- 1Instituto de Biotecnología, Universidad Nacional de San Juan (U.N.S.J), San Juan, Argentina
- 2Consejo Nacional de Investigaciones Científicas y Tecnológicas (CONICET), Godoy Cruz, Ciudad Autonoma de Buenos Aires, Argentina
- 3CEFET Centro Federal de Educação Tecnológica, Belo Horizonte, Mina Gerais, Brazil
Probiotic yeasts are gaining significant attention due to their numerous advantages over traditional bacterial probiotics. Yeasts from winemaking environments may possess unique attributes that enhance their probiotic potential. In the present study, fourteen yeast strains were evaluated based on previous in vitro studies regarding their biosecurity and tolerance to gastrointestinal tract conditions. Biosafety was assessed using an in vivo invertebrate model, specifically Galleria mellonella, and potential in vitro properties, including enzyme production, antioxidant activity, antagonistic effects against enteropathogens, and cholesterol- lowering capabilities, were explored. Notably, all strains were deemed biosafe. Assessment of enzyme production revealed that all strains produced lipase and phytase, while six exhibited protease activity and five showed β-glucosidase activity. All isolatesdemonstrated tolerance to oxidative stress, DPPH radical scavenging (with a maximum value of 76.46%), and hydroxyl radical trapping (with a maximum value of 85.69% in Wickerhamomyces anomalus). Antimicrobial activity was also evaluated, with one strain producing siderophores. Furthermore, all strains exhibited catalase activity and produced short-chain organic acids in varying proportions, including acetic acid (with a maximum value of 1.436 g l-1 in W. anomalus), lactic acid (with a maximum value of 2.196 g l-1 in Pichia manshurica), and propionic acid (with a maximum value of 2.312 g l-1 in W. anomalus. All but one strain produced ethanol, with the highest value of 9.056 g l-1 observed in P. kudriavzevii. Importantly, all strains demonstrated the ability to reduce cholesterol levels in the medium to varying extents, with a reduction of up to 47.5% observed in P. kudriavzevii. These findings provide a robust foundation for future investigations into the potential probiotic applications of these yeasts.
1 Introduction
Probiotics are microorganisms that, when administered in adequate amounts, confer health benefits to the host (Hill et al., 2014). The current probiotics market offers a wide range of products, especially of bacterial origin, but yeast probiotics have gained increased interest in both research and consumption (Staniszewski and Kordowska-Wiater, 2021). Yeasts are defined as unicellular eukaryotic microorganisms present in diverse ecological habitats like aquatic, aerial, and terrestrial environments, as well as part of the human microbiota (Azhar et al., 2017; Gil-Rodríguez and Garcia-Gutierrez, 2021). The growing interest in yeast probiotics is mainly the result of their broad applicability in the food industry and their distinctive properties in the digestive tract (Staniszewski and Kordowska-Wiater, 2021; Vergara et al., 2023a).
To determine if a microorganism can be considered a probiotic, it must first be assessed for its safety to humans (non-pathogenicity). It is important to note that one of the most relevant tests for a potential probiotic is the in vivo safety assay. In Argentina, the Argentine Food Code (AFC) requires that strains meet the protocol for evaluation of a probiotic as a food ingredient, including in vivo biosafety tests (AFC Joint Resolution 261/2011 and 22/2011). An in vivo assay with probiotic microorganisms is essential to understand their potential effects on the host. Traditionally, vertebrate animal models have been used in these tests, but due to ethical concerns, invertebrate models are gaining popularity. Invertebrate models offer several advantages, including a short life cycle, simple techniques, low larval maintenance costs, and a large number of individuals for statistical analysis (Tran et al., 2023). Additionally, there are structural and functional similarities between mammals and Galleria mellonella larvae regarding the innate humoral and cellular immune system (Garcia-Bustos et al., 2021).
Other relevant tests include tolerance to digestive tract conditions, adherence to epithelial cells, immunomodulatory effects, and exhibition of at least one beneficial effect on the host’s health (Vergara et al., 2023b). Sufficient documented studies must be required for a microorganism before being declared a probiotic (Hill et al., 2014). Some additional selection criteria proposed by authors are related to beneficial physiological effects on the body such as the production of enzymes that stimulate digestion, antioxidant activity, antagonistic activity against pathogens, and a reduction in cholesterol levels of the bloodstream (Ogunremi et al., 2015a; Fernández-Pacheco et al., 2021; Simões et al., 2021). Certain probiotic microorganisms play a crucial role in the metabolism of complex foods, as previously described by Ogunremi et al. (2015a). Through the secretion of enzymes that boost digestion, they improve the digestive capacity, facilitate nutrient absorption, and reduce the presence of specific antinutritional compounds. This metabolic activity contributes to the overall improvement of intestinal health in consumers (Mugwanya et al., 2021). The numerous enzymatic activities exhibited by probiotic microorganisms have a positive impact on digestive processes and, consequently, benefit the overall well-being of the individual (Syal and Vohra, 2013). Reactive oxygen species are mainly byproducts of respiration, the principal metabolic process occurring in eukaryotic cells. Maintaining cellular redox balance is crucial for cellular integrity; they have mechanisms to defend against and tolerate ROS, but an imbalance in their generation and elimination causes oxidative stress and damage to biomolecules (Li et al., 2012; Tripathy et al., 2021). One strategy implemented to mitigate this problem is the use of probiotic microorganisms as antioxidants. These microorganisms can play a significant role in reducing cellular damage by eliminating free radicals and preventing oxidation (Ragavan and Das, 2020). Some probiotics are documented as producers of antimicrobial compounds or antimicrobial agents that combat pathogenic microorganisms (Shruthi et al., 2022). Antagonistic mechanisms of yeasts against pathogens have been associated with: competition for specific nutrients, action of compounds excreted by yeasts in the medium, release of antimicrobial compounds, binding of pathogenic microorganisms to yeast cell walls, among others (Gut et al., 2018; Vergara et al., 2023a). According to Psomas et al. (2003), yeast cells can reduce cholesterol from liquid media. This property is sought after to prevent cardiovascular diseases, considering the limited success of drugs. Hypercholesterolemia, a significant factor in cardiovascular diseases, contributes up to 45% of myocardial infarction incidences (Kumar et al., 2012).
Over the years the Institute of Biotechnology of the National University of San Juan, Argentina, has evaluated different yeast species indigenous to the province of San Juan, Argentina. Strains were isolated from wine-related environments and examined for their properties and characteristics related to winemaking (Maturano et al., 2012, 2015, 2015b; Mestre Furlani et al., 2017; Kuchen et al., 2018, 2019). In a recent study, Vergara et al. (2023b) selected 14 non-conventional yeast isolates, which are potential probiotic candidates according to the studies conducted. Based on this background, the aim of the present study was to evaluate the pathogenicity of the previously selected non-conventional yeast using an in vivo invertebrate model. Subsequently, certain potential beneficial effects of the non-pathogenic yeasts were assessed: enzymatic production, antioxidant activity, antagonistic activity against pathogenic microorganisms (including possible mechanisms), and reduction of the cholesterol level.
2 Materials and methods
2.1 Microorganisms
The potentially probiotic yeast strains of viticulture origin, 14 non-conventional yeast isolates were selected for further investigation due to their exceptional results: Hanseniaspora guilliermondii (PB15), Pichia kudriavzevii (PB100, PB48, PB50, PB51, PB52, PB53), P. manshurica (PB54), P. occidentalis (PB56, PB57, PB58), Wickerhamomyces anomalus (PB97, PB98, PB99). These isolates were selected based on the following parameters: -Biosecurity traits: hemolytic activity and urease negativity, resistance to antibacterials (ampicillin 25, gentamicin 10, levofloxacin 10, rifampicin 20, tetracycline 80, chloramphenicol 60 μg ml-1), and sensitivity to at least 4 of the antifungals tested (ketoconazole 50, clotrimazole 10, fluconazole 10, itraconazole 10, miconazole 50, nystatin 100 μg ml-1). -Tolerance to gastrointestinal tract conditions: growth at 37, 39, and 42°C, resistance to acidic pH values (2 and 3) and bile salts (1%) with a bile resistance index (IR bilis) greater than or equal to 10. -Properties for adhering to intestinal epithelial cells: hydrophobicity, biofilm production (greater than the control strain), and autoaggregation (greater than 85%). -Tolerance to simulated gastrointestinal tract conditions (Vergara et al., 2023a, 2023c). All isolates are part of the Culture Collection of Autochthonous Microorganisms at the Institute of Biotechnology, Faculty of Engineering, UNSJ, San Juan, Argentina.
A commercial reference probiotic yeast was used: Saccharomyces cerevisiae var. boulardii CNCM I-745 (S.b.).
Yeast strains were activated using YEPD medium containing (g l-1): yeast extract 10; peptone 20; dextrose 20. Strains were incubated at 28 ± 1°C for 48 h under constant agitation at 100 rpm.
The following pathogenic bacteria and yeasts were activated in nutrient medium (g l-1): pluripeptone 5, meat extract 3. For 24 h at 37 ± 1°C: Klebsiella pneumonia ATCC 700603, Escherichia coli ATCC 25922, E. coli ATCC 35218, Pseudomonas aeruginosa ATCC 25853, Staphylococcus aureus ATCC 25923, Enterococcus faecalis ATCC 29212, Candida albicans ATCC 10231 (American type Culture Collection, ATCC, Rockville, MD, USA).
All microorganisms were cryopreserved at -80°C. Yeasts were cultivated in YEPD broth, and bacteria in Nutrient broth for 24 h before being used in experiments.
2.2 Evaluation of pathogenicity using Galleria mellonella
Six instar Galleria mellonella larvae (obtained from the Laboratory of Native Biocontroller Multiplier Center, INTA, Alto Valle) were incubated at 20 ± 1°C in the dark according the protocol by Peréz-Través et al. (2021). The larvae employed in the assay had an average weight of 0.22 ± 0.03 g and they were used within three days after reception. For each yeast species, 10 larvae were randomly selected and placed in Petri dishes with wood shavings.
Each larva was inoculated intradermally with 20 µl of a yeast suspension (1x106 cells ml-1) in the last left leg using a 1 ml insulin syringe. Larvae were acclimatized at 30 ± 1°C for 1 h prior to the experiments and subsequently incubated at 30 ± 1°C for 72 h in darkness. Survival was recorded after 24, 48, and 72 h and confirmed both visually and through physical stimulation using a sterile tip of an automatic pipette (Peréz-Través et al., 2021).
As a positive control, larvae were inoculated with 20 µl of a pathogenic strain (Pseudomonas aeruginosa ATCC 25853; 1x106 cells ml-1). As a negative control, larvae were inoculated with 20 µl of sterile PBS.
Survival was calculated as follow:
2.3 Enzyme activities
2.3.1 Qualitative enzymatic assays
In order to assay specific enzyme activities, 5 ml of a standardized suspension of each previously activated yeast (1x106 CFU ml-1) were spot inoculated on the surface of solidified agar plate, which was then incubated at 30 ± 1°C for 48 h. The following activities were assayed:
2.3.1.1 Lipase activity
Lipase activity, using the following medium (g l-1): peptone 5, yeast extract 3, tributyrin 10, and agar 15. Clear halos around colonies indicated lipase production (Maturano et al., 2009).
