- 1Connecticut Agricultural Experiment Station, New Haven, CT, United States
- 2EcoData Technology, Plantsville, CT, United States
- 3Department of Entomology, Washington State University, Pullman, WA, United States
Detecting and monitoring populations of the invasive emerald ash borer (EAB) is crucial to successful management of the pest and evaluation of its ecological impacts. However, the beetle’s cryptic habit makes accurate monitoring costly and time-consuming. Biosurveillance takes advantage of the foraging effort of a predatory wasp Cerceris fumipennis (Hymenoptera: Crabronidae). This native, solitary, ground-nesting hunting wasp hunts adult buprestid beetles to provision its brood cells. By intercepting the hunting wasps, we can learn which species of buprestids are in the surrounding forest. The resulting data provides information on the presence and relative abundance of invasive buprestids like EAB which can supplement other monitoring efforts. In this paper we share results of ten years of biosurveillance surveys of the EAB in Connecticut. Among 112 sites, we observed EAB populations; from first detection, through the population peak and then through to the population crash, matching patterns observed in other regions of the United States. We also observed the spread of the EAB relative abundance as it moved through the state following an invasion front starting in New Haven, Co. The average time from first detection to population crash was nine years. On average, populations peaked three years after first detection, and remained at peak levels for three to four years. Population decline was gradual and took another three to four years. Notably, no evidence of a second introduction to Connecticut was seen with proportional abundance increasing over time after expanding outward from the introduction point. These results corroborate other traditional monitoring efforts in the eastern U.S. and provide independent validation of predicted population dynamics in ash stands.
1 Introduction
Emerald ash borer (Agrilus planipennis Fairmaire) is native to Far Eastern Asia and was first detected in North America in 2002 (1). Emerald ash borer (EAB) is a phloem-consuming buprestid beetle that feeds on trees the genus Fraxinus (Oleacea). In its native range, the beetle is considered a secondary pest, where it rarely infests ash trees that are healthy and instead thrives in trees with weakened anti-herbivore defenses resulting from decadence, disease, or drought (2). EAB’s feeding strategy is similar to North American species of Agrilus, such as Agrilus anxius Gory and Agrilus bilineatus (Weber), whose larvae typically feed on weakened hosts in the birch and oak families (3, 4). However, healthy trees of North American species of Fraxinus are successfully attacked by EAB (5). Emerald ash borer has little competition for healthy ash phloem in North America, and is not known to have any native, specialist natural enemies. Lack of bottom-up control by plant defenses and top-down control by natural enemies is thought to contribute to the exceptionally fast and devastating spread of this invasive insect across North America (1).
EAB population dynamics after arriving to a new location follow a simple exponential curve for the growth phase of the invasion, followed by a sharp drop in EAB population after the peak of the outbreak (Figure 1). The increasing portion of the exponential curve model has been validated for tree health in three urban areas by Sadof et al. (6), and many studies that use tree health as a proxy for EAB levels show similar curves (7, 8). Longer-term empirical data on the EAB population levels that move beyond the exhaustion of the main ash source have been collected largely in the context of monitoring the impact of biological control agents (9, 10). Understanding what is happening with the EAB population itself is important for long-term planning of ash management and recovery. While the population crash of EAB at a site is attributed to decline of adult ash trees, better monitoring efforts would assist in management of secondary outbreaks co-occurring with the recovery of a new cohort of ash trees.
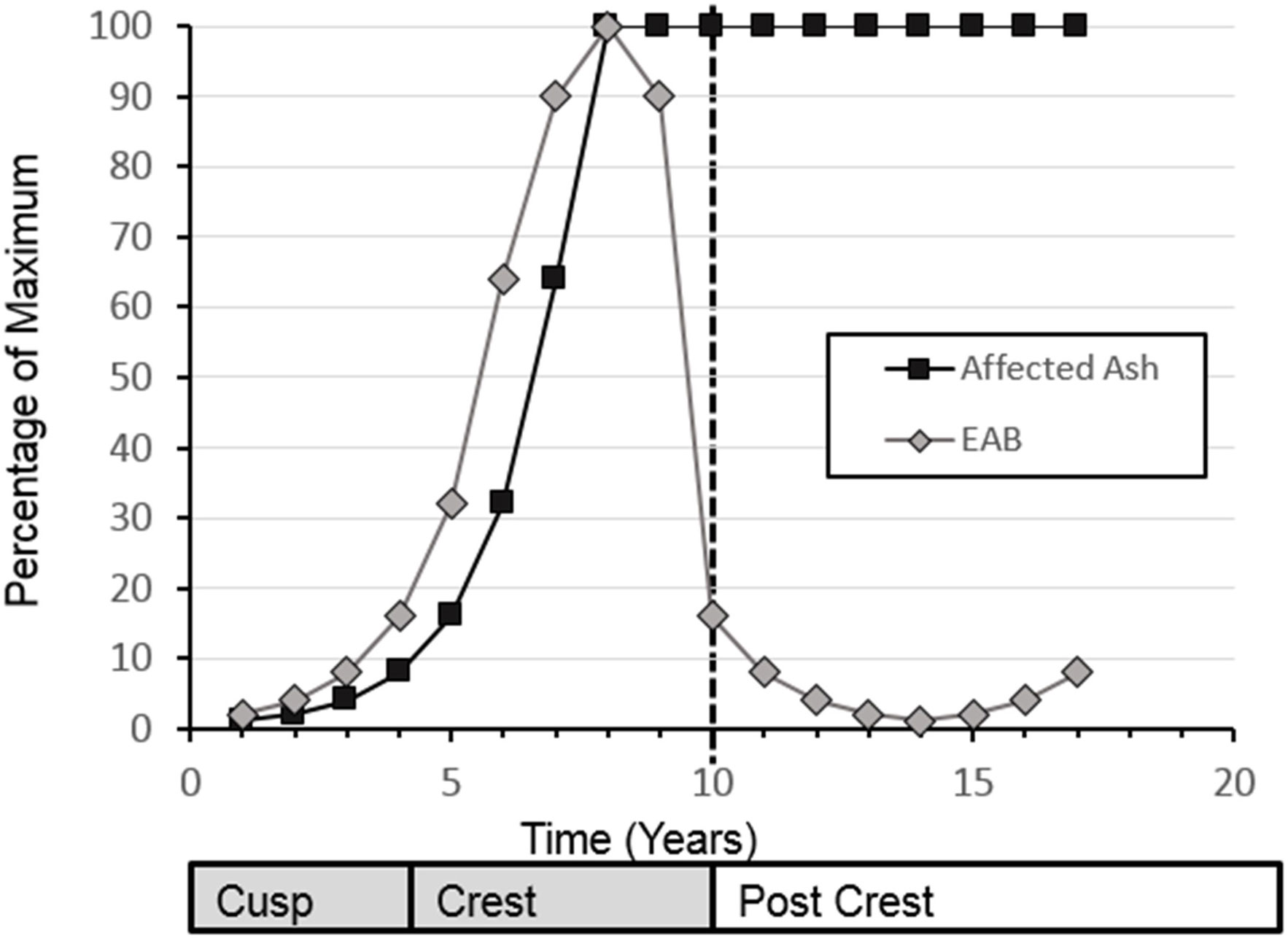
Figure 1 Conceptual diagram for emerald ash borer populations and impact on ash populations. Modified from Sadof.
