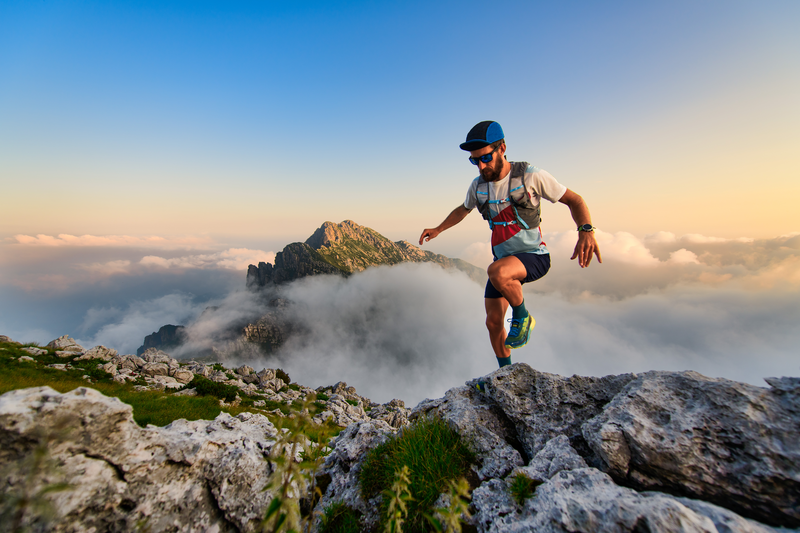
95% of researchers rate our articles as excellent or good
Learn more about the work of our research integrity team to safeguard the quality of each article we publish.
Find out more
ORIGINAL RESEARCH article
Front. Insect Sci. , 05 September 2022
Sec. Invasive Insect Species
Volume 2 - 2022 | https://doi.org/10.3389/finsc.2022.950815
This article is part of the Research Topic Focus on Fall Armyworm View all 6 articles
Fall armyworm (FAW) Spodoptera frugiperda (J.E. Smith) has become a major threat to maize production in Africa. In this study, six maize genotypes were assessed for their resistance to FAW under artificial infestation in both laboratory and net house conditions. These included two FAW-tolerant hybrids (CKHFAW180294 and CKH191221), two commercial hybrids (WE2115 and CKH10717), and two open-pollinated varieties (ZM523 and KDV4). Larval development time and reproductive potential were assessed on maize leaves in the laboratory and a life table for FAW was constructed. The maize genotypes were also artificially infested with three FAW neonates at two phenological stages (V5 and V7) and reproductive stage (R1) in the net house. Leaf and ear damage scores were recorded on a scale of 1–9. Larval development time varied significantly between maize genotypes with the highest on CKH191221 (16.4 days) and the lowest on KDV4 (13.7 days). The intrinsic rate of natural increase for life tables varied from 0.24 on CKH191221 to 0.41 on KDV4. Mean generation time of FAW ranged from 17.6 to 22.8 days on KDV4 and CKH191221, respectively. Foliar damage was the lowest on CKH191221, and the highest on KDV4 at V7 infestation stage in week 1. CKH191221 had the lowest ear damage score, whereas ZM523 had the highest scores at V5 infestation stage. The highest and lowest yield reductions were observed on ZM523 (64%) at V7 infestation stage and CKHFAW180294 (6%) at R1 infestation stage, respectively. The results indicated the potential for developing tropical mid-altitude maize germplasm with native genetic resistance to FAW.
Maize (Zea mays L.) is one of the most important staple food crops in Sub-Saharan Africa (SSA), contributing to the food security and livelihoods of millions of smallholder farmers (1). In the last 6 years, maize production in SSA has been constrained by the invasion of fall armyworm (FAW), Spodoptera frugiperda (J.E. Smith) (Lepidoptera: Noctuidae). This pest, native to the Americas, was first formally reported in Africa in 2016 (2) and, since then, has caused substantial maize yield losses (3–6). In SSA, FAW encountered suitable ecological conditions and quickly became one of the most damaging pests of maize within 2 years of arrival on the continent (7). The FAW larvae feed on young whorls, ears, and tassels of maize plants (8). Since 2016, an array of management techniques was tested, validated, and deployed in Africa. These methods include agroecological control that applies knowledge about the complex interactions and the environment, e.g., push–pull technology and cultural control such as crop rotation and intercropping. The biological control using organisms or their components has achieved an efficient strategy for managing the pest such as adoption of natural enemies, such as Trichogramma spp. and Telenomus spp., as well as the biorational control using botanicals and biopesticides, such as nucleopolyhedroviruses. Host plant resistance (HPR) including transgenic expressing Bacillus thuringiensis (Bt) toxins can also be used to control FAW and chemical pesticide; many of these products are too expensive and inaccessible to be considered by many smallholder farmers in SSA. Each of these management options has limitations when used alone. An integrated approach is the best options for sustainability in controlling FAW in Africa (7, 9).
HPR is a control strategy that focuses on identification and deployment of germplasm with native genetic resistance to FAW. It is a key component of any pest management strategy (7, 9–11). There is a need to develop and deploy insect-resistant crop varieties as a main component of an Integrated Pest Management strategy against FAW in Africa and Asia (7, 9–12). The quantitative or polygenic nature of native genetic resistance to FAW offers the opportunity to minimize selection pressure on the pest and prevents emergence of new resistant strains. Resistance to insect pests is the ability to minimize feeding damage through mechanisms/types such as antixenosis, antibiosis, and tolerance (13). “Antixenosis” denotes presence of morphological or chemical factors that alter insect behavior, resulting in poor establishment of the insect pests (poor feeding, rejection of oviposition, and delay acceptance as host). “Antibiosis” is the effect of the host plant on the biology (low survival rate, longer development period, and low adult fecundity due to biochemical factors). “Tolerance” is the capacity of the plant to withstand insect attack and give optimum yields despite the insect damage (with minimal yield penalty).
