- 1Department of Entomology, Cornell University, Ithaca, NY, United States
- 2Emerging Pests and Pathogens Research, USDA-Agricultural Research Service, Ithaca, NY, United States
- 3Jaronski Mycological Consulting LLC, Blacksburg, VA, United States
The entomopathogenic fungus Beauveria bassiana is cosmopolitan and known to infect a variety of sap-sucking pests like aphids, mealybugs, and scales in the order of Hemiptera. In Fall 2017, spotted lanternfly (SLF) adults killed by the fungal entomopathogen B. bassiana were found in Berks County, Pennsylvania. In 2018-2020 we collected SLF and nearby non-target insects killed by Beauveria spp. from 18 field sites in southeastern Pennsylvania. We identified 159 Beauveria isolates from SLF and six isolates from non-targets. Five isolates of B. bassiana and one isolate of B. brongniartii were identified from the non-targets. Based on sequence data from the nuclear B locus (Bloc) intergenic region, all the isolates from SLF were identified as B. bassiana, but there were 20 different strains within this species, grouped into two clades. Three B. bassiana strains (A, B, and L) were found in most field sites and were the most prevalent. Representative isolates for these three strains were used in laboratory bioassays and were compared to a commercial B. bassiana strain (GHA). Strain B was inferior to A, L, and GHA against nymphs; strains A and L had greater efficacy than B and GHA against adults. We also quantified conidial production on SLF cadavers. This paper discusses the diversity of these B. bassiana strains in SLF populations and implications for biological control of this abundant invasive.
Introduction
Entomopathogenic fungi infect a diversity of insects but are well known as acute pathogens of hemipterans, including aphids, leafhoppers and planthoppers (1, 2). Beauveria bassiana (Bals.-Criv.) Vuill. (Hypocreales: Cordycipitaceae) is a well-studied entomopathogen (3, 4) and has been reported causing epizootics (sometimes as part of a complex of entomopathogens) in hemipteran pests, including the chinch bug (Blissus leucopterus (Say) [Hemiptera: Blissidae]) (5), elongate hemlock scale (Fiorinia externa (Ferris) [Hemiptera: Diaspididae]) (6), and kudzu bug (Megacopta cribraria (Fabricius) [Hemiptera: Plataspidae]) (7). In 2019, a co-epizootic caused by two native fungal pathogens, B. bassiana and Batkoa major (Thaxt.) Humber (Entomophthorales: Entomophthoraceae), was reported in two populations of the new invasive planthopper, the spotted lanternfly (SLF), Lycorma delicatula (White) (Hemiptera: Fulgoridae), in southeastern Pennsylvania (8). Clifton et al. (9) discovered two more species of hypocrealean fungi infecting SLF that are assumed to be native: Metarhizium pemphigi (Driver & R.J. Milner) (Hypocreales: Clavicipitaceae) and the new species Ophiocordyceps delicatula (Hypocreales: Ophiocordycipitaceae).
The genus Beauveria includes more than 12 cryptic species that cannot be identified with morphological characters alone (10–12). Beauveria isolates can be identified to species by sequencing the nuclear B locus (Bloc) intergenic region and other genes (12, 13). Previous studies describing Beauveria isolates that naturally infected invasives like the emerald ash borer (Agrilus planipennis Fairmaire [Coleoptera: Buprestidae]) (14) and coffee berry borer (Hypothenemus hampei (Ferrari) [Coleoptera: Curculionidae]) (13, 15) discovered multiple cryptic species and a wide assemblage of B. bassiana strains. Additional studies on these invasives involved bioassays and demonstrated how some native isolates of B. bassiana had greater virulence than a commercialized strain (GHA) and produced more conidia on their cadavers, which is indicative of greater epizootic potential (14, 16).
SLF is a new invasive, univoltine planthopper that was first discovered in Berks County, Pennsylvania in 2014 (17) and has spread to 13 additional US states (18). The native range of SLF includes China, Taiwan, and Vietnam, and SLF can be a sporadic pest in China feeding on tree of heaven, Ailanthus altissima (Mill.) Swingle (Sapindales: Simaroubaceae) (19, 20). SLF prefers tree of heaven which is invasive in North America, but will feed on wild and cultivated grapes (Vitis spp. [Vitales: Vitaceae]) as well as other woody plants; this species is now infamous for its voracious feeding, which has caused reduced productivity and mortality in grapevines (21–23). These impacts, as well as others on trees such as black walnut, Juglans nigra L. (Fagales: Juglandaceae) and red maple, Acer rubrum L. (Sapindales: Sapindaceae) (24–26), have resulted in the need for means of controlling this harmful insect. Eradication of SLF seems unlikely and long-term management tools, including natural enemies like entomopathogenic microorganisms, could help control this invasive species (27–29).
Mycoinsecticides containing different B. bassiana strains are available for commercial use in the United States (30). In field trial applications, BoteGHA (Certis USA; containing B. bassiana strain GHA) killed 43-48% of SLF nymphs and adults infesting A. altissima in a public park (31). Laboratory bioassays testing mycoinsecticides found that Cordyceps javanica (Frieder. & Bally) Kepler, B. Shrestha & Spatafora (Hypocreales: Cordycipitaceae) was less effective than B. bassiana, but all B. bassiana-based products had similar efficacy against SLF of different ages (32).
In 2018-2020 we conducted parallel studies on the genetic diversity of naturally occurring B. bassiana (this study) and B. major (33) that infect SLF. The goals of the current study were to (1) isolate and identify Beauveria spp. infecting SLF and non-targets, predominantly collected in natural forested areas and edge habitats (e.g., tree lines in a neighborhood), in southeastern Pennsylvania, and (2) describe the prevalence and distribution of indigenous B. bassiana strains in these areas invaded by SLF. This study was used to select indigenous B. bassiana strains for laboratory bioassays carried out in 2021 with potential for use in biological control of this new invasive pest.
Materials and methods
Sample collection
We collected fungal isolates from dead SLF or non-targets, mostly on the ground and at bases of trees, in Pennsylvania, USA. The first B. bassiana sample in this study was sent to our laboratory in August 2017 (site a, Table 1; Figure 2). In May 2018, we found four SLF adult cadavers with B. bassiana outgrowth beneath leaf litter in site b (Table 1; Figure 2). Although these SLF adults died in Fall 2017, we isolated these B. bassiana samples in 2018. In 2018-2020, we opportunistically sampled Beauveria spp. associated with SLF and non-target insects in 17 more sites among 5 counties in southeastern Pennsylvania (Table 1; Figure 2). Most of the sampling occurred in Berks County, Pennsylvania. Dense populations of SLF were mostly restricted to municipalities in and around eastern Berks County in 2014-2016. We only sampled sites around Lancaster and Philadelphia in 2020, mainly because SLF populations had only recently established there, and the Pennsylvania counties with these sites were added to the SLF quarantine in 2018 (34).
For most of the Beauveria-infected SLF and non-target insects, we collected cadavers that already had fungal outgrowth that is characteristic for Beauveria spp. We identified non-target insects to family, genus, or species with a dissecting microscope following dichotomous keys for morphological characters. In some cases, we obtained isolates from SLF or non-target cadavers that had no fungal outgrowth at the time of sampling but later produced conidia after incubation on 1.5% water agar for 5-10 days in the laboratory. For non-target insects that already had fungal outgrowth at the time of sampling, we first isolated the fungus on selective media (see next section) before cleaning the cadaver with ethanol for identification. We found most of the cadavers in 2018-2020 near the bases of A. altissima trees (Figure 1A). In rare instances we found SLF adults with fungal outgrowth still attached to host trees by their mouthparts and/or legs (Figure 1B). In some sampling sites (e.g., Leesport and Sinking Spring) we collected live SLF and reared individuals on potted plants in a quarantine lab (for other studies), and some of these SLF later died from naturally occurring infections (i.e., these individuals were already infected with fungi before the time of sampling and rearing in the laboratory; see Supplementary Materials). Collaborators from USDA-APHIS (Kelly Murman, Stefani Cannon, Miriam Cooperband, John Baker, Regina Whitfield), Pennsylvania Department of Agriculture (Emily Fricke, Albert Ciccarone, Betsy Myers, Sandie Conway), and Penn State University (Emelie Swackhamer & Julie Urban) also helped with sampling SLF cadavers with fungal outgrowth in some sites outside of Berks County (e.g., Lancaster and Philadelphia).
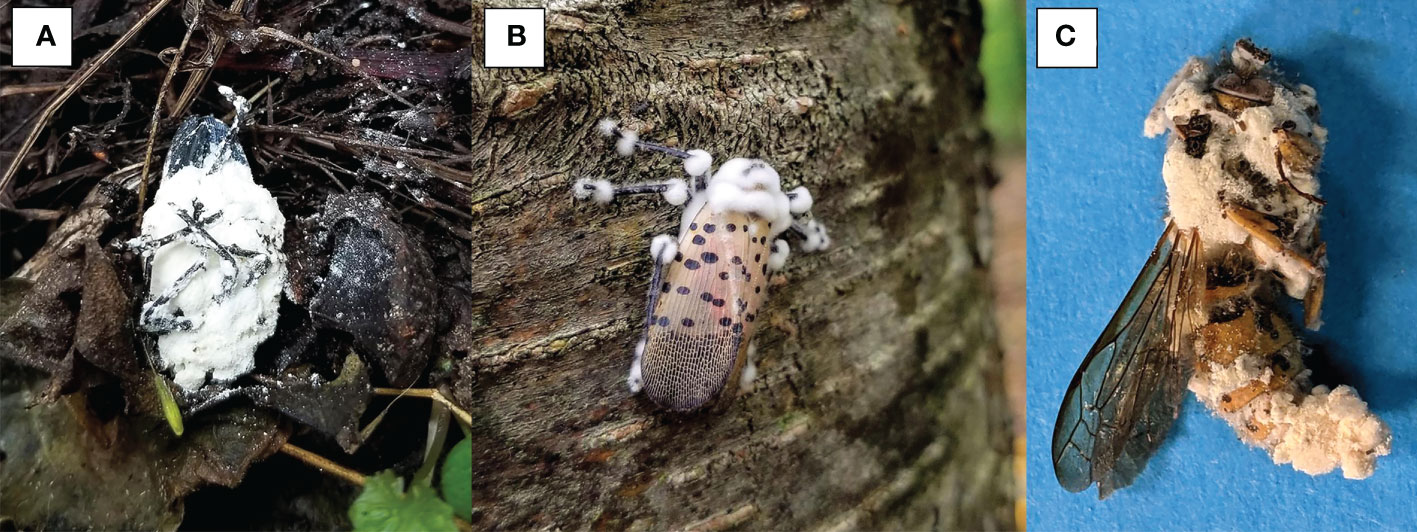
Figure 1 Examples of spotted lanternfly and a non-target with Beauveria fungal outgrowth. (A) Spotted lanternfly adult with profuse Beauveria outgrowth and conidia (infective spores). The white powdery spores are visible on the nearby ground and debris. (B) Spotted lanternfly adult killed by Beauveria and still attached to tree bark. (C) Non-target yellowjacket wasp with Beauveria outgrowth (later identified as B brongniartii).
