- 1United States Department of Agriculture - Agricultural Research (USDA-ARS) Service, Bee Research Laboratory, Beltsville, MD, United States
- 2Department of Entomology, University of Maryland, College Park, MD, United States
- 3Arkema, Inc., King of Prussia, PA, United States
Deformed wing virus (DWV) is a widespread pathogen of Apis mellifera honey bees, and is considered a major causative factor for the collapse of infected honey bee colonies. DWV can be horizontally transmitted among bees through various oral routes, including via food sharing and by interactions of bees with viral-contaminated solid hive substrates. Cold plasma ionized hydrogen peroxide (iHP) is used extensively by the food production, processing and medical industries to clean surfaces of microbial contaminants. In this study, we investigated the use of iHP to inactivate DWV particles in situ on a solid substrate. iHP-treated DWV sources were ~105-fold less infectious when injected into naïve honey bee pupae compared to DWV receiving no iHP treatment, matching injected controls containing no DWV. iHP treatment also greatly reduced the incidence of overt DWV infections (i.e., pupae having >109 copies of DWV). The level of DWV inactivation achieved with iHP treatment was higher than other means of viral inactivation such as gamma irradiation, and iHP treatment is likely simpler and safer. Treatment of DWV contaminated hive substrates with iHP, even with honey bees present, may be an effective way to decrease the impacts of DWV infection on honey bees.
Introduction
Deformed wing virus (DWV) is a positive-stranded RNA virus (Iflaviradae) that infects several hymenopteran taxa including bees, wasps, and ants (1–3). A growing body of evidence suggests that spread of the mite Varroa destructor (hereafter, Varroa) has greatly increased pathogenicity of DWV (4–6), making it the most important viral pathogen of the Western honey bee, Apis mellifera (7). Symptoms of overt DWV infections of adult honey bees include damaged appendages, particularly stubby, useless wings, shortened, rounded abdomens, and miscoloring and paralysis of the legs and wings. These symptoms are strongly correlated with elevated DWV levels (i.e., genome equivalents (GE)) (8). DWV infections can reduce the lifespan of covertly infected adult honey bees (9, 10).
In the absence of mites, the virus persists in bee populations as a covert infection, transmitted horizontally (11) via several routes, including orally among adults through trophallaxis (12) and between adults and immature bees via hypopharyngeal gland secretions (13) and brood food (14) fed to larvae, and possibly through a fecal–oral route of transmission between adult bees (15, 16). Workers may also be infected through feeding on DWV-contaminated hive products, such as pollen (17) and honey (18), or by cannibalizing infected pupae (12). Adult honey bees clean hive substrates, including the meconium and other contents remaining in pupal cells of newly-eclosed adults, possibly becoming exposed to latent DWV on interior surfaces of hives (19, 20). While little work has been conducted to investigate this latter route, honey bees were found to become infected by interacting with DWV-contaminated beeswax (18).
Persistence of virus infectivity in the environment can vary; Enveloped viruses, such as influenza and coronaviruses, are considered less stable than non-enveloped viruses, such as norovirus (21). There is little information on the persistence of DWV infectivity outside their hosts, but considering that DWV virions are similar to those of other Picorna-like viruses, it is very likely that environmental DWV contamination plays a significant role in circulation of DWV. Indeed, infection can occur in adult bees contacting contaminated beeswax (18). Thus, deactivating latent DWV and other honey bee viral pathogens on hive substrates may reduce incidence of infection for resident bees. Treatment of surfaces with iHP, or ionized hydrogen peroxide is widespread in hospitals and other spaces where decontamination from microbial pathogens is required (22). Specifically, iHP treatment has been shown to be effective against the coronavirus (23, 24), as well as other microbial pathogens (25). Hydrogen peroxide is a potent oxidizer and is believed to inactivate viruses through oxidation of protein side chains (26) and nucleotides (27). In this study, we explore the use of iHP to reduce the infectivity of DWV to naïve honey bee pupae.
Materials and methods
Source of honey bee deformed wing virus
Clone-derived DWV-A isolate Maryland/2015/422 (GenBank: MG831202) (28) was used in this experiment. The virus was propagated in honeybee pupae injected with infectious in vitro RNA transcripts (28). For preparation of extracts containing infectious DWV virus particles, individual transcript-injected pupae were homogenized with 2 mL of PBS at 3 days post-injection, subjected to three freeze–thaw cycles, clarified by centrifugation at 3000g for 5 min and filtered through 0.22 μm nylon filter (Millipore). The DWV concentration in these extracts was quantified by qRT-PCR. The original DWV-A extract used in this study had 108 genome equivalents (GE) of DWV per microliter. The infectivity of the clone-derived DWV extracts was confirmed by the presence of high levels of DWV infections (1011 to 1012 GE/pupae) in the pupae injected with 106 GE in 10 μL of PBS 3 days prior. The extracts were stored at -80°C prior to use. The identity of clonal DWV in the extract with its respective parental cDNA clone was confirmed by sequencing of RT-PCR fragments (29).