The International Maize and Wheat Improvement Center (CIMMYT) has engaged in developing germplasm with insect resistance since the 1980s, and multiple borer-resistant (MBR) and multiple insect-resistant tropical (MIRT) inbred lines and populations were developed. With the outbreak of FAW on the African continent, CIMMYT started an extensive effort to screen under artificial infestation large sets of germplasm [inbred lines, hybrids, improved open-pollinated varieties (OPVs), and populations such as MBR and MIRT] from earlier breeding efforts at CIMMYT and initiatives like the more recent Insect Resistant Maize for Africa (9–12).
From 2017 to 2019, over 3,300 maize genotypes were tested under FAW artificial infestation at Kiboko. This preliminary screening led to the identification of several inbred lines and OPVs with tolerance and/or resistance to FAW. Some of the inbred lines identified were used to form more than 2,030 hybrids that were also tested under FAW artificial infestation at Kiboko. Two promising hybrids were identified and considered tolerant due to their lower leaf, ear damage, and higher grain yield under FAW artificial infestation. However, no detailed studies have been undertaken to investigate the basis of tolerance and/or resistance to FAW in this germplasm. This study therefore investigated different parameters to explain the basis of tolerance or resistance in a set of genotypes. The bionomic parameters observed include the pre-imaginal developmental time, larval and pupal weight, adult longevity, fecundity, sex ratio, and emergence rate to FAW on six selected maize genotypes under laboratory conditions. The extent of damage caused by FAW on the same set of genotypes under FAW artificial infestation in net house conditions was also evaluated.
Six maize genotypes were selected for this study. These included two FAW-tolerant experimental hybrids (CKH191221 and CKHFAW180294), two commercial hybrid checks (WE2115 and CKH10717), and two popular commercial OPVs (ZM523 and KDV4). All the genotypes were developed at CIMMYT using different source germplasm selected for various attributes including high yield potential, drought tolerance, early/intermediate maturity, and tolerance to stem borers. CKH191221 and CKHFAW180294 were developed in 2018, and their parents have a background of insect-pest resistance. WE2115 and CKH10717 are drought-tolerant hybrids that are commercially grown in Kenya and Tanzania, respectively. ZM523 is a drought-tolerant intermediate maturity OPV that is commercially grown in several countries across SSA, whereas KDV4 is an early maturing OPV that is extensively commercialized in Kenya. These OPVs were developed from multiple stress-tolerant inbred lines.
A colony of FAW was established during the long rain season of 2017 by collecting 100 larvae and 10 pupae at Kiboko (2°15′S 37.75′E, 975 masl) and 50 larvae and 10 pupae at Machakos (1°31′S, 37°16′E) in Kenya. The colony was maintained in the insectary at Kenya Agricultural and Livestock Research Organization (KALRO) at Katumani (1°35′S, 37°15′E, 1,610 masl) on artificial diet as described by Prasanna et al. (10). In brief, an artist brush was used to place 30 neonates in a jar (12 × 14.5 × 11.5 cm). At the third instar, one larva was placed on each diet vial (2.5 × 8.5 × 2.5 cm) until pupation. The pupae were placed in a petri dish in the oviposition cage until emergence to allow the pairing and egg laying. The FAW colony is maintained under controlled conditions at 27 ± 1°C; 12:12 h (light, dark) and 70 ± 5% RH (10).
Life table studies for FAW were conducted at the KALRO-Katumani Insectary in 2019. Three maize seeds of each genotype were planted per pot measuring 15 cm diameter and 30 cm height. A total of 30 pots for each maize genotype were established in a screenhouse. Soil obtained from Katumani (Ferrosol) with dry manure at a ratio 3:1 (soil: manure) was used. Maize leaves from V5 to V10 were used as natural diet for rearing the FAW larvae. A single freshly emerged neonate was picked using a fine soft camel hairbrush and placed in a 25-ml plastic vial with screw cap (with pinholes to allow air circulation and prevent escape of neonates or larvae) measuring 2.5 × 6 cm with two pieces of fresh leaves (3.0 cm each) from each of the test genotypes. A piece of napkin was placed in the vial to absorb moisture. The vials were then kept in an insect rearing laboratory room with controlled conditions (27 ± 1°C; 12:12 h (light, dark), and 70 ± 5% RH). The neonates were then observed daily for mortality until pupation. The diet was changed after every 2 days to allow ample feeding time. Weight (mg) of the larvae was taken after 6 and 9 days using a Shimadzu electronic balance (Type ATY224 AP, Japan). The duration of larval and pupal development period was recorded. The pupae were kept under the same conditions as larvae until adult emergence. The experiment was arranged in a randomized complete block design with three replications (insect cohorts) and 80 insects (vials) in each replication (n = 3 × 80 = 240).
The newly emerged naïve adults (without oviposition experience) were paired using moths reared from the same maize genotype for mating and egg laying. Three leaf pieces (3–5 cm) from the respective maize genotypes were placed in a plastic container measuring 11 × 15 × 10 cm where moth pairs were introduced for mating and oviposition. The container was covered with a perforated lid for ventilation. The moths were fed on a 10% sugar solution soaked in cotton wool. After every 24 h, the leaf pieces were changed, and the eggs laid counted under a dissecting microscope (type Optika Vision Lite 2.13) and recorded. This procedure was repeated until the female moths died. The eggs laid on the leaf pieces of the different maize genotypes were placed in individual petri dishes for hatching. The number of neonates that emerged after hatching was counted to determine the viability of the eggs.
