- 1Department of Food Science and Engineering, Foshan University, Foshan, China
- 2Guangdong Engineering Research Center for Traditional Fermented Food, Foshan University, Foshan, China
- 3Guangdong Engineering Research Center for Safety Control of Food Circulation, Foshan University, Foshan, China
- 4Foshan Engineering Research Center for Brewing Technology, Foshan University, Foshan, China
- 5Foshan Guochuang Biological Fermented Food Technology Innovation Center, Foshan, China
- 6Foshan Nanhai Shimen Experimental School, Foshan, China
- 7Guangdong Experimental High School, Guangzhou, China
Bacillus velezensis has a wide range of beneficial activities, such as fighting plant diseases, promoting growth, improving crops’ ability to handle stress, and boosting plant defenses. These traits make it a strong candidate for agricultural use, especially as a biocontrol agent and growth-promoting bacteria. This review takes a close look at the origins, working mechanisms, and potential agricultural benefits of B. velezensis. Both lab and field studies have shown its success in reducing plant diseases and supporting crop growth by producing helpful compounds, taking up space in the environment, and strengthening plant defenses. Although B. velezensis is already used in some fertilizers and biopesticides, there are still challenges in scaling up production, selecting the right strains, and ensuring product stability. This review points out current research gaps and suggests future directions, such as improving strain selection, developing better biofertilizers, and advancing production techniques to make the most of B. velezensis in agriculture. These findings aim to guide further research and improve its use in sustainable farming.
1 Introduction
Bacillus velezensis is a functional bacterium(a class of beneficial microorganisms that exert one or more physiological effects, directly or indirectly influencing the health of the host.) with a broad range of biological activities. It is found in various habitats (including soil, plant rhizosphere, and even aquatic environments) and was first isolated and identified in 1999, although its taxonomic classification remained unresolved until 2005 (Ruiz-Garcia et al., 2005). B. velezensis is characterized by its strong enzyme production capacity, abundant secondary metabolites, robust stress resistance, broad-spectrum antibacterial activity, and high efficacy against pathogens (Masmoudi et al., 2019; Zhang et al., 2019; Keshmirshekan et al., 2024; Xu et al., 2024). It can inhibit pathogenic infections and promote plant growth under both biotic (fungi, bacteria, and insects) and abiotic (temperature, water, and saline-alkaline) stress conditions. Due to these attributes, B. velezensis is widely applied in agricultural production and holds significant value in practical applications (Ye et al., 2018; Zhang et al., 2020; Chen et al., 2022; Cao et al., 2024; Su et al., 2024).
In light of the rapid growth of the global population, ensuring the production and safety of key agricultural products, including food, has become increasingly vital. While chemical pesticides and fertilizers have been widely used to boost agricultural output, their excessive and improper application has led to various issues such as soil degradation, pest resistance, reduced crop yields, and increased pesticide residues in the environment (Khan et al., 2022). In this context, microbial-based approaches are gaining attention as sustainable alternatives. Among these, Bacillus velezensis has emerged as a promising candidate due to its ability to antagonize plant pathogens, promote plant growth, and improve crop resilience to environmental stresses (Singh et al., 2021). Unlike traditional chemical inputs, B. velezensis offers a broad spectrum of benefits, including enhancing nutrient absorption, improving soil structure, and inducing plant systemic resistance (Singh and Trivedi, 2017). Therefore, its application as a biocontrol agent and plant growth-promoting bacterium holds great potential for achieving environmentally friendly agricultural practices that support food security and crop health. This review focuses on the biological properties and agricultural potential of B. velezensis, highlighting its role in disease resistance and growth promotion, and addressing current challenges in its commercial application.
Given its wide range of beneficial activities, Bacillus velezensis has attracted increasing attention as a promising candidate for agricultural applications. This review provides a comprehensive overview of the latest research on B. velezensis, focusing on its origin, disease resistance mechanisms, and growth-promoting effects. In addition, we analyze its potential applications in biopesticides and biofertilizers. The manuscript covers both laboratory and field studies, highlighting the ability of B. velezensis to reduce plant diseases, promote crop growth, and enhance stress resistance. Finally, we discuss the current challenges related to strain selection, large-scale production, and product stability, while offering suggestions for future research to fully unlock the agricultural potential of B. velezensis. By addressing these challenges, this review aims to provide valuable insights that will support the development of more sustainable and effective agricultural practices.
2 Isolation source of B. velezensis
B.velezensis is a Gram-positive, aerobic, endospore-forming bacterium; it is widely present in various habitats (Kim et al., 2021; Zhang et al., 2022). A search of the B. velezensis genome in the NCBI database (as of September 2023) identified 625 strains; they are mainly sourced from soil (24.32%) and plant rhizosphere and phyllosphere (19.84%). The sources of B. velezensis from rivers and sediments, food, animal gastrointestinal tract and contents, and animal feces accounted for 5.28%, 9.12%, 3.52%, and 1.92%, respectively; 14.56% of strains were sourced from other environments, while 21.44% had unknown origins (Figure 1). In summary, while B. velezensis is found in diverse ecological environments, its primary sources are the rhizosphere soil and root tissue of plants.
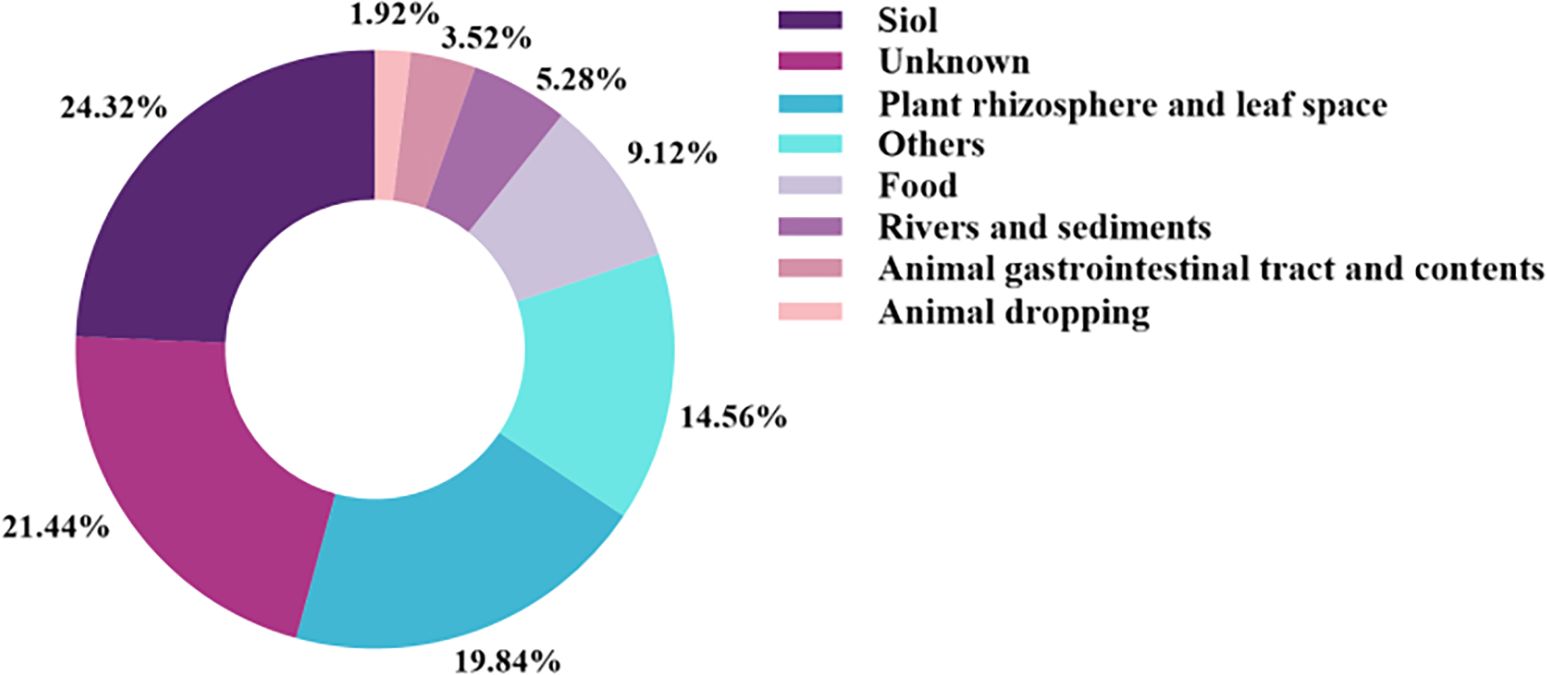
Figure 1. Source of B. velezensis. The sources of B. velezensis from soil, plant rhizosphere and leaf space, rivers and sediments, food, animal gastrointestinal tract and contents, and animal feces accounted for 24.32%, 19.84%, 5.28%, 9.12%, 3.52%, and 1.92%, respectively. Other and unknown sources accounted for 14.56% and 21.44%, respectively.