Landscape-scale spread of EAB has been an important question in modelling efforts to track the regional spread of this invasive insect. Typically, the pattern seen throughout the beetles’ history in North America is that satellite populations emerge as the result of anthropogenic activity leading to an unintentional introduction (11). While models of EAB movement have predicted a range of spread rates, non-anthropogenic dispersal of EAB is typically thought to 1 to 2 km a year after initial invasion, and to increases as the population of EAB increases (12–14). These sites then gradually coalesce with the larger invasive population. Similar regional patterns of spread have been shown through detection surveys such as the USDA APHIS purple prism trap survey (15). Improved or alternative monitoring techniques, like biosurveillance can help to validate these observations of EAB dispersal and establishment.
Biosurveillance for EAB, is conducted using Cerceris fumipennis (16). This solitary ground-nesting wasp uses adult buprestid beetles to provision their larvae. The wasp is native to eastern North America and is broadly found from Maine to Florida and west to Texas (17). Each female wasp digs and provisions her own nest, but nests are usually found in aggregations of tens to hundreds of individual withing the same area (18). Male wasps emerge first in mid-June, and mate with female wasps as they emerge from their underground nest cells. Female wasps dig burrows and once they have dug the initial brood cell begin to hunt. Females live an average of 18 days (19) but can survive up to 6 weeks. They sequentially dig cells for their young, filling each with sufficient beetle mass to support a larva through its development. The female wasp will dig, provision, and lay a single egg in as many cells as they can. The number of their offspring is limited by the rate at which they can dig and provision the cells before they die. The larvae then complete their development feeding on the paralyzed beetles and spin a cocoon (20). In the northern part of their range, including Connecticut, they overwinter as pre-pupae in their cocoons. Diapause is not obligate, and in the southern portions of its range, C. fumipennis has 2 generations a year (20) and infrequent second emergences have been observed in Connecticut (21).
Like many solitary wasps, Cerceris fumipennis preys on a single insect taxonomic group, adult beetles in the family Buprestidae. Over 100 species of buprestid beetles have been recorded as Cerceris fumipennis prey (22–25). In rare instances (< 0.1% of records) Cerceris fumipennis captures Cerambycidae and Chrysomelidae prey items (26). Otherwise, prey seems to be limited to buprestids that have an arboreal habitat as adults, are adults during the wasps’ hunting season, and are between 4-20 mm in length (27, 28).
Foraging wasps forage to maximize the mass of beetles collected for amount of effort (29). Wasps typically capture the most readily available prey rather than searching for specific prey species. Consequently, changes in the relative abundance of available prey are likely to be reflected in the abundance of species in the captured prey. Swink et al. (22) demonstrated changes in prey types for C. fumipennis in North Carolina during an outbreak of the hemlock borer, Phaenops fulvuguttata (Coleoptera: Buprestidae). Hemlocks stressed by Adelges tsugae (Hemiptera: Adelgidae) caused a proportional increase in hemlock borers as wasp prey. Changes in proportions of prey capture are demonstrated in other solitary hunting wasps as well including, Hypodynerus andeus (Packard) (30) and Sphex ichneumoneus (L.) (31).
In this paper we take advantage of data from 10 years of monitoring prey catch of Cerceris fumipennis (Hymenoptera: Crabronidae) to examine of the proportional abundance of EAB to other native buprestid insects among 112 sites in the state of Connecticut. These survey efforts have amassed a collection of over 30,000 individual buprestids that have been collected from forest fragments invaded by EAB. Due to high coverage within the state of Connecticut, these data can be used to map the spread of EAB in Connecticut since its introduction in New Haven Co., Connecticut. The aim of this study is to evaluate how informative the relative proportions of EAB in the prey of C. fumipennis colonies are for tracking EAB population levels. The resultant data examines the temporal history of the EAB infestation at single locations, as well as the spatial pattern of EAB distribution over time in Connecticut.