Isolation of fungi, DNA extraction, PCR, and sequencing
We swabbed conidia from SLF cadavers with fungal outgrowth with a sterile cotton-tip applicator and transferred to 6-cm Petri dishes containing selective media for Beauveria. The medium was adapted from Chase et al. (35), with 30 g wheat germ liter−1, autoclaved and then filtered through cheesecloth, before adding 0.25 g liter−1 chloramphenicol, 0.20 g dodine liter−1, 0.01 g crystal violet liter−1, and 15 g agar liter−1 before autoclaving again. We sealed selective media plates with Parafilm strips and placed in an incubator (20°C, 0:24 (L:D) h). After 10-14 days, we stored cultures of Beauveria isolates at 4°C until further use. Additional cultures of each Beauveria isolate were stored in 10% sterile glycerol at -80 °C in 2 mL cryovials (Nalgene, Thermo Fisher Scientific, Rochester, NY, USA).
We produced mycelium for DNA extraction from each Beauveria isolate following the protocol described in Clifton et al. (31) using potato dextrose broth. Bidirectional nucleotide sequences were determined for the nuclear Bloc intergenic region. A region of Bloc was amplified and sequenced using the primer pair B22-deg × B3.3R, following PCR conditions described by Rehner et al. (12). We used the SAP/EXO protocol to clean up reaction mixtures prior to sequencing (33). Sequencing was done by Cornell University Institute of Biotechnology on an ABI 3730x1 or through submissions to GeneWiz (https://www.genewiz.com/).
Beauveria phylogenetics and prevalence of strains
We edited/trimmed, assembled, and aligned chromatograms and sequences with Geneious Prime software (2021.1.1; Biomatters Ltd.), resulting in contigs with 931-934 base pairs for the Bloc region. Sequence data were checked with the National Center for Biotechnology Information (NCBI) nucleotide database (https://blast.ncbi.nlm.nih.gov/Blast.cgi). After we identified unique strains of B. bassiana, we included the sequence data of each representative strain in the analysis, with one strain of B. brongniartii as an outgroup (Table 2). Maximum Likelihood (ML) analysis was performed using the rapid bootstrap algorithm in RAxML-HPC2 on XEDE version 8.1.11 using the default GTR+G in CIPRES Science Gateway online system (36). The Best Tree from ML analysis was drawn using FigTree version 1.4.4 (http://tree.bio.ed.ac.uk/software/figtree/). We deposited representative isolates of each strain with the USDA ARS Collection of Entomopathogenic Fungal Cultures (ARSEF, Ithaca, NY). ARSEF accession numbers and Genbank accession numbers are listed in Table 2.
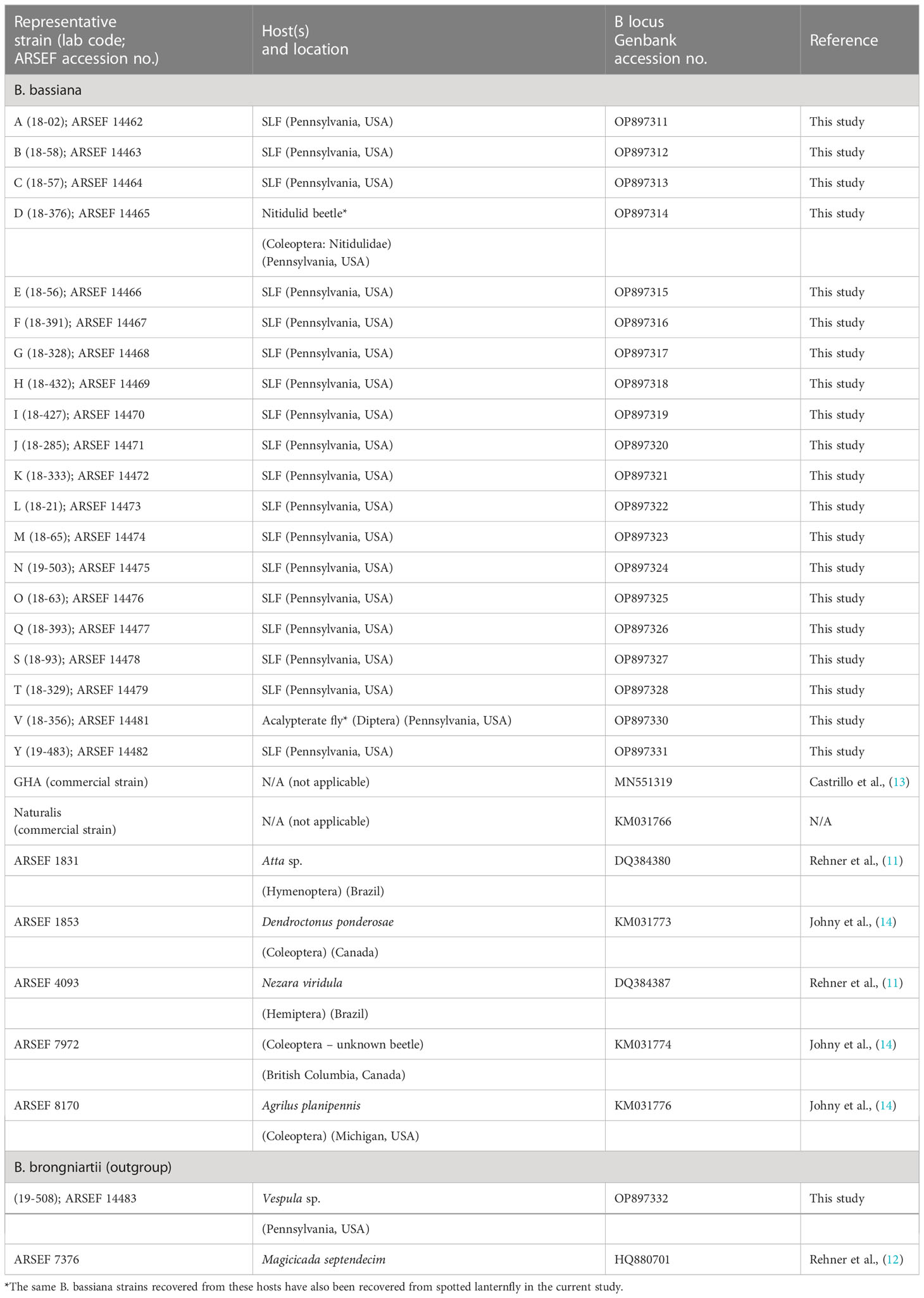
Table 2 GenBank accession numbers of Beauveria spp. strains from spotted lanternfly (SLF) and other insect hosts analyzed in this study.
Beauveria bassiana bioassays for L. delicatula nymphs and adults
Selection of B. bassiana isolates and inoculum production
Based on results from the field studies with B. bassiana strains, in early 2021 we chose six isolates to evaluate conidial production using biphasic-solid fermentation on flaked barley following the methods described by Jaronski & Jackson (37) (Table 3). These six isolates were representative of the prevalent strains (A, B, and L based on Bloc sequence data) in field sites with epizootics that occurred in 2018 (Tables 1; 3).
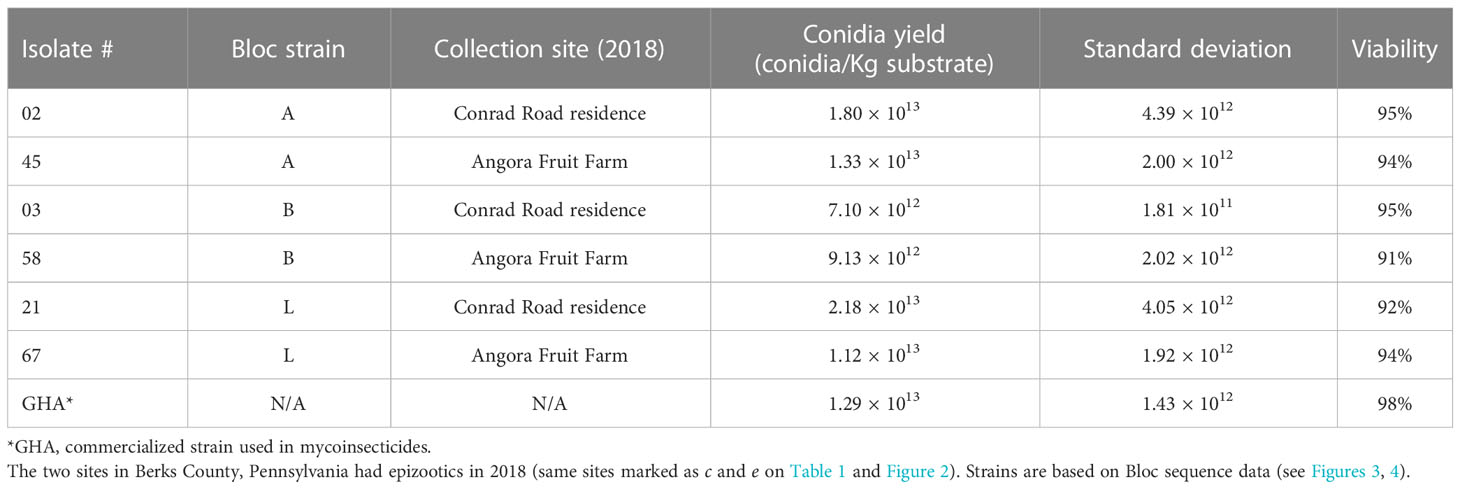
Table 3 Beauveria bassiana isolates from adult spotted lanternfly that were used for solid substrate fermentation on barley flake.