According to the results of literature research (Rabbee et al., 2022; Jia et al., 2023), B. velezensis used for disease resistance and growth promotion is mainly isolated from plant rhizosphere and soil and some from plant tissue sites, such as leaf surface and fruit surface. In addition to plant sources, some special habitat-derived strains, such as B. velezensis, exhibit antibacterial and growth promoting biological characteristics. For example, Barman et al. isolated B. velezensis from coal mining areas, which can not only tolerate various heavy metals, but also produce auxin, iron carrier, and various plant-promoting biomass, as well as dissolve phosphate (Barman et al., 2022). Alvarez et al. isolated B. velezensis MEP218 from saline soil, thereby producing lipopeptide antibacterial compounds, such as surfactin, iturin, and fengycin, and can significantly inhibit soybean stem rot caused by Sclerotinia sclerotiorum (Alvarez et al., 2012). Wekesa et al. isolated two strains of Bacillus velezensis from Lake Bogoria, a region known for its hot springs. Their study demonstrated that these strains could grow in a wide pH range, tolerate varying salinity conditions, and thrive at diverse temperatures. Additionally, both strains exhibited the ability to produce IAA and solubilize phosphorus (Wekesa et al., 2022). In summary, B. velezensis is widely distributed and has strong stress resistance. B. velezensis from different habitats also exhibit strong foundation for screening excellent strains with special requirements, thereby expanding their potential application and prospects.
3 Disease resistance and growth-promoting effects of B. velezensis
3.1 Disease resistance effect of B. velezensis
As a newly identified biocontrol bacterium, B. velezensis is synonymous with Bacillus methylotrophicus and Bacillus amyloliquefaciens subsp. Plantarum (Dunlap et al., 2016). Recently, these bacteria have been reclassified under B. velezensis. Some of the B. velezensis identified so far have broad-spectrum antibacterial activity, effectively inhibiting various plant pathogens (fungi, bacteria, viruses, and nematodes) in agricultural production and efficiently controlling various plant diseases, especially those caused by plant pathogenic fungi (Medhioub et al., 2022; Yin et al., 2022). Partial results are summarized in Table 1.
B. velezensis can significantly inhibit fungal diseases caused by pathogens, such as Fusarium oxysporum, Phytophthora capsici, and Botrytis cinerea. For example, Hong et al. isolated a strain of B. velezensis CE100 from tomato planting soil that can produce chitinase and β-Dextranase. This strain degrades fungal cell walls and effectively inhibits the growth of F. oxysporum mycelium. In addition, under co-inoculation with B. velezensis, CE 100 and plant pathogenic fungi can effectively inhibit strawberry wilt disease (Hong et al., 2022). Rahman et al. used silica gel thin-layer chromatography to isolate the antibacterial substance of B. velezensis UQ156. The results indicate that the metabolites of UQ156 contain 2,5-diketopiperazine compounds with broad-spectrum antibacterial and antiviral activities, which can effectively inhibit P. capsici (Syed-Ab-Rahman et al., 2021). Navarro et al. found that the volatile compounds produced by B. velezensis XT1 greatly inhibit the growth of B. cinerea. When exposed to these volatile compounds, the mycelial membrane and cell wall of B. cinerea underwent thinning and degradation. This process led to the complete coagulation of cytoplasmic contents and the loss of identifiable organelles, ultimately resulting in cell death. As a consequence, the growth and development of pathogenic fungi were inhibited (Toral et al., 2021).
In addition to effectively preventing and treating fungal diseases, B. velezensis exhibits strong inhibitory effects against plant diseases caused by bacteria, viruses, and nematodes. B. velezensis SC60 with strong colonization ability from sesbania was screened by Dong et al (Dong et al., 2022). This bacterium can significantly inhibit not only plant pathogens, such as P. capsici, F. oxysporum, Fusarium graminearum, and B. cinerea, but also the growth of various Bacillus subtilis, Bacillus megaterium, and B. amyloliquefaciens. A biocontrol strain B. velezensis SYL-3 that can colonize tobacco leaves was isolated by Liu et al (Liu et al., 2022). The bacterial suspension has a significant inhibitory effect on tobacco mosaic virus. With research confirming that the bacterium can alter soil microbial community structure and dynamics, thereby suppressing tobacco mosaic virus-induced diseases. B. velezensis Bv-25, isolated by Tian et al., can effectively kill most southern root knot nematodes (Tian et al., 2022). This strain readily colonizes crop roots, inhibiting the in vitro hatching of insect eggs. Furthermore, it can activate the defense signaling pathways of salicylic acid (SA) and jasmonic acid (JA) in crop roots, leading to the upregulation of plant defense genes. As a result, it induces enhanced crop resistance to southern root knot nematode infection.
3.2 Promoting effect of B. velezensis
In addition to the advantages in inhibiting plant pathogens, B. velezensis significantly promotes plant yield, root length, and fruiting rate, thereby effectively enhancing plant growth. Hasan et al. isolated a strain of B. velezensis HNH9 that can produce siderophores. In greenhouse cultivation experiments, plants treated with HNH9 showed an increase in their photosynthetic rate and stomatal conductance. The expression of genes involved in ethylene (ET) biosynthesis was down-regulated, whereas that of growth-related genes was up-regulated, promoting the growth of upland cotton plants (Hasan et al., 2022). Choub et al. isolated a strain of B. velezensis CE100 from the pot soil of tomato plants. This bacterium produces indole-3-acetic acid (up to1.4 µg/mL) and shows potential for ammonia production and phosphate solubilization. The field test results showed that after inoculation with this bacterium, the chlorophyll content, total nitrogen, and total phosphorus content of walnut trees increased by 1.3, 1.5, and 1.6 times compared with the control group, respectively. Additionally, branch length, rhizome diameter, and biomass of walnut trees were significantly enhanced (Choub et al., 2021).
Plants are subjected to various abiotic or biological stresses during their growth process, including salt alkali stress, heavy metal stress, and drought stress. The application of B. velezensis as plant growth-promoting rhizobacteria (PGPR) has been shown to enhance resistance to abiotic stress in plants (Ayaz et al., 2021), demonstrating its strong stress resistance. It not only adapts to growth under non-stress conditions but also promotes plant growth under stress. Wang Ru et al. isolated a salt-tolerant B. velezensis A-1 from saline soil; it could grow stably under 0%–10% NaCl stress. It could also improve the germination rate of wheat seeds under different salt concentrations and promote the growth of seedlings (Wang et al., 2021). B. velezensis DD10 isolated from the coal dumping area by Barman et al. can not only tolerate various heavy metals, but also produce auxin and siderophore, showing 1-aminocyclopropane-1-carboxylic acid (ACC) deaminase activity and the ability to dissolve phosphate (Barman et al., 2022). Nadeem et al. isolated and screened B. velezensis D3 from the rhizosphere soil of corn in water-deficient areas, which have ACC deaminase activity and extracellular polysaccharide production activity. In the drought resistance test, this strain showed significant effects in improving physiological parameters, such as photosynthetic rate, stomatal conductance, steam pressure, water use efficiency, and transpiration rate of maize plants. At the same time, it can significantly increase the stem length, root length, and dry and fresh weights of maize seedlings, effectively promoting plant growth (Nadeem et al., 2021).
