- 1Instituto de Biotecnología, Universidad Nacional de San Juan (U.N.S.J.), San Juan, Argentina
- 2Consejo Nacional de Investigaciones Científicas y Tecnológicas (CONICET), Buenos Aires, Argentina
The food industry generates substantial amounts of organic waste often underutilized within the system. Craft beer production, experiencing global rapid expansion, contributes to this waste stream with byproducts such as spent grain, trub, and yeast. Many craft beer industries discharge yeast residue directly into public water bodies. In recent years, yeasts have garnered attention for their potential to enhance plant growth and contribute to sustainable agriculture. This study focuses on characterizing Saccharomyces cerevisiae yeast collected at the end of the craft beer fermentation process. Biomass characterization was conducted, and the yeast’s effect on lettuce and tomato seeds and seedlings was evaluated at four concentrations (105, 106, 107, and 108 cells mL−1) in sterile substrate. After 28 days, plant height, leaf number, fresh and dry weights of both aboveground and root parts, as well as chlorophyll content, were analyzed. The most effective concentration (107 cells mL−1) was applied to tomato seedlings in sterile substrate, compared with a commercial organic fertilizer. After 21 days, growth parameters were assessed. The study demonstrated that increasing yeast doses up to 108 cells mL−1 positively affects seed germination and seedling development. Notably, a dose of 107 cells mL−1 proved effective for application in seedlings as an organic amendment and substitute for commercial products. This integrated approach showcases the potential of yeasts in sustainable agriculture, utilizing byproducts from the food industry to enhance crop performance and mitigate environmental pollution.
1 Introduction
Currently, it is undeniable that the food processing industry has a detrimental impact on the planet’s sustainability due to the substantial volume of waste it generates (Khedkar and Singh, 2018). Particularly, the craft brewing industry has seen a substantial production increase in recent years, playing a significant role in this issue. In the bioprocessing of crafting this beverage, raw materials such as barley, water, hops, and yeast are utilized. Regardless of the type of beer produced, the waste generated from these raw materials is categorized as food-grade waste, standing out for its rich composition in organically valuable compounds and mineral content. This makes it suitable for direct use as animal and human feed, as well as promoters of growth and plant health in agriculture (Thomas and Rahman, 2006; dos Santos et al., 2014). Examples of such utilization include the use of beer bagasse for sustainable food packaging, the production of bioplastics and the generation of biogas (Castro-Criado et al., 2023; Qazanfarzadeh et al., 2023; Tschoeke et al., 2023).
During the fermentation stage, the employed brewing yeast, primarily Saccharomyces cerevisiae, tends to multiply three to five times, especially in the early hours when oxygen is supplied to the wort (Briggs, 2004). Generally, the biomass produced is approximately 1.5 to 3 kg hl−1 of beer (Ferreira et al., 2010; Olajire, 2020), and at the end of the process, this biomass is primarily discharged into sewage (Kerby and Vriesekoop, 2017). This discharge can potentially lead to severe pollution issues for bodies of water as it contains organic compounds that require oxygen for degradation (Simate et al., 2011). In a context where environmental regulations are becoming more stringent and the cost of water is steadily increasing, preserving this resource is of utmost importance (Shannon et al., 2008; Sgroi et al., 2018). Simultaneously, there is a growing interest in the valorization of waste generated by industries (Lin et al., 2014; Galanakis, 2020).
In recent years, yeasts have gained relevance in agriculture, playing a crucial role in crop development, being termed “plant growth-promoting yeasts” (PGPY) (Nimsi et al., 2023). These microorganisms can be employed as bio-stimulants or bio-fertilizers, offering benefits by modulating the production of phytohormones, improving soil quality, increasing nutrient availability, enhancing resistance to abiotic stress and inhibiting the proliferation of phytopathogens (Nutaratat et al., 2014; Pandi et al., 2019; Fernandez-San Millan et al., 2020; Radić et al., 2022). Integration of PGPY into agricultural practices could be achieved through three primary methods: seed inoculation, foliar spraying and soil incorporation (EL-Ghamring et al., 1999; Nimsi et al., 2023).