2 Materials and methods
2.1 Collection sites
Aggregations of Cerceris fumipennis were identified by surveys of baseball fields throughout Connecticut. Baseball fields are the most common habitat for the wasp where they aggregate in the sandy soils within foraging range of forests (18). In these surveys, new aggregations of C. fumipennis were added each year, and others were dropped as the numbers of wasps in colonies fluctuated.
The beetles were collected in June, July, and August from 2009-2022. At least 50 beetles per site per year was the goal for the survey, as Careless et al. (16) found that this number was a good compromise between detection of as many species of beetle as possible at a site, and available time. Beetles were collected by netting and releasing wasps carrying prey or by collecting beetles abandoned on the field by the wasps. (32, 33).
2.2 Proportion of EAB as related to time of EAB detection
For this analysis, a subset of sites that had greater than 30 beetles collected in the year before initial EAB detection and 50 beetles collected at the site for one or more years subsequent to the initial detection of Emerald Ash Borer at that site, were selected. For each site and year, the proportion of EAB/total beetles was calculated and classified by year relative to EAB detection, 0 = the year of detection, 1 = the year after detection, etc. regardless of what calendar year that detection occurred. 2012 provides the first records of EAB in these surveys, therefore data from 2009-2011 was not included in analyses. For hypothesis testing, we used a conceptual diagram as point of qualitative comparison between an expected curve of EAB populations (Figure 1) to our data collected.
2.2.1 Modelling proportion of EAB against time of EAB detection
Proportion of EAB as a function of time of EAB detection was modelled using a generalized linear mixed effects model with a logit link. The full fixed effects model included time and time2, and the full random effects model included random slopes over time and time2 and random intercepts for collection site. Random effect selection was performed by comparing the full model to three simpler models (random intercepts and random slopes over time, random slopes over time only, and random intercepts only) based on (lowest) AIC (34). Modelling and model selection were conducted in R version 4.1.2 (35) using the lme4 and lmerTest packages, with model diagnosis as per Quinn & Keough (34). 95% confidence intervals were obtained using the ggeffects package version 1.1.4, and results were plotted using ggplot2 version 3.4.1.
2.3 Spatial analysis of EAB spread
For each year, starting in 2012 when EAB was first detected in Connecticut, we calculated the proportion of EAB in C. fumipennis prey by colony. Data from sites where less than 20 beetles were collected that year were excluded. We calculated Moran’s I to reveal significant spatial autocorrelation between all sites in each year (36). For visualizing the distribution of EAB proportional abundance in C. fumipennis colonies, we used Empirical Bayesian Kriging tool in ArcPro (36). In this approach, interpolated proportions between collection sites construct a series of maps, one per year, that show the density of EAB by color gradient.
3 Results
The first detection of emerald ash borer in Connecticut was made using C. fumipennis in 2012 in the town of Prospect, CT (33). Since 2012, EAB has been detected in all eight counties of Connecticut, and in 166 of its 169 towns. For three of those counties the first detection was made by C. fumipennis, as were 99 of the town detections (Supplemental Data Figure 1).
3.1 Proportion of EAB as related to time of initial EAB detection
We had 48 sites for which we had a known first EAB detection year. This was defined as a site at which more than 30 beetles (X = 70.9 ± 5.25, Median = 66) were collected directly prior to the year EAB was first collected. Three sites which date from our first detections of EAB in 2012 reached the threshold of 10 years post-detection as of 2021, therefore encapsulating the range of time predicted for population rise and fall in wave front models (modified as conceptual diagram in Figure 1). Altogether 15,601 beetles are in the data set that was used to create this curve (Figure 2).