4 Resistance and growth-promoting mechanism of B. velezensis
Bacteria used for plant disease resistance and growth promotion have many direct or indirect mechanisms of action. These mechanisms enhance plant resistance to diseases and promote growth in different agricultural environments. The currently recognized mechanisms include metabolic regulation, control of plant hormone levels, and root colonization (Ali et al., 2017; Ismail et al., 2021; Khan et al., 2021), as well as stimulation of plant resistance to various biotic and abiotic stresses (such as pathogen attacks and heavy metal pollution) to indirectly improve plant growth (Mustafa et al., 2019). At present, the research on the mechanism of disease resistance and growth promotion of B velezensis mainly includes the following points (Figure 2): nutrition and ecological site competition, production of secondary metabolites, and stimulation of plant system resistance. Moreover, B. velezensis can exert synergistic effects on disease resistance and growth promotion through one or more of these mechanisms.
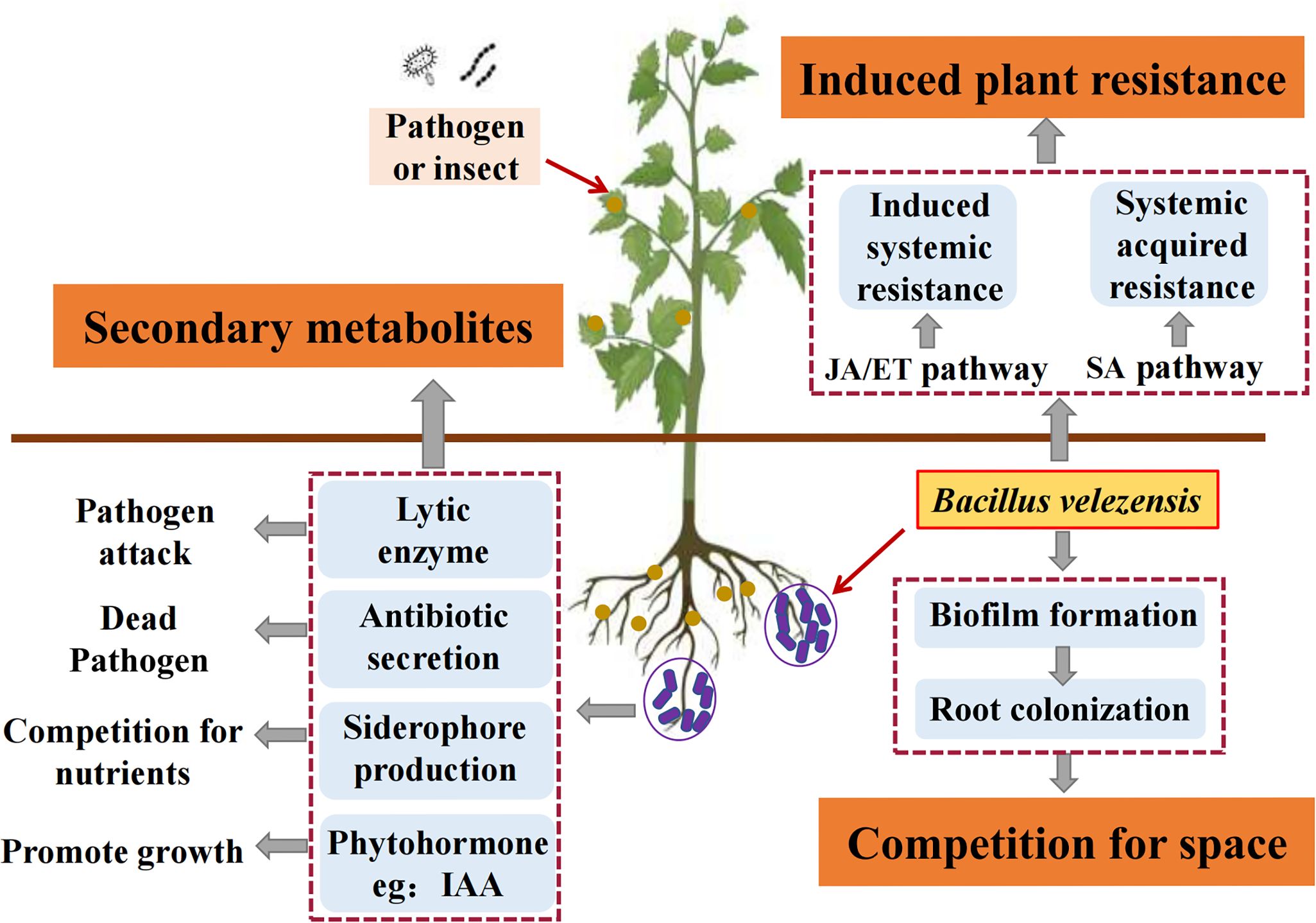
Figure 2. Resistance and growth-promoting mechanism of B. velezensis. Secondary metabolites: B. velezensis achieves disease resistance and growth promotion by producing secondary metabolites, such as lytic enzyme, antibiotic secretion, siderophore production, and phytohormone. Induced plant resistance: by activating auxin and ET hormone signaling transcription-related genes, B. velezensis significantly increased the expression levels of defense genes related to SA, JA, and ET signaling pathways and induced plant resistance, thereby achieving disease resistance and growth promotion. Competition for space: B. velezensis colonizes the rhizosphere of plants by forming biofilms that are resistant to environmental stress, enhancing its effectiveness in disease resistance and growth promotion.
4.1 Nutrition and ecological site competition
Soil microorganisms are widely distributed in plant roots; the integration of beneficial bacterial communities into host plants contributes to soil nutrient cycling and enhances nutrient use efficiency (Nwachukwu et al., 2021). Many studies have shown that microbial inoculants can induce changes in plant rhizosphere microbial communities and improve soil fertility, soil environment, and crop growth (Huang et al., 2022). B. velezensis interacts with plants through colonization and competes with pathogenic microorganisms for limited nutrient elements and spatial sites, preventing pathogenic bacteria from obtaining the necessary nutrients and living space for growth, thereby exerting their disease resistance and growth promoting effects. The competition primarily centers on critical nutrients such as iron, potassium, and phosphorus, with iron being particularly essential for biological growth. The primary metabolite siderophore produced by B. velezensis has a very high affinity for trivalent iron, thereby forming an iron-siderophore complex to promote the absorption of iron by microorganisms. At the same time, plants can use the complex to increase the iron content in plant tissues and promote plant growth (Chuljerm et al., 2020).
The competition for ecological sites is an important factor that enables B. velezensis to contribute to disease resistance, growth promotion, and the development of microbial control agents. The formation of biofilm is conducive to the colonization of microorganisms. B. velezensis with its biofilm-forming capability holds a distinct advantage in competing for ecological sites (Flemming et al., 2016). Even under the presence of pathogens, B. velezensis NKG-2 forms a biofilm that is resistant to environmental pressure and interacts symbiotically with tomato plants, thereby protecting them from the harmful effects of F. oxysporum (Myo et al., 2021). Numerous studies have shown that B. subtilis can colonize the rhizosphere of crops, such as corn, cucumber, and tomato, and have significant control effects on plant diseases, such as corn stem rot and tomato gray mold (De Fátima Dias Diniz et al., 2022; Másmela-Mendoza and Moreno-Velandia, 2022). For example, Jin et al. found that B. velezensis S3-1 can effectively colonize maize rhizosphere soil and root tissue. The collected corn root exudates significantly induced the chemotaxis, cluster movement, and biofilm formation of strain S3-1. These results indicate that maize root exudates play an important role in the colonization of S3-1 (Jin et al., 2019). Agarwal et al. isolated a growth-promoting B. velezensis strain from medicinal plants and found that it can colonize the roots of tomato seedlings, promoting growth and effectively controlling bacterial wilt disease (Agarwal et al., 2020).
