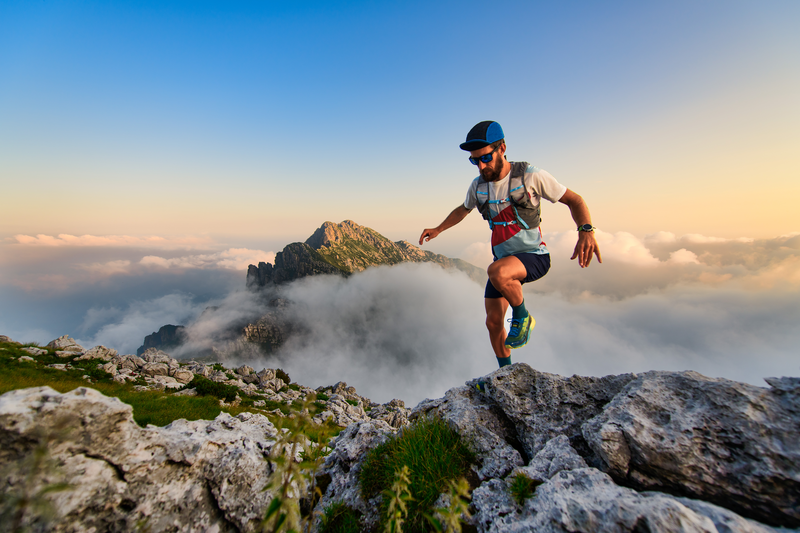
95% of researchers rate our articles as excellent or good
Learn more about the work of our research integrity team to safeguard the quality of each article we publish.
Find out more
EDITORIAL article
Front. Insect Sci. , 18 March 2024
Sec. Invasive Insect Species
Volume 4 - 2024 | https://doi.org/10.3389/finsc.2024.1378061
This article is part of the Research Topic Forest Insect Invasions – Risk Mapping Approaches and Applications View all 5 articles
Editorial on the Research Topic
Forest insect invasions – risk mapping approaches and applications
Forests around the globe are facing unprecedented threats from biotic and abiotic factors that challenge the overall health of ecosystems and potentially contribute to climate warming through positive feedback to atmospheric CO2 (1–4). Presently, some of the greatest challenges pertain to the rapid spread and resulting tree mortality caused by non-native forest insects (5, 6). Increased global trade and more favorable climates due to climate change have facilitated the establishment of non-native forest insects in new areas where conditions were previously unsuitable for their growth and survival (7, 8). The lack of co-evolutionary history between native trees and invasive insects, combined with the absence of natural enemies in the invaded habitats, has allowed aggressive non-native forest insects to rapidly colonize or kill trees and expand their invaded range in a short period of time (9). The invasion and spread of certain non-native forest insects such as the spongy moth [Lymantria dispar (L.)], the hemlock woolly adelgid [Adelges tsugae (Annand)], and the emerald ash borer (Agrilus planipennis Fairmaire) can have both short- and long-term impacts on forest health through the disruption of a range of ecological processes and ecosystem services (5, 9, 10). For example, some non-native forest insects can cause extensive defoliation or tree mortality (5), alter plant community composition (11), change soil hydrology (12), and influence carbon and nutrient cycling (13, 14). The socio-economic costs and human health-related impacts of invasive forest insects can be very significant (15, 16). These impacts will continue to be a concern owing to the consistently large number of non-native insects being intercepted at ports of entry (17–19).
Given the far-reaching consequences of non-native forest insect invasions on the environment and the economy, it is critical to address this threat for the long-term sustainability of urban and natural forest ecosystems. Pest risk models and maps are key tools for assessing the risk posed by such threats as they enable quantification and visualization of the invasion and damage potential of non-native pests (20). They may incorporate transport pathways, known or presumed responses to one or more environmental drivers and/or the dispersal capacity of invasive species to predict their seasonal activity (phenology) and population dynamics or to predict the likelihood of pest introduction, establishment, and spread (21, 22). A wide range of modeling methods are available, such as correlative (e.g., ecological niche models), semi-mechanistic (e.g., CLIMEX), and process-based approaches (e.g., insect phenology models) (20, 22, 23). When integrated with impact assessments, pest risk models can be used for decision support to guide management and surveillance strategies (21). This editorial aims to summarize published articles that cover the above-mentioned aspects under the Research Topic, Forest Insect Invasions – Risk Mapping Approaches and Applications, highlighting the latest work in predictive modeling and pest surveillance.