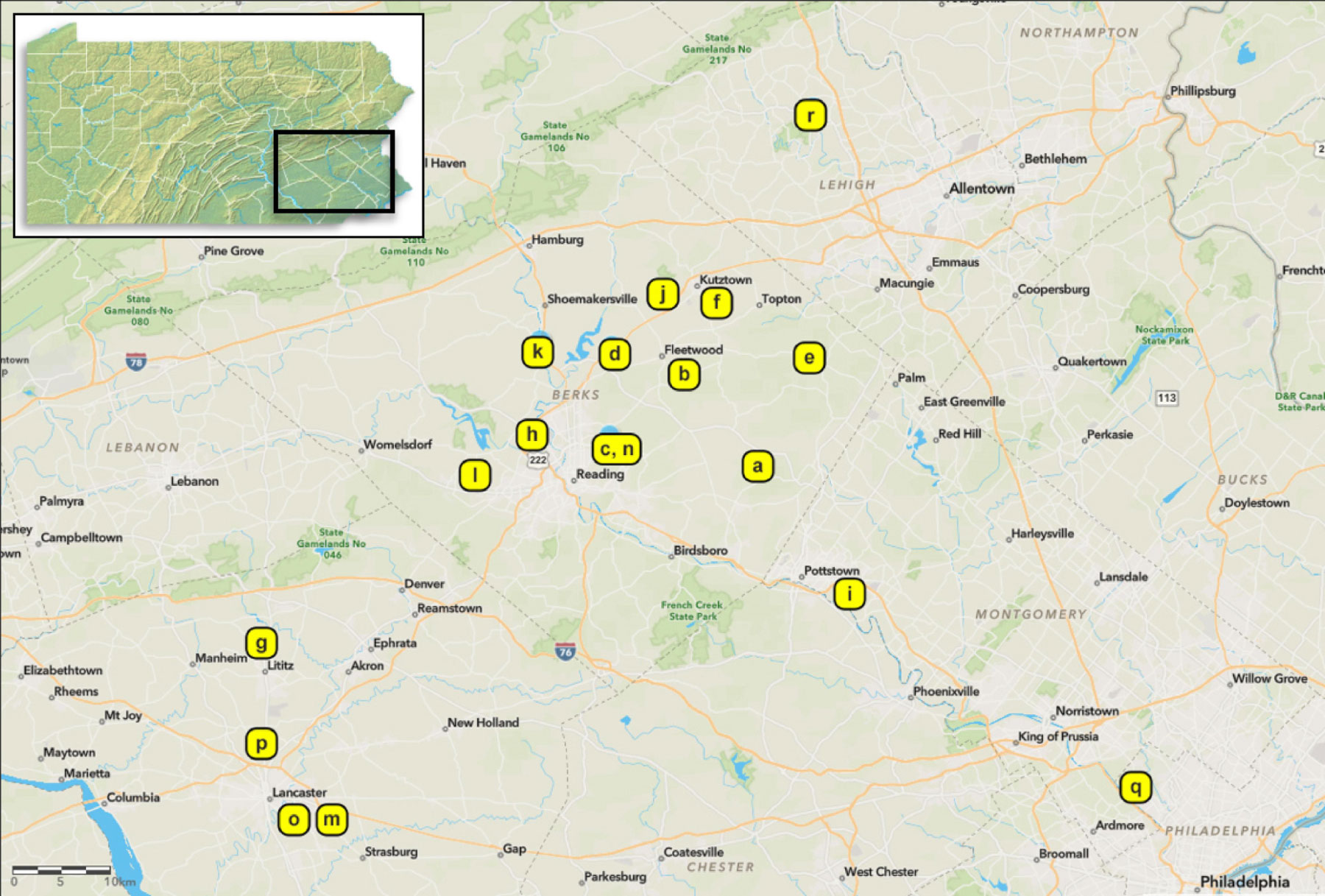
Figure 2 Sampling sites in southeastern Pennsylvania, USA. Inset image on the top left highlights the region. Isolates of Beauveria spp. were obtained from spotted lanternfly and non-targets in 2017 to 2020 (see Table 1 for sampling site information). All sampling sites were infested with tree-of-heaven (Ailanthus altissima) and other host plants. More information about isolates and sampling methods are provided in the Supplementary Materials.
We inoculated 50 ml of a liquid medium (20 g L-1 glucose, 1g L-1 yeast extract, 5 g L-1 KNO3, 2.5g L-1 KH2PO4, 150 mg L-1 chloramphenicol) with conidia from each agar culture in 100 ml Wheaton bottles, which, loosely capped, were then incubated for 4 days at 26° C. Agitation was provided by a magnetic stir bar, rotating at moderate speed in each bottle. This culture medium produces almost pure blastospore cultures. Duplicate (triplicate in the case of strain GHA) amounts of 100 g flaked barley (Grain Millers, Eden Prairie MN), hydrated to 50% V:W with reverse osmosis water, were autoclaved in vented, plastic, 30 x 20 cm mushroom spawn bags (Mycolabs, Crystal Lake IL) at 122° C for 25 minutes. After cooling, each bag was inoculated with 10 ml liquid culture and sealed. Blastospore concentrations in the inocula ranged from 4-9 x107 ml-1. We incubated these bags of solid substrate at 25-26° C for 14 days after which the sporulated barley was transferred to aluminum trays and air dried with gentle laminar air flow over the trays. Drying was complete within 4 days with a final water activity of 0.42-0.45. Conidia were mechanically harvested through stacked 12-mesh and 80-mesh sieves using a table-top automatic powder sifter (Sidasu, Amazon.com). The harvested conidia were then dried to a final water activity of 0.25-0.30 by exposure to silica dessicant in a sealed chamber for 3-4 days. The field-derived strains and commercial strain GHA were grown simultaneously to harvest conidia for bioassays. Yields were calculated based on hemocytometer counts of appropriately diluted spore suspensions in 0.01% Silwet L77 of 100 mg harvested conidial powder and 1 g samples of the spent substrate after conidia had been harvested. Yields were adjusted to conidia Kg-1 dry flaked barley. After analyzing the data on conidial production on flaked barley, conidia of each of the higher-yielding isolates of each strain (02-A, 21-L, 58-B) and GHA were used in laboratory bioassays against SLF in June – August 2021.
One to two days before bioassays, we measured the viability of strains by spreading a dilute aqueous conidial suspension on Sabouraud dextrose agar. Germinated conidia were counted at 400× magnification 14–18 h after incubation at 25°C. All strains had 90% or higher viability before the bioassays on SLF.
Collecting and rearing L. delicatula
For all bioassays, we collected SLF in Pennsylvania and reared them at the Sarkaria Arthropod Research Laboratory at Cornell University, under USDA-APHIS permits (P526-18-02512 and P526P-21-02895). We collected SLF nymphs and adults from the same field site in Sinking Spring, Pennsylvania, as described by Clifton & Hajek (32). Additional details regarding growing A. altissima plants, SLF collection, and rearing in the laboratory are also provided in the Supplementary Materials for Clifton & Hajek (32). Before bioassays, we reared SLF in 91 cm mesh cages (61 cm L × 61 cm W× 91 cm H; ASIN #B07GN4BWZ7, RESTCLOUD) containing potted A. altissima. Cages were held on shelving units in walk-in environmental chambers (10.2 m2; 22.5°C:15°C day:night) with a photoperiod of 16:8 [L:D] h and 65% RH. Light ballasts (New Wave T5 48, Sunlight Supply Inc., Vancouver, Washington) with 4 bulbs (F54T5/840, colour temperature 4000 K, Philips Lighting, Eindhoven, Netherlands) were suspended from the tops of shelving units for illumination.
Direct spray applications and daily mortality checks
We conducted bioassays with B. bassiana against 3rd instar, 4th instar, and adult SLF. We applied field-derived strains and B. bassiana strain GHA at the same concentration of 1.0 × 107 conidia mL-1 suspended in 0.05% sterile Silwet. We cold anesthetized SLF at 4°C for 8–10 min and transferred to 355 mL cardboard cups (diameter: 9.2 cm, height: 6.4 cm) before spray applications. The middle of the corresponding lid for each cardboard cup was punched out and a 15 ×15 cm piece of polyester tulle fabric (bridal veil mesh; pore size = 4 mm2 (2 mm × 2 mm; W× L); ASIN #B01NAU9OD5) was tightly secured between the lid’s rim and the cup to contain SLF. Cardboard containers were only used once for each replicate spray application and disposed. 1.0 mL of each suspension was applied via airbrush. The control treatment was 0.05% Silwet with no B. bassiana spores. Containers held 20 nymphs (3rd and 4th instars) or 15 adults (either male or female) during spray applications. After spray applications, we inverted containers on top of newspaper to dry for 10 minutes. Nymphs were transferred to 63 cm mesh cages (24.5 L × 24.5 W× 63 cm H; BugDorm 4M2260, MegaView Science Co., Ltd., Taiwan) containing one potted A. altissima plant. We sprayed one container of male adults and one container of female adults separately before they were transferred to same mesh enclosure with potted A. altissima. We used larger plants with a 100-cm mesh bag and bamboo support for the adult SLF, which is also described in Clifton & Hajek (32). We sprayed five containers for each life stage and treatment (n = 100 nymphs; 75 males; 75 females).