4.2 Producing bioactive substances
B. velezensis can produce various broad-spectrum antimicrobial substances to contribute to disease resistance. The main antimicrobial substances are antimicrobial proteins, lipopeptides, polyketides, and volatile compounds. Among them, antibacterial proteins mainly include proteases, chitinases, and β-Dextranase (Li et al., 2020). Lipopeptide substances mainly include surfactins, bacillomycin-D, and fengycins (Ongena and Jacques, 2008). Polyketone compounds mainly include bacillaene, difficidin, and macroactin (Tan et al., 2020).Volatile compounds mainly include acetylurea, isoamyl alcohol, and 2,3-butanediol (Liu et al., 2020; Toral et al., 2021). Reports showed that lipopeptide compounds are the main contributors to the biocontrol activity of B. velezensis. Notably, B velezensis possesses genetic characteristics related to the synthesis of cyclic lipopeptides (Rabbee et al., 2019).
In addition to abundant antibacterial substances, B. velezensis produces various growth promoting substances, such as auxin, iron carrier, and 1-aminocyclopropane-1-carboxylate deaminase, to promote plant growth. Pacifico et al. isolated three strains of B. velezensis from the cotton rhizosphere, producing auxin and iron carriers and possessing phosphorus and nitrogen fixing effects. These strains of B. velezensis may be promoters of plant growth (Pacifico et al., 2021). Chebotar et al. isolated the plant growth-promoting bacterium B. velezensis BS89 from the rhizosphere of winter wheat. Genome-wide sequencing revealed that the genome of BS89 contains a synthetic gene cluster of indole-3-acetic acid and volatile metabolites that promote plant growth (Chebotar et al., 2021). Wang et al. isolated 3S, 3R, and 4R-acetylbutanediol, which is obtained from the fermentation broth of B. velezensis WRN031. The fungus secreted two non-active compounds in a ratio of 1:2 into the soil when it was inoculated into the rhizosphere soil of corn seedlings. After seven days of treatment with the two compounds, the roots of corn seedlings were significantly longer than those of the control plant (Wang et al., 2020).
In recent years, many studies have shown that B.velezensis contains a large number of gene clusters encoding secondary metabolites, mainly including polyketide synthases (PKS) and non-ribosomal peptide synthetases (NRPS) (Xu et al., 2024). Different Bacillus velezensis contain different gene clusters. Nine gene clusters (srf, bmy, fen, dhb, bac, min, bae, dfn and nrs) were found in this genome (Fan et al., 2019a). Among them, surfactins (srf), bacillomycin-D (bmy) and fengycins (fen) are non-ribosomal lipopeptides (LPS). Macrolactin (mln), bacillaene (bae) and difficidin (dfn) are polyketides synthesized under the guidance of polyketide synthase.In addition, the siderophore bacillibactin (dhb) plays a role in protecting plants by competing with pathogenic microorganisms for nutrients such as iron.
The genome of Bacillus velezensis GQJK49 annotated by Ma et al. There were 12 gene clusters related to antimicrobial activity. Six of them presented high similarity with the biosynthesis gene clusters of relevant secondary metabolism. Two transAT polyketide synthase-nonribosomal peptide synthetase (TATPKS-NRPS)-type clusters showed similarity with the biosynthetic gene clusters of macrolactin and difficidin, respectively. Two gene clusters, which belonged to the transAT TATPKS-NRPS type, were related to bacillaene and fengycin, respectively. One gene cluster belonged to NRPS-bacteriocin, which was similar to the biosynthetic gene cluster of bacteriocin. One gene clusterwas related to bacilysin biosynthesis. The other gene clusters may be related to the production of new antimicrobial substances. The complete genome sequence of B. velezensis will be helpful in the study of its mechanisms for biocontrol and plant growth promotion and will facilitate the expansion of the scope of application of this strain in agriculture (Ma et al., 2017).
4.3 Inducing plant resistance
B. velezensis can induce plant resistance to protect plants from pathogen infection, including systemic acquired resistance (SAR) and induced systemic resistance (ISR). SA, JA, and ET play crucial roles in plant defense. SA-dependent signaling pathways are involved in SAR, whereas JA- and ET-dependent signaling pathways are involved in ISR. Jasmonic acid (JA) are involved in a variety of plant physiological processes, and salicylic acid (SA) plays an important role in stomatal behavior and respiration, among other functional roles. SA also functions in immune response by activating signaling pathways at the molecular level that lead to the induction of systemic disease resistance (Zou et al., 2024).
B. velezensis activates defense enzymes, such as phenylalanine ammonia lyase, ascorbic acid peroxidase, peroxidase, and catalase, in plants through its primary and secondary metabolites, such as volatile organic compounds, surface active- proteins, proteases, and acetylureas, thereby inducing plant resistance and improving plant disease resistance. Zhang et al. applied B. velezensis BY6 to the roots of poplar with root rot through pot experiments, and the plant growth indices (dry weight, fresh weight, and plant height) increased significantly. Auxin hormone signaling transcription-related genes were activated, and the expression levels of defense genes related to SA and JA signaling pathways were significantly increased, indicating that BY6 activated ISR and SAR was induced by diseased poplar seedlings to enhance their disease resistance (Zhang et al., 2022). Acetylurea produced by B. velezensis GJ1 causes callose deposition and enhances the activity of peroxidase, polyphenol oxidase, and phenylalanine ammonia lyase, thereby triggering ISR in plants. In addition, studies have found that reactive oxygen species play a crucial role in pathogen-plant interactions and play a crucial role in plant ISR (Peng et al., 2019). The subtilisin produced by B. velezensis LJ02 has no direct inhibitory effect on B. cinerea, but induced the outbreak of reactive oxygen species. This condition triggers SAR and enhances the plant’s resistance to B. cinerea (Hu et al., 2022).
5 Applications of B. velezensis in the plant field
5.1 B. velezensis as biological control agents
Bacillus is one of the most common endophytes in plants. Spores are resistant to adverse environmental conditions and can easily be developed and preserved due to their unique properties. They have unique advantages in the commercial production and application of microbial preparations (Aloo et al., 2019). The main Bacillus strains that have been developed as biological control agents include B. amyloliquefaciens, Bacillus licheniformis, B. subtilis, and Bacillus cereus (Fan et al., 2019b). B. velezensis is considered a potential biocontrol agent for plant disease prevention and control given its ability to stably colonize the rhizosphere or soil environment of plants, secrete various antibacterial active substances, and activate the host’s defense mechanisms against pathogens to inhibit harmful microorganisms (Hong et al., 2022). Research on the development of B. velezensis-based biological agents has been underway internationally for quite some time. B. velezensis FZB42 is a model strain of rhizosphere bacteria for plant biocontrol. It was isolated in 1998 and sequenced in 2007, and it is widely used in agriculture as a biological fertilizer and biocontrol agent (Fan et al., 2019b). However, the research and development of biopesticides based on B. velezensis in China are still in their infancy. As of September 2023, among the pesticide registration data available on the China Pesticide Information Network, two B. velezensis and five Bacillus methylotrophicus (currently classified as B. velezensis) have been registered; the results are shown in Table 2.
5.2 B. velezensis as bio-fertilizer
Biological fertilizer is a promising alternative to chemical fertilizer, which is greatly important for sustainable agriculture. At present, Bacillus velezensis is gradually being developed into commercial products internationally. Recognized as a model plant growth-promoting rhizobacterium, GB03 has exhibited outstanding performance in enhancing the growth and protection of many crop plants including cucumber, pepper, wheat, barley, soybean, and cotton (Jang et al., 2023). B. velezensis GB03 is commercially registered in the USA as a biocontrol and growth-promoting product, named Kodiak® (Gustafson, Inc., Plano, TX, USA), which is considered as one of the most effective biological control products currently available in the market. The use of Kodiak® is widespread and versatile. Like Kodiak®, Companion® (Advance Grass Solution Ltd., UK) is a commercial plant health product formulated from B. velezensis strain GB03, and is widely used in horticultural and agricultural production (Kloepper et al., 2004; Anckaert et al., 2021). Companion® application facilitates the rapid colonization of plant roots by GB03, leading to enhanced growth and effective pathogen inhibition across a variety of plant species. Although the number of Bacillus velezensis microbial fertilizers is increasing year by year, most products are still primarily microbial inoculants” to improve noun agreement and clarity. Commercial products such as bio-organic fertilizer, soil remediation agent, compound microbial fertilizer, microbial concentrate, and organic material decomposing agent are relatively scarce. This indicates that the types of Bacillus velezens is microbial fertilizers are not well-balanced, which means that the development and commercial application prospects of its product resources are broad.