Maps that predict the phenology of invasive insects can support efforts to detect and control populations because decision-makers often target life stages that are more observable (e.g., larvae vs. adults of wood-boring beetles) or more susceptible to control tactics such as pesticide treatments (24, 25). Similarly, maps that predict the risk of establishment and spread of invasive insects can support surveillance programs by identifying areas that have both suitable environments for population persistence and a high likelihood of pest arrival (26–28). In this Research Topic, Takeuchi et al. presented a web-based spatial analytic framework that produces forecasts of the phenology, climate suitability, and spread of high-priority invasive insects and diseases that threaten forest and agricultural ecosystems in the United States (US). The Spatial Analytic Framework for Advanced Risk Information Systems (SAFARIS) is publicly available and was developed to support the surveillance efforts conducted by the US Department of Agriculture, Animal and Plant Health Inspection Service, Plant Protection and Quarantine (USDA-APHIS-PPQ) program in the continental US. The utility of the SAFARIS system was demonstrated using two invasive insect species that threaten forests in the US, the oak ambrosia beetle (Platypus quercivorus Murayama, 1925) and the spongy moth. In a related study, Barker et al. developed and validated a spatial model that combines forecasts of emerald ash borer (EAB) phenology and risk of establishment to help with the development and implementation of effective management strategies against this major invasive pest of ash (Fraxinus spp.) in North America and other regions such as Europe. The model for EAB is one of 16 developed for use in the Degree-Days, Risk, and Phenological event mapping (DDRP) platform, which serves as an open-source modeling tool to help detect, monitor, and manage invasive threats (29). Near-real-time model forecasts of EAB for the continental US are available on two websites to support decision-making for the detection of new establishments and for controlling existing populations with pesticide treatments and parasitoid introductions.
Certain invasive insect species can be monitored using natural methods such as assessing the prey catches of predatory insects. This approach is particularly useful when traditional monitoring methods are laborious and expensive, as is the case with EAB. A study by Rutledge and Clark examined EAB catches by a predatory wasp, Cerceris fumipennis Say, to describe the occurrence and proportional abundance of EAB among all buprestids caught by C. fumipennis. The paper presents ten years of biosurveillance data on EAB in the state of Connecticut, US, to identify the time from first detection to a population decline, which was nine years on average. The outward expansion of EAB from an epicenter assessed through the prey-capture methodology supports findings on EAB dispersal using other frameworks (e.g., tree infestations) (30).
Trotter et al. introduced the Asian Longhorned Beetle Hazard Management and Monitoring (ALBHMM) 2.0 tool, which offers a structured approach to tracking progress toward eradication and optimizing future management efforts for the Asian longhorned beetle [Anoplophora glabripennis (Motschulsky)], an invasive wood borer native to China and Southeast Asia that attacks multiple hardwood species (17, 31). The Asian longhorned beetle has been introduced into the US, Canada, and Europe (17–19) raising phytosanitary concerns that have led to the adoption of policies aimed at preventing its spread and eradicating established populations (32). The beetle poses a threat to both urban tree cover and forested landscapes, making eradication efforts crucial. The ALBHMM 2.0 tool integrates information on beetle dispersal, surveys, and management activities (tree removal) to quantify changes in infestation risk at the landscape scale, allowing for measurement of changes in infestation risk over time to monitor eradication progress. The utility of the tool was demonstrated with infestation data from three US states with varying beetle dispersal behaviors and eradication program histories.