Contamination of surfaces with DWV particles
To assess the effect of iHP treatment on DWV infectivity, DWV were placed on a non-porous surface were exposed to iHP treatment, then tested for their ability to infect honey bee pupae (Figure 1). For this, 15 μL of DWV extract in PBS at 108 DWV particles per 1 μL were placed on a thin, 2 cm x 5 cm rectangular steel sheet. Prior to application of the virus suspension, the metal surface was sterilized with 100% ethanol, washed with sterile water and air-dried. As a control, 15 μL of PBS containing no virus extracts were also pipetted onto a steel sheet. Each 15 μL aliquot was evenly spread to cover approximately 2 cm2; this area was delineated with an ultra-fine marker prior to placing the liquid aliquots. Aliquots were air-dried at room temperature for 30 minutes. The contents of the aliquots were recovered from the surfaces by washing the marked areas with 150 μL of PBS; resulting in virus suspensions that were assumed to have a concentration of 107 DWV particles per 1 μL. The viral suspensions and control solutions were stored at -80 °C prior to testing for infectivity.
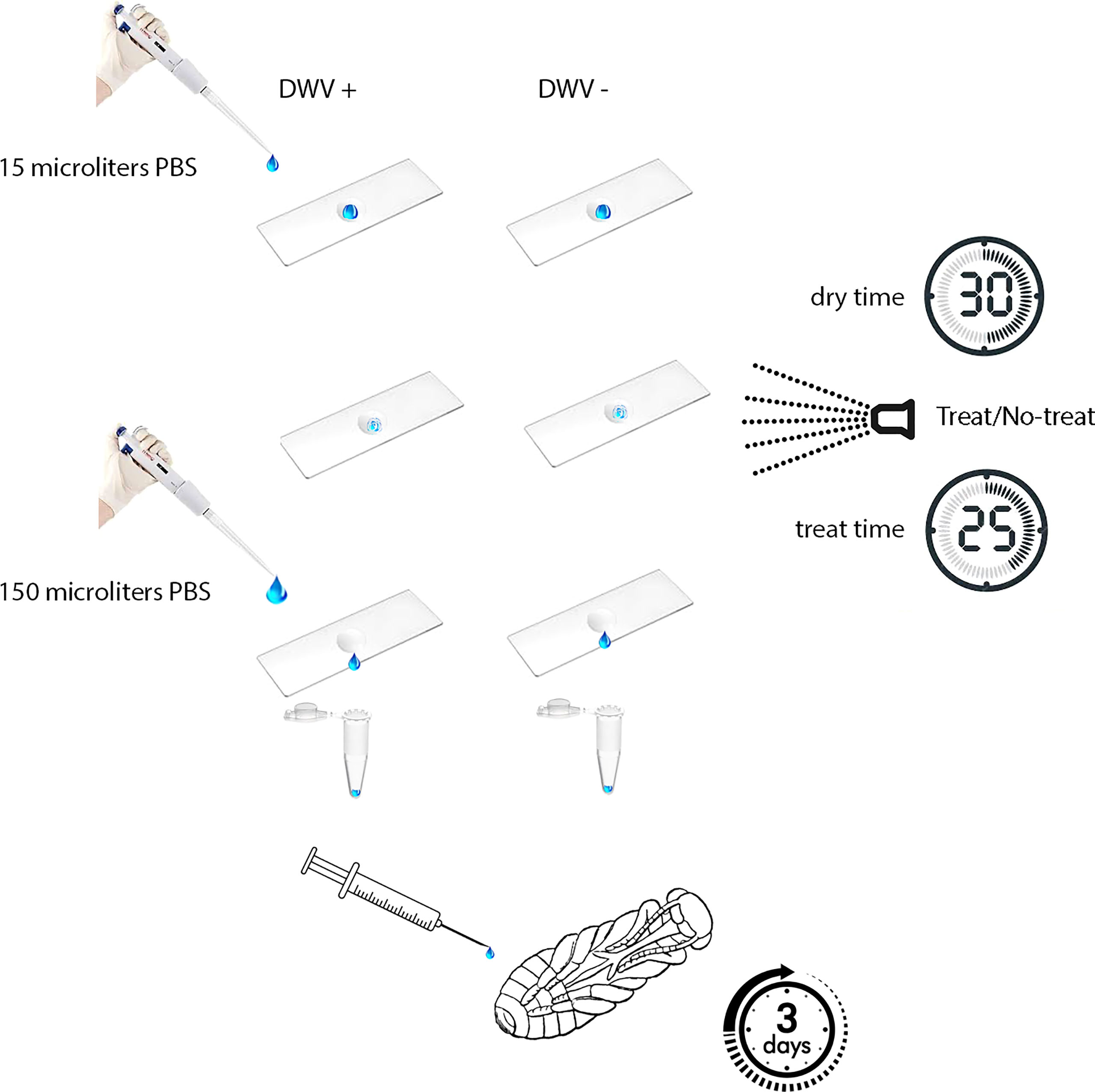
Figure 1 Experimental design. Aliquots of PBS either with or without DWV were placed on a non-porous surface, allowed to dry, then either treated or untreated with ionized hydrogen peroxide (iHP). Aliquots were recovered from surfaces and then injected in naïve honey bee pupae. DWV infection progressed for three days prior to pupae being analyzed for their DWV content (i.e., number of genome equivalents).