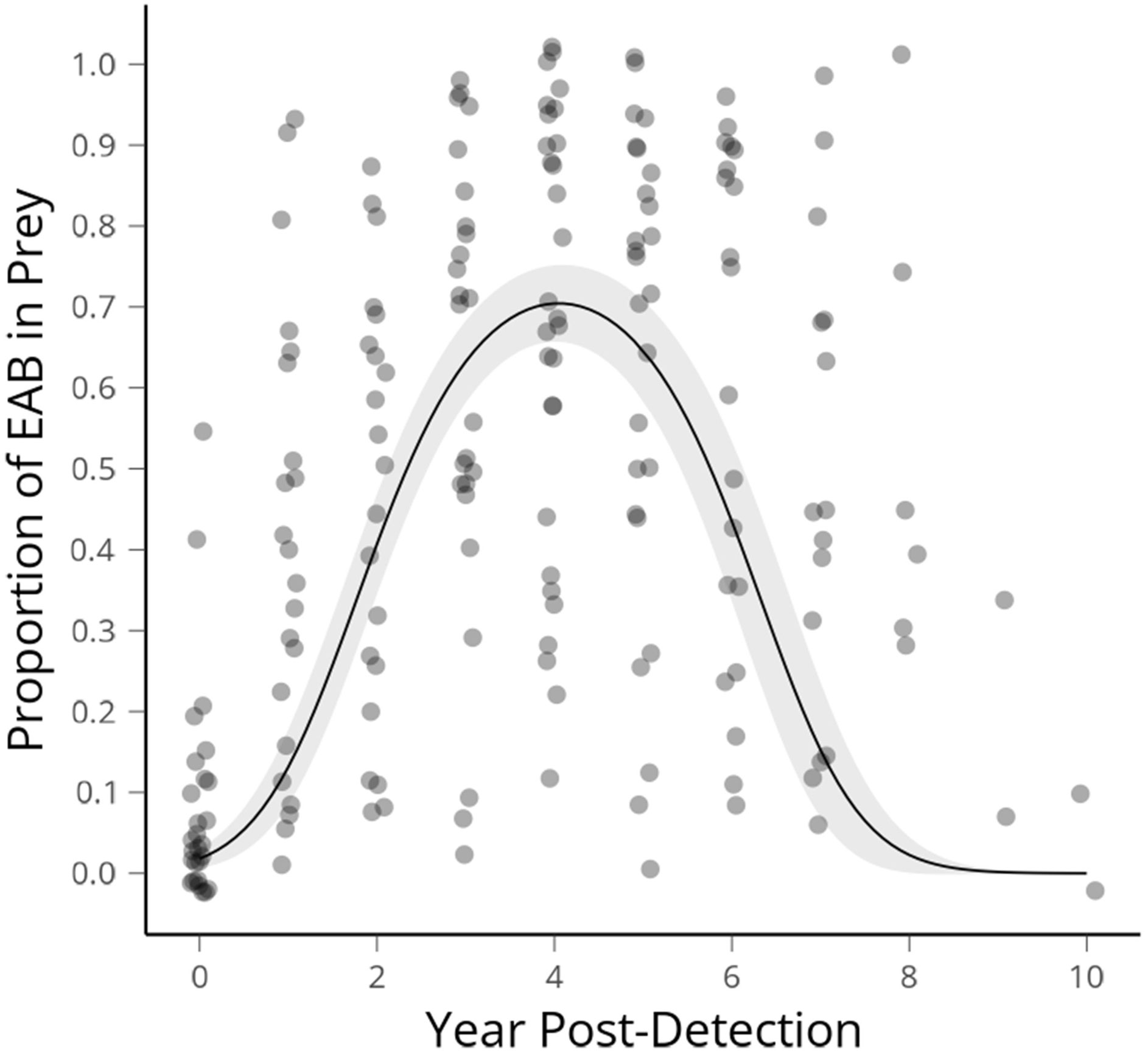
Figure 2 Average proportion of emerald ash borer in Cerceris fumipennis prey by year of detection, with the peak occurring at 4.05 years. The first year EAB is detected at a site is year 0, the next year 1 etc. Ribbon shows 95% confidence interval.
Average proportion of EAB in C. fumipennis prey predicted according to our GLMM had a peak occurring at 4.05 years. The span of arrival to population crash of EAB, as indicated by the proportional abundance of catches, spanned eight-ten years. The proportion of EAB collected in year zero was 0.108 (SE ± 0.026). If we assume the population starts at 1% and doubles each year, as in the theoretical model, that puts detection by C. fumipennis, on average, 4 to 5 years into the invasion. There was a range of EAB proportions from 0.01 – 0.52 in the first detection year. The mean proportion of EAB in C. fumipennis prey increased for 3 years after the initial detection. The proportion of EAB plateaued for the next 3 years, and then, starting 7 years after the initial detection, the proportion of EAB in wasp prey declined reaching an average of 0.108 (SE ± 0.07) by year 9.
3.2 Spatial analysis of EAB spread
The number of sites on which the mapping was based in each year averaged 56 (SE ± 4.01, range 35 – 77). In 2020 – 2021 there were fewer sites surveyed due to difficulty recruiting volunteers during COVID quarantines, and unfavorable weather conditions. This was especially true in the north-eastern corner of the state. The resultant density predictions should be taken with caution for that part of the state in those years. Moran’s I, a measure of autocorrelation in spatial data, was significant in each year (Table 1), indicating that the data were good candidates for Empirical Bayesian Kriging. The models of EAB proportion across the landscape of Connecticut generated by Empirical Bayesian Kriging are shown in Figure 3. Statistics for Empirical Bayesian Kriging are available in the Supplemental Data Table 1.
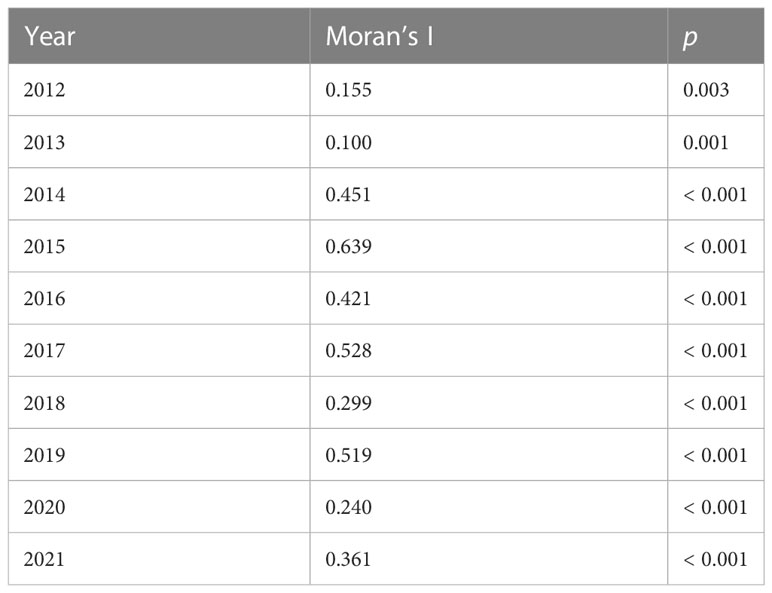
Table 1 Moran’s Index of spatial autocorrelation for the proportion of emerald ash borer in Cerceris fumipennis prey by year.