FAW artificial infestation trial was conducted at the FAW Screening Facility at Kiboko, Kenya, in 2019. The mean temperature at Kiboko ranges from 16.5°C to 28.6°C. The rainfall pattern is bimodal with sometimes unpredictable short rains between March and April and long rains from October to December. The average precipitation is 545–629 mm per year. The soils are classified as Acri-Rhodic Ferrassol. The soil obtained from Kiboko (Ferrasols to luvisols) with dry manure at a ratio 5:1 (soil manure) was used. Fertilizer application was done at the rate of 60 kg of nitrogen and 60 kg of phosphorus (P2O5) per hectare. Manual weeding was carried out when necessary to keep the plots clean. The plants were regularly irrigated.
The trial was set up as a split plot design with three replications at Kiboko, Kenya, in insect-proof screenhouses (gauges 0.4 × 0.9 mm) measuring 1,000 m2, divided into four compartments, each measuring approximately 250 m2. The main plot treatments were three phenological stages: V5 (five leaves fully emerged), V7 (seven leaves fully emerged), R1 (silking), and the control (compartment without FAW infestation). The subplots were the six maize genotypes. Each subplot consisted of nine rows measuring 3 × 6.75 m, planted at spacing of 0.25 × 0.75 m with a space of 1 m between the subplots giving a total of 324 plants per subplot. Two seeds were sown per hill, and thinning was done at 2 weeks after planting leaving one plant per hill. Maize genotypes were artificially infested with three FAW neonates per plant at each of the three phenological stages (V5, V7, and R1) following the procedure described by Prasanna et al. (10). The infestation done at V5 and V7 phenological stages had four generations of insects before harvesting, whereas the one done at R1 phenological stage had two generations of insects before harvesting. Artificial infestation was done between 4 p.m. and 6 p.m. to allow the neonates to acclimatize to the environment before the following day changes in temperatures and relative humidity that may otherwise desiccate the neonates. An insect-proof screenhouse compartment planted with maize genotypes without any FAW infestation was included as control for each replication.
Foliar damage was assessed 1 week after infestation and, subsequently, weekly on five and four instances for the V5 and V7 treatments, respectively. Foliar damage score was not assessed for the infestation done at the R1 (silking) stage. At harvest, ears were handpicked from all plants in each plot. Ear damage, percentage ear rot, number of exit holes, and insect stem tunnel length were recorded. Foliar and ear damage was assessed using a scale of 1–9, where 1 = no visible damage and 9 = completely damaged (10) (score 1 to 2 is considered as resistant, 3 to 5 as tolerant and more than 5 as susceptible for foliar damage rating). Rotten percentage on the ear was assessed by determining the percent area of the ear affected by fungal molds (following FAW attack of the ear). The rotten percentage was evaluated by determining the percentage of each ear covered by symptoms using a class rating scale of 1 to 9, in which 1 = 0%, 2 = 1%–20%, 3 = 21%–30%, 4 = 31%–40%, 5 = 41%–50%, 6 = 51%–60%, 7 = 61%–70%, 8 = 71%–80%, 9 = 81%–100% of the kernels exhibiting visible symptoms of rotten grain. Grain weight was measured, and a sample of the grain was used to determine moisture content for each plot. Grain yield (t ha−1) was calculated from grain weight with adjustment for grain moisture content and shelling percentage. In addition, the percentage yield loss was calculated.
Statistical analysis of life tables was performed using the Jackknife method as described by Hulting et al. (14). The pre-imaginal survivorship was calculated by dividing the number of individuals alive until adult emergence by the number of eggs laid by each cohort. The differences in intrinsic rate of increase (rm) values among populations reared on each maize genotype were calculated following the protocol described by Dixon (15). The intrinsic rate of increase is the number of births minus the number of deaths per generation time. Comparisons among the six hybrids were performed using Newman-Keuls sequential tests (16, 17) based on jackknife estimates of variance for rm values (18). For any difference between two rm from the sequence to be significant at the α level, the difference must be equal to or greater than
where K is the number of rm values in the set whose range is tested, and V is the degrees of freedom. The ni and nj are the sample sizes of the rm values, and Qα[K,V] is a value from the table of the studentized range. S2av is the weighted average variance of rm, and it is calculated as
The sample size of the ith rm is ni, and S2i is the jackknife estimate of the variance for the ith rm.
Analysis of variance for different parameters recorded in laboratory and net house studies was performed with the PROC GLM and PROC GLIMMIX of SAS (19) for parametric and non-parametric data, respectively. Cohort was considered as a block effect in the model and each insect unit as a sampling error. The mean percentage data on hatchability, relative growth rate (RGR), absolute increment, pre-imaginal development time, adult longevity, and female fecundity were then subjected to the Tukey’s honest significantly difference (HSD) test. The leaf damage was analyzed using a repeated measures analysis consisting of two main effects: V5 and V7 infestation stages and FAW damage in 1, 2, 3, 4, and 5 weeks, respectively. The degree of freedom (df) was calculated using the Kenward and Roger II method (20). Ear damage, percentage rotten ear, number of stem exit holes, percentage of cumulative tunnel length, grain yield, and percentage yield loss were separated using HSD test at P = 0.05.
Percentage data (rotten, cumulative tunnel length) were arc sin–transformed, whereas count data were log-transformed before analysis although non-transformed results are presented in the tables and figures. Spearman correlation analysis was performed to determine the pairwise association among various damage parameters due to FAW and grain yield using PROC CORR of SAS (19). Stepwise regression was conducted to determine the effect of different FAW damage parameters on grain yield using PROC REG of SAS (19).
Because the growth rate did not differ significantly between males and females, the full data set was used in the analysis. The RGR (defined as relative logarithmic weight/time increase in insect biomass) was calculated for each genotype as follows (21):
Absolute increment (AI, defined as the body mass growth rate relative to the initial body mass in time) was calculated as follows:
Percentage yield loss was calculated for each genotype as follows:
where yield protected was the yield under non-infestation (control) and yield infested was the yield under FAW artificial infestation at V5, V7, and R1.