In general, lysed yeasts are a rich source of organic and inorganic nutrients (Bekatorou et al., 2006). On the other hand, under non-lysed (metabolically active) conditions, they can synthesize substances and phytohormones necessary for plant growth (Boraste et al., 2009). Additionally, various studies have reported that some yeast strains exhibit positive properties as bio-fertilizers, including phosphate and zinc solubilization (Hesham and Mohamed, 2011; Amprayn et al., 2012), stress resistance in plants (El-Zohri et al., 2017), and pathogen control (Droby et al., 2002; Coelho et al., 2007; Pimenta et al., 2010; Kamal and Komatsu, 2016; Ibrahim and El-FikiI, 2019). Particularly, S. cerevisiae is characterized by its richness in proteins, carbohydrates, nucleic acids, lipids, and minerals (N, P, K, Na, Fe, Mg, S, Zn, Mn, Cu, Si, Cr, Ni, Va and Li), as well as thiamine, riboflavin, pyridoxine, hormones (such as auxins and cytokinins), and other growth-regulating substances, biotin, B12, and folic acid (Nagodawithana, 1991; Tartoura, 2001; Medani and Ragab, 2015). Due to these chemical and bioactive properties and being safe for humans (Generally Recognized as Safe - GRAS) (Poloni et al., 2017) and the environment, S. cerevisiae has emerged in recent decades as a positive alternative to chemical fertilizers (Hernández-Fernández et al., 2021).
In the field of agriculture, it is widely acknowledged that the indiscriminate use of chemical fertilizers significantly harms the environment and has detrimental effects on living organisms and soil health (Sujanya and Chandra, 2011). Despite the known benefits of Saccharomyces cerevisiae as a biofertilizer, the potential of its residue from the craft brewing industry has been largely overlooked in the existing body of knowledge. Therefore, our research seeks to fill this gap by investigating the mechanisms of action of this residue as a biofertilizer as well as its application strategy in order to provide a scientific basis for its use. To date, only Lonhienne et al. (2014) have assessed residual brewer’s yeast as a biofertilizer. However, their process involves preliminary treatments to prepare the residue. Our research aims to depart from this approach by seeking to generate a product with minimal intervention, thereby enhancing its feasibility and sustainability.
Based on the aforementioned, the revalorization of yeast residue for use as a bioinoculants in agriculture would not only contribute to reducing pollution generated by the brewing industry, but also presents a secure alternative, potentially minimizing the reliance on chemical fertilizers. Therefore, to promote the use of this residue, it is necessary to carry out a series of steps that lead to obtaining a product that is safe for plants and soil and nutritionally efficient which serves as the motivation behind the present study.
2 Materials and methods
2.1 Harvesting and preparation of yeast biomass
The residue of S. cerevisiae yeasts generated at the end of the fermentation process was employed to assess its potential as a Plant Growth-Promoting Yeast (PGPY). This yeast biomass was obtained from an ale-style beer with 20 IBU and 5.5% (v/v) alcohol. Samples were collected under sterile conditions and transported to the IBT-UNSJ (Institute of Biotechnology-National University of San Juan) laboratory under refrigeration. A triple rinsing of the yeast biomass was carried out using sterile deionized water at pH 7 to remove excess iso-α-acids from the hops and alcohol adhered by the yeast cell walls during the process. In 225 mL cylindrical-conical tubes with 150 mL of deionized water, a concentration of yeast biomass at 109 cells mL−1 were placed and subjected to centrifugation for 10 min at 5590 g, discarding the supernatant after each centrifugation cycle (Agamy et al., 2012). The washed samples were kept at 4°C under viable conditions.
2.2 Characterization of the collected biomass
The pH of the yeast sample was measured using a multiparameter benchtop pH meter (Ad1030, Adwa®, Hungary). To assess yeast cell viability, a modified version of the methylene blue staining technique proposed by White and Zainasheff (2010) was employed. The total organic matter (polysaccharides, proteins and fibers) and NPK (nitrogen, phosphorus and potassium) content were determined in washed yeast samples using the methods proposed by Bradstreet (1954); Heldrich (1990) and Walkley and Black (1934).
2.2.1 Evaluation of yeast biomass characteristics as a biofertilizer
2.2.1.1 Phosphate and zinc solubilization
A 20 µL aliquot, containing a population of washed yeast cells of 107 cells mL−1, was inoculated onto Pikovskaya agar following the methodology outlined by Zaidi et al. (2006), and zinc oxide agar as per Rokhbakhsh-Zamin et al. (2011). The plates were then incubated at 28 ± 1°C for a period of 5 days. The presence of a halo surrounding the colonies serves as an indicator of phosphate and zinc solubilization, respectively. Additionally, the SE (solubilization efficacy) value was determined as the diameter of the colony plus precipitation zone diameter to ratio of the colony (Fernandez-San Millan et al., 2020).
