feldman, R., Rosenthal, Z., and Eidelman, A. I. (2014). Maternal-preterm skin-to-skin contact enhances child physiologic organization and cognitive control across the first 10 years of life. Biol. Psychiatry 75, 56–64. doi: 10.1016/j.biopsych.2013.08.012
Fields, R. D. (2015). A new mechanism of nervous system plasticity: activity-dependent myelination. Nat. Rev. Neurosci. 16, 756–767. doi: 10.1038/nrn4023
Guy, M. W., Zieber, N., and Richards, J. E. (2016). The cortical development of specialized face processing in infancy. Child Dev. 87, 1581–1600. doi: 10.1111/cdev.12543
Harteveld, L. M., Nederend, I., ten Harkel, A. D. J., Schutte, N. M., de Rooij, S. R., Vrijkotte, T. G. M., et al. (2021). Maturation of the cardiac autonomic nervous system activity in children and adolescents. JAHA 10, e017405. doi: 10.1161/JAHA.120.017405
Hübener, M., and Bonhoeffer, T. (2014). Neuronal plasticity: beyond the critical period. Cell 159, 727–737. doi: 10.1016/j.cell.2014.10.035
Kolacz, J., Kovacic, K. K., and Porges, S. W. (2019). Traumatic stress and the autonomic brain-gut connection in development: Polyvagal Theory as an integrative framework for psychosocial and gastrointestinal pathology. Dev. Psychobiol. 61, 796–809. doi: 10.1002/dev.21852
Kolacz, J., and Porges, S. W. (2018). Chronic diffuse pain and functional gastrointestinal disorders after traumatic stress: pathophysiology through a polyvagal perspective. Front. Med. 5, 145. doi: 10.3389/fmed.2018.00145
Kotianova, A., Kotian, M., Slepecky, M., Chupacova, M., Prasko, J., and Tonhajzerova, I. (2018). The differences between patients with panic disorder and healthy controls in psychophysiological stress profile. Neuropsychiatr. Dis. Treat. 14, 435–441. doi: 10.2147/NDT.S153005
Kouider, S., Stahlhut, C., Gelskov, S. V., Barbosa, L. S., Dutat, M., de Gardelle, V., et al. (2013). A neural marker of perceptual consciousness in infants. Science 340, 376–380. doi: 10.1126/science.1232509
Mead, H., Beauchaine, T., Brenner, S., Crowell, S., Gatzke-Kopp, L., and Marsh, P. (2004). “Autonomic response patterns to reward and negative mood induction among children with conduct disorder, depression, and both psychiatric conditions,” in Annual meeting of the Society for Psychophysiological Research; Santa Fe, NM.
Morton, L., Cogan, N., Kolacz, J., Nikolic, M., Calderwood, C., Bacon, T., et al. (2021). A new measure of feeling safe: developing psychometric properties of the neuroception of psychological safety scale (NPSS). Open Sci. Framework. doi: 10.31219/osf.io/tpe5j
Motsan, S., Bar-Kalifa, E., Yirmiya, K., and Feldman, R. (2021). Physiological and social synchrony as markers of PTSD and resilience following chronic early trauma. Depress. Anxiety 38, 89–99. doi: 10.1002/da.23106
Noori, R., Park, D., Griffiths, J. D., Bells, S., Frankland, P. W., Mabbott, D., et al. (2020). Activity-dependent myelination: A glial mechanism of oscillatory self-organization in large-scale brain networks. Proc. Natl. Acad. Sci. U. S. A. 117, 13227–13237. doi: 10.1073/pnas.1916646117
Panksepp, J. (2005). Affective Neuroscience: The Foundations of Human and Animal Emotions, New York, NY: Oxford University Press.
Pereyra, P. M., Zhang, W., Schmidt, M., and Becker, L. E. (1992). Development of myelinated and unmyelinated fibers of human vagus nerve during the first year of life. J. Neurol. Sci. 110, 107–113. doi: 10.1016/0022-510X(92)90016-E
Poli, A., Maremmani, A. G. I., Chiorri, C., Mazzoni, G.-P., Orr,ù, G., Kolacz, J., et al. (2021). Item reduction, psychometric and biometric properties of the italian version of the body perception questionnaire—short form (BPQ-SF): the BPQ-22. IJERPH 18, 3835. doi: 10.3390/ijerph18073835
Porges, S. W. (2003). Social engagement and attachment. Ann. N. Y. Acad. Sci. 1008, 31–47. doi: 10.1196/annals.1301.004
Porges, S. W. (2007). The polyvagal perspective. Biol. Psychol. 74, 116–143. doi: 10.1016/j.biopsycho.2006.06.009
Porges, S. W. (2009). The polyvagal theory: new insights into adaptive reactions of the autonomic nervous system. CCJM 76, S86–S90. doi: 10.3949/ccjm.76.s2.17
Porges, S. W. (2015). Making the world safe for our children: down-regulating defence and up-regulating social engagement to ‘optimise' the human experience. Children Australia 40, 114–123. doi: 10.1017/cha.2015.12
Porges, S. W. (2021a). Cardiac vagal tone: a neurophysiological mechanism that evolved in mammals to dampen threat reactions and promote sociality. World Psychiatry 20, 296–298. doi: 10.1002/wps.20871