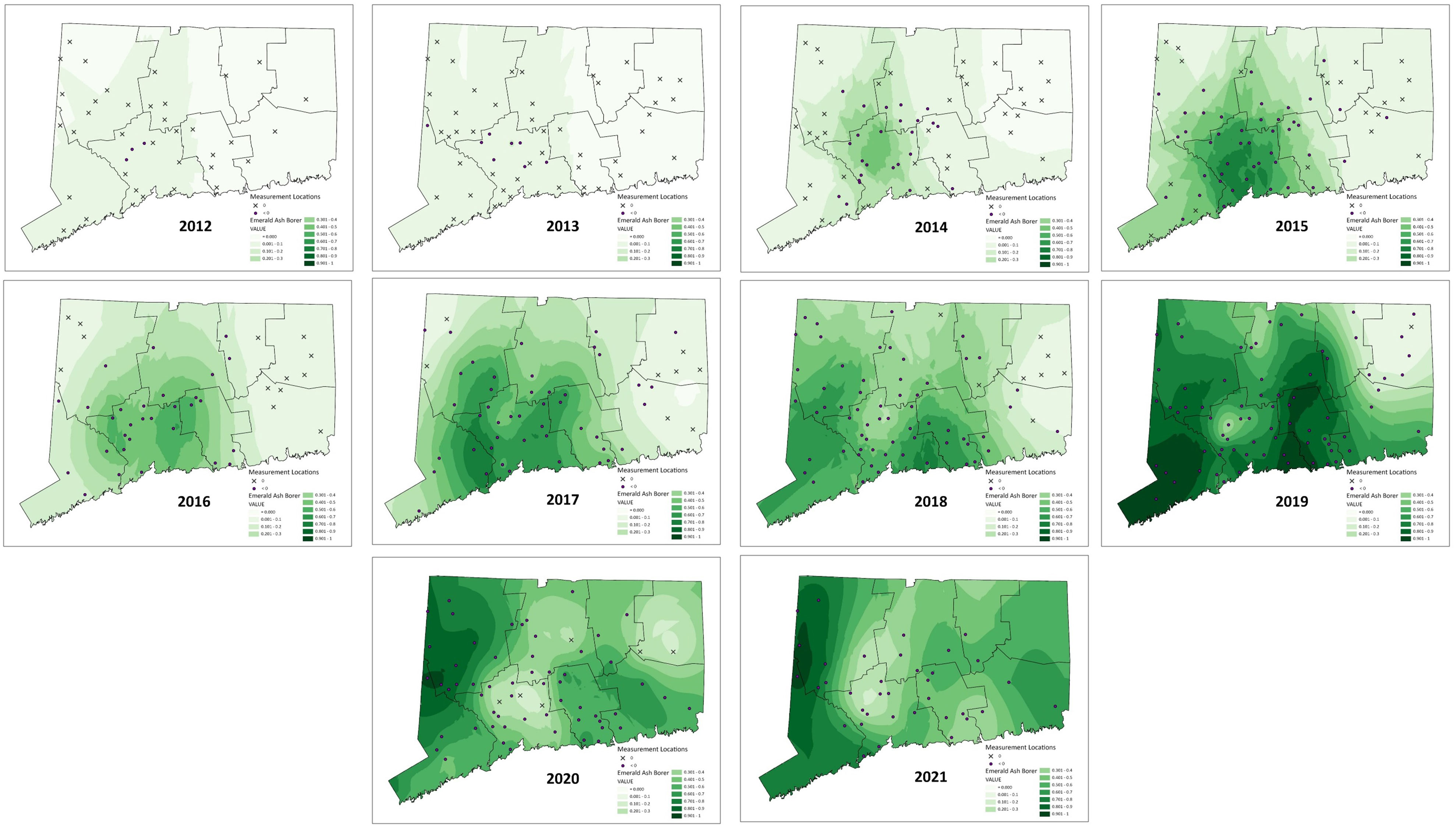
Figure 3 Maps of emerald ash borer density using the proportion of EAB in Cerceris fumipennis prey as a proxy. For each year, sites with over 20 beetles sampled that year are marked. Those marked with an ✕ are sites where no EAB were collected that year. Those marked with a purple circle represent sites where EAB was collected. The darker the green the higher the proportion of EAB found. Interpolation was done using Empirical Bayesian Kriging (36).
4 Discussion
Detection of cryptic invasive species is critical for management. However, monitoring, especially monitoring that extends beyond initial detection, is seldom sustained long-term due to the difficulty of sampling. In this study we used Cerceris fumipennis prey capture as a proxy measurement for EAB presence and prevalence. This has allowed us to document both the temporal and spatial changes of EAB populations over the 10 years since EAB was detected in Connecticut.