2.2.1.2 AIA production from yeast biomass
To assess the production of indole-3-acetic acid (IAA), a yeast population of 107 cells mL−1 was cultured in YPD broth tubes (1% yeast extract, 2% peptone, and 2% (w/v) dextrose), both with and without the addition of 0.1% (w/v) L- tryptophan (L-Trp), in a shaker at 28°C with agitation set at 3 g for 5 days. Following incubation, cells were centrifuged at 3153 g for 5 min. A 0.5 mL aliquot of the resulting supernatant was mixed with an equal volume of Salkowski’s reagent (2 mL of 0.5M iron(III) chloride and 98 mL of 35% perchloric acid). The mixture was then incubated in darkness for 30 min, and the developed red coloration was quantified at 530 nm using a spectrophotometer (Biotraza® 721 model, China) (Gordon and Weber, 1951). For quantification, a calibration curve (0-50 µg mL−1) was established using pure IAA. Yeast samples grown in YPD with and without L-Trp were utilized for the germination and phytotoxicity assays (Sun et al., 2014).
2.2.1.3 Germination rate and phytotoxicity
Lettuce sativa seeds (var. Sagges) were subjected to sterilization with 3% sodium hypochlorite for 1 min, followed by three rinses with sterile deionized water. Fifty seeds were then placed on germination trays with moist filter paper and 6 treatments were carried out. Each seed was inoculated with 10 µl of a yeast population (cell mL−1) of 105, 106, 107, 108 and two controls were conducted - one with 50 without application and the other with 50 non-sterilized seeds (SWA and SWD respectively). The trays were incubated under a photoperiod of 14 h light and 10 h dark at 25 ± 1°C. The germination percentage was determined after 24 h, considering germination successful when the radicles exceeded 2 mm in length (Fernandez-San Millan et al., 2020). After 4 days, root length, shoot length, and fresh weight of the seedlings were determined. Simultaneously, plates with Murashige-Skoog medium with vitamins solidified with 0.5% plant agar (M519 and A296 plant agar, Phytotechnology Laboratories, USA) were prepared. Three trials were conducted, each replicated three times: a) five seeds submerged in YPD + L-Trp supernatant from the previous assay; b) five seeds submerged in YPD without L-Trp supernatant; c) five seeds submerged in sterile tap water (control). The plates were incubated under a photoperiod of 14 h light and 10 h dark at 25 ± 1°C, and monitoring was conducted for 5 days to determine the germination rate and visual assessment of the emerging seedlings.
2.3 In vivo evaluation in lettuce and tomato seedlings on a laboratory scale
In the initial phase, the application of increasing doses of yeast-based fertilizer on 20-day-old lettuce seedlings (var. Sagges) was evaluated. Each seedling was transplanted into a 500 mL pot containing 150 g of sterile substrate (a mixture of peat and perlite 3:1). There were four treatments with increasing doses of yeast-based fertilizer (cell mL−1): 5 x 105 (T1), 5 x 106 (T2), 5 x 107 (T3), 5 x 108 (T4) and one control treatment (TC) without yeast biomass application. Each treatment consisted of eight seedlings. Based on the dose that yielded the best results (measured by an increase in the number of leaves, height, and fresh and dry weight of both the above-ground and root parts), a second trial was conducted with 30-day-old tomato seedlings (Solanum sect. Lycopersicon UCO 15 INTA) Similarly to the lettuce trial, each seedling was transplanted into a 500 mL pot with 150 g of sterile substrate. Two treatments were established: one with the dose of yeast-based fertilizer that presented the best results (Tomato treatment 1-Tt1) and the other with a dose of 75 mL L−1 of organic fertilizer Hampi (SAPHU SRL- Argentina) (Tt2), in addition to a control treatment with no fertilizer application (TtC). Each treatment consisted of 9 seedlings. Yeast and commercial fertilizer inoculation were done when the first roots emerged (one application of 30 mL). Starting from this point (day 0), measurements of plant height and the number of leaves were taken every seven days to assess the effect of inoculation on plant growth. The plants were kept at 25 ± 1°C under a photoperiod of 14 h light and 10 h dark. Watering was done periodically with tap water (EC 561 μS and pH 7.21). When symptoms of stress were observed in some seedlings, the trial was terminated. At this moment the chlorophyll content was measured using a chlorophyll meter (SPAD-502 Plus, Minolta®, Japon). For each seedling, an average chlorophyll value was obtained from three leaves (one apical, one intermediate, and one basal), excluding wilted leaves. The seedlings were then removed, the above-ground and root parts were separated and washed with tap water, and the fresh weight of each part was determined. Additionally, the root length was measured. Finally, the above-ground and root parts were individually dried in an oven at 60°C until a constant weight was achieved to obtain the dry weight of each part.
2.4 Statistical analysis
The data obtained in the trial were statistically processed by t-tests and one-way ANOVA with Fisher pairwise comparison as a post hoc test for multiple comparisons (p<0.05). In order to simplify the interpretation of parameters measured on lettuce seedlings, principal components analysis (PCA) was applied. The results represented the mean value of the determinations with their respective standard deviations ( ± SD). The software employed was INFOSTAT.