Treatment with ionized hydrogen peroxide
iHP mist exposure occurred in a clean, sealed 14 ft by 9 ft by 9 ft (~4.3 m x ~2.7 m x ~2.7 m) rectangular environmental chamber located at the USDA-ARS Bee Research Laboratory, Beltsville, MD. iHP mist was introduced into the treatment chamber using SteraMist application technology (from TOMI™ Environmental Solutions). Binary Ionization Technology (BIT) Solution (TOMI Environmental Solutions, Inc.) was applied at a dose rate of 0.5 mL/ft3 (~0.03 m3). In the application process, first the BIT Solution passed through a nozzle that generated an ultra-fine 0.05 – 3-micron particle mist (25). Subsequently, the mist was ionized by cold plasma generated between two pin electrodes. Two applicators were placed in opposite corners of the room. The room was sterilized prior to treating the inoculated steel plates by applying 567 mL of BIT Solution at a rate of 25 mL/min per applicator, resulting in the dose concentration of 0.5 mL per cubic foot for the treated area. The application time for the dose was 10 min and was followed by a contact time of 15 min (Figure 1). Following the application and contact time, remaining iHP mist was removed from the chamber through an inline exhaust fan to outside until the hydrogen peroxide ppm levels in the chamber were measured at <1 ppm (Draeger, Inc. USA) (~15 mins). This aeration process was shortened by the natural breakdown of the hydrogen peroxide to water and oxygen. When the room was cleared of residual hydrogen peroxide, steel plates were placed with the inoculated area facing up on a cart previously placed in the room and sterilized, and the room was again treated as above. Following aeration, the steel plates were removed from the room and returned to the laboratory where both the virus and PBS control aliquots were recovered from steel plates as described above. The presence of hydrogen peroxide mist in the room after the application dose was applied and confirmed by iodine indicator strips (LaMotte, Maryland, USA), which change color from yellow to various blue shades based on the level of hydrogen peroxide present in the room.
Blank treatments were conducted by running sterile water through the SteraMist application equipment and removing the electrodes to prevent the plasma arc from occurring, so ionization did not occur.
Assessment of treated DWV extract infectivity
The recovered virus suspensions and PBS controls, both treated and untreated, were diluted prior to being injected into pupae for tests of viral infectivity. Pink-eye stage honey bee pupae, with no exposure to Varroa mite feeding, were extracted from a brood frame with low Varroa infestation (less than 1 mite-infested pupae per 100). Pupae were intra-abdominally injected into the hemolymph to introduce 105 and 106 GE of surface-recovered DWV, H2O2-treated or control untreated, in 10 μL of PBS or buffer control (PBS) as described previously (5, 30). The injected pupae were incubated at +33° C, 75% relative humidity (31), for 3 days prior to RNA extraction (Figure 1). DWV RNA copy numbers were quantified in 8 individual pupae per treatment group by qRT-PCR (28). In brief, total RNA was extracted using Trizol (Ambion) method according to the manufacturer’s instructions. Then, cDNA was produced using Superscript II reverse transcriptase (Invitrogen) and random hexanucleotide primers according to manufacturer’s instructions. The cDNA samples were used to determine DWV copy numbers by quantitative PCR with the oligonucleotide primers 5’-GAGATCGAAGCGCATGAACA-3’ and 5’-TGAATTCAGTGTCGCCCATA-3’, targeting the region 6497 to 6626 in the DWV genome using SsoAdvanced Universal SYBR Green Supermix (Bio-Rad, Hercules, CA, USA). A series of 10-fold dilutions of the full-length cDNA clone of DWV, pDWV-422 (28) was used to establish a standard curve by plotting Ct values against the log-transformed concentrations of DWV cDNA. The Ct values showed linearity (r2 = 0.998) and gave an amplification efficiency of 1.95.
Statistical analyses
Data for the log10-transformed qPCR thresholds (indicating DWV loads) were not normally distributed, thus these data were analyzed using a Wilcoxon test. A Wilcoxon signed ranks test for multiple comparisons was used as a post-hoc test. This test generates Z-scores which are compared to a critical statistic, W (32). For the eight honey bees per treatment, those having greater than 109 viral copies were contrasted with those having lower virus levels using a Chi-square test. For the latter, a contingency test was used to distinguish between-group differences.
Results
The development of DWV infection in honey bees injected with the DWV inocula treated for 15 minutes with ionized hydrogen peroxide (iHP), for both the 105 or 106 GE doses, were significantly reduced compared to the honey bees injected with the untreated (X2 = 19.77; P < 0.0006). The PBS-injected (Control) pupae had similar levels of DWV GE to iHP-treated samples for both inoculations (all P > 0.05), while DWV levels were significantly elevated in non-treated samples compared to PBS-injected samples and both of the iHP-treated samples (all P < 0.05) (Table 1). Pupae injected with inocula containing either 105 or 106 copies of DWV that were untreated with iHP showed DWV titers elevated up to ~1011 copies of DWV per bee after 3 days, while pupae injected with iHP-treated DWV inoculates had the level of DWV statistically indistinguishable from those in the control, PBS-injected bees, ~103-104 time lower (Figure 2A). High DWV levels characteristic of overt infections (i.e., > 109 copies/bee) were observed only in 1 of 8 (12.5%) of honey bees injected with either PBS or hydrogen peroxide-treated inocula, regardless of the DWV dose, while 7 of 8 (87.5%) and (8 of 8) 100% of honey bees injected with 105 and 106 viral copies, respectively, showed high DWV loads, 1010 - 1011, typical for overt DWV infection (Figure 2B). Such reduction of DWV infectivity after iHP treatment was statistically significant, for the 106 GE inoculum, Fisher exact probability test, P = 0.0014).