In summary, this Research Topic sought to collate contributions describing novel techniques and recent advancements in modeling to assess and reduce the risk posed by non-native forest insect invasions. The studies published here presented innovative tools (SAFARIS, DDRP, and ALBHMM 2.0) to support and improve strategic and tactical decisions for insect surveillance and management, in addition to a novel biosurveillance approach for population monitoring (EAB monitoring using a predatory wasp). The modeling approaches included in this Research Topic provide unique perspectives on pest risk assessment due to differences in their modeling frameworks, but they can also be complementary. For instance, the DDRP system, which was used to model EAB in Barker et al., is complementary to SAFARIS in that model forecasts can support decision-making for the surveillance of invasive pests and for the management of already-established invasive pests.
The adoption of pest forecasting tools and map products requires engagement with end users such as pest control managers, government officials, and the general public (33). However, model complexity, a lack of training opportunities for end users, and insufficient outreach can hinder the broader uptake of these tools and products. Therefore, providing educational opportunities, requesting user feedback, and improving the delivery and formats of map products based on the feedback received are recommended (33). Considering the potential for future invasions of destructive forest insects under climate change, utilizing new and powerful technologies to model pest risk and filling gaps in end-user outreach and training are critical to safeguarding forest health.
KS: Writing – review & editing, Writing – original draft. VS: Writing – review & editing, Writing – original draft. BB: Writing – review & editing, Writing – original draft. MK: Writing – review & editing. MO: Writing – review & editing. AC: Writing – review & editing.
The author(s) declare that no financial support was received for the research, authorship, and/or publication of this article.
The authors declare that the research was conducted in the absence of any commercial or financial relationships that could be construed as a potential conflict of interest.
The handling editor RV declared a shared affiliation with the author MAK at the time of review.
The author(s) declared that they were an editorial board member of Frontiers, at the time of submission. This had no impact on the peer review process and the final decision.
All claims expressed in this article are solely those of the authors and do not necessarily represent those of their affiliated organizations, or those of the publisher, the editors and the reviewers. Any product that may be evaluated in this article, or claim that may be made by its manufacturer, is not guaranteed or endorsed by the publisher.
1. Dymond CC, Neilson ET, Stinson G, Porter K, MacLean DA, Gray DR, et al. Future spruce budworm outbreak may create a carbon source in eastern Canadian forests. Ecosystems. (2010) 13:917–31. doi: 10.1007/s10021-010-9364-z
2. Hicke JA, Allen CD, Desai AR, Dietze MC, Hall RJ, Ted Hogg EH, et al. Effects of biotic disturbances on forest carbon cycling in the United States and Canada. Global Change Biol. (2012) 18:7–34. doi: 10.1111/j.1365-2486.2011.02543.x
3. Kurz WA, Stinson G, Rampley GJ, Dymond CC, Neilson ET. Risk of natural disturbances makes future contribution of Canada's forests to the global carbon cycle highly uncertain. Proc Natl Acad Sci U.S.A. (2008) 105:1551–5. doi: 10.1073/pnas.0708133105
4. Sambaraju K, Goodsman D. Mountain pine beetle: an example of a climate-driven eruptive insect impacting conifer forest ecosystems. CAB Rev. (2021) 16:1–18. doi: 10.1079/PAVSNNR202116018
5. Fei S, Morin RS, Oswalt CM, Liebhold AM. Biomass losses resulting from insect and disease invasions in US forests. Proc Natl Acad Sci U.S.A. (2019) 116:17371–6. doi: 10.1073/pnas.1820601116
6. Lovett GM, Weiss M, Liebhold AM, Holmes TP, Leung B, Lambert KF, et al. Nonnative forest insects and pathogens in the United States: Impacts and policy options. Ecol Appl. (2016) 26:1437–55. doi: 10.1890/15-1176
7. Aukema JE, McCullough DG, Von Holle B, Liebhold AM, Britton K, Frankel SJ. Historical accumulation of nonindigenous forest pests in the continental United States. Bioscience. (2010) 60:886–97. doi: 10.1525/bio.2010.60.11.5
8. Roques A. Alien forest insects in a warmer world and a globalised economy: impacts of changes in trade, tourism and climate on forest biosecurity. N Z J For Sci. (2010) 40:S77–94.