3 Results
3.1 Characterization of yeast biomass
Table 1 displays the results obtained through the characterization of the collected and washed yeast biomass. The recorded pH was 4.2, with a viability of 49.7% at the time of harvest. Organic matter in the biomass exhibited values of 13.06, with NPK values of 5.38, 0.21, and 0.50% (w/w), respectively.
Figure 1 illustrates the phosphate and zinc solubilization activity by the evaluated yeasts, SE solubilization of PO4 3.34 and Zn 2.58. Additionally, catalase activity also yielded positive results under exposure to 3% H2O2. These results are summarized in Table 1.
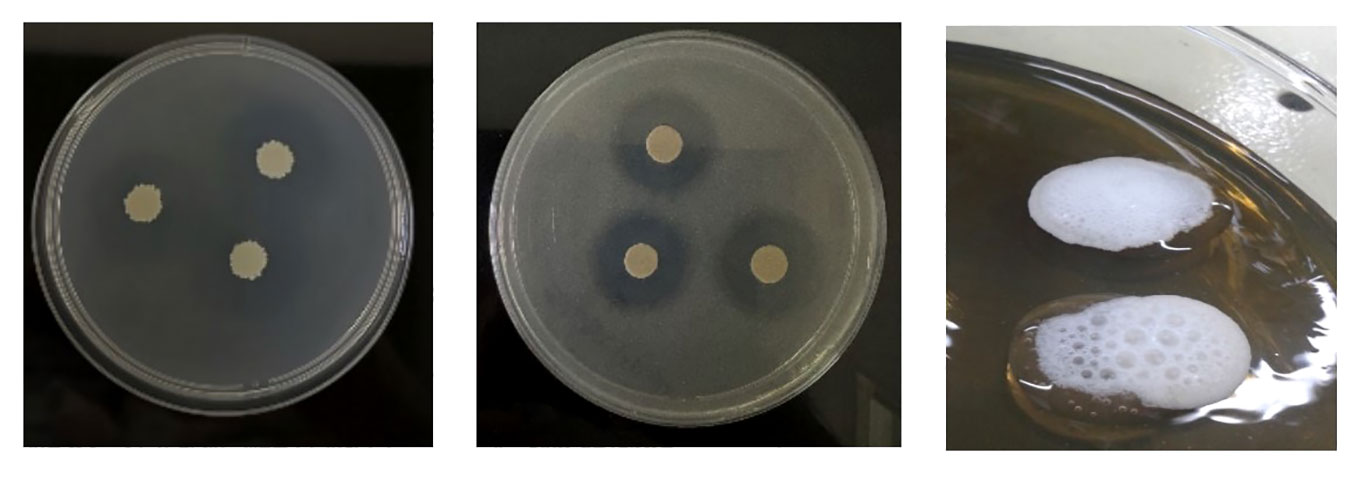
Figure 1 Plates with solubilization assays and catalase activity from left to right. (A) Phosphate solubilization on Pikovskaya agar medium, (B) zinc solubilization on Zn agar medium, (C) catalase activity on YPD medium.
Based on the results obtained from the colorimetric assay using the Salkowski reagent, yeast grown in the presence of Trp produced AIA (Indole-3-acetic acid) concentrations of 10.89 µg mL−1, compared to 6.55 µg mL−1 when grown in L-Trp-free YPD (Table 1).
3.2 Germination rate and phytotoxicity
When evaluating the germination rate, it was found that seeds inoculated with a biomass of 107 and 108 cell mL−1 showed the highest germination percentages (94% and 90%, respectively), while the lowest germination (68%) was observed in non-sterilized seeds (SWD). Treatments with 106 y 105 cell mL−1 exhibited germination percentages of 82 and 80% respectively, and seeds without application of yeast biomass (SWA) had 76%. Cotyledon expansion was recorded at 24 h post-germination, 87.2 and 86.6% was registered with inoculation of 107 and 108 cell mL−1. On the other hand, the lowest expansion levels were recorded on the SWD and 105 cell mL−1 treatments. Principal component analysis (PCA) was performed in order to characterize the attributes (root and shoot length and fresh weight) of the seedling (Figure 2). PC1 explains 66.6% of the total variability, while PC2 explains 27.7%. The attributes that best described the first component (with their respective eigenvector values indicated in parenthesis) were fresh weight (0.64) and root length (-0.39). These attributes allowed a clear separation between treatments inoculated with a population of 106,107 and 108 cell mL−1 and the rest of the treatments. Fresh weight and shoot length were linked to the highest doses inoculated, whereas the root length exhibited correlation with the SWA and 105 cell mL−1 treatments.