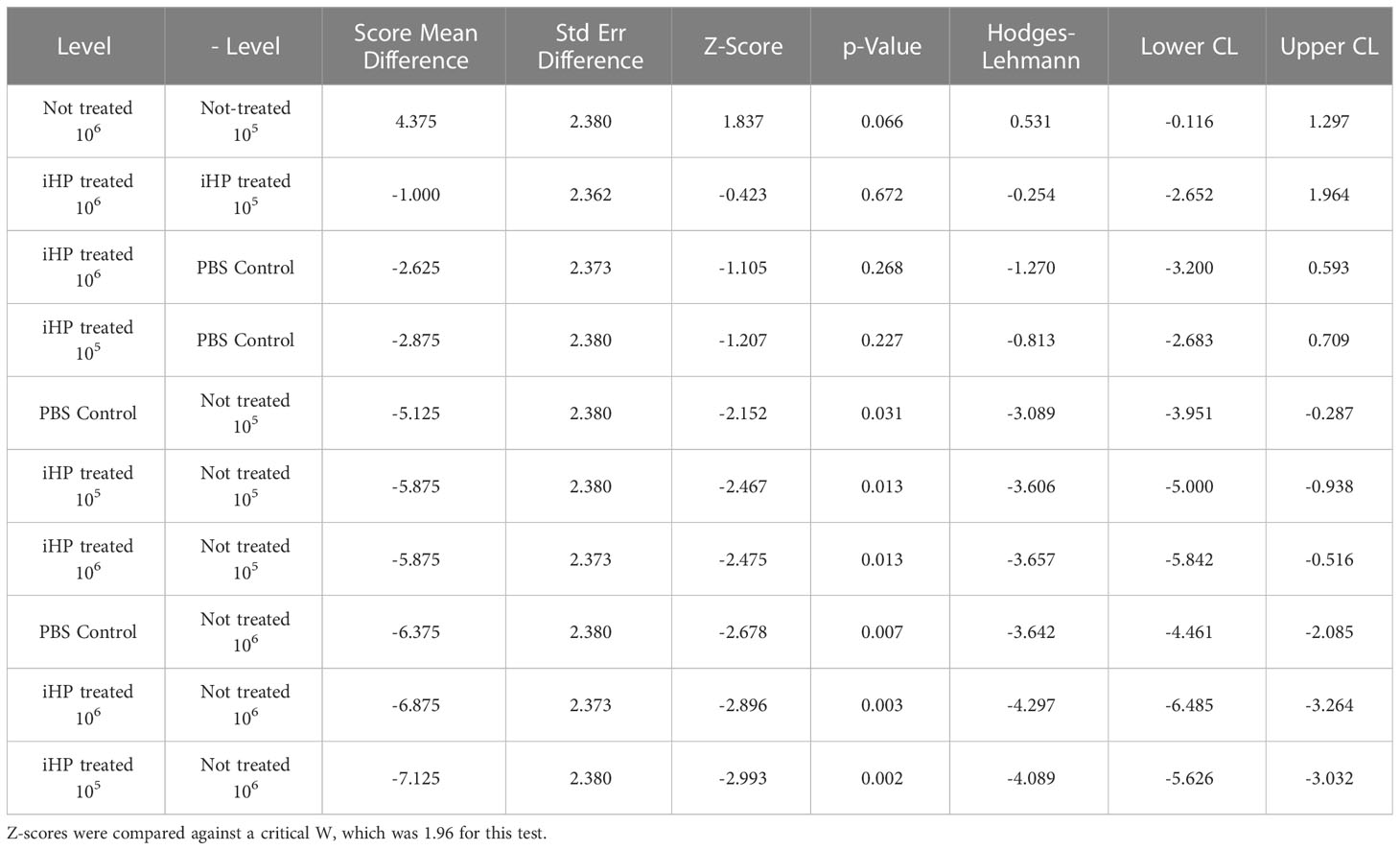
Table 1 Statistical results from the Wilcoxon signed rank test for multiple comparisons of the log10 -transformed DWV GE quantified by RT-qPCR in honey bee pupae after three days post-injection with PBS having either 105 or 106 DWV copies of DWV or without DWV (Control).