2.3.1.2 Amylase activity
Amylase activity, using the medium (g l-1): (NH4)2SO4 1, KH2PO4 1, Na2HPO4 0.9, MgSO4.7H2O 1, yeast extract 1, soluble starch 10, agar 15. After incubation, plates were streaked with Lugol’s iodine solution and left to react for 5 to 10 minutes. The presence of clear halos around colonies indicated amylase activity (Maturano et al., 2009).
2.3.1.3 Esterase activity
Esterase activity, using the following medium (g l-1): Glucose 20, yeast extract 5, peptone 5, CaCl2 0.1, tween 80 10, and agar 15. An opaque halo around the colony implied an esterase production (Maturano et al., 2009).
2.3.1.4 Protease activity
Protease activity, using the following medium (g l-1): yeast extract 3, malt extract 3, peptone 5, glucose 10, NaCl 5, and agar 15. Milk powder was dissolved in sterile distilled water (100g l-1 skim milk). Both solutions were mixed and poured into petri dishes under sterile conditions. The presence of a translucent halo around the colony indicated enzyme activity (Mestre Furlani et al., 2017).
2.3.1.5 β-galactosidase activity
β-galactosidase activity was examined on PDA medium (g l-1): potato extract 4, dextrose 20, and agar 15. 100 µl of IPTG (isopropyl β-D-1-thiogalactopyranoside) and 20 µl of X-gal (5-bromo-4-chloro-indolyl β-D-galactopyranoside) were poured on the plates and spread with a Drigalsky spatula. After 4 h at 25°C, 20 µl of each yeast strain were spot inoculated onto the plates. Colonies that turned blue were considered positive, while those that remained white were considered negative (Syal and Vohra, 2013, with modifications).
2.3.1.6 Phytase activity
Previously activated yeasts were inoculated at a concentration of 1x106 cells ml-1 in modified YEPD induction medium (g l-1): yeast extract 2, peptone 20, glucose 10, and sodium phytate 0.65. Cultures were incubated at 30 ± 1°C for 18 h, centrifuged at 6800 g for 10 min, and resuspended in saline solution (0.9% NaCl). Three growth media were prepared. The first was a phosphate-free minimal medium, which served as the negative control (g l-1): glucose 15, NH4NO3 5, CaCl2 2, MgSO4.7H2O 0.5, KCl 0.5, FeSO4.7H2O 0.01, MnSO4.H2O 0.01, and agar 15. A second medium containing minimal phosphate content was used as the positive control (g l-1): phosphate-free minimal medium + KH2PO4 2. Finally, a medium containing a minimal amount of phytate (g l-1): phosphate-free minimal medium + sodium phytate 2. Subsequently, 5 µl of each yeast strain was spot inoculated onto each agar growth medium. After the incubation period at 30°C for 48 h, the growth diameter for each colony was compared (Ogunremi et al., 2015a).
2.3.1.7 β-glucosidase
(g l-1): YNB without amino acids 6.7, Arbutin 5, Agar 20; 20 ml of 1% ferric ammonium citrate solution was added after autoclaving at 1 atm. After incubation (25°C for 168 h), medium turning brown was considered positive (Mestre Furlani et al., 2017).
2.3.2 Quantitative enzyme assays
2.3.2.1 Phytase activity
Activated yeast were centrifuged at 6,800 g for 10 min and then resuspended in saline solution. Then each yeast was inoculated at 1x106 cells ml-1 in a medium with minimal phytate content (g l-1): glucose 15, NH4NO3 5, CaCl2 2, MgSO4.7H2O 0.5, KCl 0.5, FeSO4.7H2O 0.01, MnSO4.H2O 0.01, and sodium phytate 3. Yeasts were incubated at 30 ± 1°C for 48 h under agitation at 85 g. Growth was determined by measuring optical density at 620 nm (Ogunremi et al., 2020).
Relative growth was then calculated using the following formula:
Where Ai is the absorbance of phosphate-free medium or medium containing minimal phytate, and Ao is the initial absorbance.
2.3.2.2 β-glucosidase activity
Previously activated yeasts were centrifuged at 11,000 g for 15 min and washed twice with saline solution (0.9% NaCl). Subsequently, cells were incubated in an inductor medium containing (g l-1): YNB 7, (NH4)2SO4 5, yeast extract 5, peptone 5, and glucose 5, pH was adjusted 5.0. Media were incubated for 72 h at 25 ± 1°C, and at 85 g for β-glucosidase production. Then, samples were centrifuged again at 11,000 g for 10 min, and the supernatant was separated from the pellet. The pellet was washed twice with sterile distilled water and placed at 105°C to obtain the dry weight of the yeast biomass in 1ml. In order to determine the location of enzymatic activity, cell- free supernatant (extracellular) (1) and pellet resuspended in citrate-phosphate buffer pH 5.0 (periplasmic) (2) were examined. The reaction system consisted of 300 µl of solution 1) or 2), mixed with 300 µl of p-nitrophenyl-β-D-glucoside (pNPG) substrate (0.75 g l-1 in 100 mM citrate-phosphate buffer, at pH 5). The mixture was incubated for 1 h at 30 ± 1°C. The reaction was stopped by adding 1,500 µl of 1M Na2CO3, and was determined at 400 nm. One unit (U) of β-glucosidase was defined as the amount of enzyme that released 1 μmol of p-nitrophenol per minute under the given assay conditions (Maturano, 2011). Nitrophenol was used to perform the calibration curve.
Spectrophotometer readings were obtained using a Thermo Fisher Scientific Multiskan™ FC Microplate Photometer.
2.4 Antioxidant activity
2.4.1 Hydrogen peroxide resistance (oxidative stress tolerance)
The Hydrogen Peroxide Resistance was examined using the semiquantitative protocol of Mestre Furlani et al. (2017). Each yeast previously activated (1x106 cells ml-1) was suspended in molten YEPD-agar to develop as a lawn. To assess tolerance, four wells (5mm diameter) were punched on each plate, and 70 µl of H2O2 solution at different concentrations (0.2 mM, 0.4 mM, 0.8 mM, and 1.4 mM) were added to the wells. Plates were then incubated at 25 ± 1°C for 48 h. If inhibition halos were formed around the wells, this indicated that the yeast strain was unable to tolerate the oxidative stress conditions induced by hydrogen peroxide concentration.
2.4.2 DPPH radical scavenging assay
A reduction in DPPH was examined using the protocol of Gil-Rodríguez et al. (2015). Previously activated yeasts at 1x106 cell ml-1 were centrifuged at 10,200 g for 5 min. Subsequently, yeasts were washed twice with saline solution (0.9% NaCl), and the pellet was resuspended in 1 ml of saline solution.
Next, 800 µl of the yeast suspension was transferred to another tube containing 1 ml of DPPH solution (0.2 mmol l-1 in methanol). The mixture was vortexed for 30 s and then incubated for 30 min at room temperature in the dark. After the incubation period, the mixture was centrifuged, and the supernatant was transferred to a 96-well microplate for absorbance measurement at 517 nm. The reduction in DPPH was calculated using the following formula:
Where: Ai is the absorbance of the sample and Ao is the absorbance of the blank.
The results were categorized into 5 groups according to Gil-Rodríguez et al. (2015): 1- values below 20% (very low activity), 2- values between 20 and 30% (low activity), 3- values between 30 and 40% (moderate activity), 4- values between 40 and 50% (high activity), 5- values above 50% (very high activity).
2.4.3 Hydroxyl radical scavenging activity
The hydroxyl radical scavenging activity was determined using the method described by Ragavan and Das (2020) with minimal modifications. One ml of yeast suspension (1x106 cells ml-1) was mixed with 1 ml of 0.435 mM brilliant green solution. Subsequently, 2 ml of 0.5Mm FeSO4 solution and 1.5 ml of a 3.0% (w v-1) H2O2 solution were added. The resulting mixture was incubated at room temperature for 15 min to allow the Fenton reaction to occur.
After the incubation period, the absorbance of the solution was measured at 624 nm. The absorbance is indicative of the hydroxyl radical scavenging activity of the yeast.
Where A is the absorbance after the Fenton reaction, Ao is the absorbance of the saline solution and As in the absorbance of the initial sample.
Spectrophotometer readings were obtained using a Thermo Fisher Scientific Multiskan™ FC Microplate Photometer.
2.5 Antagonistic action
2.5.1 Antimicrobial activity
This activity was assessed by the double-layer agar technique according to Perricone et al. (2014). Selected yeasts (1x106 cells ml-1) were distributed onto the surface of YEPD- agar, and plates were incubated at 30 ± 1°C for 24 h. Afterward, a second soft layer of CN agar or YEPD agar (0.7% agar) was distributed onto the surface of YEPD-agar plates inoculated with pathogens (6-7 log cells ml-1). Plates were incubated at 37 ± 1°C for 24 h (Perricone et al., 2014). The presence of an inhibition zone showed antimicrobial activity of yeasts towards pathogens.
For this assay, the following pathogenic microorganisms were used: Klebsiella pneumoniae ATCC 700603, Escherichia coli ATCC 25922, E. coli ATCC 35218, Pseudomonas aeruginosa ATCC 25853, Staphylococcus aureus ATCC 25923, Enterococcus faecalis ATCC 29212, and Candida albicans ATCC 10231 (American Type Culture Collection, ATCC, Rockville, MD, USA).
2.5.2 Siderophore production
Siderophore production was determined according to Nally et al. (2015). Plates were poured with CAS-HDTMA-PIPES-YNB-Glucose-Agar medium (g ml-1: CAS 0.0605, HDTMA 0.0729, PIPES 30.24, 1 mM FECl3.6H2O in 10 mM HCl, YNB 6.7, glucose 20, agar 20, pH 5). Subsequently, yeasts were spot inoculated onto the plates, which were then incubated at 25 ± 1°C in the dark for 120 h. Presence of siderophores changes the color of the medium to orange.
2.5.3 Organic acid and ethanol production
The yeasts were previously activated to reach a concentration of 1x106 cells ml-1. In order to deproteinize the samples, 1ml of the yeast sample was centrifuged at 6,800 g for 10 min. Then 250 µl of 12% trichloroacetic acid (TCA) was added to 750 µl of the supernatant, and the solution was homogenized using a vortex mixer. The samples were kept at 4 ± 1°C for 15 min and then centrifuged at 8,500 g for 10 min. Finally, the supernatants were diluted 1:3 to obtain a final TCA concentration of 4%. The final pH should be between 4 and 6. The samples were stored at -20°C until analysis (Andrade et al., 2017).
Organic acids were determined using a Shimadzu chromatograph equipped with a refractive index detector (RID-10A) and a Rezex ROA-Organic Acid H+ column (300 x 7.8mm), and operated at 42°C. The flow rate was 0.6 ml min-1 and the injection volume was 20 µl. Standards of lactic acid, formic acid, acetic acid, propionic acid, butyric acid, and ethanol were used under the same conditions for compound identification and quantification.