Before spraying SLF with B. bassiana suspensions, we prepared 1 cm2 squares of water agar that were cut from 150 mm Petri dishes that were prepared in the laboratory. Each agar square was transferred to a smaller 60 mm Petri dish. As we started to spray SLF in each cardboard container, we briefly stopped and sprayed a water agar square for one second before the remainder of the 1.0 ml suspension was applied to SLF. A similar method was used by Poprawski et al. (38) to measure conidia coverage. The lid was placed on the 60 mm Petri dish with the sprayed water agar squares, labelled with the treatment and replicate, and then placed in the refrigerator. Within 48 h of spray applications, we stained the agar squares with lactophenol cotton blue to count conidia and confirm that spray coverage was consistent among treatments. For each water agar square, we scanned 20 random microscope fields at 400×. Counts were averaged and expressed as dosages applied per square millimeter.
We checked SLF daily for 14 days after treatment. We removed SLF that died within 24 h of treatment from cages and excluded them from analysis. Mortality within 24 h was low; for example, 4th instar SLF sprayed with B. bassiana averaged 0.65 ± 0.22 dead nymphs 1 day after spraying. During daily mortality checks, we transferred SLF cadavers from each replicate cage to plastic well plates (24 wells per plate; 1.9 cm2 surface area per well) held inside sealed plastic food containers (13 × 13 × 5 cm), lined with moistened filter paper, to promote fungal outgrowth and confirm mortality from fungal infections (39). We kept SLF cadavers in these sealed containers for 10–14 days after the time of death to allow for fungal outgrowth. After fungal outgrowth, we covered these well plates with respective lids and stored them in a refrigerator for 20-30 days. The well plates allowed for easy separation of cadavers before we removed B. bassiana conidia for quantification (described in next section).
Quantification of conidial production on L. delicatula cadavers
While carrying out the bioassays and handling SLF that were killed by B. bassiana strains, we found apparent differences among strains for conidial production on cadavers (Supplementary Figure 1). Previous studies have also quantified B. bassiana conidial production on host cadavers as one measurement of epizootic potential (14, 16). For each life stage and treatment, we randomly selected 20 cadavers with B. bassiana fungal outgrowth to quantify B. bassiana conidial production. Detailed methods for removing and quantifying conidia with 70% ethanol are described in the Supplementary Materials. After removing B. bassiana conidia from SLF, we dried these cadavers under a fan in a biosafety cabinet for one hour before weighing them on a precision scale for dry body mass (mg). We divided the numbers of total conidia for each cadaver by its dry body mass. While there is less variation in body mass for nymphs, adult SLF can vary a great deal, and adult females are usually heavier than adult males, as noted by Clifton & Hajek (32).
Data analysis
We calculated mean survival times and standard errors for SLF receiving each treatment based on Kaplan-Meier survival distribution functions using PROC LIFETEST in SAS 9.4 (40). For multiple comparisons of survival times among different treatments, we used the Cox proportional hazards model with PROC PHREG. Contrasts between treatments were conducted using least-square means, adjusted with the Bonferroni correction. For 3-4 instar nymphs, we combined data before we compared survival times (n = 200 per treatment). For adult SLF, we combined data for males and females and compared mycoinsecticide treatments. (n = 150 per treatment).
For each spray trial on 3rd instars, 4th instars, adult males, and adult females, we compared mean conidial coverage (# per mm2 on water agar squares) using a one-way ANOVA with Fisher’s least-significant-difference test, using PROC ANOVA. For counts of conidial production on SLF cadavers, we also used one-way ANOVA and compared total conidia per dry mg of body mass. We checked these data with the Shapiro-Wilk Test (α=0.05) and they did have normal distribution before analysis (41).
Results
Identification and diversity
Sequencing of the Bloc region resulted in 931-934 bp of reliable data for 165 isolates, with 812 constant sites and 119 variable characters. One strain (F) had an extra 3 bp (CCC) between positions 527-528 in the Bloc sequence. All Beauveria isolates from SLF were identified as B. bassiana (Figure 3). One isolate from a nontarget yellowjacket wasp was identified as B. brongniartii (Figure 1C). Bloc sequence data revealed 20 distinct strains among the 164 B. bassiana isolates from SLF and non-targets in Pennsylvania, and these strains were grouped into two clades (Figure 3).
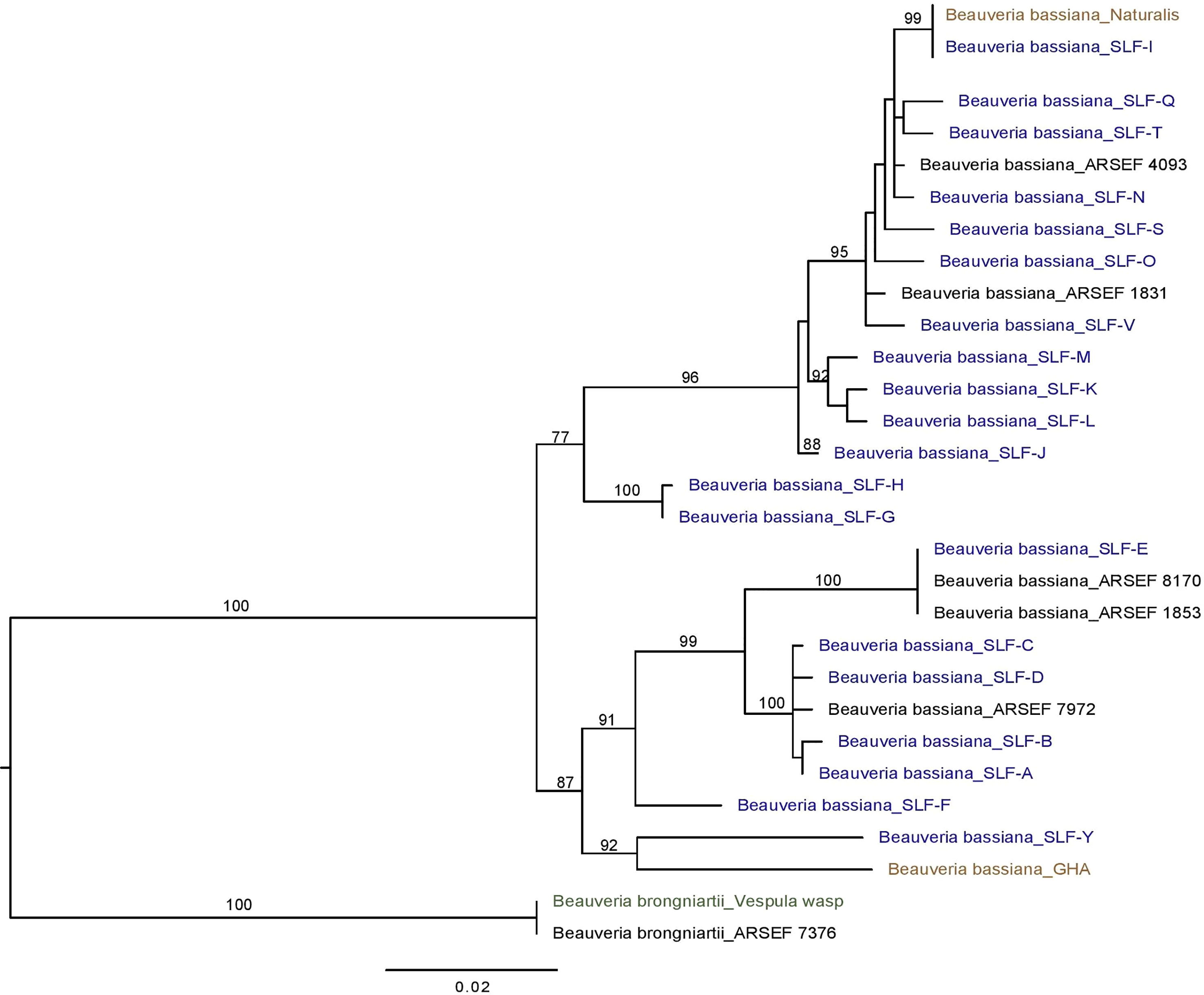
Figure 3 Phylogeny of B bassiana associated with the spotted lanternfly (SLF; in blue text), L. delicatula. Best tree from Bayesian analysis of the B locus sequence data (alignments of 934 bp). The analysis includes 20 B bassiana strains from SLF, the commercial strains GHA and Naturalis (in brown text) and B brongniartii as outgroup, including one strain obtained from a yellowjacket (Vespula sp.; in green text).
Non-targets killed by Beauveria spp.
Seven non-target cadavers with fungal outgrowth were collected in 2018-2020. Two of these seven non-targets were yellowjacket wasps infected by Beauveria spp. Six non-targets (Table 4) were killed by B. bassiana strains A, B, D, and V, which were also isolated from SLF. B. bassiana strains A, B, and D were placed in one clade while strain V was placed in a separate clade. One isolate of B. brongniartii was recovered from a yellowjacket wasp in 2019, but at this time we have not yet recovered this species from SLF.
Prevalence of B. bassiana strains
Strains A, B, C, L were the only strains recovered from both SLF nymphs and adults. In total, ten B. bassiana isolates were recovered from nymphs. Strains A, B, and L were the most prevalent, accounting for 114 isolates out of the total 164 isolates (70%) that were analyzed in this study (Figure 4). These dominant strains were found in multiple years for some sites, including Angora Fruit Farm, Blandon, Conrad Road, and Sinking Spring (Table 5). Many of the B. bassiana isolates sampled from Angora Fruit Farm and Conrad Road in October 2018 came from SLF adults during an epizootic that caused localized population collapses (8).