5.3 B. velezensis as soil amendment
Soil is a dynamic and living resource. With the extensive use of pesticides and fertilizers, the discharge of industrial waste gases and wastewater, and the smelting of metal ores, the global soil environment has deteriorated. At present, many physical, chemical and biological improvement measures for soil remediation have been proposed by scientists. Plant-microorganism combined remediation technology has become the main research direction of soil remediation due to its advantages of in-situ remediation, low cost, environmental friendliness and high remediation efficiency (Chen et al., 2020; Chen et al., 2023).
According to the literature, Bacillus velezensis mainly focuses on the remediation of heavy metal contaminated land in soil remediation. The mechanisms by which Bacillus velezensis decontaminates heavy metals involves various methods, including siderophore chelation, biological adsorption, and biodegradation. Bacillus velezensis secrete metabolites that can release iron carriers and organic acids, which aid in the chelation of soil toxic metal ions and contribute to the adsorption of heavy metals (Li et al., 2022). The exopolysaccharides (EPS) produced by Bacillus velezensis can adsorb some metal ions such as Cd2+, Pb2+, Cu2+, etc (Abd-El-Haleem, 2023). When used as a soil remediation agent, Bacillus velezensis is often combined with Paenibacillus polymyxa, Paenibacillus mucilaginosus, Bacillus subtilis, Bacillus licheniformis, etc.to achieve better remediation effects. Combine Bacillus velezensis with Pseudomonas azotoformans, Serratia rubidaea, Paenibacillus pabuli. by Saadony et al. Compared to no or single PGPR treatment, the consortia increased the photosynthetic pigment contents, relative water content, and membrane stability index but lowered the electrolyte leakage and contents of malondialdehyde and hydrogen peroxide by suppressing the (non) enzymatic antioxidants in plant tissues. The contents of Cd, Cu, Pb and Ni in pepper were significantly decreased (El-Saadony et al., 2024). At present, the soil remediation agent on the market is also dominated by multi-strain combined bacteria. The commercial microbial fertilizer of Bacillus velezensis single bacteria as soil remediation agent has not been reported. It can be seen that the application of Bacillus velezensis in soil remediation has great development value.
6 Conclusion and future perspectives
After the taxonomic status of Bacillus velezensisi was determined, it has attracted extensive attention from researchers. In recent years, the research on the application of Bacillus velezensis in agriculture has become increasingly popular. The related research mainly focuses on plant growth promotion and disease prevention and control, animal feed, aquaculture and other aspects. More and more studies have shown that Bacillus velezensis has broad application prospects as a biocontrol bacterium:(1) It produces abundant secondary metabolites, induces plant systemic resistance, promotes plant growth, and reduces the use of chemical fertilizers; (2) Competitive ecological sites, reduce the abundance of pathogenic bacteria in the environment, inhibit the infection of pathogenic bacteria, and reduce the use of chemical drugs.
Laboratory and field experiments have proven that B. velezensis has excellent disease resistance and growth promoting effect on plants. At present, biological fungicides based on B. velezensis have been registered as pesticides and successfully applied to microbial fertilizers. However, the current research on the growth-promoting and disease-resistant aspects of the bacteria mainly focuses on theoretical aspects, such as its effectiveness and mechanism. Moreover, the industrial scale of using the bacteria for microbial preparations is small. Although B. velezensis has great potential, it has not been fully utilized in the agricultural field. On the one hand, highly active bacterial resources are limited, and on the other hand, the preparation technology of bacterial agents is insufficient to ensure the stability of product quality, resulting in a short shelf life of the product. Therefore, in the future, the development and utilization of B. velezensis for growth promotion and disease resistance for B. velezensis can be strengthened through the following aspects:
(1) exploration of highly active bacterial resources, improve the screening system, and selectively screen highly active agricultural strains; (2) develop bio fertilizers with minimal environmental impact and strengthen research on their biosafety assessment; (3) strain improvement through appropriate physical, chemical, biological, and other technological approaches to enhance the growth-promoting and disease-resistance performance of B. velezensis.
Author contributions
XZ: Funding acquisition, Writing – original draft. YJ: Writing – original draft. HR: Writing – original draft. TH: Supervision, Writing – review & editing. JZ: Data curation, Writing – review & editing. WF: Conceptualization, Writing – review & editing. JH: Formal analysis, Writing – review & editing. ZC: Formal analysis, Writing – review & editing. AW: Formal analysis, Writing – review & editing. HL: Formal analysis, Writing – review & editing. KZ: Visualization, Writing – review & editing. GH: Writing – review & editing.
Funding
The author(s) declare financial support was received for the research, authorship, and/or publication of this article. This work was supported by National Natural Science Foundation of China (32302023 and 32372284); Basic and Applied Basic Research Foundation of Guangdong Province (32072198, 2023A1515012536 and 2021B1515120042); Guangdong University Innovation Team Project (2021KCXTD034); Rural Revitalization Strategy Project Seed Industry Vitalization Action Project of Guangdong Province (2023WPY00002); Special Project for Research and Development in Key areas of Guangdong Province (2023KTSCX131).
Conflict of interest
The authors declare that the research was conducted in the absence of any commercial or financial relationships that could be construed as a potential conflict of interest.
Publisher’s note
All claims expressed in this article are solely those of the authors and do not necessarily represent those of their affiliated organizations, or those of the publisher, the editors and the reviewers. Any product that may be evaluated in this article, or claim that may be made by its manufacturer, is not guaranteed or endorsed by the publisher.