2.5.4 Killer toxin production
To determine if yeasts showed killer activity, the protocol according to Santos et al. (2009) was used with minimal modifications. A YEPD-Agar medium, supplemented with 3% methylene blue was used: 0.3% Yeast extract, 0.3% Malt extract, 0.5% Peptone, 1% Dextrose, 3% NaCl, 10% (v v-1) Glycerol, 2% Agar, and 0.003% (v v-1) Methylene blue in 0.2 M citrate phosphate buffer). The medium was tested at two different pH values: 4.5 and 6. Potentially pathogenic bacteria and yeast were seeded at a concentration of 1x106 cells ml-1 onto the medium to develop as a lawn. The probiotic yeasts were spot inoculated, and plates were incubated at 37 ± 1°C for 72 h. Killer toxin production was detected as clear inhibition zones around the colonies.
2.5.5 Catalase activity
For the qualitative determination of catalase activity, the protocol of Mestre Furlani et al. (2017) was used. Previously activated yeasts were inoculated on YEPD-Agar plates and incubated at 28 ± 1°C for 48 h. Once grown, a few drops of 3% hydrogen peroxide were placed on the yeast. Catalase activity was evidenced by the formation of bubbles on the colony. Criteria by Perricone et al. (2014) with minimal modifications were used to interpret the results: “0” (no activity), “1” (weak activity), “2” (good activity), and “3” (quick activity).
2.6 Ability to reduce cholesterol
The capacity to reduce cholesterol was determined using the method by Alakeji et al. (2015) with modifications. Previously activated yeasts were inoculated at a concentration of 1x106 cells ml-1 for 48 h at 37 ± 1°C in YPGCHO medium containing (g l-1): peptone 20, glucose 20, yeast extract 10, bile 3, and cholesterol 50 mg l-1. Subsequently, 5 ml of the medium were centrifuged (6800 g, 15 min) and the cholesterol content of the supernatant was measured with a cholesterol test kit (enzymatic AA liquid Colestat method, Wiener Lab).
Cholesterol reduction was calculated using the following formula:
Where: Ai = cholesterol content in culture broth and Ao = cholesterol content in non-inoculated broth.
2.7 Data analysis
All assays were performed independently in triplicate. Results are presented as means ± standard deviations of the three independent determinations. Normality was tested using InfoStat software (version 2020). To assess normality and homoscedasticity, residuals and absolute residuals were evaluated using the Shapiro-Wilk test and an analysis of variance of absolute residuals, respectively. For normally distributed data, significant differences were determined using the Tukey test. For non-normally distributed data, the Kruskal-Wallis test was used to determine significant differences. Statistical significance was set at p< 0.05. Graphs were generated using GraphPad Prism software (version 8).
3 Results
3.1 Evaluation of pathogenicity using an in vivo model with Galleria mellonella larvae
A trial was carried out with Galleria mellonella larvae. The insect showed a survival rate of 100% in 13 of the 14 isolates used in this work, with the exception of Pichia kudriavzevii (PB100), which showed a survival rate of 94.4%; 19 of the 20 larvae inoculated with the yeast survived. Figure 1 shows the three different assay conditions of the larvae: positive control (A), negative control (B) and larvae inoculated with W. anomalus PB97 (C). D shows the container of the larvae of the PB97 group on the Petri dish. All larvae of the negative control group (inoculated with sterile PBS) survived, while all the larvae inoculated with the pathogenic microorganism (Pseudomonas aeruginosa ATCC 25853), the positive control group, did not survive in the first 24h.
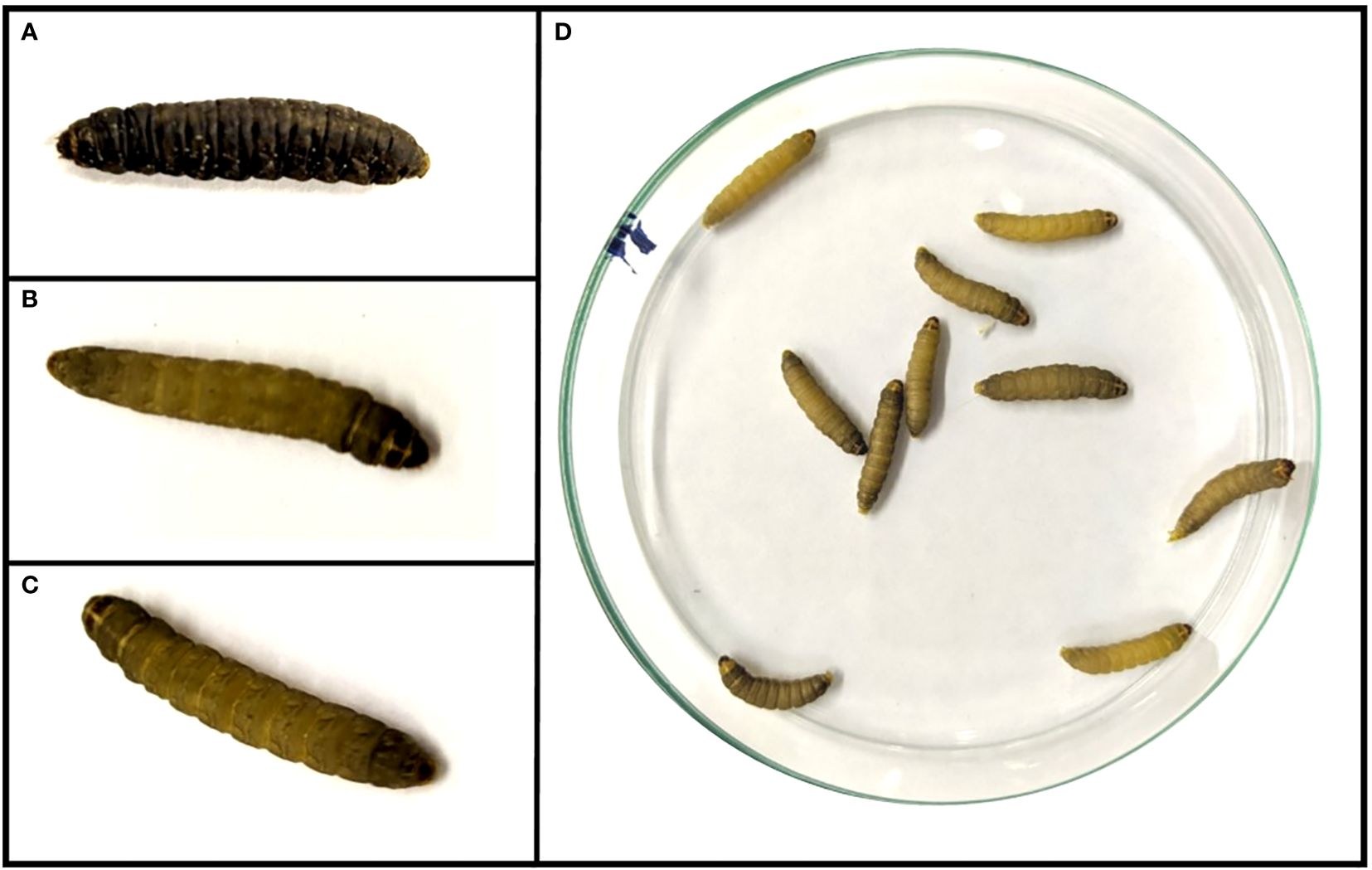
Figure 1. Pathogenicity assay using Galleria mellonella larvae. The left box shows larvae from different groups can be observed at 100X magnification: (A) Larva belonging to the positive control, in death phase, inoculated with Pseudomonas aeruginosa ATCC 25853, (B) Larva belonging to the negative control, inoculated with sterile saline solution (0.9% NaCl), and (C) Larva belonging to the PB97 study group. The right box shows a group of larvae belonging to only one group at 40X stereoscope magnification: (D) Plate with 10 larvae belonging to the PB97 study group.
3.2 Enzyme activities detection
Figure 2 displays qualitative enzyme activities in the studied yeasts. It can be observed that all yeast strains were able to grow in minimal medium supplemented with sodium phytate compared with growth in phosphate-supplemented medium. Additionally, all 14 yeasts demonstrated the ability to degrade tributyrin, indicating the presence of lipolytic enzymes, as evidenced by the formation of a translucent halo. H. guilliermondii (PB15), P. kudriavzevii (PB50), P. manshurica (PB54), and W. anomalus (PB97, PB98) exhibited protease activity in milk-supplemented medium, with a halos similar to that presented by the control yeast S. boullardii. Furthermore, W. anomalus (PB97, PB98, PB99), H. guillermondii (PB15), and P. kudriadzevii (PB100) showed β-glucosidase enzymatic activity on plates. None of the yeasts exhibited β-galactosidase, esterase, or amylase activities.
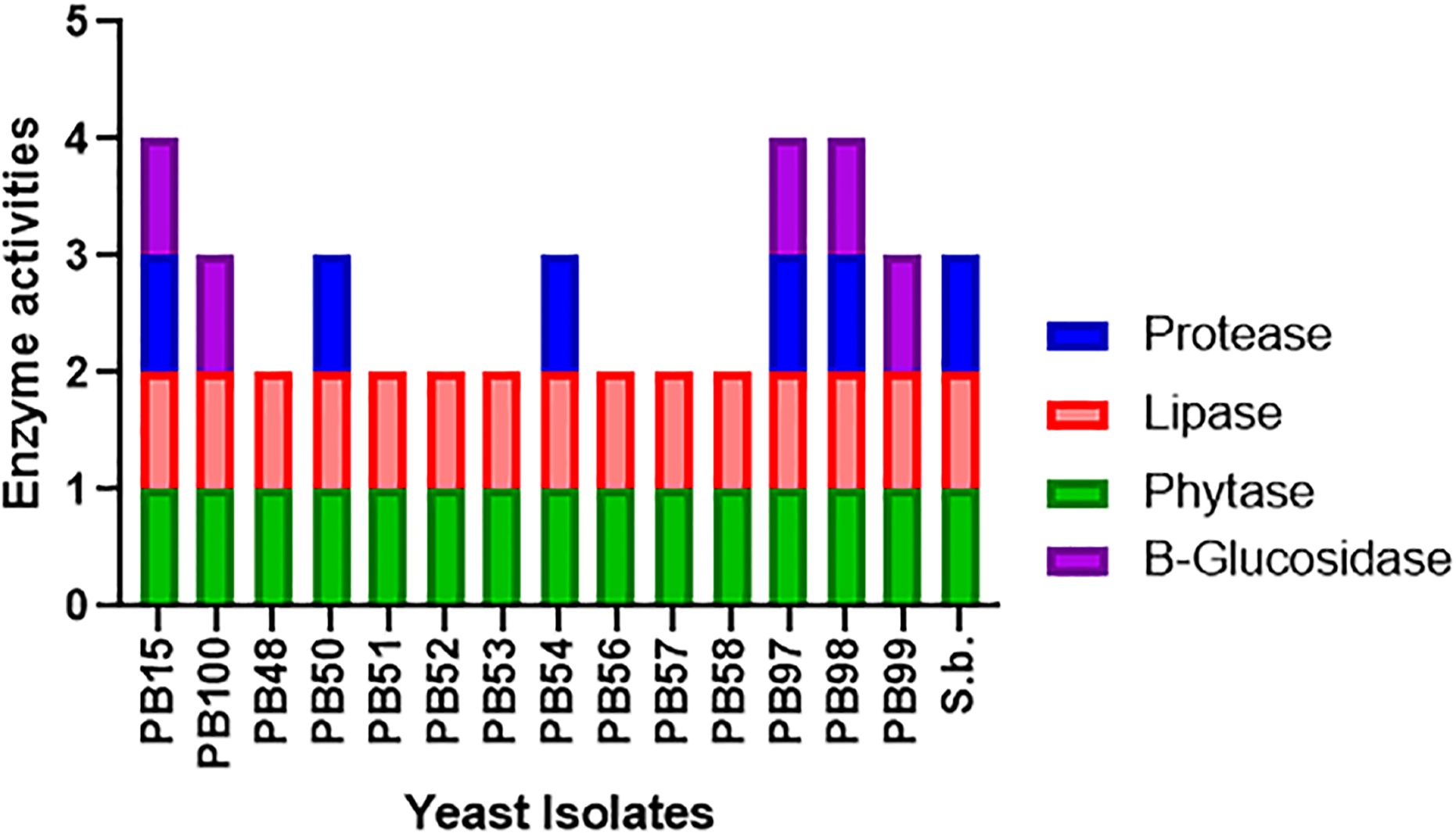
Figure 2. Detection of extracellular enzyme production by yeast strains. The figure shows the qualitative enzyme activities presented by each yeast. A value of 1 was assigned if the activity was present, and a value of 0 if it was absent. References: blue color represents protease activity, red color represents lipase activity, green color represents phytase activity and the purple color represents β-glucosidase activity.