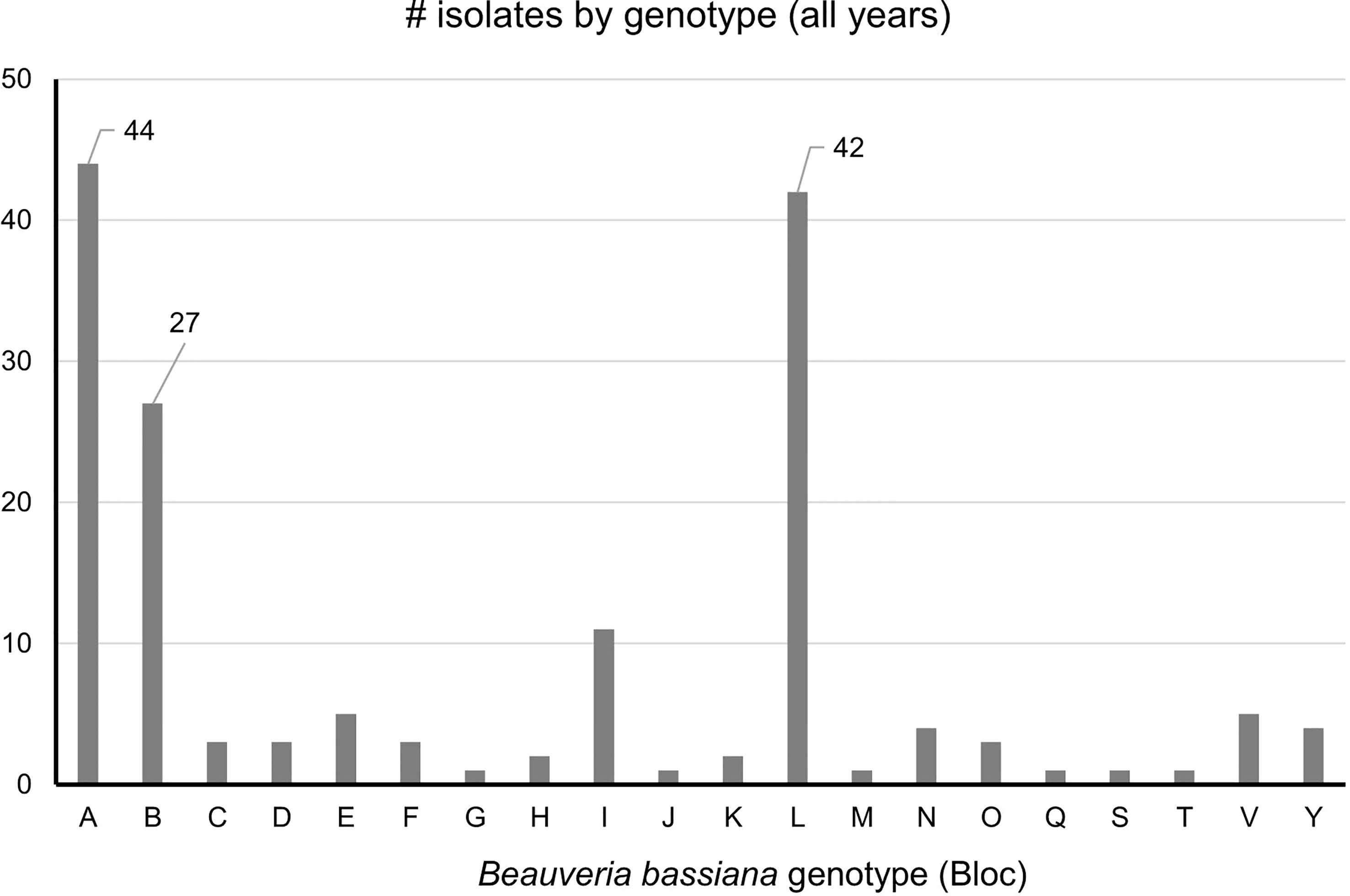
Figure 4 Prevalence of B bassiana strains associated with spotted lanternfly adult and nymphs collected in 2017-2020 from Pennsylvania, USA. The most common strains (A, B, and L) have data labels. n = 164.
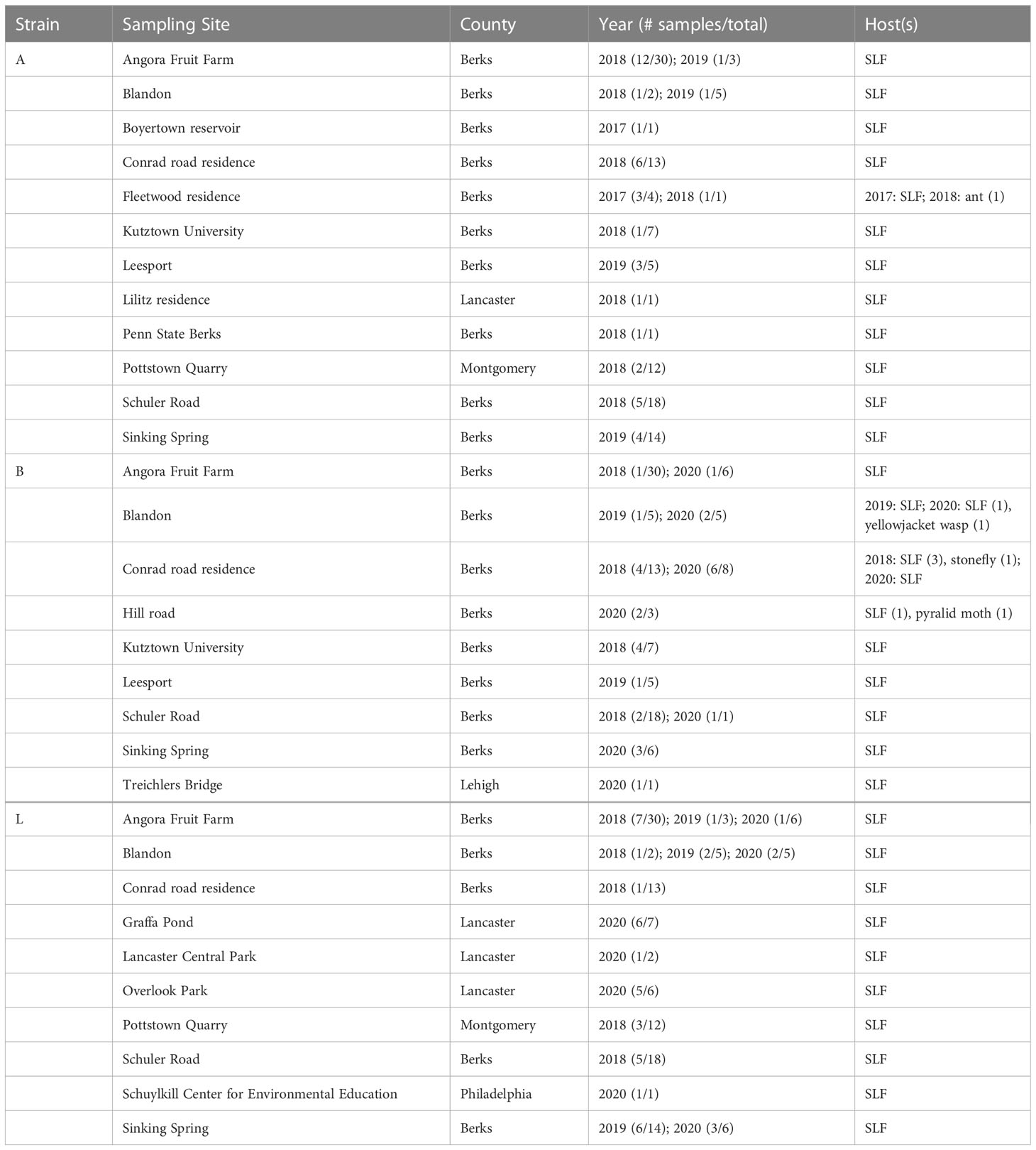
Table 5 The most common B. bassiana strains (A, B, and L) based on B locus sequences, associated with spotted lanternfly (SLF) and other hosts in sites in Pennsylvania, USA.
Bioassays with B. bassiana and L. delicatula
For all the spray experiments with B. bassiana strains, there was no significant difference among treatments for conidia coverage on squares of water agar. Third instar nymphs: no significant differences (F = 1.28, df = 3, 16, P = 0.3156), with averages that ranged from 467 to 573 conidia per mm2. Fourth instar nymphs: no significant differences (F = 2.39, df = 3, 16, P = 0.1068), with averages that ranged from 472 to 563 conidia per mm2. Adult males: no significant differences (F = 2.28, df = 3, 16, P = 0.1182), with averages that ranged from 525 to 571 conidia per mm2. Adult females: no significant differences (F = 0.78, df = 3, 16, P = 0.5215), with averages that ranged from 538 to 572 conidia per mm2. These data provided confidence that SLF exposed to the B. bassiana strains had received similar doses.
The survival times of SLF nymphs were significantly different among treatments, (Log-rank χ2 = 306.41; df = 4; P < 0.0001); those exposed to B. bassiana died significantly faster than controls (Figure 5). Additionally, B. bassiana strain B caused significantly less mortality to SLF nymphs compared to B. bassiana strains A, L, and GHA.
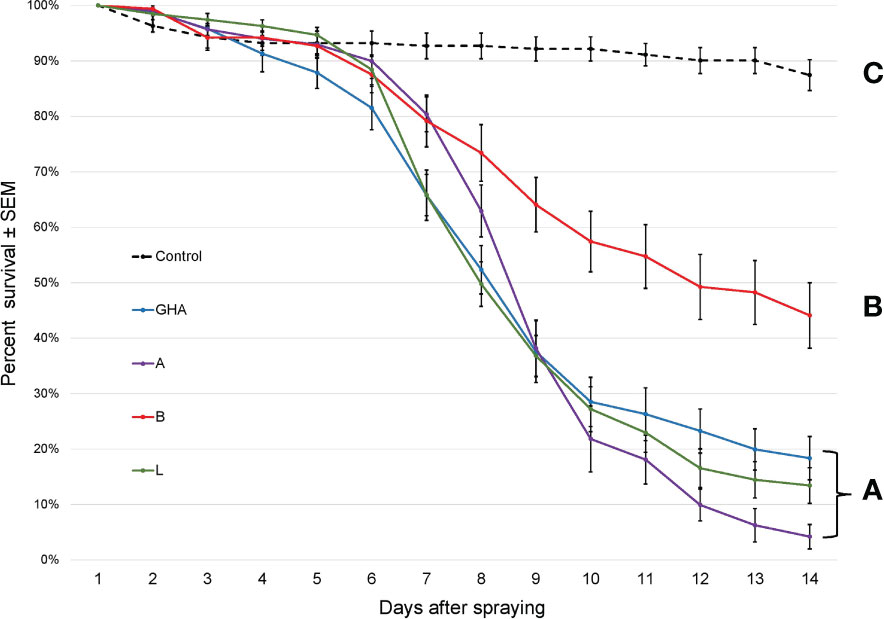
Figure 5 Survival of L. delicatula nymphs on potted A altissima plants. Data are combined for 3rd and 4th instar nymphs. Lines represent different B bassiana strains (control = dotted line). Letters represent significant differences among treatments for survival curves based on Cox proportional hazards.
