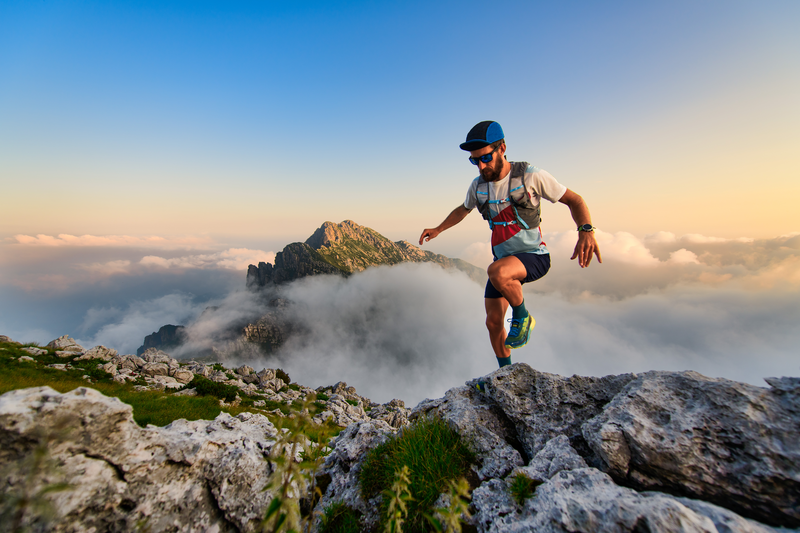
94% of researchers rate our articles as excellent or good
Learn more about the work of our research integrity team to safeguard the quality of each article we publish.
Find out more
ORIGINAL RESEARCH article
Front. Insect Sci.
Sec. Invasive Insect Species
Volume 5 - 2025 | doi: 10.3389/finsc.2025.1527130
The final, formatted version of the article will be published soon.
You have multiple emails registered with Frontiers:
Please enter your email address:
If you already have an account, please login
You don't have a Frontiers account ? You can register here
Genotypic variation was discovered in the Solenopsis invicta venom 2 gene in a small number of Solenopsis invicta field colonies. Many of these unique genotypes exhibited strong identity to the Solenopsis richteri venom 2 ortholog from the congener, Solenopsis richteri. Phylogenetic analysis of these sequences revealed a significant evolutionary relationship with Solenopsis richteri despite being obtained from Solenopsis invicta. A unique, truncated, Solenopsis invicta venom 2-like protein was also discovered in these colonies originating from a unique locus on chromosome 10 where multiple duplication events have apparently copied this gene. Western blotting confirmed translation of the truncated Solenopsis invicta venom 2-like transcript. Expression patterns for these venom genes was were altered when the truncated form of the venom was expressed.
Keywords: Solenopsis invicta, Solenopsis richteri, venom, Alkaloids, Formicidae
Received: 12 Nov 2024; Accepted: 06 Mar 2025.
Copyright: © 2025 Valles, Vander Meer and Estep. This is an open-access article distributed under the terms of the Creative Commons Attribution License (CC BY). The use, distribution or reproduction in other forums is permitted, provided the original author(s) or licensor are credited and that the original publication in this journal is cited, in accordance with accepted academic practice. No use, distribution or reproduction is permitted which does not comply with these terms.
* Correspondence:
Steven M Valles, USDA Agricultural Research Service, Gainesville, Florida, Unites States, Gainesville, United States
Disclaimer: All claims expressed in this article are solely those of the authors and do not necessarily represent those of their affiliated organizations, or those of the publisher, the editors and the reviewers. Any product that may be evaluated in this article or claim that may be made by its manufacturer is not guaranteed or endorsed by the publisher.
Research integrity at Frontiers
Learn more about the work of our research integrity team to safeguard the quality of each article we publish.