Due to the importance of phytase activity and considering that most of the studied yeasts showed significant growth in the qualitative assay, quantification of this activity was carried out (Figure 3). The three W. anomalus strains, PB97, PB98, and PB99, exhibited highest phytase activity: 76.3, 77.16, and 73.91%, respectively. Additionally, two Pichia yeasts, P. kudriadzevii (PB48) and P. occidentalis (PB56), showed statistically similar values to those exhibited by the Wickerhamomyces yeasts, with 70.77% and 73.54%, respectively. It is important to mention that most yeasts recorded activity similar to or higher than that of the reference probiotic strain, S. boulardii, except for H. guillermondii PB15 (48.9%) and P. manshurica PB54 (52.67%) (Figure 3).
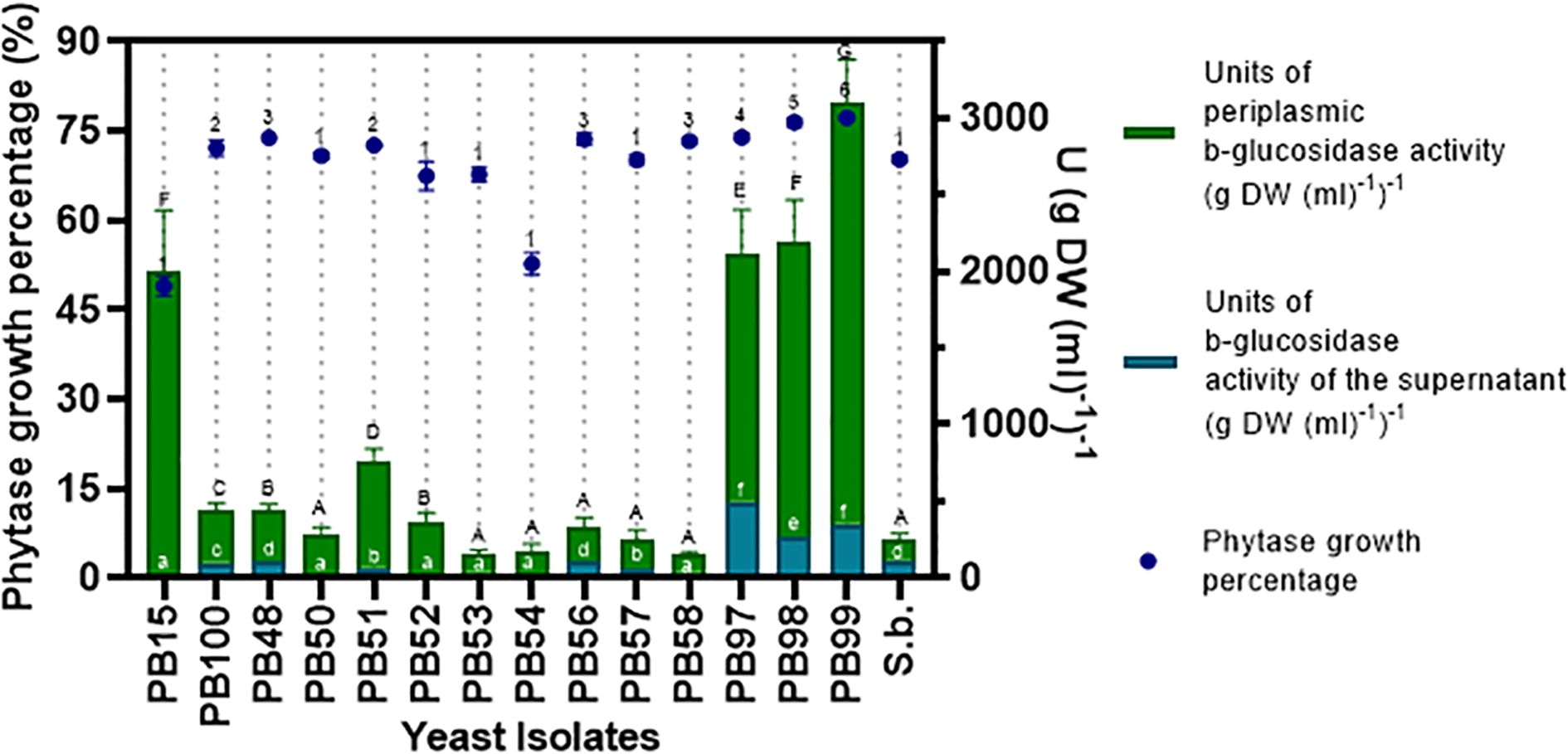
Figure 3. Quantitative enzymatic activity of phytase and β-glucosidase. The left axis shows the mean percentage growth value of phytase enzyme activity, plotted with dots. The right axis shows the concentration of β-glucosidase enzyme activity, evaluated in the supernatant and periplasm, plotted with stacked bars. Statistical similarities are indicated with numbers for the evaluation of phytase activity growth percentage, uppercase letters for similarities in periplasmic β-glucosidase activity, and lowercase letters for β-glucosidase activity in the supernatant. Identical symbols indicate no statistical differences.
In the quantitative β-glucosidase activity assay, the W. anomalus yeasts (PB97, PB98, PB99) showed the highest activity in the supernatant fraction: 1,592.51, 1,901.67, and 2,722.58 Units g-1, respectively. The reference yeast S. boulardii followed with a value of 138.10 Units g-1. Additionally, these same three W. anomalus yeasts demonstrated the highest enzyme production in this study, in the periplasmic space, with values of 483.57, 265.16, and 347.83 Units g-1, respectively.
3.3 Antioxidant activity
All yeasts showed tolerance to different concentrations of hydrogen peroxide assayed, which means that the strains would be able to grow under conditions when peroxide is present.
The DPPH radical scavenging activity was also evaluated, and the results are shown in Figure 4. All yeasts showed antioxidant activity, and PB56, PB97, PB100, PB57, and PB58 were most prominent as their values were higher than the control, S. boulardii.
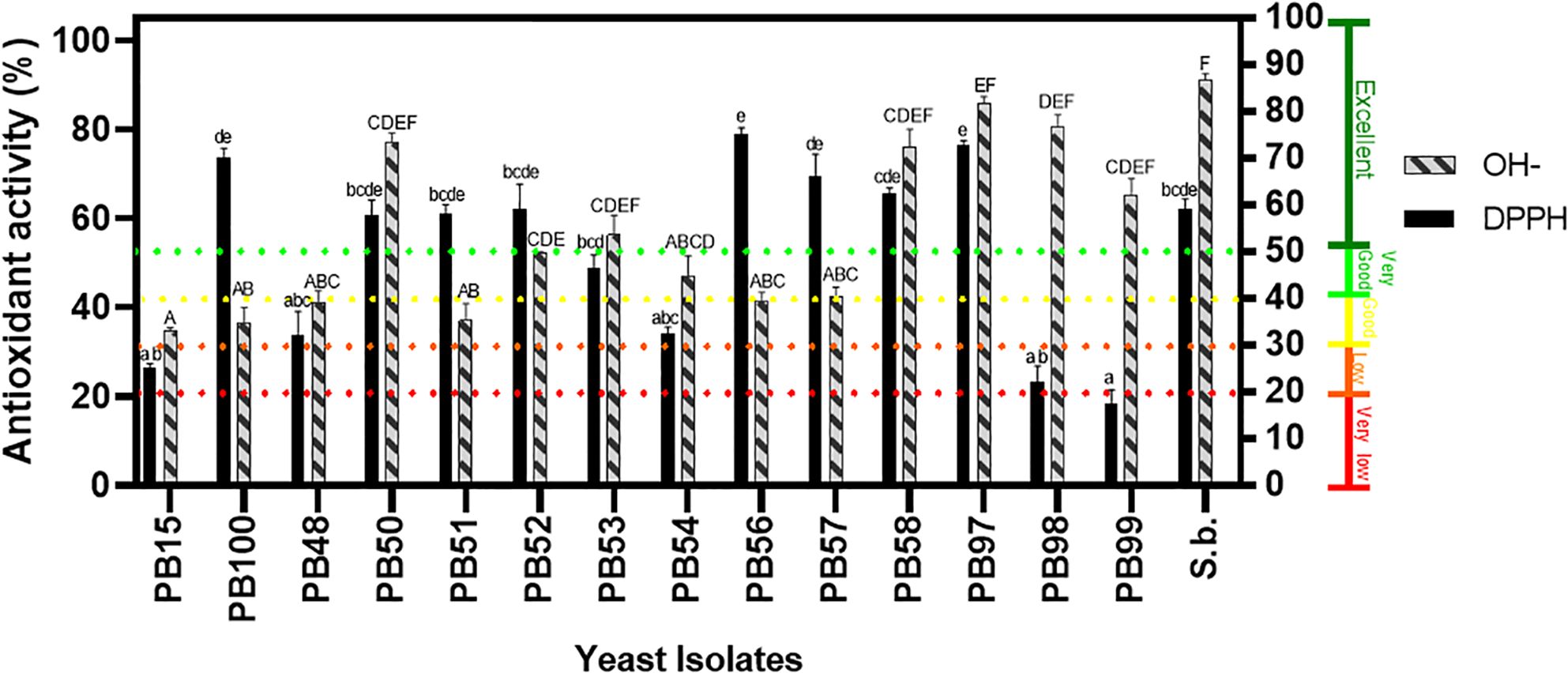
Figure 4. Screening for antioxidant activity production by yeast isolates. The solid black color represents DPPH radical scavenging activity. The bars with textured patterns indicate hydroxyl radical activity. Isolates marked with letters are statistically similar, with a probability greater than 0.05. Lowercase letters denote bars that are statistically similar in DPPH activity, while uppercase letters indicate similarity in hydroxyl radical scavenging activity. The left axis represents the percentage, while the right axis shows a subclassification into 5 groups based in the percentage value, according to Gil-Rodríguez et al. (2015): <20% very low activity, 20-30% low activity, 30-40% good activity, 40-50% very good activity, and above 50% excellent antioxidant activity.