The survival times of SLF adults were significantly different among treatments, (Log-rank χ2 = 216.68; df = 4; P < 0.0001); those exposed to B. bassiana died significantly faster than controls (Figure 6). Additionally, SLF adults exposed to B. bassiana strains A and L died significantly faster than those exposed to strain B and GHA.
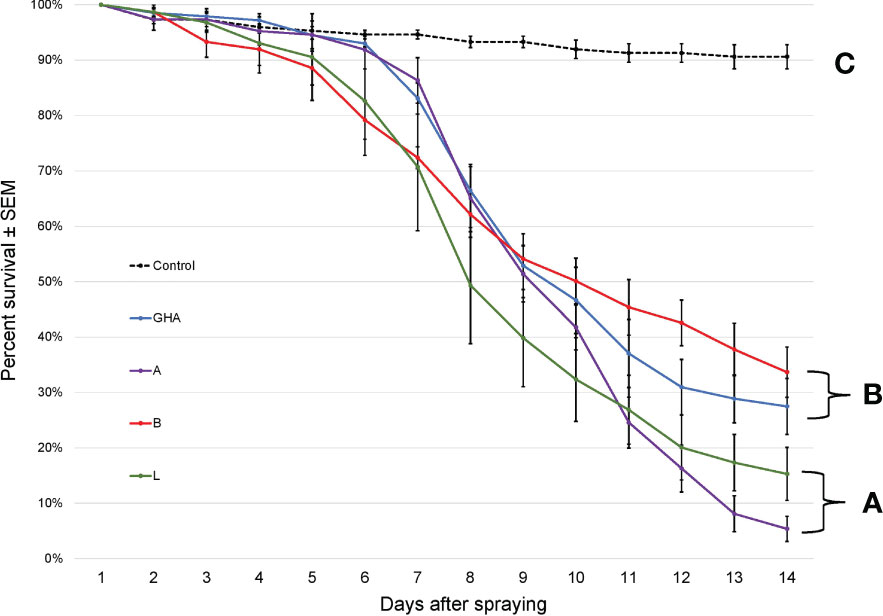
Figure 6 Survival of L. delicatula adults on potted A altissima plants. Lines represent different B bassiana strains (control = dotted line). Letters represent significant differences among treatments for survival curves based on Cox proportional hazards.
Conidial production for B. bassiana strains A, B, and L was significantly higher than strain GHA for both the 3rd instar nymphs (F = 44.87, df = 3, 75, P < 0.0001; Figure 7A) and 4th instar nymphs (F = 29.67, df = 3, 76, P < 0.0001; Figure 7B). Conidial production for B. bassiana strains B and L was significantly higher than strain A for adult males, which was significantly higher than GHA (F = 72.94, df = 3, 76, P < 0.0001; Figure 7C). For adult females, conidial production for strain A was significantly higher than strain L, but strain B was not significantly different compared to both A and L (F = 40.78, df = 3, 76, P < 0.0001; Figure 7D). All three field-derived B. bassiana strains had significantly higher conidial production than GHA for adult females.
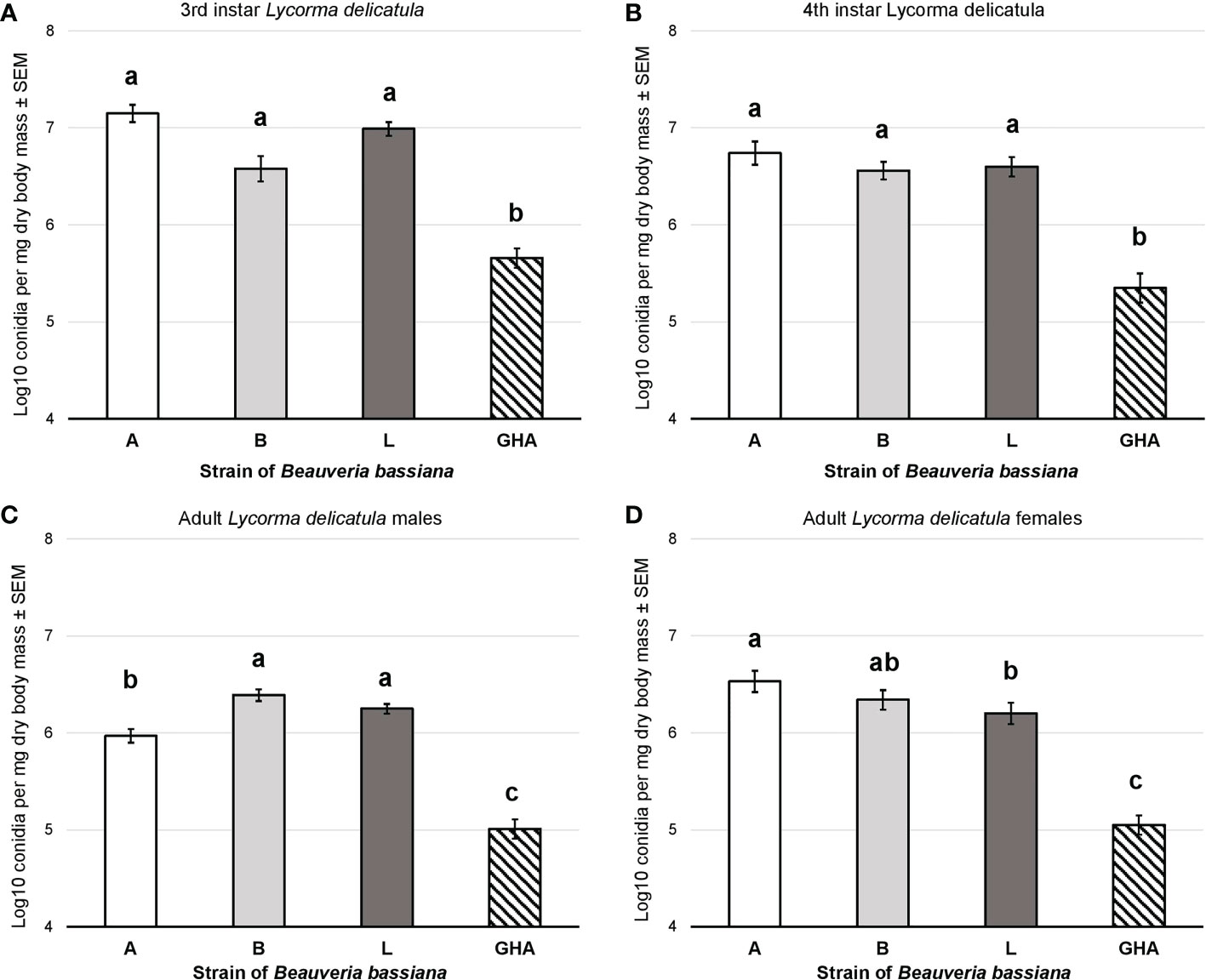
Figure 7 Conidial production of B bassiana strains on L. delicatula cadavers from experiments on (A) third instar nymphs, (B) fourth instar nymphs, (C) adult males, and (D) adult females. Bar shading represent different B bassiana strains used in bioassays. Lowercase letters above bars represent significant differences among treatments based on Fisher’s least-significant-difference test.
Discussion
All Beauveria isolates infecting SLF collected in Pennsylvania from 2017 to 2020 were identified as B. bassiana. Beauveria bassiana is also known to infect SLF in China (42), where this invasive host is native. Beauveria bassiana was also a major entomopathogen infecting invasive scolytines attacking coffee berries in Hawaii (13) and infecting emerald ash borers in Michigan and southwestern Canada (14). This fungal species acts as an entomopathogen but also persists in ecosystems as a plant endophyte, or a saprophyte in the soil (43). As an entomopathogen B. bassiana is a generalist, occurring worldwide and known to infect > 200 species of insects across many insect orders (3), although host range is more limited for individual strains (44). Perhaps the breadth in host range of B. bassiana as well as life as a saprophyte and endophyte help to explain the natural occurrence of abundant genetic diversity in B. bassiana. In our study alone, among B. bassiana isolates from SLF in southeastern Pennsylvania we found 20 different strains based on Bloc sequence data. Other studies, each based on one host species, have also documented genetic diversity in B. bassiana isolates (13, 14). Our genetic analysis is based on only one locus commonly used for identification of Beauveria species (12), but sequencing additional loci or genes could show more genetic differences among these 20 strains.
We hypothesize that the B. bassiana isolates from our study are native and their ability as generalists facilitates their switching over to use the relatively new resource constituted by abundant SLF populations. Some of the same B. bassiana strains that infected SLF also infected non-targets in this study (Table 4), suggesting that these native hosts could constitute some of the sources for the naturally occurring strains. Infection levels by B. bassiana will vary over time and space, depending on population densities of susceptible hosts, the fungal titers in the soil and on phylloplanes, and weather (45, 46). Additional sampling methods, e.g., the “Galleria bait method” (47) and serial dilutions of soil samples on selective media, could help to better describe the communities of native entomopathogens in these sites that were invaded by SLF.