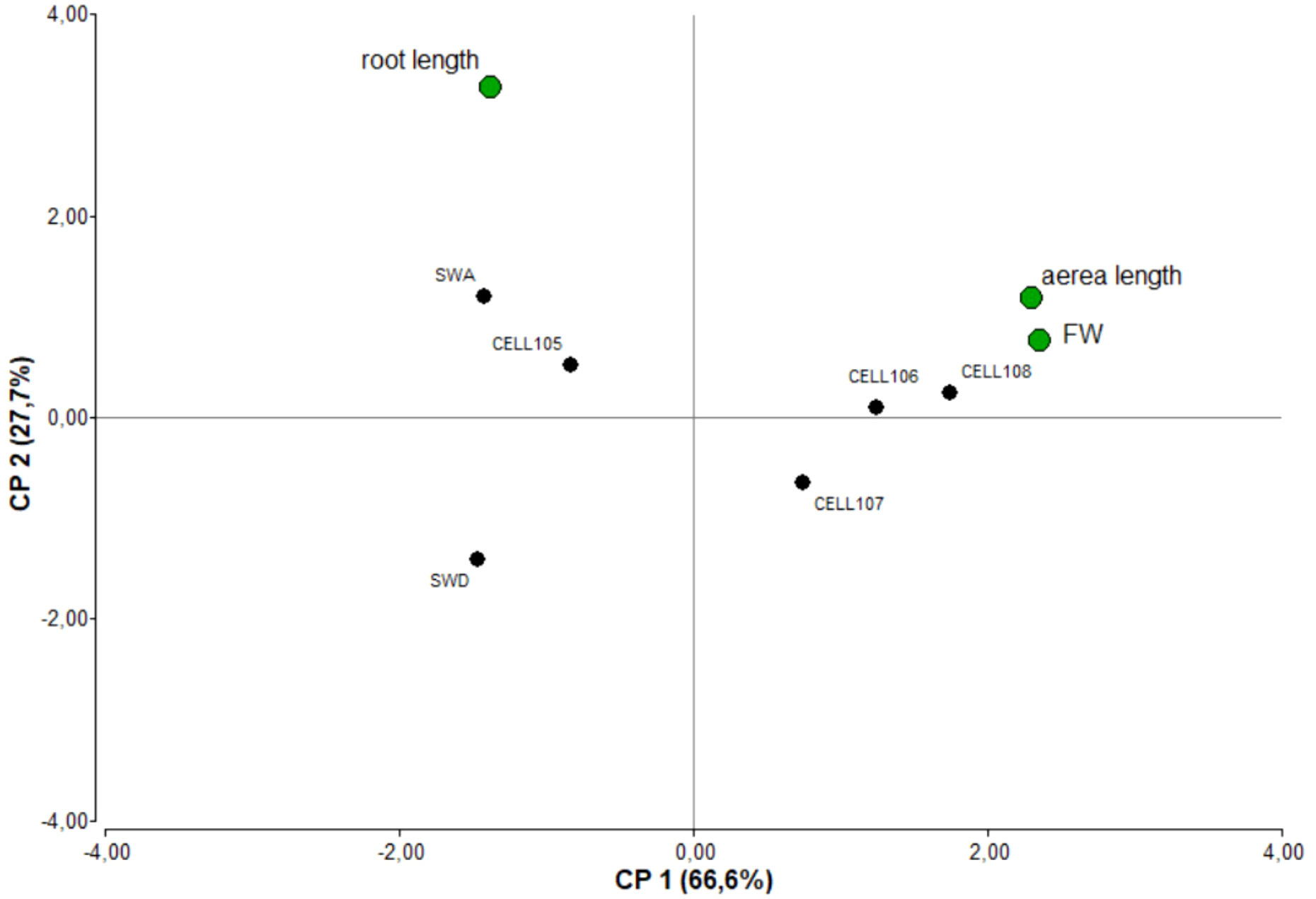
Figure 2 Principal component analysis of root and shoot length and fresh weight for seed treatments: 105, 106, 107 and 108 cells mL −1 and controls (SWA and SWD).
Regarding the architecture of the germinated seedlings evaluated on MS agar medium, seeds treated with YPD supernatant with and without L-Trp experienced a delay in the germination process of approximately 12 h compared to seeds from the untreated control. Although the resulting seedlings exhibited a smaller caliber, cotyledon emergence was recorded at 48 h of incubation, 12 h earlier than seeds from the control treatments. Despite the reduced length of roots in inoculated seeds, a greater development of absorbent hairs was noted, particularly in those treated with YPD + L-Trp supernatant (Figure 3).