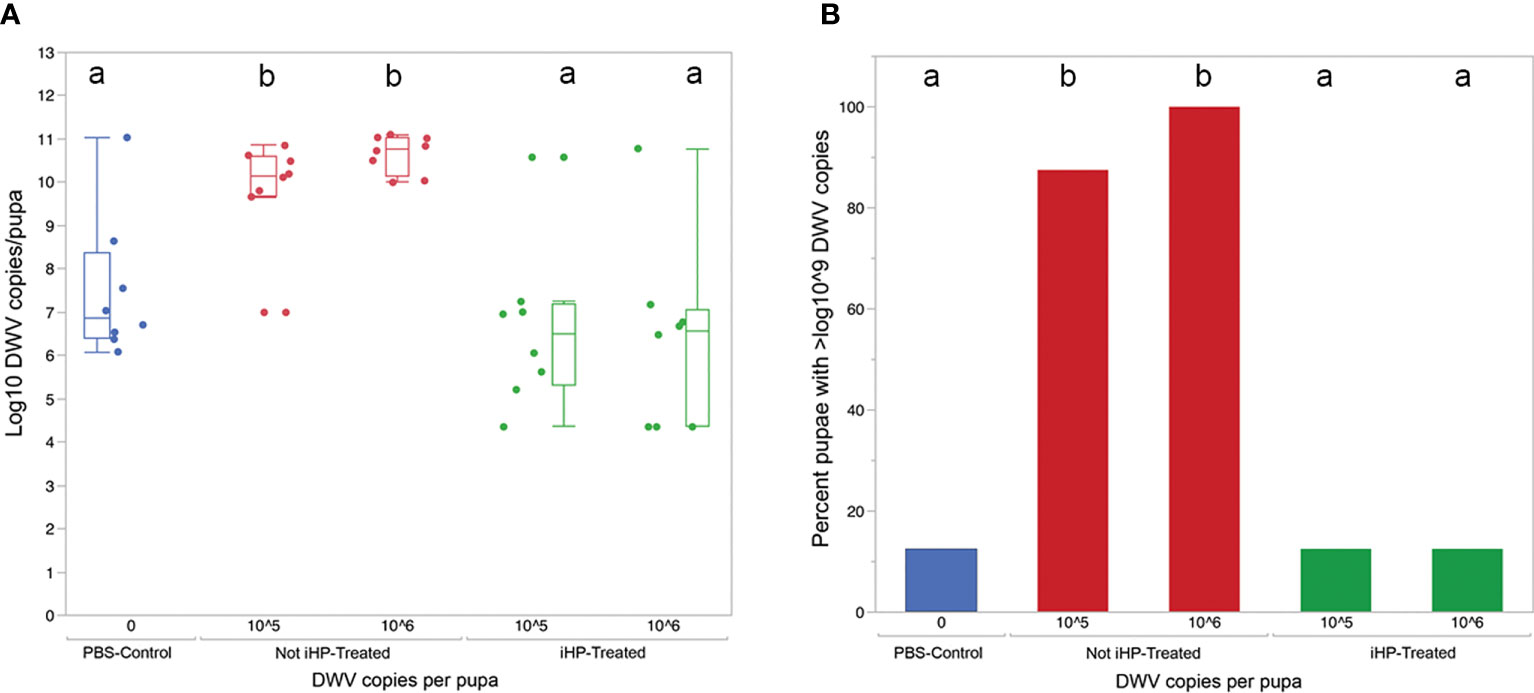
Figure 2 (A) Boxplot shows development of virus infection (log10 copy number of DWV) in honey bee pupae 3 days after injection with PBS (Control), 105 and 106 copies of DWV recovered from the surfaces and for the latter, either untreated (Not-iHP-treated) or treated with ionized hydrogen peroxide for 30 minutes (iHP-treated). Dots represent virus levels in individual pupae. Different ded letters above boxes indicate significantly different groups (p < 0.05, pairwise Wilcoxon test). (B) Percent of honey bee pupae having greater than 109 GE of DWV.
Discussion
Deformed wing virus (DWV) negatively affects honeybee health and can lead to colony losses (33, 34). Horizontal transmission of DWV among honey bees can occur via oral routes (12–16, 18), and DWV on hive surfaces could act as a reservoir for the virus. Research has demonstrated that overt DWV infections in honey bees can be caused by interactions between adult honey bees and hive substrates (e.g., wax) (18), and 60% - 80% of beeswax from brood frames was positive for a number of honey bee pathogenic viruses, including DWV (20). Virus picked up on body surfaces and ingested from fastidious hygiene of worker honey could be enough to cause overt infections; oral exposure of bumblebees to a single dose of 0.5 x 107 viral particles can cause overt infections (35). Here, we show that treatment of DWV-contaminated surfaces with iHP significantly reduces both the infectivity of the virus to honey bee pupae (104-105 reduction) and incidence of overt infections (> 109 GE of virus) with DWV in these hosts injected with iHP-treated DWV inoculates.
Past efforts to inactivate viral and other honeybee pathogens have employed a number of different disinfecting agents, including ozone, ethylene oxide, and gamma irradiation (20, 35–38). Focusing on viral inactivation, the ~105-fold reduction in viral infectivity from iHP treatment seen in our study is similar to that shown for gamma irradiation. Irradiated honey bee-collected pollen containing an inoculum (10-1 to 10-5 dilution) of the honey bee virus, Israeli acute paralysis virus (IAPV), resulted in a 1000-fold reduction of the infectivity of this virus to bumblebees (35), and reduced viability of other honey bee viruses (37), while inoculate solutions containing DWV exposed to gamma radiation for nearly 10 hours, then subsequently injected into honey bee pupae, showed 104 - 105-fold reduction in the infectivity of the virus (38). Treatment of pathogen-laden corbicular pollen with ozone had limited effect on contaminating microbes (37). Ethylene oxide treatment of hive substrates successfully inactivates bacterial and microsporidian honey bee pathogens, but this method may not be as effective against viral pathogens (36).