Maize genotype had a significant effect on larval development time, pre-imaginal development time, and the longevity of female FAW (Table 1). Larval development time was longest on the FAW-tolerant hybrid CKH191221 and shortest on FAW-susceptible OPV KDV4. Similarly, pre-imaginal development time was shortest on KDV4 and longest for larvae reared on the FAW-tolerant hybrids CKHFAW180294 and CKH191221 (Table 1). Female longevity was longer on CHK191221, CKHFAW180294, and KDV4 compared with ZM523.
Table 1 Mean (± S.E.) development time and female longevity of FAW larvae reared on leaf discs of six maize genotypes under controlled conditions at Katumani, Kenya, in 2019.
The RGR of FAW, absolute increment, fecundity, and egg hatchability differed significantly (P ≤ 0.0001) among the maize genotypes (Table 2). The lowest growth rate was observed for larvae reared on CKHFAW180294 and highest on OPVs, KDV4, and ZM523. The lowest and highest absolute increment was observed on the tolerant hybrid CKH191221 and OPV KDV4, respectively. Significantly more eggs were laid by FAW adults on the susceptible commercial check CKH10717 compared with adults on the tolerant hybrid CKH191221. Percent egg hatchability ranged from 55.2% for the tolerant hybrid CKHFAW180294 to 91.5% for KDV4 (Table 2).
Table 2 Mean (± S.E.) relative growth rate, absolute increment of FAW larvae, female fecundity, and percentage egg hatchability on six maize genotypes, at Katumani, Kenya, in 2019.
There were significant differences among genotypes for intrinsic rates of increase (rm), net reproductive rate (Ro), and mean generation time (G) (Table 3). A significantly lower rm was observed for larvae reared on tolerant hybrid CKH191221 compared with the commercial checks (Table 3). A similar trend was observed for net reproductive rate (Ro). Conversely, mean generation time (G) was higher for larvae reared on the tolerant hybrids, compared with the commercial hybrid and OPVs.
There was a significant difference in foliar damage among the scores taken at different periods (F = 346.25; df = 7,47.72; P< 0.0001) and among the genotypes tested (F = 11.69; df = 5,95.63; P = 0.0008). The interaction between the genotypes tested and the time of leaf damage score was significant (F = 2.49; df = 35,40.92; P = 0.0028) as well as the interaction between the genotypes tested and the infestation treatments (V5 and V7) (F = 3.65; df = 5,9.604; P = 0.0407). The lowest leaf damage was recorded in week 1 irrespective of treatment. The tolerant hybrid CKH191221 had the lowest leaf damage at V5 and V7 infestation stages at 1, 2, 3, and 5 weeks after infestation (Figure 1). Average leaf damage was lower for the tolerant hybrids and higher for the commercial checks and OPVs for both vegetative infestation stages.
Figure 1 Leaf damage score on maize at V5 and V7 phenological stages under artificial infestation with FAW in an insect-proof net house at Kiboko, Kenya in 2019. Bars with the same lower-case letter(s) by genotypes and same capital letter in weeks are not signific antly different (SNK test; P < 0.05).
Ear damage, percentage rotten ear, exit holes, tunnel length, and grain yield differed significantly among the maize genotypes at all three phenological stages (Table 4). Hybrid CKH191221 had the lowest ear damage for the three phenological stages (V5, V7, and R1), whereas the highest ear damage was recorded on ZM523 and WE2115 at V5 and V7 phenological stages. For infestation done at R1 stage, WE2115 showed the highest ear damage (F = 4.90; df = 15,36; P< 0.0001). Rotten ear percentage significantly differed between the hybrids and OPVs with the lowest percentage recorded on hybrids CKH191221 and CKHFAW180294 (F = 9.44; df = 3,36; P< 0.0001). The number of stem exit holes was higher on OPV KDV4 for infestation at all three phenological stages compared with CKH191221 (F = 2.00; df = 15,36; P = 0.0425). A similar trend was recorded with percentage tunnel length for all infestation treatments (F = 1.94; df = 15,36; P = 0.0493).
Table 4 Mean (± S. E.) ear damage, grain yield, and other parameters of six maize genotypes under artificial infestation with FAW at different phenological stages and control (no infestation) at Kiboko, Kenya, 2019.
Grain yield varied significantly among genotypes for all treatments (Table 4) (F = 10.34; df = 15,36; P< 0.0001). Grain yield was significantly higher in the non-infested controls compared with the infested plots. Compared with the corresponding controls, grain yield was significantly reduced in the commercial check hybrid WE2115 by 46.8% and 56.9% at V5 and V7 phenological stages, respectively. Similarly, there was 64.2% yield reduction on the OPV ZM523 at V7 stage. However, on the FAW-tolerant hybrids, yield reductions were significantly lower: 38% at V7 stage for CKH191221 and 25% at V5 for CKHFAW180294 (Table 4) (F = 0.92; df = 10,24; P = 0.0333).
For quantitative and statistical analysis of the effect of FAW damage to maize grain yields, spearman correlation and stepwise regressions were used with grain yields in each phenological stage as a dependent variable and leaf damage (weeks 1–5), ear damage, rotten ear percentage, number stem exit holes, and percentage cumulative tunnel length as independent variables.