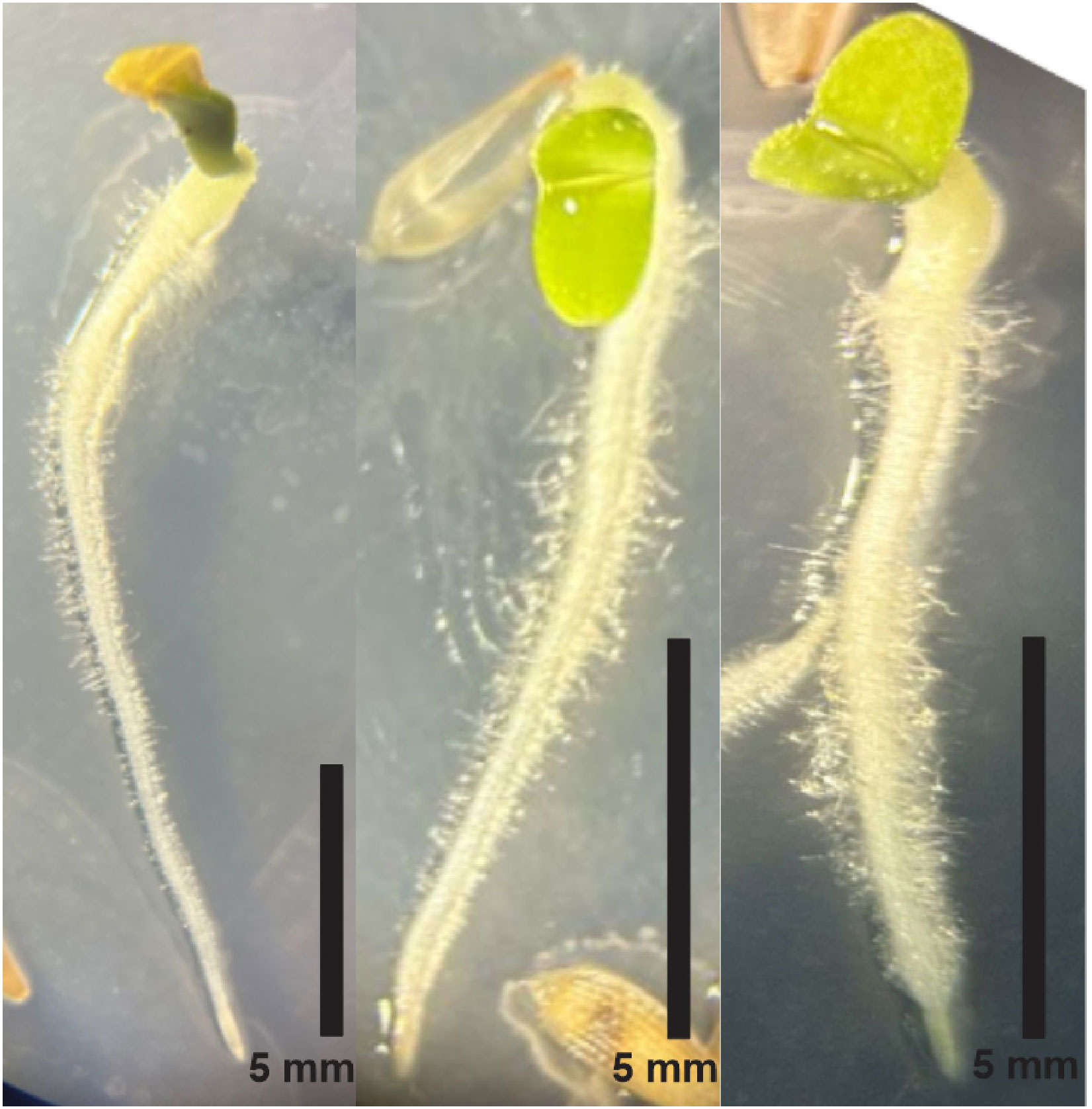
Figure 3 Lettuce seedlings treated from left to right (A) control treatment, (B) YPD supernatant; (C) YPD supernatant + L-Trp.
3.3 Lettuce in sterile substrate
At the beginning of the experiment, the plants displayed no significant differences in height, although the number of leaves per seedling varied. Throughout the evaluated periods, it was observed that while T3 showed higher mean height values (p<0.05) on days 14 and 21, there were no significant height differences among inoculated treatments by the end of the trial. On the other hand, TC with an average of 17.88 ± 1.06 cm, recorded the lowest height value (Table 2). Although T3 initially presented the lowest mean value of leaf number, the final average was significantly higher by day 28, revealing an increase over the 28-day period of 7.88 ± 0.83 leaves. Conversely, T4 experienced the least growth in height and number of leaves during the initial 2 weeks, with its mean values trailing behind compared to the other treatments (Table 2). However, this treatment displayed the most significant growth during the last two weeks of the trial, aligning its height mean with the other treatments and matching the number of leaves with T2 and TC.