Porges, S. W. (2021b). Polyvagal theory: a biobehavioral journey to sociality. Compreh. Psychoneuroendocrinol. 7, 100069. doi: 10.1016/j.cpnec.2021.100069
Porges, S. W. (2022). Polyvagal theory: a science of safety. Front. Integr. Neurosci. 16, 871227. doi: 10.3389/fnint.2022.871227
Ribot, J., Breton, R., Calvo, C.-F., Moulard, J., Ezan, P., Zapata, J., et al. (2021). Astrocytes close the mouse critical period for visual plasticity. Science 373, 77–81. doi: 10.1126/science.abf5273
Romero-Martínez, A., Lila, M., and Moya-Albiol, L. (2021). Sympathetic nervous system predominance in intimate partner violence perpetrators after coping with acute stress. J. Interpers. Violence 37, NP10148–NP10169. doi: 10.1177/0886260520985494
Sassover, E., Abrahamovitch, Z., Amsel, Y., Halle, D., Mishan, Y., Efrati, Y., et al. (2021). A study on the relationship between shame, guilt, self-criticism and compulsive sexual behaviour disorder. Curr. Psychol. doi: 10.1007/s12144-021-02188-3
Snoek, A., McGeer, V., Brandenburg, D., and Kennett, J. (2021). Managing shame and guilt in addiction: a pathway to recovery. Addict. Behav. 120, 106954. doi: 10.1016/j.addbeh.2021.106954
Sroufe, L. A. (1996). Emotional Development: The Organization of Emotional Life in the Early Years. 1st ed. New York, NY: Cambridge University Press.
Steenbergen, L., Maraver, M. J., Actis-Grosso, R., Ricciardelli, P., and Colzato, L. S. (2021). Recognizing emotions in bodies: vagus nerve stimulation enhances recognition of anger while impairing sadness. Cogn. Affect. Behav. Neurosci. 21, 1246–1261. doi: 10.3758/s13415-021-00928-3
Taylor, K. S., Kucyi, A., Millar, P. J., Murai, H., Kimmerly, D. S., Morris, B. L., et al. (2016). Association between resting-state brain functional connectivity and muscle sympathetic burst incidence. J. Neurophysiol. 115, 662–673. doi: 10.1152/jn.00675.2015
Teissier, A., Le Magueresse, C., Olusakin, J., Andrade da Costa, B. L. S., De Stasi, A. M., Bacci, A., et al. (2020). Early-life stress impairs postnatal oligodendrogenesis and adult emotional behaviour through activity-dependent mechanisms. Mol. Psychiatry 25, 1159–1174. doi: 10.1038/s41380-019-0493-2
Weissman, D. G., and Mendes, W. B. (2021). Correlation of sympathetic and parasympathetic nervous system activity during rest and acute stress tasks. Int. J. Psychophysiol. 162, 60–68. doi: 10.1016/j.ijpsycho.2021.01.015
Wilkowski, B. M., and Robinson, M. D. (2010). The anatomy of anger: an integrative cognitive model of trait anger and reactive aggression. J. Pers. 78, 9–38. doi: 10.1111/j.1467-6494.2009.00607.x
Williams, R. (2017). Anger as a basic emotion and its role in personality building and pathological growth: the neuroscientific, developmental and clinical perspectives. Front. Psychol. 8, 1950. doi: 10.3389/fpsyg.2017.01950
Woodard, K., and Pollak, S. D. (2020). Is there evidence for sensitive periods in emotional development? Curr. Opin. Behav. Sci. 36, 1–6. doi: 10.1016/j.cobeha.2020.05.004
Xie, W., McCormick, S. A., Westerlund, A., Bowman, L. C., and Nelson, C. A. (2019). Neural correlates of facial emotion processing in infancy. Dev. Sci. 22, e12758. doi: 10.1111/desc.12758
Keywords: anger, interpersonal violence, polyvagal theory, hierarchical emotional response, vagus nerve, myelination, development
Citation: Poli A, Gemignani A, Chiorri C and Miccoli M (2022) A critical period for experience-dependent development of the feelings of safety during early infancy: A polyvagal perspective on anger and psychometric tools to assess perceived safety. Front. Integr. Neurosci. 16:915170. doi: 10.3389/fnint.2022.915170
Received: 08 April 2022; Accepted: 27 June 2022;
Published: 18 July 2022.
Edited by:
Darren J. Edwards, Swansea University, United KingdomReviewed by:
Elisabetta Patron, University of Padua, ItalyCopyright © 2022 Poli, Gemignani, Chiorri and Miccoli. This is an open-access article distributed under the terms of the Creative Commons Attribution License (CC BY). The use, distribution or reproduction in other forums is permitted, provided the original author(s) and the copyright owner(s) are credited and that the original publication in this journal is cited, in accordance with accepted academic practice. No use, distribution or reproduction is permitted which does not comply with these terms.
*Correspondence: Andrea Poli, YW5kcmVhLnBvbGlAbWVkLnVuaXBpLml0
†ORCID: Andrea Poli orcid.org/0000-0001-7163-9627
Angelo Gemignani orcid.org/0000-0001-7249-874X
Carlo Chiorri orcid.org/0000-0002-1640-3897
Mario Miccoli orcid.org/0000-0002-8632-6145