The correlations between grain yield and FAW damage parameters exhibited significant association among the test genotypes. Grain yield was significantly and negatively correlated with leaf damage at V5 (−0.505 on WE2115, −0.952 on CKH10717, and −0.735 for ZM523) and and V7 (−0.799, −0.657, −0.804, and −0.574 for CHKFAW190284, WE2115, ZM523, and KDV4, respectively). Similarly, grain yield was significantly and negatively correlated with ear damage at V5 (−0.754 for WE2115 and −0.739 for ZM523), V7 (−0.549, −0.928, and −0.663 for CHK191221, WE2115, and ZM523, respectively), and R1 (−0.603, −0.933, and −0.584 for CKH10717, ZM523, and KDV4, respectively). The number of exit holes was negatively correlated with yield grain at V5 (−0.506, −0.614, −0.855, −0.695, −0.609, and −0.573 for CHK191221, CHKFAW190284, WE2115, CHK10717, ZM523, and KDV4, respectively), V7 (−0.873 for WE2115), and R1 (−0.768, −0.780, −0.731, −0.843, and −0.547 for CHK191221, WE2115, CHK10717, ZM523, and KDV4, respectively). Grain yield was significantly and negatively correlated with the percentage of rotten ear [phenological stages V5 (−0.559, −0.625, −0.659, and −0.504 for WE2115, CHK10171, ZM523, and KDV4, respectively), V7 (−0.873 for WE2115), and R1 (−0.508, −0.487, and −0.931 for CHKFAW190284, WE2115, and ZM523, respectively)]. The percentage cumulative tunnel length was negatively correlated to the grain yield among tested genotypes at V5 (−0.649, −0.830, −0.875, −0.762, and −0.515 for CHKFAW190284, WE2115, CHK10717, ZM523, and KDV4, respectively), V7 (−0.577, −0.787, −0.566, −0.610, −0.732, and −0.851 for CHK191221, CHKFAW190284, WE2115, CHK10717, ZM523, and KDV4, respectively), and R1 (−0.528, −0.839, −0.530, and −0.928 for CHK191221, CHKFAW190284, WE2115, and ZM523, respectively).
For all three infested treatments at different phenological stages (V5, V7, and R1), stepwise regressions showed that leaf damage across 5 weeks negatively affected grain yields (Table 5). Across all genotypes and among all phenological stages, ear damage significantly affected grain yield.
Table 5 Stepwise regression analysis between FAW damage parameters and grain yield of six maize genotypes artificially infested with FAW at V5, V7, and R1 stages (n = 432).
This study showed that the FAW-tolerant hybrids exhibited longer pre-imaginal development time in FAW than susceptible genotypes and, in the case of CKH191221, a significantly lower female fecundity. Development time recorded in this study was lower by 50% to 60% compared with that reported on maize infested by FAW in the United States (22). Tendeng et al. (23) demonstrated that the total duration of FAW reared on maize was between 22 and 28 days at 25°C, with FAW producing an average of 15 generations per year. In this study, a similar trend was observed though with a longer developmental time on the FAW-tolerant hybrids compared with the susceptible genotypes. Previous studies on larval developmental time in maize reported 11 days (24), 21 days (25), and 14 days (23) at 25°C. These differences larval developmental period can be explained by the environmental conditions and the larval capacity to consume the host plant as well as the experimental setup (26, 27).
The two FAW-tolerant genotypes showed the lowest RGRs and absolute increments, indicating an antibiosis effect. Similarly, Lima et al. (28) and de Paiva et al. (29) reported antibiosis for FAW as shown by a low larval viability, small larval weight, short adult longevity, and low emergence rates. Genotypes CKH191221 and CKHFAW180294 had the least number of eggs laid. Female fecundity recorded in this study was similar to that reported in several studies (25, 30, 31) but is lower than those reported by Kumela et al. (32) and Tendeng et al. (23). The low female fecundity observed on the tolerant maize genotypes could be attributed to components of host plant quality such as carbon, nitrogen, and defensive metabolites, which directly affect the fecundity (33).
Differences in life table parameters like in developmental time, survival rate, and fecundity of FAW reared on the different genotypes might be explained by allelochemicals present in the leave such as alkaloids, glucosinolates, protein inhibitors, and lipids (34, 35). The mean generation times (G) recorded in this study were 9% to 40% shorter than those reported by Pinto et al. (36) on five maize genotypes. Pinto et al. (36) reported a Ro of 755 to 920, which was in the same range as that obtained on the susceptible genotypes. The number of eggs laid by an insect is usually determined at oogenesis, in which physiological processes are determined by availability of nutrients in the female’s body (37). This is mainly caused by the adequate food ingested and assimilated during larval development (36, 38). The performance of FAW on the susceptible hybrids and OPVs in this study may be related to the higher concentration of nutrients such as proteins and amino acids in the food resource as well the ease of digestibility (35). Conversely, the tolerant hybrids appeared to have factors that inhibit the growth of FAW, resulting into lower fecundity.
Under artificial infestation, FAW-tolerant hybrids showed lower damage at V5 and V7 phenological stages. Maize infested early suffered more damage compared with that infested at a later phenological stage (39). On older leaves, foliar damage was reduced, which is attributed to leaf tissues being tough and indigestible, thereby limiting FAW feeding and growth (40). A significant negative relationship between grain yield and FAW damage was observed at V5 and V7 phenological stages, implying that leaf damage at V5 and V7 significantly affected yields of all the tested genotypes. A study by Thomison et al. (41) estimated that 70% defoliation at the 12-leaf stage would result in a 15% yield reduction, whereas 25% defoliation never caused more than 9% yield loss and 50% defoliation at 18-leaf stage the damage caused less than 5% yield loss.
Under high infestation pressure, some larvae evade cannibalism by entering the stem resulting tunneling and exit holes (8, 42). Tunnel length was shorter in the tolerant than susceptible hybrids and OPVs. Tunneling leads to reduced movement of nutrient in the plants. Thus, tunnel length and number of stem exit holes can be translated into grain yield loss (43–45) and can be used as parameters to assess severity of FAW infestation. Similar to the findings by Nwanze et al. (46), grain yield was negatively correlated with number of exit holes and cumulative tunnel length in this study.