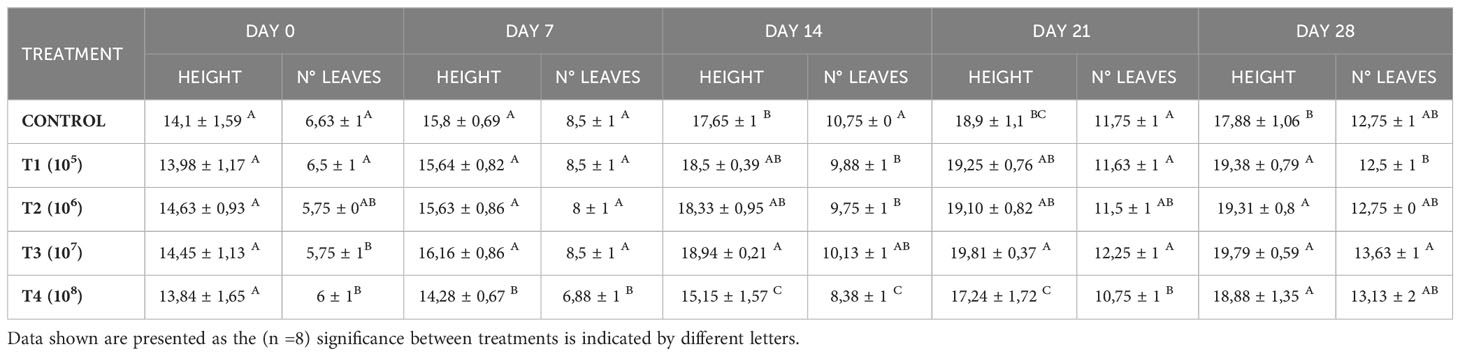
Table 2 Weekly effects of different doses of yeast residue inoculation in height and number of leaves in lettuce seedlings during the 28-day trial.
In Table 3, it can be observed that T3 also exhibited a higher fresh and dry weight of the above-ground part, consistent with the significant increase in the number of leaves recorded for this treatment (Table 2). Additionally, the chlorophyll content is higher for T3 (p<0.05).
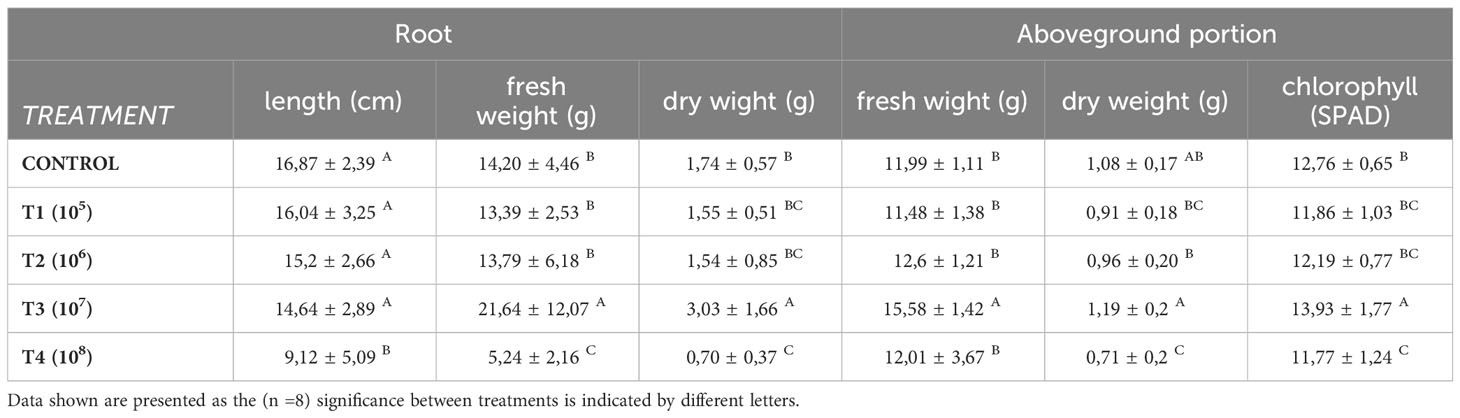
Table 3 Influence in the root and aboveground portion of different doses of yeast residue inoculation in lettuce plants.
Based on the data obtained for the parameters evaluated in roots (Table 3), it can be observed that the lowest values (p<0.05) of length, fresh weight, and dry weight were recorded in T4 plants, as evidenced in Figure 4. Conversely, the dry and fresh weight of the roots were higher for T3 plants, even though the root length showed no significant differences between the rest of the treatments.