Consideration of using the different available decontaminating treatments for inactivating honey bee microbial pathogens from hive surfaces should examine factors such as the treatment’s efficacy against a broad range of microbes, its penetration of hive space, compatibility with hive substrates (e.g., wax, wood), the process of treatment application (i.e., cost and equipment availability) (see, (39)), and the effect of iHP on honey bees. iHP treatment appears to inactivate honey bee microbial pathogens as efficaciously as other tested treatments, yet other tradeoffs remain that could make its use more or less desirable than other treatment types. For example, both iHP (and H2O2 gas) and ethylene oxide have limited penetration of hive substrates, and water repellency of wax and possibly propolis, make iHP (H2O2 in aqueous mist) less likely to penetrate inside these substrates. This is in contrast with gamma irradiation, which can penetrate dense materials (22). However, as a gas, iHP can reach hive substrates more readily than can irradiation from a point source, hence the inability to penetrate substrates is less important. Further, both ethylene oxide and gamma irradiation are harmful to users, and at least for the former, can produce biproducts (e.g., ethylene glycol) that are toxic to honey bees (40). Finally, iHP treatment appears to have the shortest treatment duration, with high efficacy achieved with only minutes of exposure, compared to hours for gamma irradiation and days for ethylene oxide (see, (22)). Overall, iHP, ethylene oxide, and gamma irradiation show disinfectant properties across a broad range of materials (see, (39)), and decisions for which method is most suitable for the beekeeping industry will depend on cost, availability and safety. Future work could investigate the utility of H2O2 gas, which may be easier and less costly to apply than iHP vapor, to inactivate honey bee viral pathogens. Both iHP and H2O2 gas have been tested (the former in limited field trials, the latter in lab only) for their safety to honey bees. In no cases were detrimental effects on honey bees observed (data not shown).
Data availability statement
The raw data supporting the conclusions of this article will be made available by the authors, without undue reservation.
Author contributions
SC, CB and ER conceptualized the research. SC, ER, FP and CR conducted the research. SC and ER wrote the manuscript. SC, JE and YC provided funding. All authors contributed to the article and approved the submitted version.
Funding
This research was supported by external funding from the United States Department of Agriculture (USDA) National Institute of Food and Agriculture (NIFA) grants 2017-06481 (JDE, YPC and EVR) and 2021-67013-33560 (SCC, EVR and YPC), and a Material Transfer and Research Agreement (#68816) between Arkema, Inc. and USDA. The latter funder was not involved in the study design, collection, analysis, interpretation of the data, the writing of this article or the decision to submit for publication. All authors declare no other competing interests.
Acknowledgments
We would like to thank TOMI Environmental Systems, Inc. (8430 Spires Way, Suite N Frederick, MD 21701) for their technical support, and providing the Binary Ionization Technology® (BIT™) Solution and lending the disinfection fogging equipment (SteraMist™ Environment System) for this study.
Conflict of interest
Author CB is employed by Arkema, Inc.
The remaining authors declare that the research was conducted in the absence of any commercial or financial relationships that could be constructed as a potential conflict of interest.
The authors ER and JE declared that they were editorial board members of Frontiers, at the time of submission. This had no impact on the peer review process and the final decision.
Publisher’s note
All claims expressed in this article are solely those of the authors and do not necessarily represent those of their affiliated organizations, or those of the publisher, the editors and the reviewers. Any product that may be evaluated in this article, or claim that may be made by its manufacturer, is not guaranteed or endorsed by the publisher.
References
1. Genersch E, Yue C, Fries I, de Miranda JR. Detection of Deformed wing virus, a honey bee viral pathogen, in bumble bees (Bombus terrestris and Bombus pascuorum) with wing deformities. J Invertebr Pathol (2006) 91(1):61–3. doi: 10.1016/j.jip.2005.10.002
2. Brettell LE, Schroeder DC, Martin SJ. RNAseq of deformed wing virus and other honey bee-associated viruses in eight insect taxa with or without varroa infestation. Viruses (2020) 12(11):1229. doi: 10.3390/v12111229