The effect on grain yields due to FAW infestation across all the phenological stages differed significantly among genotypes. Higher yield loss was recorded for infestation treatments V5 and V7 as compared with R1. When the infestation is done at the vegetative stage, larvae move to the whorl, thereafter to the emerging tassel, and then to the developing ear (40). The larvae first feed on the husks before reaching the developing kernels after penetrating the ear through the tip (47, 48). A yield reduction of 15% when 98% of plants were artificially infested by FAW at 8- to 10-leaf stage was reported by Cruz and Turpin (8). These authors also reported 18% yield reduction with 31% FAW infestation. This is in line with studies by Hruska and Gladstone (49), who carried out a study with 100% infestation of the plants and recorded a 34% yield reduction by FAW. In early stages of crop development FAW, attacks maize extensively causing a lot of defoliation. In case of attack during the late stages of crop growth, FAW larvae may cause lesser damage to the grain yield compared with early attack. The results indicate that the larval stage at the early phenological stages (V5 and V7) might be the most appropriate time to target management options because a much higher population suppression is achievable with small increment of mortality (50) and for germplasm screening.
The FAW-tolerant experimental hybrids led to lower FAW growth rates and fecundity in laboratory studies and showed lower insect damage parameters and grain yield loss, as compared with the susceptible genotypes in net house experiments. This study should serve as a baseline for further studies on resistance to FAW in tropical maize germplasm and validation of resistance in other sources of maize germplasm. Laboratory assays and net house trials do not necessarily reflect the exact conditions encountered under field conditions. Therefore, it is important to screen the genotypes under field conditions to ascertain their performance under natural FAW pressure in the target agroecological conditions.
The original contributions presented in the study are included in the article/supplementary material. Further inquiries can be directed to the corresponding author.
Conceptualization: GNA, AYB, MA, BMP, DM, and YB. Methodology: GNA, AYB, RK-G, and SEN, MA, DM, and YB. Software: GNA and AYB. Validation: AYB, RK-G, and SEN. Formal analysis: GNA and AYB. Data curation: GNA and AYB. Writing—original draft preparation: GNA. Reviewing: AYB, RK-G, DM, YB, MA, SEN, and BMP. Project administration: BMP and MA. Funding acquisition: BMP and AB. All authors contributed to the article and approved the submitted version.
The study was undertaken through the financial support from the United States Agency for International Development (USAID) Bureau for Resilience and Food Security under Grant # BFS-IO-17-00005 as part of Feed the Future activity, under the Fall Armyworm Management Project to CIMMYT, and the CGIAR Research Program on Maize (MAIZE) Windows 1 and 2. Any opinions, findings, conclusions, or recommendations expressed here are those of the authors alone.
The authors are grateful for the collaboration extended by the Kenya Agricultural and Livestock Research Organization (KALRO) Research Centers at Machakos and Kiboko and to the Department of Agriculture, Science and Technology, Kenyatta University, for necessary support. Special thanks to CIMMYT Technicians Charles Marangu and Gerphas Ogola for their technical support for this study.
Author MA is employed by Amobant LLC but previously worked at CIMMYT.
The remaining authors declare that the research was conducted in the absence of any commercial or financial relationships that could be construed as a potential conflict of interest.
All claims expressed in this article are solely those of the authors and do not necessarily represent those of their affiliated organizations, or those of the publisher, the editors and the reviewers. Any product that may be evaluated in this article, or claim that may be made by its manufacturer, is not guaranteed or endorsed by the publisher.
1. Erenstein O, Jaleta M, Sonder K, Mottaleb K, Prasanna BM. Global maize production, consumption, and trade: trends and R&D implications. Food Secur (2022) 11:1–25. doi: 10.1007/s12571-022-01288-7
2. Goergen G, Kumar PL, Sankung SB, Togola A, Tamò M. First report of outbreaks of the fall armyworm Spodoptera frugiperda (J e smith) (Lepidoptera, noctuidae), a new alien invasive pest in West and central Africa. PloS One (2016) 11(10):1–9. doi: 10.1371/journal.pone.0165632
3. Day R, Abrahams P, Bateman M, Beale T, Clottey V, Cock M, et al. Fall armyworm: Impacts and implications for Africa. Outlook Pest Manage (2017) 28(5):196–201. doi: 10.1564/v28_oct_02
4. Rwomushana I, Bateman M, Beale T, Beseh P, Cameron K, Chiluba M, et al. Fall armyworm: Impacts and implications for Africa evidence note update, October 2018. Outlook Pest Manag (2017), 51. doi: 10.1564/v28_oct_02
5. de Groote H, Kimenju SC, Munyua B, Palmas S, Kassie M, Bruce A. Spread and impact of fall armyworm (Spodoptera frugiperda J.E. smith) in maize production areas of Kenya. Agric Ecosyst Environ (2020) 292:106804. doi: 10.1016/j.agee.2019.106804
6. Kassie M, Wossen T M, de Groote H, Tefera T, Sevgan S, Balew S. Economic impacts of fall armyworm and its management strategies: evidence from southern Ethiopia. Eur Rev Agric Econ (2020) 47(4):1473–1501. doi: 10.1093/erae/jbz048
7. Prasanna BM, Huesing JE, Peschke RM, Virginiam M. Fall armyworm in Africa: A guide for integrated pest management. 1st ed. Mexico, CDMX: CIMMYT (2018).
8. Cruz I, Turpin FT. Yield impact of larval infestations of the fall armyworm Spodoptera frugiperda (J. e. smith) to midwhorl growth stage of Corn1. J Econ Entomol (1983) 76:1052–4. doi: 10.1093/jee/76.5.1052
9. Prasanna BM, Huesing JE, Peschke RM, Virginiam M. Fall armyworm in Asia: A guide for integrated pest management. 1st ed. Mexico, CDMX: CIMMYT (2021).
10. Prasanna BM, Bruce A, Winter S, Otim M, Asea G, Sevgan S, et al. Host plant resistance to fall armyworm. In: Prasanna BM, Huesing JE, Eddy R, Peschke VM, editors. Fall armyworm in Africa: A guide for integrated pest management, 1st ed. Mexico, CDMX: CIMMYT (2018).