References
Abassum B., Raheem T., Ullah H., Khan A. (2022). Characterization of broad-spectrum biocontrol efficacy of Bacillus velezensis against Fusarium oxysporum in Triticum aestivum L. Not Bot. Horti Agrobo 50, 12590. doi: 10.15835/nbha50112590
Abd-El-Haleem D. (2023). Alpha-glucan: a novel bacterial polysaccharide and its application as a biosorbent for heavy metals. J. Genet. Eng. Biotechnol. 21, 133. doi: 10.1186/s43141-023-00609-3
Agarwal H., Dowarah B., Baruah P. M., Bordoloi K. S., Krishnatreya D. B., Agarwala N. (2020). Endophytes from Gnetum gnemon L. can protect seedlings against the infection of phytopathogenic bacterium Ralstonia solanacearum as well as promote plant growth in tomato. Microbiol. Res. 238, 126503. doi: 10.1016/j.micres.2020.126503
Ali M. A., Naveed M., Mustafa A., Abbas A. (2017). The good, the bad, and the ugly of rhizosphere microbiome. Probiotics Antimicrob. Proteins 2017, 253–290. doi: 10.1007/978-981-10-3473-2_11
Aloo B. N., Makumba B. A., Mbega E. R. (2019). The potential of Bacilli rhizobacteria for sustainable crop production and environmental sustainability. Microbiol. Res. 219, 26–39. doi: 10.1016/j.micres.2018.10.011
Alvarez F., Castro M., Príncipe A., Borioli G., Fischer S., Mori G., et al. (2012). The plant-associated Bacillus amyloliquefaciens strains MEP218 and ARP23 capable of producing the cyclic lipopeptides iturin or surfactin and fengycin are effective in biocontrol of sclerotinia stem rot disease. J. Appl. Microbiol. 112, 159–174. doi: 10.1111/j.1365-2672.2011.05182.x
Anckaert A., Arguelles Arias A., Hoff G., Calonne-Salmon M., Declerck S., Ongena M. (2021). The use of Bacillus spp. as bacterial biocontrol agents to control plant diseases. Oapen 2021, 54. doi: 10.19103/AS.2021.0093.10
Ayaz M., Ali Q., Farzand A., Khan H., Ling X., Gao S. (2021). Nematicidal volatiles from Bacillus atrophaeus GBSC56 promote growth and stimulate induced systemic resistance in tomato against Meloidogyne incognita. Int. J. Mol. Sci. 22, 5049. doi: 10.3390/ijms22095049
Barman D., Dutta I., Jha D. K. (2022). Heavy metal resistant bacteria from coal dumping site with plant growth promoting potentials. Biologia 77, 533–545. doi: 10.1007/s11756-021-00963-y
Cao Y. Z., Gu Y. L., Gao L., Fan X., Wang X. Y., Liu Y. C., et al. (2024). Research status and application prospect of Bacillus velezensis. Chin. J. Microecol. 36, 351–356. doi: 10.13381/j.cnki.cjm.202403017
Chakraborty N., Chakraborty N., Acharyya P., Acharya K. J. (2021). Isolation, characterization and identification of novel broad spectrum bacterial antagonist (s) to control Fusarium wilt of eggplant. Physiol. Mol. Plant Pathol. 116, 101711. doi: 10.1016/j.pmpp.2021.101711
Chebotar V. K., Voshol G. P., Malfanova N. V., Chizhevskaya E. P., Zaplatkin A. N., Komarova O. V., et al. (2021). Draft genome sequence of plant growth-promoting Bacillus velezensis BS89. Microbiol. Resour Ann. 10, 01294–01220. doi: 10.1128/MRA.01294-20
Chen D. Y., Gan Y., Zhang W. J., Yang Z. M., Chen L. B., Ma Y. C., et al. (2022). Screening, identification and stress resistance of antagonistic bacteria against tobacco target spot disease. J. Sichuan Agric. Univ. 40, 1–13. doi: 10.16036/j.issn.1000-2650.202108044
Chen H., Tian D., Li Z. (2023). Technologies for environmental ecological restoration and agricultural sustainability are the focus of future safeguarded agriculture development. Agronomy 14, 12. doi: 10.3390/agronomy14010012
Chen X., Kumari D., Cao C. J., Plaza G., Achal V. (2020). A review on remediation technologies for nickel-contaminated soil. Hum. Ecol. Risk Assessment: Int. J. 26, 571–585. doi: 10.1080/10807039.2018.1539639
Choub V., Ajuna H. B., Won S. J., Moon J. H., Choi S. I., Maung C. E., et al. (2021). Antifungal activity of Bacillus velezensis CE 100 against anthracnose disease (Colletotrichum gloeosporioides) and growth promotion of walnut (Juglans regia L.) trees. Int. J. Mol. Sci. 22, 10438. doi: 10.3390/ijms221910438
Chuljerm H., Deeudom M., Fucharoen S., Mazzacuva F., Hider R. C., Srichairatanakool S., et al. (2020). Characterization of two siderophores produced by Bacillus megaterium: A preliminary investigation into their potential as therapeutic agents. BBA-General Subj. 1864, 129670. doi: 10.1016/j.bbagen.2020.129670
De Fátima Dias Diniz G., Cota L. V., Figueiredo J. E., Aguiar F. M., da Silva D. D., de Paula Lana U. G., et al. (2022). Antifungal activity of bacterial strains from maize silks against Fusarium verticillioides. Arch. Microbiol. 204, 89. doi: 10.1007/s00203-021-02726-4
Ding H., Mo W., Yu S., Cheng H., Peng L., Liu Z. (2021). Whole genome sequence of Bacillus velezensis strain GUMT319: a potential biocontrol agent against tobacco black shank disease. Front. Microbiol. 12. doi: 10.3389/fmicb.2021.658113
Dong X., Tu C., Xie Z., Luo Y., Zhang L., Li Z. (2022). The genome of Bacillus velezensis SC60 provides evidence for its plant probiotic effects. Microorganisms 10, 767. doi: 10.3390/microorganisms10040767
Dunlap C. A., Kim S. J., Kwon S. W., Rooney A. P. (2016). Bacillus velezensis is not a later heterotypic synonym of Bacillus amyloliquefaciens; Bacillus methylotrophicus, Bacillus amyloliquefaciens subsp. plantarum and ‘Bacillus oryzicola’are later heterotypic synonyms of Bacillus velezensis based on phylogenomics. Int. J. Syst. Evol. Microbiol. 66, 1212–1217. doi: 10.1099/ijsem.0.000858
El-Saadony M. T., Desoky E. S. M., El-Tarabily K. A., AbuQamar S. F., Saad A. M. (2024). Exploiting the role of plant growth promoting rhizobacteria in reducing heavy metal toxicity of pepper (Capsicum annuum L.). Environ. Sci. pollut. Res. 2024, 1–20. doi: 10.1007/s11356-024-32874-1
Fan B., Wang C., Ding X., Zhu B., Song X., Borriss R. (2019a). AmyloWiki: an integrated database for Bacillus velezensis FZB42, the model strain for plant growth-promoting Bacilli. Database, baz071. doi: 10.1093/database/baz071
Fan B., Wang C., Song X., Ding X., Wu L., Wu H., et al. (2019b). Bacillus velezensis FZB42 in 2018: the gram-positive model strain for plant growth promotion and biocontrol. Front. Microbiol. 9. doi: 10.3389/fmicb.2019.01279
Flemming H. C., Wingender J., Szewzyk U., Steinberg P., Rice S. A., Kjelleberg S. (2016). Biofilms: an emergent form of bacterial life. Nat. Rev. Microbiol. 14, 563–575. doi: 10.1038/nrmicro.2016.94
Han X., Shen D., Xiong Q., Bao B., Zhang W., Dai T., et al. (2021). The plant-beneficial rhizobacterium Bacillus velezensis FZB42 controls the soybean pathogen Phytophthora sojae due to bacilysin production. Appl. Environ. Microbiol. 87, e01601–e01621. doi: 10.1128/AEM.01601-21
Hasan N., Khan I. U., Farzand A., Heng Z., Moosa A., Saleem M., et al. (2022). Bacillus altitudinis HNH7 and Bacillus velezensis HNH9 promote plant growth through upregulation of growth-promoting genes in upland cotton. J. Appl. Microbiol. 132, 3812–3824. doi: 10.1111/jam.15511
He P., Cui W., Peng L. (2022). Biocontrol efficacy of Bacillus velezensis HC-8 against powdery mildew of honeysuckle caused by Erysiphe lonicerae var. Lonicerae. Biol. Control 166, 104834. doi: 10.1016/j.biocontrol.2021.104834