Additionally, hydroxyl radical scavenging activity was determined in all yeast strains. Figure 4 shows that PB97 (85.69%), PB98 (79.99%), PB58 (77.73%), PB50 (77.14%) and PB99 (65.44%) presented the highest values.
3.4 Antagonistic effect
The qualitative antimicrobial assay according to Perricone et al. (2014) revealed that yeast strains PB54, PB97, PB98 and PB99 inhibited growth of at least one pathogenic microorganism (Figure 5). However, possible mechanisms of action were evaluated in all the strains studied (Figure 5). Only one strain, P. manshurika (PB54), exhibited siderophore production, represented by a strong orange color. This killer activity assay was performed at optimum pH (4.5), where activity of yeast PB54 against pathogenic C. albicans was found. However, at pH values close to that of the intestine (pH 6 and 7), no yeast with killer activity was observed.
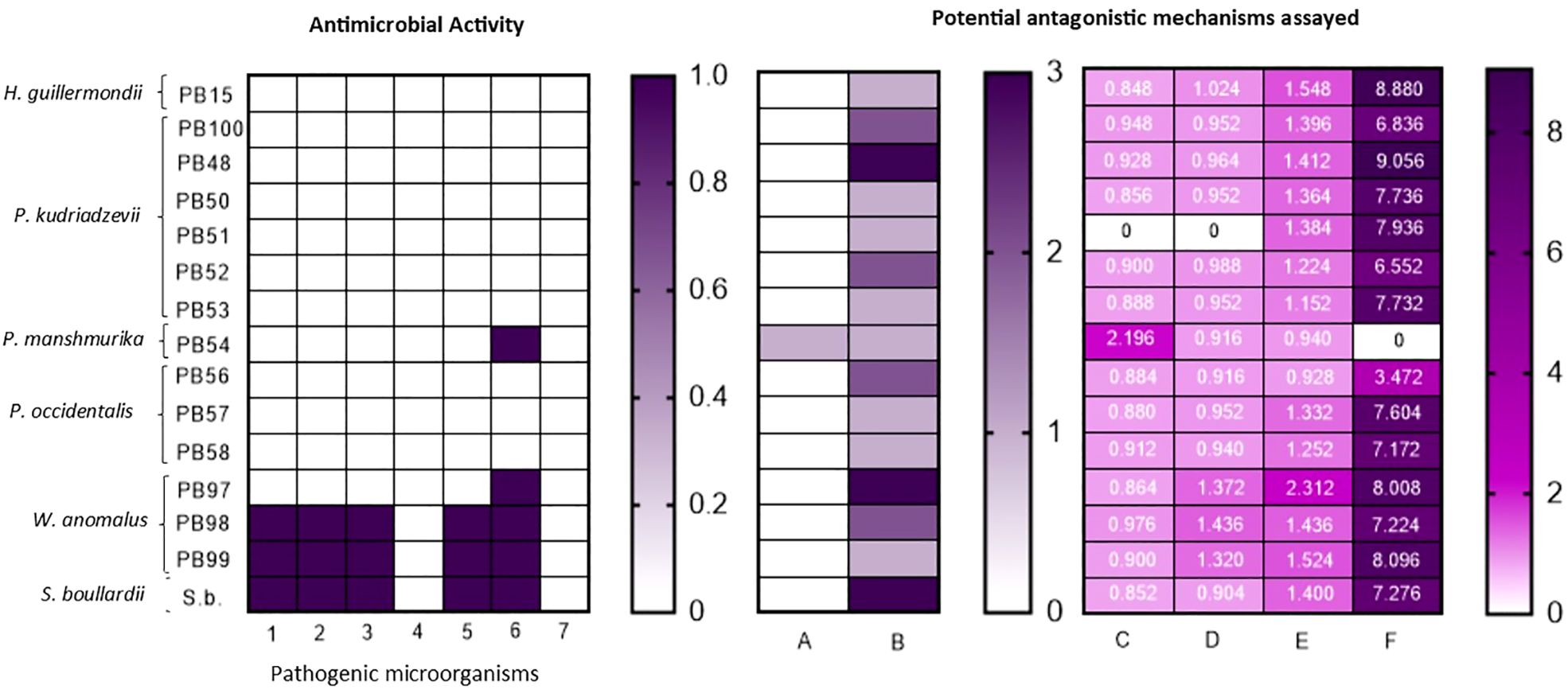
Figure 5. Antimicrobial activity and tested mechanisms. From left to right: (A) First Graph-First double-layer antimicrobial activity assay according to Perricone et al. (2014). On the vertical axis the yeasts assayed are visualized, while on the horizontal axis with numbers the pathogenic microorganisms used are differentiated. (B) Second graph- Scheme of qualitatively assayed antagonistic activities, on the vertical axis the yeasts used are differentiated and on the horizontal axis differentiated with letters the qualitative assays performed. (C) Third graph- Quantitative antagonistic activity assayed, the vertical axis shows the different yeasts and the horizontal axis shows the different quantitative assays performed. In each box it can see the value obtained in g l-1. References: (1) Klebsiella pneumoniae ATCC 700603, (2) Escherichia coli ATCC 25992, (3) E. coli ATCC 35218, (4) Pseudomonas aeruginosa ATCC 25853, (5) Staphylococcus aureus ATCC 25923, (6) Enterococcus faecalis ATCC 29212, (7) Candida albicans ATCC 10231, (A) Siderophore production, (B) catalase activity, (C) lactic acid production, (D) acetic acid production, (E) propionic acid production, (F) ethanol production.
Catalase activity was assayed, and strains were classified according to their response: yeasts with a very fast response scored a 3, those that demonstrated fast activity scored a 2 and if their activity was not so fast, they were given a value of 1 (Figure 5).
Thirteen yeast presented lactic acid production similar to that of the reference yeast S. boullardii, with the exception of P. mansmurika PB54, which twice the amount (2.196 g l-1) produced by the reference strain (Figure 5). With respect to acetic acid, 13 yeasts showed acetic acid production, with values close to those of the reference yeast (Figure 5). The genus Wickerhamomyces produced the most acetic acid, with values around 1.320 -1.436 g l-1. W. anomalus PB97 had a higher propionic acid production than the control probiotic yeast (2.312 vs 1.400 g l-1). The remaining yeasts showed considerable production close to those of the control yeast, with the exception of 3 Pichia strains (PB53, PB54, PB56), which were lower (Figure 5). For more details, please refer to the Supplementary Material which includes chromatograms and calibration curves.
3.5 Ability to reduce cholesterol
All yeast cells were able to reduce the concentration of cholesterol in the medium, showing a reduction that ranged from 0.38 to 47.50%, shown in Table 1. Highest reduction was observed with P. kudriavzevii PB48 (47.5% ± 12.99), which was statistically similar to P. kudriavzevii (PB50), with a reduction of 44.42% ± 7.75 and W. anomalus (PB98) with a value of 38.35% ± 3.24.
4 Discussion
The selected yeasts in this study were evaluated following the Argentine regulatory guidelines for microorganisms intended for human consumption, as established in the Argentine Food Code (Resolution 261/2011 and 22/201). This approach, aligned with FAO and WHO guidelines, ensures compliance with national safety and efficacy standards for potential probiotic applications. At a global level, yeast species such as Saccharomyces cerevisiae, Saccharomyces boulardii, and Kluyveromyces marxianus are commercially available, highlighting their widespread acceptance and utilization in various health-related applications.
In recent years, there has been an increasing number of studies using larvae as an invertebrate model to evaluate the toxicity of potential probiotic bacterial strain (Wang et al., 2020) and to a lesser extent in yeast (Peréz-Través et al., 2021). in vivo The initial yeast concentration used in this study was 1x106 cells ml-1, as some reports had indicated that this would be the minimum concentration for probiotic to exert their function (Staniszewski and Kordowska-Wiater, 2021; Lai et al., 2024). At this concentration, Tran et al. (2023) reported a 93.33% survival rate for larvae exposed to Pichia kudriavzevii, indicating its biosafety. Our results, with 100% survival for W. anomalus, align with the findings of Peréz-Través et al. (2021) under similar conditions. While biosafety data are limited for H. guillermondii, P. manshurica, and P. occidentalis, the high survival rate (100%) observed in this study for all 14 yeast strains, supported by previous research (Vergara et al., 2023b), suggests their biosafety at 106 cells ml-1.