Temporally, the rise of EAB as a proportion of prey captured by C. fumipennis, roughly mirrors the theoretical model. On average, the proportion of EAB in C. fumipennis prey was 8.7% in the first year of detection. This is consistent with detection at year 5 of the infestation and is the threshold at which infestations are commonly detected by trapping (6, 33). The proportion of EAB in C. fumipennis prey increased year over year, plateaued for 3 years, and then decreased. One striking difference when comparing the conceptual diagram of EAB populations to our results is the prolonged crest and gradual drop in EAB density. The theoretical curve drops steeply one year after the peak as it posits that all available ash has been exhausted. However, the sudden population drop seen in many eruptive forest pests, such as Lymantria dispar dispar (L.), is driven not only by decreases in food quality and availability, but by increasing predation by generalist predators, and by epizootics (37, 38). While food availability, and generalist predation, primarily by woodpeckers and generalist parasitoids of woodborers, are likely drivers of EAB population decline (39, 40), there is as-of-yet no evidence of epizootics impacting EAB in North America (41). The lack of specialized pathogens may explain the more gradual population descent seen in the C. fumipennis-based data.
Alternatively, the prolonged crest and gradual population decline seen in our data could be due to an averaging effect across many sites. There was variation between sites and years in these patterns and several factors that could be contributing to that variation. Ash is not distributed equally across the landscape. Although we did not test this explicitly it seems likely the density of ash in the 1.5 km foraging range of each C. fumipennis site (42) will impact both the peak proportion of EAB at the site, and the length of that peak. Another source of variation between sites is the timing of data collection each year. In Connecticut, C. fumipennis hunting season typically starts in mid-late June, with a peak during the first 2 weeks of July and lasting into August. Emerald ash borer, by contrast, typically emerges in early June and adult numbers tend to drop off by mid-July. Thus, sites for which a year’s data was collected in late July is likely to have a lower proportion of EAB in their catch than sites for which a year’s data was collected in early July. Finally, there were sites at which EAB was detected early or late in the infestation. For example, we had a site for which EAB had been detected in neighboring sites ringing it, but no EAB prey was detected at this site for 2 years. In contrast, we had sites for which EAB was detected one year, and it was then another 3 years before another EAB was caught. However, despite these factors, the aggregated data clearly reflect population patterns of EAB.
We were also able to obtain data on not only the spatial distribution of detection of EAB as it moved through the state, but its density at each site in each year it was sampled. We could then use empirical Bayesian kriging to generate maps to provide a clear picture of the invasion ‘wave’ as it moved across the state. The data clearly show the beetle spreading out in a radial fashion from its presumptive invasion site in northern New Haven County. The density dynamics are as expected, in each new area the density is at first low, and then quickly increases before gradually decreasing. Another pattern that is shown is that there was likely only one successful establishment of EAB in the state. At no point do we see loci of high EAB density that are not consistent with spread from the original detection area of northern New Haven County. This spatially explicit information can be used to assist managers to tailor EAB responses.
Future work will look toward understanding the movement we have seen in the light of more explicit landscape analysis. Factors to be investigated include impact of local ash density on rate of spread, and the presence of barriers to or corridors for EAB movement. Continued monitoring of C. fumipennis colonies is planned. Of particular interest is the dynamics of EAB populations in post-crest areas as the ash resource begins to recover.
Data availability statement
The raw data supporting the conclusions of this article will be made available by the authors, without undue reservation.
Author contributions
CR conceived of and designed of study. RC performed statistical analysis. CR wrote the first draft of the manuscript. RC wrote sections of the manuscript. All authors contributed to manuscript revision, read, and approved the submitted version.
Funding
Financial support was provided by USFS Forest Health Program (#13-DG-11420004-155), USDA Plant Protection Act 7721(#AP21PPQFO000C036, #AP20PPQFO000C033, and #AP19PPQO000190), and CAPS funding.
Acknowledgments
This data set is the result of the hard work and support of many people and agencies. A tremendous amount of fieldwork was spearheaded by the Wasp Watchers of Connecticut, providing half of the beetle data included in this analysis. Critical assistance by seasonal workers, J. Fitzgerald, J. Tate, E. Kim, D. Hill, S., A. Kahn was provided in curation and data entry. M. Scott deserves special recognition as the primary collector, identifier and curator of the buprestid collection. A. Blake (EcoData Technology) aided with logistic regression models. Many thanks to G. Molaei and J. White and two reviewers for helpful comments on this manuscript. Finally, S. Stebbins has lent much GIS support to the project.
Conflict of interest
Author RC was employed by company EcoData Technology.
The remaining author declares that the research was conducted in the absence of any commercial or financial relationships that could be construed as a potential conflict of interest.
Publisher’s note
All claims expressed in this article are solely those of the authors and do not necessarily represent those of their affiliated organizations, or those of the publisher, the editors and the reviewers. Any product that may be evaluated in this article, or claim that may be made by its manufacturer, is not guaranteed or endorsed by the publisher.