Three B. bassiana strains (A, B, and L) were most prevalent and widespread among field sites in Pennsylvania. Beauveria bassiana strain A did not have any 100% matches to B. bassiana isolates on GenBank, but it did have high similarity (99-99.8% matches) to Bloc sequences from B. bassiana isolates recovered from emerald ash borer populations in Canada (GenBank JN849673.1 and others in PopSet 379045698). Strain L and other strains in the top clade (Figure 3) had high similarity to Brazilian strains of B. bassiana (ARSEF 4093 and 1831; Table 2), and the B. bassiana strains in this top clade already have a wide distribution in mainland North America and South America. According to the Bloc sequence data, strain I has a 100% match to B. bassiana strain ATCC 74040, which is used in the mycoinsecticide Naturalis® (GenBank KM031766.1), but other loci would need to be sequenced to confirm that strain’s similarity. Beauveria bassiana strain ATCC 74040 was originally isolated from the cotton boll weevil, Anthonomus grandis (Boheman) (Coleoptera: Curculionidae), in Texas and is known to naturally occur throughout the United States (48). Genetic analysis of B. bassiana isolates infecting SLF in other countries is needed to further assess the specificity of these fungal entomopathogens and insect hosts.
In the field studies, we found B. bassiana strain A infecting one ant and strain B infecting 3 different non-target insects (Table 4). Among the three most collected B. bassiana strains, strain L was the only one not found infecting non-targets. Two of the seven non-targets that we found infected by Beauveria were yellowjacket wasps (Vespula sp. [Hymenoptera: Vespdae]), which were regularly observed foraging for honeydew produced by SLF feeding. We isolated and identified one isolate of B. brongniartii from a yellowjacket wasp in 2019 (Table 4). Beauveria brongniartii has a more restricted host range than B. bassiana, principally infecting Coleoptera but also known to infect other insect hosts (12), but to our knowledge this fungus is not associated with SLF or other fulgorids, even though it was collected in the same areas as SLF. It is likely that there is horizontal transmission of Beauveria spores between SLF and these hymenopterans, especially since the other vespid had been infected by strain B, one of the most common strains infecting SLF. It should be noted that our sampling of non-targets may not capture the full range of potential non-targets killed by Beauveria spp. in these sites. We primarily sampled near A. altissima trees, collecting any dead and mycosed non-targets that we could find, often in proximity to dead SLF; otherwise, we did not spend additional time in field sites exclusively looking for non-targets. A variety of insect traps, nets, and other sampling tools are needed to thoroughly describe potential infections of these native non-targets and to find any possible fungus-infected hosts before they are lost to scavengers and weathering.
The laboratory bioassays revealed differences in efficacy, with B. bassiana strain B killing significantly fewer nymphs than strains A, L, and GHA, but the bioassays against adults showed that strains A and L had greater efficacy than strains B and GHA. All the field-derived B. bassiana strains had significantly greater conidial production on cadavers compared to commercial strain GHA on all SLF life stages that were tested. Wraight et al. (16) found that B. bassiana strain HI-25 produced >2.5 times greater numbers of conidia than strain GHA on coffee berry borer cadavers. In our study, we found that strains A, B, and L produced >15 times more conidia than strain GHA on 4th instar SLF (Figure 7B), and these results on conidial production suggest these strains have greater epizootic potential than strain GHA. A similar pattern of conidial production was observed for the same B. bassiana strains growing on selective medium, with strain GHA having smaller colony growth compared to the field-derived strains (Supplementary Figure 2).
More work is needed on these field-derived B. bassiana strains to discern any potential for commercialization, in particular relative to the ability to economically mass produce them. If the fungal growth process were scaled up to the larger spawn bags used by industry, it is not yet clear how cost effective it would be to grow these different B. bassiana strains even though some strains produced similar if not higher yields than strain GHA on a small scale (Table 3). Aside from considerations regarding feasibility of mass production of new B. bassiana strains, there would also need to be evaluations of shelf-life, toxicity testing, and other criteria (49). Additional studies are needed to further characterize these B. bassiana strains, which would include non-target testing, nutritional requirements for large scale fermentation, and more bioassays to determine virulence to SLF (LC50 tests).
In summary, aside from epizootics driven by B. major in 2018, B. bassiana is the most common entomopathogenic fungus that naturally infects SLF each year in the areas we studied. Surprisingly, among Beauveria spp. isolated from SLF, we only found the species B. bassiana. Twenty-one B. bassiana strains infected SLF, with three being more common. Based on bioassay data, strains A and L are more promising candidates for biological control of SLF, exhibiting similar efficacy to a commercialized strain (GHA) and high epizootic potential due to more abundant conidial production.
Data availability statement
The datasets presented in this study can be found in online repositories. The names of the repository/repositories and accession number(s) can be found in the article/Supplementary Material. Additional raw data supporting the conclusions of this article will be made available by the authors, without undue reservation.
Author contributions
All authors designed the study. EC and SJ conducted the experiments. All authors analyzed the data and wrote the manuscript. All authors contributed to the article and approved the submitted version.
Funding
This work was funded by USDA NIFA 2019-51181-30014 (AEH).
Acknowledgments
We thank Abby Davis, Kelly Murman, Stefani Cannon, Miriam Cooperband, John Baker, Regina Whitfield, Victor Colon-Ortiz, Kristen Wickert, Emelie Swackhamer, Emily Fricke, Albert Ciccarone, Betsy Myers, Sandie Conway, Sven-Erik Spichiger, Gregory Parra, and Julie Urban for assistance with sample collection. David Harris, Daniela Rochez, Sarah Stefanik, Allyson Wentworth, and Sharon Wu assisted with laboratory and field work.
Conflict of interest
Author SJ was employed by company Jaronski Mycological Consulting LLC.
The remaining authors declare that the research was conducted in the absence of any commercial or financial relationships that could be construed as a potential conflict of interest.
Publisher’s note
All claims expressed in this article are solely those of the authors and do not necessarily represent those of their affiliated organizations, or those of the publisher, the editors and the reviewers. Any product that may be evaluated in this article, or claim that may be made by its manufacturer, is not guaranteed or endorsed by the publisher.
Supplementary material
The Supplementary Material for this article can be found online at: https://www.frontiersin.org/articles/10.3389/finsc.2023.1127682/full#supplementary-material
References
1. Araújo JPM, Hughes DP. Diversity of entomopathogenic fungi: which groups conquered the insect body? Adv Gen (2016) 94:1–39. doi: 10.1016/bs.adgen.2016.01.001
2. Soper RS. Pathogens of leafhoppers and planthoppers. In: Nault LR, Rodriguez JG, editors. The leafhoppers and planthoppers. New York: Wiley (1985). p. 469–88.
3. Feng MG, Poprawski TJ, Khachatourians GG. Production, formulation and application of the entomopathogenic fungus Beauveria bassiana for insect control: current status. Biocontrol Sci Technol (1994) 4(1):3–34. doi: 10.1080/09583159409355309
4. Chandler D. Basic and applied research on entomopathogenic fungi. In: Lacey LA, editor. Microbial control of insect and mite pests. Amsterdam: Academic Press (2017). p. 69–89.
5. Krueger SR, Nechols JR, Ramoska WA. Habitat distribution and infection rates of the fungal pathogen, Beauveria bassiana (Balsamo) vuillemin in endemic populations of the chinch bug, Blissus leucopterus leucopterus (Say) (Hemiptera: Lygaeidae) in Kansas. J Kans Entomol Soc (1992) 65:115–24. Available at: https://www.jstor.org/stable/25085341
6. Marcelino JA, Gouli S, Giordano R, Gouli VV, Parker BL, Skinner M. Fungi associated with a natural epizootic in Fiorinia externa Ferris (Hemiptera: Diaspididae) populations. J Appl Entomol (2009) 133(2):82–9. doi: 10.1111/j.1439-0418.2008.01301.x
7. Seiter NJ, Grabke A, Greene JK, Kerrigan JL, Reay-Jones FP. Beauveria bassiana is a pathogen of Megacopta cribraria (Hemiptera: Plataspidae) in south Carolina. J Entomol Sci (2014) 49(3):326–30. doi: 10.18474/0749-8004-49.3.326
8. Clifton EH, Castrillo LA, Gryganskyi A, Hajek AE. A pair of native fungal pathogens drives decline of a new invasive herbivore. PNAS (2019) 116(19):9178–80. doi: 10.1073/pnas.1903579116
9. Clifton EH, Castrillo LA, Hajek AE. Discovery of two hypocrealean fungi infecting spotted lanternflies, Lycorma delicatula: Metarhizium pemphigi and a novel species, Ophiocordyceps delicatula. J Invertebr Pathol (2021) 186:107689. doi: 10.1016/j.jip.2021.107689
10. Rehner SA, Buckley EA. Beauveria phylogeny inferred from nuclear ITS and EF1-α sequences: evidence for cryptic diversification and links to Cordyceps teleomorphs. Mycologia (2005) 97(1):84–98. doi: 10.1080/15572536.2006.11832842
11. Rehner SA, Posada F, Buckley EP, Infante F, Castillo A, Vega FE. Phylogenetic origins of African and Neotropical Beauveria bassiana s.l. pathogens of the coffee berry borer, Hypothenemus hampei. J Invertebr Pathol (2006) 93(1):11–21. doi: 10.1016/j.jip.2006.04.005
12. Rehner SA, Minnis AM, Sung GH, Luangsa-ard JJ, Devotto L, Humber RA. Phylogeny and systematics of the anamorphic, entomopathogenic genus. Beauveria. Mycologia (2011) 103(5):1055–73. doi: 10.3852/10-302
13. Castrillo LA, Wraight SP, Galaini-Wraight S, Matsumoto TK, Howes RL, Keith LM. Genetic diversity among naturally-occurring strains of Beauveria bassiana associated with the introduced coffee berry borer, Hypothenemus hampei (Coleoptera: Curculionidae), on hawai’i island. J Invertebr Pathol (2020) 175:107456. doi: 10.1016/j.jip.2020.107456
14. Johny S, Kyei-Poku G, Gauthier D, van Frankenhuyzen K, Krell PJ. Characterization and virulence of Beauveria spp. recovered from emerald ash borer in southwestern Ontario, Canada. J Invertebr Pathol (2012) 111(1):41–9. doi: 10.1016/j.jip.2012.05.008
15. Wraight SP, Galaini-Wraight S, Howes RL, Castrillo LA, Carruthers RI, Smith RH, et al. Prevalence of naturally-occurring strains of Beauveria bassiana in populations of coffee berry borer Hypothenemus hampei on hawai’i island, with observations on coffee plant-h. hampei-B. bassiana interactions. J Invertebr Pathol (2018) 156:54–72. doi: 10.1016/j.jip.2018.07.008
16. Wraight SP, Howes RL, Castrillo LA, Griggs MH, Galaini-Wraight S, Carruthers RI, et al. Laboratory studies assessing the microbial biocontrol potential of diverse strains of Beauveria bassiana isolated from coffee berry borer, with emphasis on strains from hawai’i island and comparisons to commercial strain GHA. J Invertebr Pathol (2022) 194:107819. doi: 10.1016/j.jip.2022.107819
17. Dara SK, Barringer L, Arthurs SP. Lycorma delicatula (Hemiptera: Fulgoridae): a new invasive pest in the united states. J Integr Pest Manag (2015) 6(1):20. doi: 10.1093/jipm/pmv021
18. New York State IPM (Integrated Pest Management). Confirmed spotted lanternfly locations (2022). Available at: https://cals.cornell.edu/new-york-state-integrated-pest-management/outreach-education/whats-bugging-you/spotted-lanternfly/spotted-lanternfly-reported-distribution-map.