The reported effects of probiotic yeasts in the human gastrointestinal tract are diverse, and many of them complement and/or enhance one another, producing multiple benefits. One notable effect is the production of enzymes that are mainly involved in the assimilation and digestion of foods. In accordance with the results of this study, several studies report protease production by non-conventional yeasts, often assessed qualitatively (Esteve-Zarzoso et al., 1998; Ogunremi et al., 2015a; Mestre Furlani et al., 2017). In line with the findings, protease activity has been reported for P. kudriavzevii, W. anomalus, and H. uvarum (Ogunremi et al., 2015a; Merchán et al., 2022; Elhalis et al., 2021). protease activity suggests these yeasts may contribute to protein breakdown, yielding smaller bioactive peptides with potential antihypertensive, immunomodulatory, opioid, antimicrobial, and antioxidant properties (Esteve-Zarzoso et al., 1998). On the other hand, pancreatic lipase is crucial for triglyceride digestion. This activity has been observed in various non-conventional yeasts, including Pichia and Hanseniaspora species, under assay conditions similar to those employed in the study (Maturano et al., 2009; Ogunremi et al., 2015a). Furthermore, Parafati et al. (2022), using a different methodology, reported lipase activity in P. kluyveri and W. anomalus strains. The presence of lipase activity in the yeasts studied infers a potential contribution to triglyceride digestion, a finding consistent with previous observations (Espejo Venegas, 2022). This study demonstrated phytase production by P. kudriavzevii, P. occidentalis, and W. anomalus, exceeding the reference strain’s activity by 60%. Similarly, Ogunremi et al. (2015a) and Parafati et al. (2022) reported significantly increased phytase activity (over 95%) in Pichia species compared to positive controls. The study’s findings show that the presence of phytase in the yeast strains is noteworthy because this specific phosphatase enhances mineral bioavailability and exhibits anticancer properties (Vucenik and Shamsuddin, 2006). This study reveals β-glucosidase production in all strains analyzed, with particularly high activity in W. anomalus (PB97, PB98 and PB99), indicating its ability to catalyze the hydrolysis of β-glucans found in various cereals (Seng, 2014). These findings align with previous studies reporting high β-glucosidase activity in H. guilliermondii and W. anomalus, particularly in the periplasmic space (Maturano et al., 2009; Huang et al., 2021). This study quantitatively assessed phytase and β-glucosidase activity, given their previously mentioned importance. A quantitative analysis of all enzymatic activities, both extracellular and intracellular, is considered crucial. Based on the findings of Parafati et al. (2022), some enzymatic activities that were negative extracellularly (such as amylase and β-galactosidase) might show different results when examined intracellularly. A crucial characteristic of probiotic yeasts is their antioxidant capacity or their contribution to the bioavailability of antioxidant compounds (Vergara et al., 2023a). In the first assay, yeast survival was assessed after exposure to hydrogen peroxide, an oxidative stress agent produced during oxidative metabolism (Tran et al., 2023). The results showed favorable tolerance in all strains, consistent with previous studies (Ragavan and Das, 2020). In the DPPH radical scavenging assay, the yeasts showed positive results, with values comparable to the reference probiotic strain W. anomalus and P. kudriavzevii stood out, in line with other studies (Gil-Rodríguez et al., 2015; Ciafardini and Zullo, 2020; Mogmenga et al., 2023), although some studies report scavenging activity below 35% for P. kudriavzevii (Menezes et al., 2020). In the hydroxyl radical scavenging assay, six yeasts showed a reduction greater than 50%, highlighting W. anomalus, P. kudriavzevii, and P. occidentalis (PB58). These results are similar to those reported by Fakruddin et al. (2017) and Ragavan and Das (2020) in Saccharomyces and other non-conventional yeasts. Overall, P. kudriavzevii (PB50), P. occidentalis (PB58), and W. anomalus (PB97) showed good antioxidant activity in all three assays, comparable to the reference probiotic strain S. boullardii CNCM I-745 (D’Antongiovanni et al., 2023), which is crucial considering the role of probiotics in reducing cell damage and their potential function as antimicrobial agents (Monika et al., 2021). Although three assays were used to evaluate antioxidant activity, additional evaluation strategies could be employed to complement the results and infer the mechanisms of action of the yeasts. The ability of probiotics to inhibit the growth of other species largely depends on their antimicrobial properties (Monika et al., 2021). Iron competition was assessed through siderophore activity. In our study, Pichia manshurica PB54 produced this metabolite, as did species of the same genus (P. membranifasciens, P. kudriavzevii, W. anomalus) reported by other authors (Nally et al., 2015; Prabina et al., 2019; Srinivasan et al., 2022). This iron competition is considered beneficial, although it has been suggested that it may actually be a prerequisite for pathogenicity (Ismail et al., 1985). Killer toxins are among the most important antimicrobial compounds produced by yeasts. However, while killer toxin production has been reported in non-conventional yeasts under different pH and temperature conditions (Belda et al., 2017; Hodgson et al., 1995; Muccilli et al., 2013; Gil-Rodríguez and Garcia-Gutierrez, 2021; Kuchen et al., 2021; Santos et al., 2009), this study did not detect such activity in the tested yeasts under simulated gastrointestinal conditions. Anothers metabolite produced by yeasts that can influence microbial activity is short-chain fatty acids. There were three acids detected in the yeasts of this study (acetic acid, lactic acid, and propionic acid). Considering that acetic acid has a stronger antagonistic effect (Monika et al., 2021), strains of W. anomalus (PB97, PB98 and PB99) produced the highest levels of this compound. The yeasts evaluated recorded lower productions of lactic acid and acetic acid than those produced by bacteria (Neal-McKinney et al., 2012; Tejero-Sariñena et al., 2012; Hauka et al., 2014). The production of propionic acid by yeasts is higher than that produced by lactic acid bacteria (Hauka et al., 2014). While one of the acids stands out for its antimicrobial activity, it is considered that they may act due to a synergy between them (Danial et al., 2021). The production of propionic, acetic, and lactic acids by all the yeasts in this study could also have neuroactive properties, as well as influence the restoration of intestinal permeability (Van de Wouw et al., 2018). Unlike studies on non-conventional yeasts, previous research on short-chain fatty acid production has primarily focused on S. boulardii (Ling et al., 2023; Di Martino et al., 2023), therefore, the findings of this study are particularly significant. Ethanol, an important metabolite produced by yeasts, is known for its antimicrobial properties. The non-conventional yeasts evaluated in this study produced a concentration of ethanol similar to that produced by the reference strain S. boulardii CNCM I-745. Although ethanol-induced membrane fluidification can negatively affect host cells (Lee et al., 2015; Manzo-Avalos and Saavedra-Molina, 2010), it has been shown that low concentrations of alcohol produced by yeasts inhibit the growth of Escherichia coli (Etienne-Mesmin et al., 2011). This may occur because the fermentable sugars used by alcohol-producing microorganisms are no longer available to pathogenic microorganisms (Bongaerts and Severijnen, 2001). The ability of an organism to produce catalase, an antioxidant enzyme, gives it a competitive advantage (Zhao and Drlica, 2014; Jia et al., 2015). This study found that all yeasts produced catalase and tolerated hydrogen peroxide concentrations ranging from 0.2 to 1.4 mM. Comparable catalase activity was reported in ten non-conventional yeasts (genera Pichia, Hanseniaspora, among others.) by Fernández-Pacheco et al. (2021), and also in a W. anomalus strain by Zhao and Drlica (2014).The presence of this enzyme in the yeasts studied not only gives them a defense mechanism (França et al., 2007; Herrero et al., 2008), but it could also be effective in alleviating intestinal inflammation (Tomusiak-Plebanek et al., 2018). From our findings, it can be deduced that antimicrobial activity may be the result of a combination of several mechanisms, some of which have been addressed in the present study. Although not all yeasts in our study showed antimicrobial activity, it cannot be ruled out that they possess this property, as only 7 pathogens were tested. The link between cholesterol levels, coronary heart disease risk, and colon cancer has garnered increasing attention in recent years (Psomas et al., 2003). While several mechanisms by which probiotic strains might reduce cholesterol have been proposed (e.g., assimilation, cell surface binding, cell membrane incorporation, bile acid deconjugation, cholesterol coprecipitation), yeast primarily reduces cholesterol through assimilation by growing cells (Psomas et al., 2003). This study demonstrates cholesterol-reducing capacity in all yeast strains tested. Consistent with our findings, cholesterol reductions of 44.3% and 60% have been reported for P. kudriavzevii (Chen et al., 2010; Ogunremi et al., 2015b). Given that a 1% reduction in cholesterol is associated with a 2-3% decrease in cardiovascular disease risk (Manson et al., 1992), the cholesterol-lowering effect observed in all our strains is considered significant.
5 Conclusion
All 14 non-conventional yeast isolates from the viticultural environment tested in this study were found to be non-pathogenic, indicating their potential safety for applications such as probiotics. The innovative use of Galleria mellonella to assess toxicity highlights the need for further investigation to determine whether non-toxicity is strain- or species-dependent. Future studies should investigate the strain and species dependence of toxicity, and define dose-response relationships to determine the threshold for adverse effects. Additionally, the study emphasized the production of hydrolytic enzymes and antioxidant activity by certain yeast strains, as well as their antagonistic activity against pathogens, which could be linked to mechanisms like catalase activity, organic acid production, and ethanol synthesis. Although the cholesterol reduction observed was modest compared to other studies, some strains, particularly P. kudriavzevii and W. anomalus, showed notable potential. These findings lay a strong foundation for the future selection of probiotic yeasts for various applications, from health-related uses to the food industry, with plans to continue in vivo trials to evaluate their probiotic efficacy.
Data availability statement
The raw data supporting the conclusions of this article will be made available by the authors, without undue reservation.
Ethics statement
The animal study was approved by COBIOET Comité de Bioética de la FCEFN - UNSJ. The study was conducted in accordance with the local legislation and institutional requirements.
Author contributions
ML: Conceptualization, Investigation, Methodology, Software, Validation, Writing – original draft. SV: Conceptualization, Formal Analysis, Investigation, Writing – original draft. MM: Conceptualization, Software, Writing – review & editing. FV: Conceptualization, Supervision, Writing – review & editing. PM: Conceptualization, Writing – review & editing. YM: Conceptualization, Funding acquisition, Investigation, Resources, Supervision, Writing – review & editing.
Funding
The author(s) declare that financial support was received for the research and/or publication of this article. This work was supported by the following projects: PICT 2019 -02866 and PIP -11220200101257CO.
Acknowledgments
We are especially grateful to the institutions to which we belong to: Universidad Nacional de San Juan (UNSJ) and Consejo Nacional de Investigaciones Científicas y Técnicas (CONICET). The collaboration of Dr. Cristina Nally (director of Biotechnology Institute- UNSJ) is appreciated for facilitating the management and transportation of Galleria mellonella larvae. Furthermore, we are grateful to the Decentralized Hospital Doctor Guillermo Rawson for providing the reference pathogenic microorganisms (American Type Culture Collection -ATCC) used in the study. Their collaboration has been essential in advancing the research. To enhance readability and language clarity, the authors utilized Google's Gemini AI writing tool during the preparation of this work. Following, the authors thoroughly reviewed and edited the content as necessary. The authors assume full responsibility for the final content of the publication.
Conflict of interest
The authors declare that the research was conducted in the absence of any commercial or financial relationships that could be construed as a potential conflict of interest.
Publisher’s note
All claims expressed in this article are solely those of the authors and do not necessarily represent those of their affiliated organizations, or those of the publisher, the editors and the reviewers. Any product that may be evaluated in this article, or claim that may be made by its manufacturer, is not guaranteed or endorsed by the publisher.
Supplementary material
The Supplementary Material for this article can be found online at: https://www.frontiersin.org/articles/10.3389/finmi.2025.1494334/full#supplementary-material
References
Alakeji T. P., Banwo K., Ogunremi O. R., Sanni A. I. (2015). Functional properties of yeasts isolated from some Nigerian traditional fermented foods. J. Microbiol. Biotech. Food Sci. 4, 437. doi: 10.15414/jmbfs.2015.4.5.437-441
Andrade R. P., Melo C. N., Genisheva Z., Schwan R. F., Duarte W. F. (2017). Yeasts from Canastra cheese production process: Isolation and evaluation of their potential for cheese whey fermentation. Food Res. Int. 91, 72–79. doi: 10.1016/j.foodres.2016.11.032
Azhar S. H. M., Abdulla R., Jambo S. A., Marbawi H., Gansau J. A., Faik A. A. M., et al. (2017). Yeasts in sustainable bioethanol production: A review. Biochem. Biophys. Rep. 10, 52–61. doi: 10.1016/j.bbrep.2017.03.003
Belda I., Ruiz J., Alonso A., Marquina D., Santos A. (2017). The biology of Pichia membranifaciens killer toxins. Toxins 9, 112. doi: 10.3390/toxins9040112
Bongaerts G. P. A., Severijnen R. S. V. M. (2001). The beneficial, antimicrobial effect of probiotics. Med. Hypotheses 56, 174–177. doi: 10.1054/mehy.2000.1135
Chen L., Ying M., Maubois J. L., Chen L. J., Liu Q. H., Guo J. P., et al. (2010). Identification of yeasts from raw milk and selection for some specific antioxidant properties. Int. l. J. Dairy Technol. 63, 47–54. doi: 10.1111/j.1471-0307.2009.00548.x
Ciafardini G., Zullo B. A. (2020). In vitro potential antioxidant activity of indigenous yeasts isolated from virgin olive oil. J. Appl. Microbiol. 128, 853–861. doi: 10.1111/jam.v128.3
D’Antongiovanni V., Antonioli L., Benvenuti L., Pellegrini C., Di Salvo C., Calvigioni M., et al. (2023). Use of Saccharomyces boulardii CNCM I-745 as therapeutic strategy for prevention of nonsteroidal anti-inflammatory drug-induced intestinal injury. Br. J. Pharmacol. 180, 3215–3233. doi: 10.1111/bph.16200
Danial A. M., Medina A., Magan N. (2021). Lactobacillus plantarum strain HT-W104-B1: potential bacterium isolated from Malaysian fermented foods for control of the dermatophyte Trichophyton rubrum. W. J. Microbiol. Biotechnol. 37, 1–11. doi: 10.1007/s11274-021-03020-7
Di Martino L., Osme A., Ghannoum M., Cominelli F. (2023). A novel probiotic combination ameliorates Crohn’s disease–like ileitis by increasing short-chain fatty acid production and modulating essential adaptive immune pathways. Inflam. Bowel Dis. 29, 1105–1117. doi: 10.1093/ibd/izac284
Elhalis H., Cox J., Frank D., Zhao J. (2021). Microbiological and biochemical performances of six yeast species as potential starter cultures for wet fermentation of coffee beans. Lwt 137, 110430. doi: 10.1016/j.lwt.2020.110430
Espejo Venegas V. H. (2022). La lipasa pancreática. Benemérita Universidad Autónoma de Puebla, Puebla, México: Colección de ESMOS, Licenciatura en Biotecnología Facultad de Ciencias Biológicas. doi: 10.5281/zenodo.7343248
Esteve-Zarzoso B., Manzanares P., Ramón D., Querol A. (1998). The role of non-Saccharomyces yeasts in industrial winemaking. Int. Microbiol. 1, 143–148.