Supplementary material
The Supplementary Material for this article can be found online at: https://www.frontiersin.org/articles/10.3389/finsc.2023.1179368/full#supplementary-material
References
1. Herms D, McCullough D. Emerald ash borer invasion of north America: history, biology, ecology, impacts, and management. Annu Rev Entomol (2014) 59(1):13–30. doi: 10.1146/annurev-ento-011613-162051
2. Wang XY, Yang ZQ, Gould JR, Zhang YN, Liu GJ, Liu ES. The biology and ecology of the emerald ash borer, Agrilus planipennis, in China. J Insect Sci (2010) 10(1):1–23. doi: 10.1673/031.010.12801
3. Haack R, Benjamin D. The biology and ecology of the twolined chestnut borer, Agrilus bilineatus (Coleoptera: buprestidae), on oaks, quercus spp., in Wisconsin. Can Entomol (1982) 114(5):385–96. doi: 10.4039/Ent114385-5
4. Muilenburg V, Herms D. A review of bronze birch borer (Coleoptera: buprestidae) life history, ecology, and management. Environ Entomol (2012) 41(6):1372–85. doi: 10.1603/EN12238
5. Rebek E, Herms D, Smitley D. Interspecific variation in resistance to emerald ash borer (Coleoptera: Buprestidae) among north American and Asian ash (Fraxinus spp.). Environ Entomol (2008) 37(1):242–6. doi: 10.1603/0046-225X(2008)37[242:IVIRTE]2.0.CO;2
6. Sadof CS, Hughes GP, Witte AR, Peterson DJ, Ginzel MD. Tools for staging and managing emerald ash borer in the urban forest. Arboric Urban For (2017) 43:15–26. doi: 10.48044/jauf.2017.002
7. Klooster WS, Herms DA, Knight KS, Herms CP, McCullough DG, Smith A, et al. Ash (Fraxinus spp.) mortality, regeneration, and seed bank dynamics in mixed hardwood forests following invasion by emerald ash borer (Agrilus planipennis). Biol Invasions (2014) 16:859–73. doi: 10.1007/s10530-013-0543-7
8. Miller F, Mueller D. Protection of ash trees under extended emerald ash borer pressure. Gt Lakes Entomol (2020) 53(1):4.
9. Duan J, Bauer L, Van Driesche R. Emerald ash borer biocontrol in ash saplings: the potential for early stage recovery of north American ash trees. For Ecol Manage (2017) 394:64–72. doi: 10.1016/j.foreco.2017.03.024
10. Duan JJ, Van Driesche RG, Crandall RS, Schmude JM, Rutledge CE, Slager BH, et al. Establishment and early impact of Spathius galinae (Hymenoptera: braconidae) on emerald ash borer (Coleoptera: buprestidae) in the northeastern united states. J Econ Entomol (2019) 112(5):2121–30. doi: 10.1093/jee/toz159
11. Mercader R, Siegert N, Liebhold A, McCullough D. Dispersal of the emerald ash borer, Agrilus planipennis, in newly-colonized sites. Agric For Entomol (2009) 11(4):421–4. doi: 10.1111/j.1461-9563.2009.00451.x
12. Sargent C, Raupp M, Bean D, Sawyer A. Dispersal of emerald ash borer within an intensively managed quarantine zone. Arboric Urban For (2010) 36(4):160–3. doi: 10.48044/jauf.2010.021
13. Siegert NW, McCullough DG, Liebhold AM, Telewski FW. Dendrochronological reconstruction of the epicentre and early spread of emerald ash borer in north America. Divers Distrib (2014) 20(7):847–58. doi: 10.1111/ddi.12212
14. Siegert NW, Mercader RJ, McCullough DG. Spread and dispersal of emerald ash borer (Coleoptera: buprestidae): estimating the spatial dynamics of a difficult-to-detect invasive forest pest. Can Entomol (2015) 147(3):338–48. doi: 10.4039/tce.2015.11
15. USDA APHIS. Emerald ash borer infestation time series spread map (2022). Available at: https://www.youtube.com/watch?v=6fo2Sf_8Iug.
16. Careless P, Marshall S, Gill B. The use of Cerceris fumipennis (Hymenoptera: crabronidae) for surveying and monitoring emerald ash borer (Coleoptera: buprestidae) infestations in eastern north America. Can Entomol (2014) 146(1):90–105. doi: 10.4039/tce.2013.53
17. Krombein KHP, Smith D, Burks B. Catalog of hymenoptera in America north of Mexico. Washington, DC: Smithsonian Institution Press (1979).
18. Nalepa CA, Teerling C, Rutledge CE, Swink W, Arellano C. Ball diamonds as habitat for nests of Cerceris fumipennis (Hymenoptera: crabronidae): comparisons among three states. J Kansas Entomological Soc (2012) 85(3):219–25. doi: 10.2317/JKES120418.1
19. Nalepa C. Wing wear is a poor estimate of age in Cerceris fumipennis (Hymenoptera, crabronidae). J Hymenoptera Res (2012) 24:43. doi: 10.3897/jhr.24.2091
20. Hook A, Evans H. Prey and parasites of Cerceris fumipennis (Hymenoptera: sphecidae) from central Texas, with description of the larva of Dasymutilla scaevola (Hymenoptera: mutillidae). J Kansas Entomological Soc (1991) 64(3):257–64. Available at: https://www.jstor.org/stable/25085285.