11. Prasanna BM, Bruce A, Beyene Y, Makumbi D, Gowda M, Asim M, et al. Host plant resistance for fall armyworm management in maize: relevance, status and prospects in Africa and Asia. Theor Appl Genet (2022). doi: 10.1007/s00122-022-04073-4
12. Otim MH, Fiaboe KKM, Akello J, Mudde B, Obonyom AT, Bruce AY, et al. Managing a transboundary pest: The fall armyworm on maize in Africa. Intechopen (2021) 96637:10.5772. doi: doi.org/10.5772/intechopen.96637
13. Painter RH. Insect resistance in crop plants, Vol. 72. (1951). p. 481. Macmillan, New York: Soil Science.
14. Hulting FL, Orr DB, Obrycki JJ. A computer program for calculation and statistical comparison of intrinsic rates of increase and associated life table parameters. Fla Entomol (1990) 73:600–612. doi: 10.2307/3495274
15. Dixon AFG. Parthenogenetic reproduction and the rate of increase in aphids. Amsterdam: Elsevier (1987) p. 269–87. Minks A.K. & P. Harrewijn.
16. Sokal RR, Rohlf FJ. Biometry. the principles and practice of statistics in biological research. Robert r. sokal and f. James rohlf. science. w. h. freeman and co., San Francisco, xxi + 776 pp. J Mammal (1970) 51(2):440–1. doi: 10.2307/1378519
17. Bailey K, Sokal RR, Rohlf J. Biometry: The principles and practice of statistics in biological research (2nd ed.). J Am Stat Assoc (1982) 77:946. doi: 10.2307/2287349
18. Meyer JS, Ingersoll CG, McDonald LL, Boyce MS. Estimating uncertainty in population growth rates: jackknife vs. bootstrap techniques. Ecology (1986) 67:1156–1166. doi: 10.2307/1938671
19. SAS Institute. SAS/STAT software: changes and enhancements through release Vol. 6. Cary, North Carolina (2019). p. 12.
20. Kenward MG, Roger JH. An improved approximation to the precision of fixed effects from restricted maximum likelihood. Comput Stat Data Anal (2009) 53:2583–95. doi: doi.org/10.1016/j.csda.2008.12.013
21. Scriber JM, Slansky F. The nutritional ecology of immature insects. Ann Rev Entomol (1981) 26:183–211. doi: 10.1146/ANNUREV.EN.26.010181.001151
22. Capinera JL. Fall armyworm, spodoptera frugiperda (J.E. smith) (Insecta: Lepidoptera: Noctuidae). Fla Entomol (2014) 7(2):873–9.
23. Tendeng E, Labou B, Diatte M, Djiba S, Diarra K. The fall armyworm spodoptera frugiperda (J.E. smith), a new pest of maize in Africa: biology and first native natural enemies detected. Int J Biol Chem (2019) 13(2):1011. doi: 10.4314/ijbcs.v13i2.35
24. Santos LM, Redaelli LR, Diefenbach LM, Efrom CF. Larval and pupal stage of spodoptera frugiperda (J. e. smith) (Lepidoptera: Noctuidae) in sweet and field corn genotypes. Braz J Biol (2003) 63(4):627–33. doi: 10.1590/S1519-69842003000400009
25. da Silva DM, Bueno ADF, Andrade K, Stecca CDS, Neves PMOJ, Oliveira MCND. Biology and nutrition of spodoptera frugiperda (Lepidoptera: Noctuidae) fed on different food sources. Sci Agric (2017) 7(1):18–31. doi: 10.1590/1678-992X-2015-0160
26. Barros EM, Torres JB, Ruberson JR, Oliveira MD. Development of spodoptera frugiperda on different hosts and damage to reproductive structures in cotton. Entomol Exp Appl (2010) 137:237–45. doi: 10.1111/j.1570-7458.2010.01058.x
27. Flanders K, Don B, Patricia C. Management of fall armyworm in pastures and hayfields. Ala Coop Ext Syst Ala Am Univ Auburn Univ (2017), 8.
28. Lima FWN, Ohashi OS, Souza FRS, Gomes FS. Avaliação de acessos de milho para a resistência a spodoptera frugiperda (Smith) (Lepidoptera: Noctuidae) em laboratório. Acta Amaz (2006) 32:147–50.
29. de Paiva LA, Corrêa F, Silva CLT, Moura TL, Silva FC, Araújo MS, et al. Resistance of corn genotypes to fall armyworm spodoptera frugiperda (Lepidoptera: Noctuidae). Afr J Biotechnol (2016) 15:1877–82. doi: 10.5897/AJB2016.15399
30. Murúa MG, Vera MT, Abraham S, Juaréz ML, Prieto S, Head GP, et al. Fitness, and mating compatibility of spodoptera frugiperda (Lepidoptera: Noctuidae) populations from different host plant species and regions in Argentina. Ann Entomol Soc Am (2008) 101:639–49. doi: 10.1603/0013-8746(2008)101
31. Sharanabasappa SD, Prasanna BM, Kalleshwaraswamy CM, Jagdish Jaba BC. Fall armyworm (Spodoptera frugiperda). In: Omkar, editor. Polyphagous pests of crops, 1st ed. Singapore: Springer. (2021). p. 350–72.