3. Lin C-Y, Lee C-C, Nai Y-S, Hsu H-W, Lee C-Y, Tsuji K, et al. Deformed wing virus in two widespread invasive ants: geographical distribution, prevalence, and phylogeny. Viruses (2020) 12(11):1309. doi: 10.3390/v12111309
4. Martin SJ, Highfield AC, Brettell L, Villalobos EM, Budge GE, Powell M, et al. Global honey bee viral landscape altered by a parasitic mite. Science (2012) 336(6086):1304–6. doi: 10.1126/science.1220941
5. Ryabov EV, Wood GR, Fannon JM, Moore JD, Bull JC, Chandler D, et al. A Virulent Strain of Deformed Wing Virus (DWV) of Honeybees (Apis mellifera) Prevails after Varroa destructor-Mediated, or in vitro, Transmission. PloS Pathog (2014) 10(6):e1004230. doi: 10.1371/journal.ppat.1004230
6. Wilfert L, Long G, Leggett HC, Schmid-Hempel P, Butlin R, Martin SJ, et al. Deformed wing virus is a recent global epidemic in honeybees driven by Varroa mites. Science (2016) 351(6273):594–7. doi: 10.1126/science.aac9976
7. Traynor KS, Mondet F, de Miranda JR, Techer M, Kowallik V, Oddie MAY, et al. Varroa destructor: A complex parasite, crippling honey bees worldwide. Trends Parasitol (2020) 36(7):592–606. doi: 10.1016/j.pt.2020.04.004
8. Brettell LE, Mordecai GJ, Schroeder DC, Jones IM, Da Silva JR, Vicente-Rubiano M, et al. A comparison of deformed wing virus in deformed and asymptomatic honey bees. Insects (2017) 8(1):28. doi: 10.3390/insects8010028
9. McMahon DP, Natsopoulou ME, Doublet V, Fürst M, Weging S, Brown MJF, et al. Elevated virulence of an emerging viral genotype as a driver of honeybee loss. Proc R Soc B: Biol Sci (2016) 283(1833):20160811. doi: 10.1098/rspb.2016.0811
10. Benaets K, Van Geystelen A, Cardoen D, De Smet L, de Graaf DC, Schoofs L, et al. Covert deformed wing virus infections have long-term deleterious effects on honeybee foraging and survival. Proc Biol Sci (2017) 284(1884):20162149. doi: 10.1098/rspb.2016.2149
11. Möckel N, Gisder S, Genersch E. Horizontal transmission of deformed wing virus: pathological consequences in adult bees (Apis mellifera) depend on the transmission route. J Gen Virol (2011) 92(Pt 2):370–7. doi: 10.1099/vir.0.025940-0
12. Posada-Florez F, Lamas ZS, Hawthorne DJ, Chen Y, Evans JD, Ryabov EV. Pupal cannibalism by worker honey bees contributes to the spread of deformed wing virus. Sci Rep (2021) 11(1):8989. doi: 10.1038/s41598-021-88649-y
13. Fievet J, Tentcheva D, Gauthier L, de Miranda J, Cousserans F, Colin ME, et al. Localization of deformed wing virus infection in queen and drone Apis mellifera L. Virol J (2006) 3:16. doi: 10.1186/1743-422x-3-16
14. Yue C, Genersch E. RT-PCR analysis of Deformed wing virus in honeybees (Apis mellifera) and mites (Varroa destructor). J Gen Virol (2005) 86(Pt 12):3419–24. doi: 10.1099/vir.0.81401-0
15. Chen YP, Pettis JS, Collins AR, Feldlaufer MF. Prevalence and transmission of honeybee viruses. Appl Environ Microbiol (2006) 72:606–611. doi: 10.1128/AEM.72.1.606-611.2006
16. Figueroa LL, Blinder M, Grincavitch C, Jelinek A, Mann EK, Merva LA, et al. Bee pathogen transmission dynamics: deposition, persistence and acquisition on flowers. Proc R Soc B: Biol Sci (2019) 286(1903):20190603. doi: 10.1098/rspb.2019.0603
17. Singh R, Levitt AL, Rajotte EG, Holmes EC, Ostiguy N, Vanengelsdorp D, et al. RNA viruses in hymenopteran pollinators: evidence of inter-Taxa virus transmission via pollen and potential impact on non-Apis hymenopteran species. PloS One (2010) 5(12):e14357. doi: 10.1371/journal.pone.0014357
18. Schittny D, Yañez O, Neumann P. Honey bee virus transmission via hive products. Vet Sci (2020) 7(3):96. doi: 10.3390/vetsci7030096
19. Colwell MJC, Pernal SF. Viruses in unexpected places: new transmission routes of European honey bee (Apis mellifera) viruses. Bee World (2017) 93(4):106–7.
20. de Guzman LI, Simone-Finstrom M, Frake AM, Tokarz P. Comb irradiation has limited, interactive effects on colony performance or pathogens in bees, varroa destructor and wax based on two honey bee stocks. Insects (2019) 10(1):15. doi: 10.3390/insects10010015
21. de Graaf M, Villabruna N, Koopmans MPG. Capturing norovirus transmission. Curr Opin Virol (2017) 22:64–70. doi: 10.1016/j.coviro.2016.11.008
22. McEvoy B, Rowan NJ. Terminal sterilization of medical devices using vaporized hydrogen peroxide: a review of current methods and emerging opportunities. J Appl Microbiol (2019) 127(5):1403–20. doi: 10.1111/jam.14412
23. Cheng VCC, Wong SC, Kwan GSW, Hui WT, Yuen KY. Disinfection of N95 respirators by ionized hydrogen peroxide during pandemic coronavirus disease 2019 (COVID-19) due to SARS-CoV-2. J Hosp Infect (2020) 105(2):358–9. doi: 10.1016/j.jhin.2020.04.003
24. Welch JL, Xiang J, Mackin SR, Perlman S, Thorne P, O'Shaughnessy P, et al. Inactivation of severe acute respiratory coronavirus virus 2 (SARS-CoV-2) and diverse RNA and DNA viruses on three-dimensionally printed surgical mask materials. Infect Control Hosp Epidemiol (2021) 42(3):253–60. doi: 10.1017/ice.2020.417