Etienne-Mesmin L., Livrelli V., Privat M., Denis S., Cardot J. M., Alric M., et al. (2011). Effect of a new probiotic Saccharomyces cerevisiae strain on survival of Escherichia coli O157: H7 in a dynamic gastrointestinal model. Appl. Environm. Microbiol. 77, 1127–1131. doi: 10.1128/AEM.02130-10
Fakruddin M. D., Hossain M. N., Ahmed M. M. (2017). Antimicrobial and antioxidant activities of Saccharomyces cerevisiae IFST062013, a potential probiotic. BMC Complement. Alternat. Med. 17, 1–11. doi: 10.1186/s12906-017-1591-9
Fernández-Pacheco P., García-Béjar B., Jiménez-del-Castillo M., Carreño-Domínguez J., Briones Perez A., Arévalo-Villena M. (2021). Potential probiotic and food protection role of wild yeasts isolated from pistachio fruits (Pistacia vera). J. Sci. Food Agric. 101, 2201–2209. doi: 10.1002/jsfa.10839
França M. B., Panek A. D., Eleutherio E. C. A. (2007). Oxidative stress and its effects during dehydration. Comp. Biochem. Physiol. Part A: Mol. Integr. Physiol. 146 (4), 621–631. doi: 10.1016/j.cbpa.2006.02.030
Garcia-Bustos V., Ruiz-Saurí A., Ruiz-Gaitán A., Sigona-Giangreco I. A., Cabañero-Navalon M. D., Sabalza-Baztán O., et al. (2021). Characterization of the Differential Pathogenicity of Candida auris in a Galleria mellonella Infection Model. Microbiol. Spect. 9, e00013–e00021. doi: 10.1128/Spectrum.00013-21
Gil-Rodríguez A. M., Carrascosa A. V., Requena T. (2015). Yeasts in foods and beverages: In vitro characterisation of probiotic traits. LWT-Food Sci. Technol. 64, 1156–1162. doi: 10.1016/j.lwt.2015.07.042
Gil-Rodríguez A. M., Garcia-Gutierrez E. (2021). Antimicrobial mechanisms and applications of yeasts. Adv. Appl. Microbiol. 114, 37–72. doi: 10.1016/bs.aambs.2020.11.002
Gut A. M., Vasiljevic T., Yeager T., Donkor O. N. (2018). Salmonella infection–prevention and treatment by antibiotics and probiotic yeasts: a review. Microbiol 164, 1327–1344. doi: 10.1099/mic.0.000709
Hauka F. I. A., Selim A. E. I., El-Sawah M. M. A., Rashad E. M. (2014). Organic acids production and antagonistic effect of some strains of probiotics. J. Agric. Chem. Biotechnol. 5, 101–112. doi: 10.21608/jacb.2014.49886
Herrero E., Ros J., Bellí G., Cabiscol E. (2008). Redox control and oxidative stress in yeast cells. Biochim. Biophys. Acta (BBA)-General Subj. 1780 (11), 1217–1235. doi: 10.1016/j.bbagen.2007.12.004
Hill C., Guarner F., Reid G., Gibson G. R., Merenstein D. J., Pot B., et al. (2014). Expert consensus document: The International Scientific Association for Probiotics and Prebiotics consensus statement on the scope and appropriate use of the term probiotic. Nat. Rev. Gastroenterol. Hepatol. 11, 506–514.
Hodgson V., Button J. D., Walker G. M. (1995). Anti-candida activity of a novel killer toxin from the yeast Williopsis mrakii. Microbiology 141, 2003–2012. doi: 10.1099/13500872-141-8-2003
Huang R., Zhang F., Yan X., Qin Y., Jiang J., Liu Y., et al (2021). Characterization of the β‐Glucosidase activity in indigenous yeast isolated from wine regions in China. J Food Sci. 86 (6), 2327–2345. doi: 10.1111/1750-3841.15741
Ismail A., Bedell G. W., Lupan D. M. (1985). Siderophore production by the pathogenic yeast, Candida albicans. Biochem. Biophys. Res. Communic. 130, 885–891. doi: 10.1016/0006-291X(85)90499-1
Jia R. L., Liu J. B., Wei Y. S., Cai X. (2015). Effect of residual hydrogen peroxide on hydrolysis acidification of sludge pretreated by microwave-H2O2-alkaline process. Huan Jing ke Xue Huanjing Kexue 36, 3801–3808.
Kuchen B., Maturano Y. P., Mestre M. V., Combina M., Toro M. E., Vazquez F. (2019). Selection of native non-Saccharomyces yeasts with biocontrol activity against spoilage yeasts in order to produce healthy regional wines. Fermentation 5, 60. doi: 10.3390/fermentation5030060
Kuchen B., Vazquez F., Maturano Y. P., Scaglia G. J. E., Pera L. M., Vallejo M. D. (2021). Toward application of biocontrol to inhibit wine spoilage yeasts: The use of statistical designs for screening and optimisation. Oneo One 2, 75–96. doi: 10.20870/oeno-one.2021.55.2.4510
Kuchen B., Vazquez F., Mestre M. V., Toro M. E., Maturano Y. P. (2018). Influence of cold maceration time on chromatic and microbiological characteristics of Cabernet Sauvignon wines. S. Afr. J. Enol. Viticult. 39, 89–99. doi: 10.21548/39-1-2460
Kumar M., Nagpal R., Kumar R., Hemalatha R., Verma V., Kumar A., et al. (2012). Cholesterol-lowering probiotics as potential biotherapeutics for metabolic diseases. J. Diab. Res. 2012 (1), 902917. doi: 10.1155/2012/902917
Lai G., Addis M., Caredda M., Fiori M., Dedola A. S., Furesi S., et al. (2024). Development and characterization of a functional ice cream from sheep milk enriched with microparticulated whey proteins, inulin, omega-3 fatty acids, and bifidobacterium BB-12®. Dairy 5, 134–152. doi: 10.3390/dairy5010011
Lee S. Y., Park H. J., Best-Popescu C., Jang S., Park Y. K. (2015). The effects of ethanol on the morphological and biochemical properties of individual human red blood cells. PloS One 10, e0145327. doi: 10.1371/journal.pone.0145327
Li S., Zhao Y., Zhang L., Zhang X., Huang L., Li D., et al. (2012). Antioxidant activity of Lactobacillus plantarum strains isolated from traditional Chinese fermented foods. Food Chem. 135, 1914–1919. doi: 10.1016/j.foodchem.2012.06.048
Ling H., Liu R., Sam Q. H., Shen H., Chai L. Y. A., Chang M. W. (2023). Engineering of a probiotic yeast for the production and secretion of medium-chain fatty acids antagonistic to an opportunistic pathogen Candida albicans. Front. Bioeng. Biotechnol. 11, 1090501. doi: 10.3389/fbioe.2023.1090501
Manson J. E., Tosteson H., Ridker P. M., Satterfield S., Hebert P., O’Connor G. T., et al. (1992). The primary prevention of myocardial infarction. New Engl. J.edic 326, 1406–1416. doi: 10.1056/NEJM199205213262107
Manzo-Avalos S., Saavedra-Molina A. (2010). Cellular and mitochondrial effects of alcohol consumption. Int. J. Environm. Res. Public Health 7, 4281–4304. doi: 10.3390/ijerph7124281
Maturano Y. P. (2011). Cultivos mixtos de levaduras en Enología: aprovechamiento de actividades enzimáticas y fenómenos antagónicos (Tucumán, Argentina: Universidad Nacional de Tucumán, Facultad de bioquímica, química y farmacia. Trabajo de posgrado para la obtención del grado académico superior de Doctor/a en Ciencias Biológicas. Carrera de Doctorado en Ciencias Biológicas).
Maturano Y. P., Assaf L. A. R., Toro M. E., Nally M. C., Vallejo M., Castellanos de Figueroa L. I., et al. (2012). Multi-enzyme production by pure and mixed cultures of Saccharomyces and non-Saccharomyces yeasts during wine fermentation. Int. J. Food Microbiol. 155, 43–50. doi: 10.1016/j.ijfoodmicro.2012.01.015
Maturano Y. P., Assof M., Fabani M. P., Nally M. C., Jofré V., Rodríguez Assaf L. A., et al. (2015a). Enzymatic activities produced by mixed Saccharomyces and non-Saccharomyces cultures: relationship with wine volatile composition. Antonie Van Leeuwenhoek 108, 1239–1256. doi: 10.1007/s10482-015-0578-0
Maturano Y. P., Mestre M. V., Esteve-Zarzoso B., Nally M. C., Lerena M. C., Toro M. E., et al. (2015b). Yeast population dynamics during prefermentative cold soak of Cabernet Sauvignon and Malbec wines. Int. J. Food Microbiol. 199, 23–32. doi: 10.1016/j.ijfoodmicro.2015.01.005
Maturano Y. P., Nally M. C., Eugenia T. M., Figueroa de Castellanos L. I., Vazquez F. (2009). Estudio cualitativo de actividades enzimáticas y fenómeno killer en levaduras vínicas. Rev. Enol. 1, 1–11.