19. Liu H. Occurrence, seasonal abundance, and superparasitism of Ooencyrtus kuvanae (Hymenoptera: Encyrtidae) as an egg parasitoid of the spotted lanternfly (Lycorma delicatula) in north America. Forests (2019) 10(2):79. doi: 10.3390/f10020079
20. Liu H. Seasonal development, cumulative growing degree-days, and population density of spotted lanternfly (Hemiptera: Fulgoridae) on selected hosts and substrates. Environ Entomol (2020) 49(5):1171–84. doi: 10.1093/ee/nvaa074
21. Barringer L, Ciafré CM. Worldwide feeding host plants of spotted lanternfly, with significant additions from north America. Environ Entomol (2020) 49(5):999–1011. doi: 10.1093/ee/nvaa093
22. Leach H, Leach A. Seasonal phenology and activity of spotted lanternfly (Lycorma delicatula) in eastern US vineyards. J Pest Sci (2020) 93(4):1215–24. doi: 10.1007/s10340-020-01233-7
23. Urban JM. Perspective: shedding light on spotted lanternfly impacts in the USA. Pest Manag Sci (2020) 76(1):10–7. doi: 10.1002/ps.5619
24. Leach H. Spotted lanternfly management for landscape professionals (2020). Available at: https://extension.psu.edu/spotted-lanternfly-management-for-landscape-professionals.
25. Murman K, Setliff GP, Pugh CV, Toolan MJ, Canlas I, Cannon S, et al. Distribution, survival, and development of spotted lanternfly on host plants found in north America. Environ Entomol (2020) 49(6):1270–81. doi: 10.1093/ee/nvaa126
26. Urban JM, Leach H. Biology and management of the spotted lanternfly, Lycorma delicatula (Hemiptera: Fulgoridae), in the united states. Annu Rev Entomol (2023) 68:151–67. doi: 10.1146/annurev-ento-120220-111140
27. Hajek AE, McManus ML, Delalibera J. A review of introductions of pathogens and nematodes for classical biological control of insects and mites. Biol Control (2007) 41(1):1–13. doi: 10.1016/j.biocontrol.2006.11.003
28. Liu H, Mottern J. An old remedy for a new problem? identification of Ooencyrtus kuvanae (Hymenoptera: Encyrtidae), an egg parasitoid of Lycorma delicatula (Hemiptera: Fulgoridae) in north America. J Insect Sci (2017) 17(1):18. doi: 10.1093/jisesa/iew114
29. Broadley HJ, Gould JR, Sullivan LT, Wang XY, Hoelmer KA, Hickin ML, et al. Life history and rearing of Anastatus orientalis (Hymenoptera: Eupelmidae), an egg parasitoid of the spotted lanternfly (Hemiptera: Fulgoridae). Environ Entomol (2021) 50(1):28–35. doi: 10.1093/ee/nvaa124
30. Arthurs S, Dara SK. Microbial biopesticides for invertebrate pests and their markets in the united states. J Invertebr Pathol (2019) 165:13–21. doi: 10.1016/j.jip.2018.01.008
31. Clifton EH, Hajek AE, Jenkins NE, Roush RT, Rost JP, Biddinger DJ. Applications of Beauveria bassiana (Hypocreales: Cordycipitaceae) to control populations of spotted lanternfly (Hemiptera: Fulgoridae), in semi-natural landscapes and on grapevines. Environ Entomol (2020) 49(4):854–64. doi: 10.1093/ee/nvaa064
32. Clifton EH, Hajek AE. Efficacy of Beauveria bassiana and Cordyceps javanica mycoinsecticides against spotted lanternflies, Lycorma delicatula, in laboratory bioassays. Biocontrol Sci Technol (2022) 32(7):824–36. doi: 10.1080/09583157.2022.2052804
33. Gryganskyi AP, Golan J, Hajek AE. Season-long infection of diverse hosts by the entomopathogenic fungus Batkoa major. PloS One (2022) 17(5):e0261912. doi: 10.1371/journal.pone.0261912
34. Pennsylvania Department of Agriculture. Spotted lanternfly quarantine (2023). Available at: https://www.agriculture.pa.gov/Plants_Land_Water/PlantIndustry/Entomology/spotted_lanternfly/quarantine/Pages/default.aspx.
35. Chase AR, Osborne LS, Ferguson VM. Selective isolation of the entomopathogenic fungi Beauveria bassiana and Metarhizium anisopliae from an artificial potting medium. Fla Entomol (1986) 69:285–92. doi: 10.2307/3494930
36. Miller MA, Pfeiffer W, Schwartz T. Creating the CIPRES science gateway for inference of large phylogenetic trees. In: 2010 gateway computing environments workshop, GCE 2010. New Orleans, LA: IEEE (2010). p. 1–8. Available at: https://ieeexplore.ieee.org/abstract/document/5676129
37. Jaronski ST, Jackson MA. Mass production of entomopathogenic hypocreales. In: Lacey LA, editor. Manual of techniques in invertebrate pathology, 2nd edition. New York: Elsevier/Academic Press (2012). p. 255–84.
38. Poprawski TJ, Greenberg SM, Ciomperlik MA. Effect of host plant on Beauveria bassiana-and Paecilomyces fumosoroseus-induced mortality of Trialeurodes vaporariorum (Homoptera: Aleyrodidae). Environ Entomol (2000) 29(5):1048–53. doi: 10.1603/0046-225X-29.5.1048
39. Reay SD, Brownbridge M, Gicquel B, Cummings NJ, Nelson TL. Isolation and characterization of endophytic Beauveria spp. (Ascomycota: Hypocreales) from Pinus radiata in new Zealand forests. Biol Control (2010) 54(1):52–60. doi: 10.1016/j.biocontrol.2010.03.002
40. SAS Institute Inc. SAS software release 9.4. Cary, North Carolina (NC): SAS Institute Inc (2021).
41. Shapiro SS, Wilk MB. An analysis of variance test for normality (complete samples). Biometrika (1965) 52(3/4):591–611. doi: 10.2307/2333709
42. Li MY, Lin HF, Li SG, Xu AM, Feng MF. Efficiency of entomopathogenic fungi in the control of eggs of the brown planthopper Nilaparvata lugens stål (Homoptera: Delphacidae). Afr J Microbiol Res (2012) 6(44):7162–7. doi: 10.5897/AJMR12.611
43. Meyling NV, Eilenberg J. Ecology of the entomopathogenic fungi Beauveria bassiana and Metarhizium anisopliae in temperate agroecosystems: potential for conservation biological control. Biol Control (2007) 43(2):145–55. doi: 10.1016/j.biocontrol.2007.07.007
44. Goettel M, Poprawski T, Vandenberg J, Li Z, Roberts D. Safety to nontarget invertebrates of fungal bio-control agents. In: Laird M, Lacey LA, Davidson EW, editors. Safety of microbial insecticides. Boca Raton, FL: CRC Press (1989). p. 209–29.
45. Hajek AE, St. Leger RJ. Interactions between fungal pathogens and insect hosts. Annu Rev Entomol (1994) 39:293–322. doi: 10.1146/annurev.en.39.010194.001453
46. Jaronski ST. Ecological factors in the inundative use of fungal entomopathogens. BioControl (2010) 55:159–85. doi: 10.1007/s10526-009-9248-3
47. Zimmermann G. The ‘Galleria bait method’ for detection of entomopathogenic fungi in soil. J Appl Entomol (1986) 102(1-5):213–5. doi: 10.1111/j.1439-0418.1986.tb00912.x
48. Mayoral F, Benuzzi M, Ladurner E. Efficacy of the Beauveria bassiana strain ATCC 74040 (Naturalis®) against whiteflies on protected crops. IOBC WPRS Bull (2006) 29(4):83–8.
Keywords: Beauveria, Lycorma delicatula, entomopathogenic fungi, planthopper, invasive insect
Citation: Clifton EH, Castrillo LA, Jaronski ST and Hajek AE (2023) Cryptic diversity and virulence of Beauveria bassiana recovered from Lycorma delicatula (spotted lanternfly) in eastern Pennsylvania. Front. Insect Sci. 3:1127682. doi: 10.3389/finsc.2023.1127682
Received: 19 December 2022; Accepted: 16 March 2023;
Published: 25 April 2023.
Edited by:
Houping Liu, Pennsylvania Department of Conservation and Natural Resources, United StatesReviewed by:
Kier Klepzig, University of Georgia, United StatesPaul Abram, Agriculture and Agri-Food Canada (AAFC), Canada
Copyright © 2023 Clifton, Castrillo, Jaronski and Hajek. This is an open-access article distributed under the terms of the Creative Commons Attribution License (CC BY). The use, distribution or reproduction in other forums is permitted, provided the original author(s) and the copyright owner(s) are credited and that the original publication in this journal is cited, in accordance with accepted academic practice. No use, distribution or reproduction is permitted which does not comply with these terms.
*Correspondence: Eric H. Clifton, ZWNsaWZ0b25AYmlvd29ya3NpbmMuY29t