25. Jiang Y, Sokorai K, Pyrgiotakis G, Demokritou P, Li X, Mukhopadhyay S, et al. Cold plasma-activated hydrogen peroxide aerosol inactivates Escherichia coli O157:H7, Salmonella Typhimurium, and Listeria innocua and maintains quality of grape tomato, spinach and cantaloupe. Int J Food Microbiol (2017) 249:53–60. doi: 10.1016/j.ijfoodmicro.2017.03.004
26. Finnegan M, Linley E, Denyer SP, McDonnell G, Simons C, Maillard J-Y. Mode of action of hydrogen peroxide and other oxidizing agents: differences between liquid and gas forms. J Antimicrobial. Chemother. (2010) 65(10):2108–15. doi: 10.1093/jac/dkq308
27. Hofer T, Badouard C, Bajak E, Ravanat J-L, Mattsson Å., Cotgreave IA. Hydrogen peroxide causes greater oxidation in cellular RNA than in DNA. Biological Chemistry (2005) 386(4):333–7. doi: 10.1515/BC.2005.040
28. Ryabov EV, Childers AK, Lopez D, Grubbs K, Posada-Florez F, Weaver D, et al. Dynamic evolution in the key honey bee pathogen deformed wing virus: Novel insights into virulence and competition using reverse genetics. PloS Biol (2019) 17(10):e3000502. doi: 10.1371/journal.pbio.3000502
29. Posada-Florez F, Childers AK, Heerman MC, Egekwu NI, Cook SC, Chen Y, et al. Deformed wing virus type A, a major honey bee pathogen, is vectored by the mite Varroa destructor in a non-propagative manner. Sci Rep (2019) 9(1):12445. doi: 10.1038/s41598-019-47447-3
30. Ryabov EV, Fannon JM, Moore JD, Wood GR, Evans DJ. The Iflaviruses Sacbrood virus and Deformed wing virus evoke different transcriptional responses in the honeybee which may facilitate their horizontal or vertical transmission. PeerJ (2016) 4:e1591. doi: 10.7717/peerj.1591
31. Egekwu NI, Posada F, Sonenshine DE, Cook S. Using an in vitro system for maintaining Varroa destructor mites on Apis mellifera pupae as hosts: studies of mite longevity and feeding behavior. Exp Appl Acarol (2018) 74(3):301–15. doi: 10.1007/s10493-018-0236-0
32. Wilcoxon F. Individual comparisons by ranking methods. Biometrics Bull (1945) 1(6):80–3. doi: 10.2307/3001968
33. Highfield AC, El Nagar A, Mackinder LC, Noël LM, Hall MJ, Martin SJ, et al. Deformed wing virus implicated in overwintering honeybee colony losses. Appl Environ Microbiol (2009) 75(22):7212–20. doi: 10.1128/aem.02227-09
34. Schroeder DC, Martin SJ. Deformed wing virus. Virulence (2012) 3(7):589–91. doi: 10.4161/viru.22219
35. Meeus I, Mosallanejad H, Niu J, de Graaf DC, Wäckers F, Smagghe G. Gamma irradiation of pollen and eradication of Israeli acute paralysis virus. J Invertebr Pathol (2014) 121:74–7. doi: 10.1016/j.jip.2014.06.012
36. Shimanuki H, Knox DA, Herbert EW Jr. Fumigation with ethylene oxide to control diseases of honey bees1. J Econ. Entomo. (1970) 63(4): 1062–3. doi: 10.1093/jee/63.4.1062
37. Graystock P, Jones JC, Pamminger T, Parkinson JF, Norman V, Blane EJ, et al. Hygienic food to reduce pathogen risk to bumblebees. Invertebr Pathol (2016) 136:68–73. doi: 10.1016/j.jip.2016.03.007
38. Simone-Finstrom M, Aronstein K, Goblirsch M, Rinkevich F, de Guzman L. Gamma irradiation inactivates honey bee fungal, microsporidian, and viral pathogens and parasites. J Invertebrate Pathol (2018) 153:57–64. doi: 10.1016/j.jip.2018.02.011
39. Goblirsch M, Eakins J, Rowan NJ. Disease-mitigating innovations for the pollination service industry: Challenges and opportunities. Curr Opin Environ Sci Health (2021) 22:100265. doi: 10.1016/j.coesh.2021.100265
Keywords: Apis mellifera, DWV, IHP, infectivity, Varroa destructor
Citation: Cook SC, Ryabov EV, Becker C, Rogers CW, Posada-Florez F, Evans JD and Chen YP (2023) Deformed wing virus of honey bees is inactivated by cold plasma ionized hydrogen peroxide. Front. Insect Sci. 3:1216291. doi: 10.3389/finsc.2023.1216291
Received: 03 May 2023; Accepted: 18 July 2023;
Published: 02 August 2023.
Edited by:
Victoria Soroker, Agricultural Research Organization (ARO), IsraelReviewed by:
Qiang Huang, Jiangxi Agricultural University, ChinaMariana Bulgarella, Victoria University of Wellington, New Zealand
Copyright © 2023 Cook, Ryabov, Becker, Rogers, Posada-Florez, Evans and Chen. This is an open-access article distributed under the terms of the Creative Commons Attribution License (CC BY). The use, distribution or reproduction in other forums is permitted, provided the original author(s) and the copyright owner(s) are credited and that the original publication in this journal is cited, in accordance with accepted academic practice. No use, distribution or reproduction is permitted which does not comply with these terms.
*Correspondence: Steven C. Cook, c3RldmVuLmNvb2tAdXNkYS5nb3Y=