Hong S., Kim T. Y., Won S. J., Moon J. H., Ajuna H. B., Kim K. Y., et al. (2022). Control of fungal diseases and fruit yield improvement of strawberry using Bacillus velezensis CE 100. Microorganisms 10, 365. doi: 10.3390/microorganisms10020365
Hu J., Chang R., Yuan Y., Li Z., Wang Y. (2022). Identification of key residues essential for the activation of plant immunity by subtilisin from Bacillus velezensis LJ02. Front. Microbiol. 13. doi: 10.3389/fmicb.2022.869596
Huang Z., Ruan S., Sun Y., Cheng X., Dai J., Gui P., et al. (2022). Bacterial inoculants improved the growth and nitrogen use efficiency of Pyrus betulifolia under nitrogen-limited conditions by affecting the native soil bacterial communities. Appl. Soil Ecol. 170, 104285. doi: 10.1016/j.apsoil.2021.104285
Ismail M. A., Amin M., Eid A., Hassan S., Mahgoub H., Lashin I., et al. (2021). Comparative Study between exogenously applied plant growth hormones versus metabolites of microbial endophytes as plant growth-promoting for Phaseolus vulgaris L. Cells 10, 1059. doi: 10.3390/cells10051059
Jang S., Choi S. K., Zhang H., Zhang S., Ryu C. M., Kloepper J. W. (2023). History of a model plant growth-promoting rhizobacterium, Bacillus velezensis GB03: from isolation to commercialization. Front. Plant Sci. 14. doi: 10.3389/fpls.2023.1279896
Jia S., Song C., Dong H., Yang X., Li X., Ji M., et al. (2023). Evaluation of efficacy and mechanism of Bacillus velezensis CB13 for controlling peanut stem rot caused by Sclerotium rolfsii. Front. Microbiol. 14. doi: 10.3389/fmicb.2023.1111965
Jin Y., Zhu H., Luo S., Yang W., Zhang L., Li S., et al. (2019). Role of maize root exudates in promotion of colonization of Bacillus velezensis strain S3-1 in rhizosphere soil and root tissue. Curr. Microbiol. 76, 855–862. doi: 10.1007/s00284-019-01699-4
Keshmirshekan A., de Souza Mesquita L. M., Ventura S. P. (2024). Biocontrol manufacturing and agricultural applications of Bacillus velezensis. Trends Biotechnol. 42, 986–1001. doi: 10.1016/j.tibtech.2024.02.003
Khan A. R., Mustafa A., Hyder S., Valipour M., Rizvi Z. F., Gondal A. S., et al. (2022). Bacillus spp. as bioagents: uses and application for sustainable agriculture. Biology 11, 1763. doi: 10.3390/biology11121763
Khan N., Ali S., Shahid M., Mustafa A., Sayyed R., Curá J. (2021). Insights into the interactions among roots, rhizosphere, and rhizobacteria for improving plant growth and tolerance to abiotic stresses: a review. Cells 10, 1551. doi: 10.3390/cells10061551
Kim M. J., Shim C. K., Park J. (2021). Control efficacy of Bacillus velezensis AFB2-2 against potato late blight caused by Phytophthora infestans in organic potato cultivation. Plant Pathol. J. 37, 580. doi: 10.5423/PPJ.FT.09.2021.0138
Kloepper J. W., Reddy M. S., Rodríguez-Kabana R., Kenney D. S., Kokalis-Burelle N., Martinez-Ochoa N., et al. (2004). Application for rhizobacteria in transplant production and yield enhancement. Acta Hortic. 2004, 217–230. doi: 10.17660/actahortic.2004.631.28
Li X., Sun M., Zhang L., Finlay R. D., Liu R., Lian B. (2022). Widespread bacterial responses and their mechanism of bacterial metallogenic detoxification under high concentrations of heavy metals. Ecotoxicol. Environ. Saf. 246, 114193. doi: 10.3390/agronomy14010012
Li B., Wan J., Sha J., Tian M., Wang M., Zhang X., et al. (2020). Genomics assisted functional characterization of Bacillus velezensis E as a biocontrol and growth promoting bacterium for lily. Microbiol. Res. 238. doi: 10.3389/fmicb.2022.976918
Lilai S. A., Kapinga F. A., Nene W. A., Mbasa W. V., Tibuhwa D. (2022). The efficacy of biofungicides on cashew wilt disease caused by Fusarium oxysporum. Eur. J. Plant Pathol. 163, 453–465. doi: 10.1007/s10658-022-02489-8
Liu H., Jiang J., An M., Li B., Xie Y., Xu C., et al. (2022). Bacillus velezensis SYL-3 suppresses Alternaria alternata and tobacco mosaic virus infecting Nicotiana tabacum by regulating the phyllosphere microbial community. Front. Microbiol. 13. doi: 10.3389/fmicb.2022.840318
Liu R., Li J., Zhang F., Zheng D., Chang Y., Xu L., et al. (2021). Biocontrol activity of Bacillus velezensis D4 against apple Valsa canker. Biol. Control 163, 104760. doi: 10.1016/j.biocontrol.2021.104760
Liu L., Zhao X., Huang Y., Ke L., Wang R., Qi G. (2020). Protecting tobacco plants from O3 injury by Bacillus velezensis with production of acetoin. Physiol. Plantarum 170, 158–171. doi: 10.1111/ppl.13120
Ma J., Liu H., Liu K., Wang C., Li Y., Hou Q., et al. (2017). Complete genome sequence of Bacillus velezensis GQJK49, a plant growth-promoting rhizobacterium with antifungal activity. Genome Announc 5, 10–1128. doi: 10.1128/genomeA.00922-17
Másmela-Mendoza J., Moreno-Velandia C. (2022). Bacillus velezensis supernatant mitigates tomato Fusarium wilt and affects the functional microbial structure in the rhizosphere in a concentration-dependent manner. Rhizosphere 21, 100475. doi: 10.1016/j.rhisph.2022.100475
Masmoudi F., Abdelmalek N., Tounsi S., Dunlap C. A., Trigui M. (2019). Abiotic stress resistance, plant growth promotion and antifungal potential of halotolerant bacteria from a Tunisian solar saltern. Microbiol Res. 229, 126331. doi: 10.1016/j.micres.2019.126331
Medhioub I., Cheffi M., Tounsi S., Triki M. (2022). Study of Bacillus velezensis OEE1 potentialities in the biocontrol against Erwinia amylovora, causal agent of fire blight disease of rosaceous plants. Biol. Control 167, 104842. doi: 10.1016/j.biocontrol.2022.104842
Moradi Pour M., Saberi Riseh R., Skorik Y. (2022). Sodium alginate–gelatin nanoformulations for encapsulation of Bacillus velezensis and their use for biological control of pistachio gummosis. Material 15, 2114. doi: 10.3390/ma15062114
Mustafa A., Naveed M., Saeed Q., Ashraf M., Hussain A., Abbas T., et al. (2019). Application potentials of plant growth promoting rhizobacteria and fungi as an alternative to conventional weed control methods. Sustain. Crop Product 1, 1–23. doi: 10.5772/intechopen.86339
Myo E. M., Than W., Win T., Khai A. (2021). Evaluation of the symbiotic performance of tomato plants inoculated with Bacillus velezensis NKG-2 and challenged with Fusarium wilt pathogen. Arch. Phytopathol. 54, 1391–1404. doi: 10.1038/nrmicro.2016.94
Nadeem S. M., Ahmad M., Tufail M. A., Asghar H. N., Nazli F., Zahir Z. (2021). Appraising the potential of EPS-producing rhizobacteria with ACC-deaminase activity to improve growth and physiology of maize under drought stress. Physiol. Plantarum 172, 463–476. doi: 10.1111/ppl.13212
Naseem S., Hussain A., Wang X., Iqbal Z., Mustafa A., Mumtaz M. Z., et al. (2022). Exopolysaccharide and siderophore production ability of Zn solubilizing bacterial strains improve growth, physiology and antioxidant status of maize and wheat. Pol. J. Environ. Stud. 31, 1223–1236. doi: 10.15244/pjoes/140563
Nwachukwu B. C., Ayangbenro A. S., Babalola O. (2021). Elucidating the rhizosphere associated bacteria for environmental sustainability. Agriculture 11, 75. doi: 10.3390/agriculture11010075
Ongena M., Jacques P. (2008). Bacillus lipopeptides: versatile weapons for plant disease biocontrol. Trends Microbiol. 16, 115–125. doi: 10.1016/j.tim.2007.12.009
Pacifico M. G., Eckstein B., Bettiol W. (2021). Screening of Bacillus for the development of bioprotectants for the control of Fusarium oxysporum f. sp. vasinfectum and meloidogye incognita. Biol. Control 164, 104764. doi: 10.1016/j.biocontrol.2021.104764