9. Gandhi KJK, Herms DA. Direct and indirect effects of alien insect herbivores on ecological processes and interactions in forests of eastern North America. Biol Invasions. (2010) 12:389–405. doi: 10.1007/s10530-009-9627-9
10. Morin RS, Liebhold AM. Invasions by two non-native insects alter regional forest species composition and successional trajectories. For Ecol Manag. (2015) 341:67–74. doi: 10.1016/j.foreco.2014.12.018
11. Anderson-Teixeira KJ, Herrmann V, Cass WB, Williams AB, Paull SJ, Gonzalez-Akre EB, et al. Long-term impacts of invasive insects and pathogens on composition, biomass, and diversity of forests in Virginia’s Blue Ridge Mountains. Ecosystems. (2021) 24:89–105. doi: 10.1007/s10021-020-00503-w
12. Robertson WM, Robinett M, McCullough DG. Soil moisture response to white ash mortality following emerald ash borer invasion. Environ Earth Sci. (2018) 77:356. doi: 10.1007/s12665-018-7525-0
13. Lovett GM, Christenson LM, Groffman PM, Jones CG, Hart JE, Mitchell MJ. Insect defoliation and nitrogen cycling in forests. Bioscience. (2002) 52:335–41. doi: 10.1641/0006-3568(2002)052[0335:IDANCI]2.0.CO;2
14. Clark KL, Skowronski N, Hom J. Invasive insects impact forest carbon dynamics. Global Change Biol. (2010) 16:88–101. doi: 10.1111/j.1365-2486.2009.01983.x
15. Aukema JE, Leung B, Kovacs K, Chivers C, Britton KO, Englin J, et al. Economic impacts of non-native forest insects in the continental United States. PloS One. (2011) 6:e24587. doi: 10.1371/journal.pone.0024587
16. Donovan GH, Butry DT, Michael YL, Prestemon JP, Liebhold AM, Gatziolis D, et al. The relationship between trees and human health: evidence from the spread of the emerald ash borer. Am J Prev Med. (2013) 44:139–45. doi: 10.1016/j.amepre.2012.09.066
17. Haack RA. Exotic bark-and wood-boring Coleoptera in the United States: recent establishments and interceptions. Can J For Res. (2006) 36:269–88. doi: 10.1139/x05-249
18. Humble L, Allen E. Forest biosecurity: alien invasive species and vectored organisms. Can J Plant Pathol. (2006) 28:S256–69. doi: 10.1080/07060660609507383
19. Roques A, Auger-Rozenberg MA. Tentative analysis of the interceptions of non-indigenous organisms in Europe during 1995–20041. EPPO Bull. (2006) 36:490–6. doi: 10.1111/j.1365-2338.2006.01049.x
20. Venette RC, Kriticos DJ, Magarey RD, Koch FH, Baker RH, Worner SP, et al. Pest risk maps for invasive alien species: a roadmap for improvement. Bioscience. (2010) 60:349–62. doi: 10.1525/bio.2010.60.5.5
21. Lafond V, Lingua F, Lumnitz S, Paradis G, Srivastava V, Griess VC. Challenges and opportunities in developing decision support systems for risk assessment and management of forest invasive alien species. Environ Rev. (2020) 28:218–45. doi: 10.1139/er-2019-0024
22. Venette RC. Climate analyses to assess risks from invasive forest insects: simple matching to advanced models. Curr For Rep. (2017) 3:255–68. doi: 10.1007/s40725-017-0061-4
23. Régnière J, Sharov A. Phenology of Lymantria dispar (Lepidoptera: Lymantriidae), male flight and the effect of moth dispersal in heterogeneous landscapes. Int J Biometeorol. (1998) 41:161–8.