21. Rutledge C, Fierke M, Careless P, Teerling C. Degree-day model for emergence of Cerceris fumipennis (Hymenoptera: crabronidae) in northeastern America based on field observations. Ann Entomological Soc America. (2015) 108(6):971–7. doi: 10.1093/aesa/sav082
22. Swink W, Paiero S, Nalepa C. Buprestidae collected as prey by the solitary, ground-nesting philanthine wasp Cerceris fumipennis (Hymenoptera: crabronidae) in north Carolina. Ann Entomological Soc America (2013) 106(1):111–6. doi: 10.1603/AN12113
23. Brownie C, Virgets W, Allison JD, Johnson CW, MacRae TC. Observations of Cerceris fumipennis (Hymenoptera: crabronidae) phenology and variation in its buprestid prey in Louisiana. Florida Entomol (2015) 98(4):1106–13. doi: 10.1653/024.098.0415
24. Bohne MJ, Rutledge CE, Hanson T, Carrier NC, Teerling C, Weimer J, et al. Utilizing prey captures by Cerceris fumipennis say (Hymenoptera: crabronidae) for a survey of buprestidae (Coleoptera) in new England, USA. Coleopterists Bull (2019) 73(2):369–79. doi: 10.1649/0010-065X-73.2.369
25. Hallinen M, Wittman J, Aukema BH. Factors associated with diversity and distribution of buprestid prey captured by foraging Cerceris fumipennis (Hymenoptera: crabronidae). Environ Entomol (2020) 49(6):1363–73. doi: 10.1093/ee/nvaa114
26. Rutledge C, Hellman W, Teerling CF. Two novel prey families for the buprestid-hunting wasp Cerceris fumipennis say (Hymenoptera: crabronidae). Coleopterists Bull (2011) 65(2):194–6. doi: 10.1649/072.065.0223
27. Volkova T, Matthews R, Barber M. Spider prey of two mud dauber wasps (Hymenoptera: sphecidae) nesting in georgia's okefenokee swamp. J Entomological Sci (1999) 34(3):322–7. doi: 10.18474/0749-8004-34.3.322
28. Hellman W, Fierke M, Erbilgin N. Evaluating buprestid preference and sampling efficiency of the digger wasp, Cerceris fumipennis, using morphometric predictors. J Insect Sci (2014) 14(1):4. doi: 10.1093/jis/14.1.4
29. Pyke G. Optimal foraging theory: an introduction. In: Encyclopedia of animal behavior. Amsterdam, NE: Elsevier Academic Press (2019). p. 111–7.
30. Vargas H, Vargas-Ortiz M, Huanca-Mamani W, Hausmann A. Prey identification in nests of the potter wasp Hypodynerus andeus (Packard) (Hymenoptera, vespidae, eumeninae) using DNA barcodes. Rev Bras Entomologia (2014) 58:157–60. doi: 10.1590/S0085-56262014000200007
31. Brockmann H. Provisioning behavior of the great golden digger wasp, Sphex ichneumoneus (L.) (Sphecidae). J Kansas Entomological Soc (1985) 58(4):631–55. Available at: https://www.jstor.org/stable/25084707.
32. Carrier N, Jackson L. Cerceris wasp survey protocol. USDA-APHIS-PPQ (2012) p. 30. Available at: http://download.ceris.purdue.edu/file/2582.
33. Rutledge C, Fierke M, Careless P, Worthley T. First detection of Agrilus planipennis in Connecticut made by monitoring Cerceris fumipennis (Crabronidae) colonies. J Hymenoptera Res (2013) 32. doi: 10.3897/jhr.32.4865
34. Quinn GP, Keough MJ. Experimental design and data analysis for biologists. Cambridge, UK: Cambridge University Press (2002).
35. R Core Team. R: a language and environment for statistical computing. Vienna, Austria: R Foundation for Statistical Computing (2021).
37. Elkinton JS. Population dynamics of gypsy moth in north America. Annu Rev Entomol (1990) 35:517–96. doi: 10.1146/annurev.en.35.010190.003035
38. Hoch G, Zubric M, Novotny J, Schopf A. The natural enemy complex of the gypsy moth, Lymantria dispar (Lepidoptera, lymantriidae) in different phases of its population dynamics in eastern Austria and Slovakia, a comparative study. J Appl Entomol (2001) 125:217–27. doi: 10.1046/j.1439-0418.2001.00540.x
39. Jennings D, J.R. JD, Vandenberg JD, Duan JJ, Shrewsbury PM. Quantifying the impact of woodpecker predation on population dynamics of the emerald ash borer (Agrilus planipennis). PLoS One (2013) 8:12. doi: 10.1371/journal.pone.0083491
40. Jennings D, Duan J, Shrewsbury P. Biotic mortality factors affecting emerald ash borer (Agrilus planipennis) are highly dependent on life stage and host tree crown condition. Bull Entomological Res (2015) 105(5):598–606. doi: 10.1017/S0007485315000498
41. Castrillo LA, Bauer LS, Liu H, Griggs MH, Vandenberg JD. Natural infections of beauveria bassiana in agrilus planipennis populations in Michigan. MD, USDA Research Forum on Invasive Species: Annapolis (2009).
Keywords: biosurveillance, invasive species, Agrilus Planipennis, monitoring, temporal dynamics, spatial dynamics
Citation: Rutledge CE and Clark RE (2023) Temporal and spatial dynamics of the emerald ash borer invasion in Connecticut as shown by the native digging wasp Cerceris fumipennis (Hymenoptera: Crabronidae). Front. Insect Sci. 3:1179368. doi: 10.3389/finsc.2023.1179368
Received: 04 March 2023; Accepted: 25 April 2023;
Published: 15 May 2023.
Edited by:
Vivek Srivastava, University of British Columbia, CanadaReviewed by:
Celia Boone, British Columbia Ministry of Forests, Lands, Natural Resource Operations and Rural Development, CanadaDebra Wertman, University of British Columbia, Canada
Copyright © 2023 Rutledge and Clark. This is an open-access article distributed under the terms of the Creative Commons Attribution License (CC BY). The use, distribution or reproduction in other forums is permitted, provided the original author(s) and the copyright owner(s) are credited and that the original publication in this journal is cited, in accordance with accepted academic practice. No use, distribution or reproduction is permitted which does not comply with these terms.
*Correspondence: Claire E. Rutledge, Y2xhaXJlLnJ1dGxlZGdlQGN0Lmdvdg==