Menezes A. G. T., Ramos C. L., Cenzi G., Melo D. S., Dias D. R., Schwan R. F. (2020). Probiotic potential, antioxidant activity, and phytase production of indigenous yeasts isolated from indigenous fermented foods. Probiot. Antimicrob. Proteins 12, 280–288. doi: 10.1007/s12602-019-9518-z
Merchán A. V., Ruiz-Moyano S., Hernández M. V., Benito M. J., Aranda E., Rodríguez A., et al. (2022). Characterization of autochthonal yeasts isolated from Spanish soft raw ewe milk protected designation of origin cheeses for technological application. J. Dairy Sci. 105, 2931–2947. doi: 10.3168/jds.2021-21368
Mestre Furlani M. V., Maturano Y. P., Combina M., Mercado L. A., Toro M. E., Vazquez F. (2017). Selection of non-Saccharomyces yeasts to be used in grape musts with high alcoholic potential: A strategy to obtain wines with reduced ethanol content. FEMS Yeast Res. 17, fox010. doi: 10.1093/femsyr/fox010
Mogmenga I., Somda M. K., Ouattara C. A. T., Keita I., Dabiré Y., Diguţă C. F., et al. (2023). Promising probiotic properties of the yeasts isolated from rabilé, a traditionally fermented beer produced in Burkina Faso. Microorganisms 11, 802. doi: 10.3390/microorganisms11030802
Monika K., Malik T., Gehlot R., Rekha K., Kumari A., Sindhu R., et al. (2021). Antimicrobial property of probiotics. Environm. Conserv. J. 22, 33–48. doi: 10.36953/ECJ.2021.SE.2204
Muccilli S., Wemhoff S., Restuccia C., Meinhardt F. (2013). Exoglucanase-encoding genes from three Wickerhamomyces anomalus killer strains isolated from olive brine. Yeast 30, 33–43. doi: 10.1002/yea.v30.1
Mugwanya M., Dawood M. A., Kimera F., Sewilam H. (2021). Updating the role of probiotics, prebiotics, and synbiotics for tilapia aquaculture as leading candidates for food sustainability: A review. Probiot. Antimicrob. Proteins, 1–28.
Nally M. C., Pesce V. M., Maturano Y. P., Assaf L. R., Toro M. E., De Figueroa L. C., et al. (2015). Antifungal modes of action of Saccharomyces and other biocontrol yeasts against fungi isolated from sour and grey rots. Int. J. Food Microbiol. 204, 91–100. doi: 10.1016/j.ijfoodmicro.2015.03.024
Neal-McKinney J. M., Lu X., Duong T., Larson C. L., Call D. R., Shah D. H., et al. (2012). Production of organic acids by probiotic Lactobacilli can be used to reduce pathogen load in poultry. doi: 10.1371/journal.pone.0043928
Ogunremi O. R., Agrawal R., Sanni A. (2020). Production and characterization of volatile compounds and phytase from potentially probiotic yeasts isolated from traditional fermented cereal foods in Nigeria. J. Genet. Eng. Biotechnol. 18, 1–8. doi: 10.1186/s43141-020-00031-z
Ogunremi O. R., Sanni A. I., Agrawal R. (2015a). Probiotic potentials of yeasts isolated from some cereal-based Nigerian traditional fermented food products. J. Appl. Microbiol. 119, 797–808. doi: 10.1111/jam.12875
Ogunremi O. R., Sanni A. I., Agrawal R. (2015b). Hypolipidaemic and antioxidant effects of functional cereal-mix produced with probiotic yeast in rats fed high cholesterol diet. J. Funct. Foods 17, 742–748. doi: 10.1016/j.jff.2015.06.031
Parafati L., Palmeri R., Pitino I., Restuccia C. (2022). Killer yeasts isolated from olive brines: Technological and probiotic aptitudes. Food Microbiol. 103, 103950. doi: 10.1016/j.fm.2021.103950
Peréz-Través L., de Llanos R., Flockhart A., García-Domingo L., Groenewald M., Pérez-Torrado R., et al. (2021). Virulence related traits in yeast species associated with food; Debaryomyces hansenii, Kluyveromyces marxianus, and Wickerhamomyces anomalus. Food Control 124, 107901. doi: 10.1016/j.foodcont.2021.107901
Perricone M., Bevilacqua A., Corbo M. R., Sinigaglia M. (2014). Technological characterization and probiotic traits of yeasts isolated from Altamura sourdough to select promising microorganisms as functional starter cultures for cereal-based products. Food Microbiol. 38, 26–35. doi: 10.1016/j.fm.2013.08.006
Prabina B. J., Kumutha K., Anandham R., Durga P. (2019). Isolation and characterization of multifunctional yeast as plant probiotics for better crop nutrition in pulses. Int. J. Curr. Microbiol. Appl. Sci. 8, 2711–2718. doi: 10.20546/ijcmas.2019.801.286
Psomas E. I., Fletouris D. J., Litopoulou-Tzanetaki E., Tzanetakis N. (2003). Assimilation of cholesterol by yeast strains isolated from infant feces and Feta cheese. J. Dairy Sci. 86, 3416–3422. doi: 10.3168/jds.S0022-0302(03)73945-9
Ragavan M. L., Das N. (2020). In vitro studies on therapeutic potential of probiotic yeasts isolated from various sources. Curr. Microbiol. 77, 2821–2830. doi: 10.1007/s00284-020-02100-5
Santos A., San Mauro M., Bravo E., Marquina D. (2009). PMKT2, a new killer toxin from Pichia membranifaciens, and its promising biotechnological properties for control of the spoilage yeast Brettanomyces bruxellensis. Microbiology 155, 624–634. doi: 10.1099/mic.0.023663-0
Seng K. (2014). Bioprocess strategy for the production of Lactobacillus rhamnosus NRRL B442 with high cell-b-glucosidase activity (Malaysia: Faculty of Chemical Engineering, University Technology of Malaysia).
Shruthi B., Deepa N., Somashekaraiah R., Adithi G., Divyashree S., Sreenivasa M. Y. (2022). Exploring biotechnological and functional characteristics of probiotic yeasts: A review. Biotechnol. Rep. 34, e00716. doi: 10.1016/j.btre.2022.e00716
Simões L. A., Cristina de Souza A., Ferreira I., Melo D. S., Lopes L. A. A., Magnani M., et al. (2021). Probiotic properties of yeasts isolated from Brazilian fermented table olives. J. Appl. Microbiol. 131, 1983–1997. doi: 10.1111/jam.v131.4
Srinivasan R., Krishnan S. R., Ragunath K. S., Ponni K. K., Balaji G., Prabhakaran N., et al. (2022). Prospects of utilizing a multifarious yeast (MSD1), isolated from the South Indian coast as an Agricultural input. Biocatal. Agric. Biotechnol. 39, 102232. doi: 10.1016/j.bcab.2021.102232
Staniszewski A., Kordowska-Wiater M. (2021). Probiotic and potentially probiotic yeasts—Characteristics and food application. Foods 10, 1306. doi: 10.3390/foods10061306
Syal P., Vohra A. (2013). Probiotic potential of yeasts isolated from traditional Indian fermented foods. Int. J. Microbiol. Res. 5, 390. doi: 10.9735/0975-5276.5.2.390-398
Tejero-Sariñena S., Barlow J., Costabile A., Gibson G. R., Rowland I. (2012). In vitro evaluation of the antimicrobial activity of a range of probiotics against pathogens: evidence for the effects of organic acids. Anaerobe 18, 530–538. doi: 10.1016/j.anaerobe.2012.08.004
Tran K. D., Le-Thi L., Vo H. H., Dinh-Thi T. V., Nguyen-Thi T., Phan N. H., et al. (2023). Probiotic Properties and Safety Evaluation in the Invertebrate Model Host Galleria mellonella of the Pichia kudriavzevii YGM091 Strain Isolated from Fermented Goat Milk. Probiot. Antimicrob. Proteins 16 (4), 1288–1303.
Tripathy A., Dash J., Kancharla S., Kolli P., Mahajan D., Senapati S., et al. (2021). Probiotics: a promising candidate for management of colorectal cancer. Cancers 13, 3178. doi: 10.3390/cancers13133178
Tomusiak-Plebanek A., Heczko P., Skowron B., Baranowska A., Okoń K., Thor P. J., et al. (2018). Lactobacilli with superoxide dismutase-like or catalase activity are more effective in alleviating inflammation in an inflammatory bowel disease mouse model. Drug Design Dev. Ther. 12, 3221–3233. doi: 10.2147/DDDT.S164559
Van de Wouw M., Boehme M., Lyte J. M., Wiley N., Strain C., O’Sullivan O., et al. (2018). Short-chain fatty acids: microbial metabolites that alleviate stress-induced brain–gut axis alterations. J. Physiol. 596, 4923–4944. doi: 10.1113/JP276431
Vergara S. C., Leiva M. J., Mestre M. V., Vazquez F., Mancha P., Nally M. C., et al. (2023b). Bioprospecting of the probiotic potential of yeasts isolated from a wine environment. Fungal Genet. Biol. 164, 103767. doi: 10.1016/j.fgb.2022.103767
Vergara S. C., Leiva M. J., Mestre M. V., Vazquez F., Nally M. C., Maturano Y. P. (2023a). Non-Saccharomyces yeast probiotics: revealing relevance and potential. FEMS Yeast Res., foad041. doi: 10.1093/femsyr/foad041
Vergara S. C., Leiva M. J., Terrera N., Mestre M. V., Petrignani D. B., Nally M., et al. (2023c). Probiotic properties in indigenous wine yeast (San Juan, Argentina: XLI Reunión Anual de la sociedad de biología de Cuyo), 81.
Vucenik I., Shamsuddin A. M. (2006). Protection against cancer by dietary IP6 and inositol. Nutr. Cancer 55, 109–125. doi: 10.1207/s15327914nc5502_1
Wang J., Da R., Tuo X., Cheng Y., Wei J., Jiang K., et al. (2020). Probiotic and safety properties screening of Enterococcus faecalis from healthy Chinese infants. Probiot. Antimicrob. Proteins 12, 1115–1125. doi: 10.1007/s12602-019-09625-7
Keywords: probiotic yeasts, biosecurity, enzyme production, antioxidant activity, antimicrobial properties, cholesterol-lowering
Citation: Leiva Alaniz MJ, Vergara SC, Mestre Furlani MV, Vazquez F, Mancha-Agresti P and Maturano YP (2025) Assessment of yeasts from winemaking environments: biosafety and functional perspectives on probiotic capabilities. Front. Ind. Microbiol. 3:1494334. doi: 10.3389/finmi.2025.1494334
Received: 10 September 2024; Accepted: 17 March 2025;
Published: 08 April 2025.
Edited by:
Eleftherios H. Drosinos, Agricultural University of Athens, GreeceReviewed by:
John Kapolos, University of Peloponnese, GreeceFrancesco Martelli, University of Parma, Italy
Katarzyna Pobiega, Warsaw University of Life Sciences, Poland
Copyright © 2025 Leiva Alaniz, Vergara, Mestre Furlani, Vazquez, Mancha-Agresti and Maturano. This is an open-access article distributed under the terms of the Creative Commons Attribution License (CC BY). The use, distribution or reproduction in other forums is permitted, provided the original author(s) and the copyright owner(s) are credited and that the original publication in this journal is cited, in accordance with accepted academic practice. No use, distribution or reproduction is permitted which does not comply with these terms.
*Correspondence: Yolanda Paola Maturano, cGFvbGFtYXR1cmFub0BnbWFpbC5jb20=
†These authors have contributed equally to this work and share first authorship