24. Barker BS, Coop L. Phenological mapping of invasive insects: decision support for surveillance and management. Insects. (2024) 15:6. doi: 10.3390/insects15010006
25. Bowers JH, Malayer JR, Martínez-López B, LaForest J, Bargeron CT, Neeley AD, et al. Surveillance for early detection of high-consequence pests and pathogens. In: Tactical Sciences for Biosecurity in Animal and Plant Systems. Cardwell KF, Bailey KL. Hershey, PA: IGI Global (2022). p. 120–77. doi: 10.4018/978-1-7998-7935-0.ch005
26. Jones C, Skrip MM, Seliger BJ, Jones S, Wakie T, Takeuchi Y, et al. Spotted lanternfly predicted to establish in California by 2033 without preventative management. Commun Biol. (2022) 5:558. doi: 10.1038/s42003-022-03447-0
27. Jones CM, Jones S, Petrasova A, Petras V, Gaydos D, Skrip MM, et al. Iteratively forecasting biological invasions with PoPS and a little help from our friends. Front Ecol Environ. (2021) 19:411–8. doi: 10.1002/fee.2357
28. Lantschner MV, de la Vega G, Corley JC. Predicting the distribution of harmful species and their natural enemies in agricultural, livestock and forestry systems: an overview. Int J Pest Manag. (2019) 65:190–206. doi: 10.1080/09670874.2018.1533664
29. Barker BS, Coop L, Wepprich T, Grevstad F, Cook G. DDRP: real-time phenology and climatic suitability modeling of invasive insects. PloS One. (2020) 15:e0244005. doi: 10.1371/journal.pone.0244005
30. Sargent C, Raupp M, Bean D, Sawyer AJ. Dispersal of emerald ash borer within an intensively managed quarantine zone. Arboric Urban For. (2010) 36:160–3. doi: 10.48044/jauf.2010.021
31. MacLeod A, Evans H, Baker R. An analysis of pest risk from an Asian longhorn beetle (Anoplophora glabripennis) to hardwood trees in the European community. Crop Prot. (2002) 21:635–45. doi: 10.1016/S0261-2194(02)00016-9
32. Srivastava V, Roe AD, Keena MA, Hamelin RC, Griess VC. Oh the places they’ll go: improving species distribution modelling for invasive forest pests in an uncertain world. Biol Invasions. (2021) 23:297–349. doi: 10.1007/s10530-020-02372-9
Keywords: forest insects, invasive species, risk assessment, modelling, risk mapping, climate change
Citation: Sambaraju KR, Srivastava V, Barker BS, Keena MA, Ormsby MD and Carroll AL (2024) Editorial: Forest insect invasions – risk mapping approaches and applications. Front. Insect Sci. 4:1378061. doi: 10.3389/finsc.2024.1378061
Received: 29 January 2024; Accepted: 06 March 2024;
Published: 18 March 2024.
Edited and Reviewed by:
Robert C Venette, Forest Service, United States Department of Agriculture (USDA) St. Paul, Minnesota, United StatesCopyright © 2024 Sambaraju, Srivastava, Barker, Keena, Ormsby and Carroll. This is an open-access article distributed under the terms of the Creative Commons Attribution License (CC BY). The use, distribution or reproduction in other forums is permitted, provided the original author(s) and the copyright owner(s) are credited and that the original publication in this journal is cited, in accordance with accepted academic practice. No use, distribution or reproduction is permitted which does not comply with these terms.
*Correspondence: Kishan R. Sambaraju, a2lzaGFuLnNhbWJhcmFqdUBucmNhbi1ybmNhbi5nYy5jYQ==
Disclaimer: All claims expressed in this article are solely those of the authors and do not necessarily represent those of their affiliated organizations, or those of the publisher, the editors and the reviewers. Any product that may be evaluated in this article or claim that may be made by its manufacturer is not guaranteed or endorsed by the publisher.
Research integrity at Frontiers
Learn more about the work of our research integrity team to safeguard the quality of each article we publish.