Peng G., Zhao X., Li Y., Wang R., Huang Y., Qi G. (2019). Engineering Bacillus velezensis with high production of acetoin primes strong induced systemic resistance in Arabidopsis thaliana. Microbiol. Res. 227, 126297. doi: 10.1016/j.micres.2019.126297
Rabbee M. F., Ali M. S., Choi J., Hwang B. S., Jeong S. C., Baek K. H. (2019). Bacillus velezensis: a valuable member of bioactive molecules within plant microbiomes. Molecules 24, 1046. doi: 10.3390/molecules24061046
Rabbee M. F., Islam N., Baek K. H. (2022). Biocontrol of citrus bacterial canker caused by Xanthomonas citri subsp. citri by Bacillus velezensis. Saudi J. Biol. Sci. 29, 2363–2371. doi: 10.1016/j.sjbs.2021.12.005
Ruiz-Garcia C., Bejar V., Martinez-Checa F., Llamas I., Quesada E. (2005). Bacillus velezensis sp. nov., a surfactan t-producing bacterium isolated from the river Vélez in Málaga, southern Spain. Int. J. Systematic Evolutionary Microbiol. 55, 191–195. doi: 10.1099/ijs.0.63310-0
Saravanan R., Nakkeeran S., Saranya N., Kavino M., Ragapriya V., Varanavasiappan S., et al. (2022). Biohardening of banana cv. karpooravalli (ABB; pisang awak) with Bacillus velezensis YEBBR6 promotes plant growth and reprograms the innate immune response against Fusarium oxysporum f. sp. cubense. Front. Sustain. Food Syst. 6. doi: 10.3389/fsufs.2022.845512
Shi Z., Hong W., Wang Q. (2022). Complete genome resource of Bacillus velezensis J17-4, an endophyte isolated from stem tissues of rice. Plant Dis. 106, 727–729. doi: 10.1094/PDIS-05-21-0996-A
Singh B. K., Trivedi P. (2017). Microbiome and the future for food and nutrient security. Microb. Biotechnol. 10, 50–53. doi: 10.1111/1751-7915.12592
Singh B. K., Trivedi P., Egidi E., Macdonald C. A., Delgado-Baquerizo M. (2021). Crop microbiome and sustainable agriculture. Nat. Rev. Microbiol. 19, 601. doi: 10.1038/s41579-020-00483-7
Su T., Shen B., Hu X., Teng Y., Weng P., Wu Z., et al. (2024). Research advance of Bacillus velezensis: bioinformatics, characteristics, and applications. Food Sci. Hum. Wellness 13, 1756–1766. doi: 10.26599/FSHW.2022.9250148
Sugiyama T., Natsuaki K. T., Tanaka N., Shiwa Y., Irie M. (2022). Antagonism of Bacillus velezensis isolate from anaerobically digested dairy slurry against Fusarium wilt of spinach. Agronomy 12, 1058. doi: 10.3390/agronomy12051058
Syed-Ab-Rahman S., Chua E., Schenk P. J. (2021). Characterisation and isolation of bioactive compounds of anti-oomycete bacterial isolates inhibiting the growth of Phytophthora capsici. Australasian. Plant Pathol. 50, 651–659. doi: 10.1007/s13313-021-00806-z
Tan Z., Clomburg J., Cheong S., Qian S., Gonzalez R. (2020). A polyketoacyl-CoA thiolase-dependent pathway for the synthesis of polyketide backbones. Nat. Catal 3, 593–603. doi: 10.1038/s41929-020-0471-8
Tian X., Zhao X., Zhao S., Zhao J., Mao Z. (2022). The biocontrol functions of Bacillus velezensis strain Bv-25 against Meloidogyne incognita. Front. Microbiol. 13. doi: 10.3389/fmicb.2022.843041
Toral L., Rodríguez M., Martínez-Checa F., Montaño A., Cortés-Delgado A., Smolinska A., et al. (2021). Identification of volatile organic compounds in extremophilic bacteria and their effective use in biocontrol of postharvest fungal phytopathogens. Front. Microbiol. 12. doi: 10.3389/fmicb.2023.1267324
Wang A., Hua J., Wang Y., Zhang G., Luo S. (2020). Stereoisomers of nonvolatile acetylbutanediol metabolites produced by Bacillus velezensis WRN031 improved root elongation of maize and rice. J. Agric. Food Chem. 68, 6308–6315. doi: 10.1021/acs.jafc.0c01352
Wang R., Wang C., Feng Q., Liou R., Lin Y. (2021). Biological inoculant of salt-tolerant bacteria for plant growth stimulation under different saline soil conditions. J. Microbiol. Biotechnol. 31, 398–407. doi: 10.4014/jmb.2009.09032
Wekesa T. B., Wekesa V. W., Onguso J. M., Wafula E. N., Kavesu N. (2022). Isolation and Characterization of Bacillus velezensis from Lake Bogoria as a Potential Biocontrol of Fusarium solani in Phaseolus vulgaris L. Bacteria 1, 279–293. doi: 10.3390/bacteria1040021
Xu P. D., Yi J. F., Chen D., Pan L., Xie B. Y., Zhao W. J. (2024). Research progress in the biocontrol secondary metabolites of bacillus velezensis. Biotechnol. Bull. 40, 75. doi: 10.13560/j.cnki.biotech.bull.1985.2023-0867
Xu P., Yi J., Chen D., Pan L., Xie B. Y., Zhao W. J. (2024). Research progress on biocontrol secondary metabolites of Bacillus velezensis. Biotechnol. Bull. 40, 75–88. doi: 10.13560/j.cnki.biotech.bull.1985.2023-0867
Ye M., Tang X., Yang R., Zhang H., Li F., Tao F., et al. (2018). Characteristics and application of a novel species of Bacillus: Bacillus velezensis. ACS Chem. Biol. 13, 500–505. doi: 10.1021/acschembio.7b00874
Yin X., Li T., Jiang X., Tang X., Zhang J., Yuan L., et al. (2022). Suppression of grape white rot caused by Coniella vitis using the potential biocontrol agent Bacillus Velezensis GSBZ09. Pathogens 11, 248. doi: 10.3390/pathogens11020248
Zhang H., Chen J., Liu Y., Xu Q., Inam M., He C., et al. (2022). Discovery of a novel antibacterial protein CB6-C to target methicillin-resistant Staphylococcus aureus. Microb. Cell Fact 21, 4. doi: 10.1186/s12934-021-01726-9
Zhang C. W., Cheng K., Zhang X., Liu B., Du H. B., Yao S. (2019). Taxonomy and functions of Bacillus velezensis: a review. Food Fermentation Industries 45, 258–265. doi: 10.13995/j.cnki.11-1802/ts.020737
Zhang D. F., Gao Y. X., Wang Y. J., Du H. B., Yao L. (2020). Advances in classification, antagonistic function and application of Bacillus velezensis. Microbiol. Bull. 47, 3634–3649. doi: 10.13344/j.microbiol.China.190947
Zhang P., Xie G., Wang L., Xing Y. (2022). Bacillus velezensis BY6 promotes growth of poplar and improves resistance contributing to the biocontrol of Armillaria solidipes. Microorganisms 10, 2472. doi: 10.3390/microorganisms10122472
Keywords: B. velezensis, disease resistance, promoting effect, mechanism, application
Citation: Zhong X, Jin Y, Ren H, Hong T, Zheng J, Fan W, Hong J, Chen Z, Wang A, Lu H, Zhong K and Huang G (2024) Research progress of Bacillus velezensis in plant disease resistance and growth promotion. Front. Ind. Microbiol. 2:1442980. doi: 10.3389/finmi.2024.1442980
Received: 03 June 2024; Accepted: 17 September 2024;
Published: 03 October 2024.
Edited by:
Marika Pellegrini, University of L’Aquila, ItalyReviewed by:
Shrivardhan Dheeman, MVN University, IndiaVlad Stoian, University of Agricultural Sciences and Veterinary Medicine of Cluj-Napoca, Romania
Copyright © 2024 Zhong, Jin, Ren, Hong, Zheng, Fan, Hong, Chen, Wang, Lu, Zhong and Huang. This is an open-access article distributed under the terms of the Creative Commons Attribution License (CC BY). The use, distribution or reproduction in other forums is permitted, provided the original author(s) and the copyright owner(s) are credited and that the original publication in this journal is cited, in accordance with accepted academic practice. No use, distribution or reproduction is permitted which does not comply with these terms.
*Correspondence: Guidong Huang, guidongh78@126.com
†These authors have contributed equally to this work