32. Kumela T, Simiyu J, Sisay B, Likhayo P, Mendesil E, Gohole L, et al. Farmers’ knowledge, perceptions, and management practices of the new invasive pest, fall armyworm (Spodoptera frugiperda) in Ethiopia and Kenya. Int J Pest Manage (2019) 65(1):1–9. doi: 10.1080/09670874.2017.1423129
33. Awmack CS, Leather SR. Host plant quality and fecundity in herbivorous insects. Ann Rev Entomol (2002) 47:817–44. doi: 10.1146/annurev.ento.47.091201.145300
34. Browne LB. Regulatory mechanisms in insect feeding. Adv In Insect Phys Elsevier (1975) 11:1–116. doi: 10.1016/S0065-2806(08)60162-9
35. Panizzi AR, Parra JRP. Introdução à bioecologia e nutrição de insetos como base para o manejo integrado de pragas. Brasília Embrapa Informação Tecnológica (2009), 1164p.
36. Pinto JRL, Torres AF, Truzi CC, Vieira NF, Vacari AM, De Bortoli SA. Artificial corn-based diet for rearing spodoptera frugiperda (Lepidoptera: Noctuidae). J Insect Sci (2019) 19(4):1–8. doi: 10.1093/jisesa/iez052
37. Cury KM, Prud’homme B, Gompel N. A short guide to insect oviposition: when where and how to lay an egg. J Neurogenet (2019) 33(2):75–85. doi: 10.1080/01677063.2019.1586898
38. Wiseman BR, Carpenter JE, Wheeler GS. Growth inhibition of fall armyworm (Lepidoptera: Noctuidae) larvae reared on leaf diets of non-host plants. Fla Entomol (1996) 79(3):302–311. doi: 10.2307/3495578
39. Harrison FP. Observations on the infestation of corn by fall armyworm (Lepidoptera: Noctuidae) with reference to plant maturity. Fla Entomol (1984) 67:333–5.
40. Kasoma C, Shimelis H, Laing M, Shayanowako AIT, Mathew I. Screening of inbred lines of tropical maize for resistance to fall armyworm, and for yield and yield-related traits. Crop Prot (2020) 136:105–218. doi: 10.1016/j.cropro.2020.105218
41. Thomison PR, Nafziger ED, Coulter JA, Zarnstorff ME, Geyer AB, Lindsey A. Response of corn to multiple defoliation events, in: oster presented at theAnnual Meeting of the American Society of Agronomy, November 2016. Poster Number 165-1520 at "Resilience Emerging from Scarcity and Abundance" 6-9 November 2016, Phoenix
42. Cruz IA. Lagarta−do−cartucho na cultura do milho. sete lagoas: Embrapa−CNPMS. Embrapa−CNPMS. Circulartécnica, 21 (1995). 45p.
43. Cardwell KF, Schulthess F, Ndemah R, Ngoko Z. A systems approach to assess crop health and maize yield losses due to pests and diseases in Cameroon. Agric Ecosyst Environ (1997) 65:33–47. doi: doi.org/10.1016/S0167-8809(97)00056-X
44. Chouraddi M, Mallapur C. Assessment of crop loss and economic injury level of maize stem borer, chilo partellus (Swinhoe). J Entomol Zool Stud (2017) 5(4):1530–5.
45. Ndemah R, Schulthess F, Korie S, Borgemeister C, Cardwell KF. Distribution, relative importance and effect of lepidopterous borers on maize yields in the forest zone and mid-altitude of Cameroon. J Econ Entomol (2001) 94:1434–44. doi: 10.1603/0022-0493-94.6.1434
46. Nwanze JAC, Bob-Manuel RB, Zakka U, Kingsley-Umana EB. Population dynamics of fall army worm [(Spodoptera frugiperda) J.E. smith] (Lepidoptera: Noctuidae) in maize-cassava intercrop using pheromone traps in Niger delta region. Bull Natl Res Cent 45 (2021) 44(1):1–8. doi: 10.1186/s42269-021-00500-6
47. Morrill WL, Greene GL. Distribution of fall armyworm larvae. 1. regions of field corn plants infested by larvae. Environ Entomol (1973) 2:195–8.
48. Morrill WL, Greene GL. Distribution of fall armyworm larvae. 2. influence of biology and behavior of larvae on selection of feeding sites. Environ Entomol (1973) 2:415–8. doi: 10.1093/EE/2.3.415
49. Hruska AJ, Gladstone SM. Effect of period and level of infestation of the fall armyworm, spodoptera frugiperda, on irrigated maize yield. Fla Entomol (1988) 71(3):249–254. doi: 10.2307/3495428
Keywords: maize hybrids, fall armyworm, life table, yield loss, artificial infestation
Citation: Anyanda GN, Bruce AY, Makumbi D, Ahonsi M, Kahuthia-Gathu R, Namikoye ES, Beyene Y and Prasanna BM (2022) Reproductive potential of fall armyworm Spodoptera frugiperda (J.E. Smith) and effects of feeding on diverse maize genotypes under artificial infestation. Front. Insect Sci. 2:950815. doi: 10.3389/finsc.2022.950815
Received: 23 May 2022; Accepted: 09 August 2022;
Published: 05 September 2022.
Edited by:
Fangneng Huang, Louisiana State University, United StatesReviewed by:
Michael J Stout, Louisiana State University, United StatesCopyright © 2022 Anyanda, Bruce, Makumbi, Ahonsi, Kahuthia-Gathu, Namikoye, Beyene and Prasanna. This is an open-access article distributed under the terms of the Creative Commons Attribution License (CC BY). The use, distribution or reproduction in other forums is permitted, provided the original author(s) and the copyright owner(s) are credited and that the original publication in this journal is cited, in accordance with accepted academic practice. No use, distribution or reproduction is permitted which does not comply with these terms.
*Correspondence: Anani Y. Bruce, QS5icnVjZUBjZ2lhci5vcmc=
Disclaimer: All claims expressed in this article are solely those of the authors and do not necessarily represent those of their affiliated organizations, or those of the publisher, the editors and the reviewers. Any product that may be evaluated in this article or claim that may be made by its manufacturer is not guaranteed or endorsed by the publisher.
Research integrity at Frontiers
Learn more about the work of our research integrity team to safeguard the quality of each article we publish.