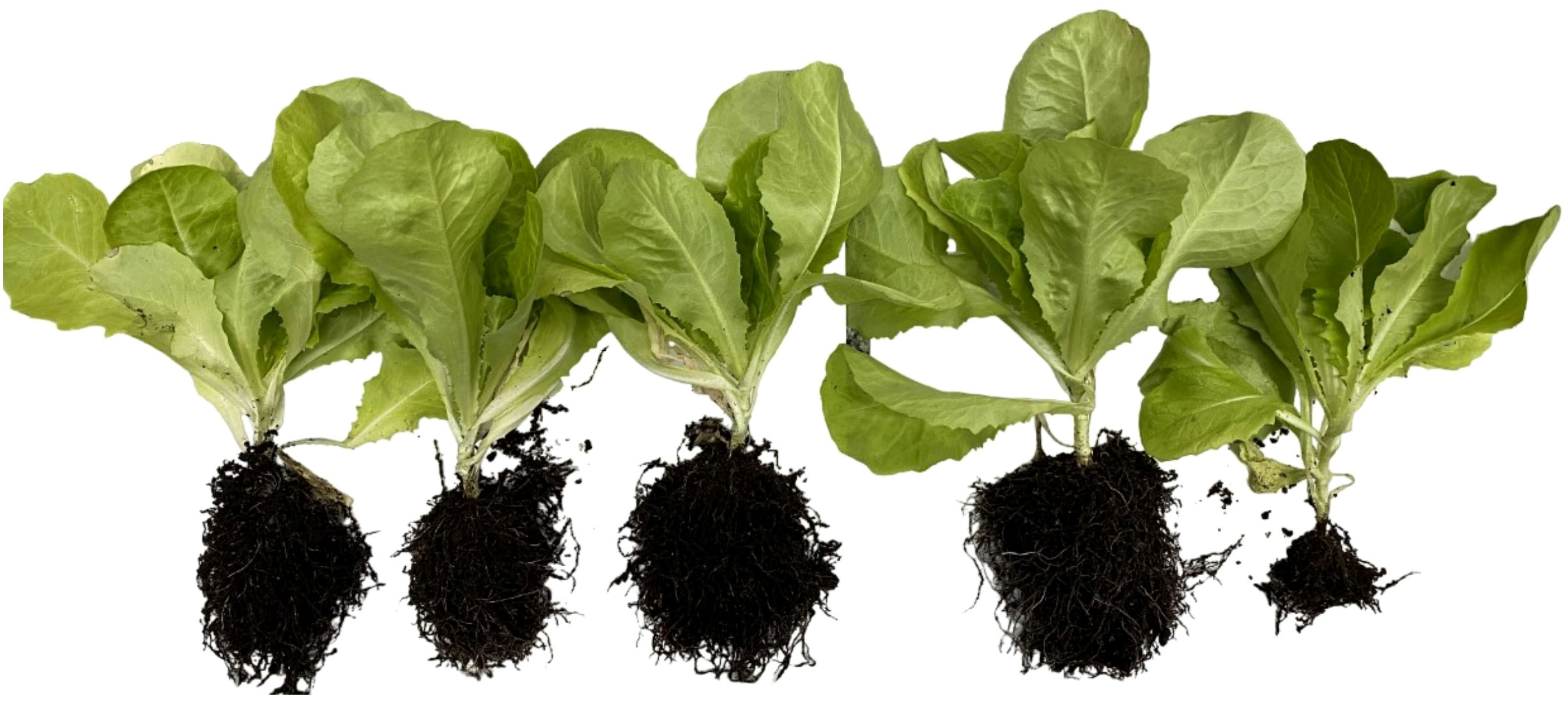
Figure 4 Effect of biofertilizer on lettuce growth at the end of the 28-day trial, from left to right (A) control treatment-TC, (B) 5 x 105 cells mL −1 -T1; (C) 5 x 106 cells mL −1 -T2; (D) 5 x 107 cells mL −1 -T3; (E) 5 x 108 cells mL −1 -T4.
3.4 Tomato in sterile substrate
From Figure 5A, it can be observed that at the beginning of the trial, there were no significant differences in the number of leaves, although the height of the plants varied. At the end of the treatment, the average height of Tt1 was significantly greater compared to Tt2 and TtC. Even though Tt2 started with a lower average height than Tt1 and TtC, both of which had equal initial values (p<0.05), the height variation over the 21-day period showed no significant differences between the treatments (Tt1:8.28 ± 2.05; Tt2:8.28 ± 1.69 and TtC: 7.87 ± 2.40). On the other hand, the yeast-treated plants (Tt1) exhibited the highest mean value (p<0.05) of number of leaves over the three weeks of the trial (Figures 5B, C), with a rise in the leaf count over the 21-day period of 1.71 ± 0.76 leaves.
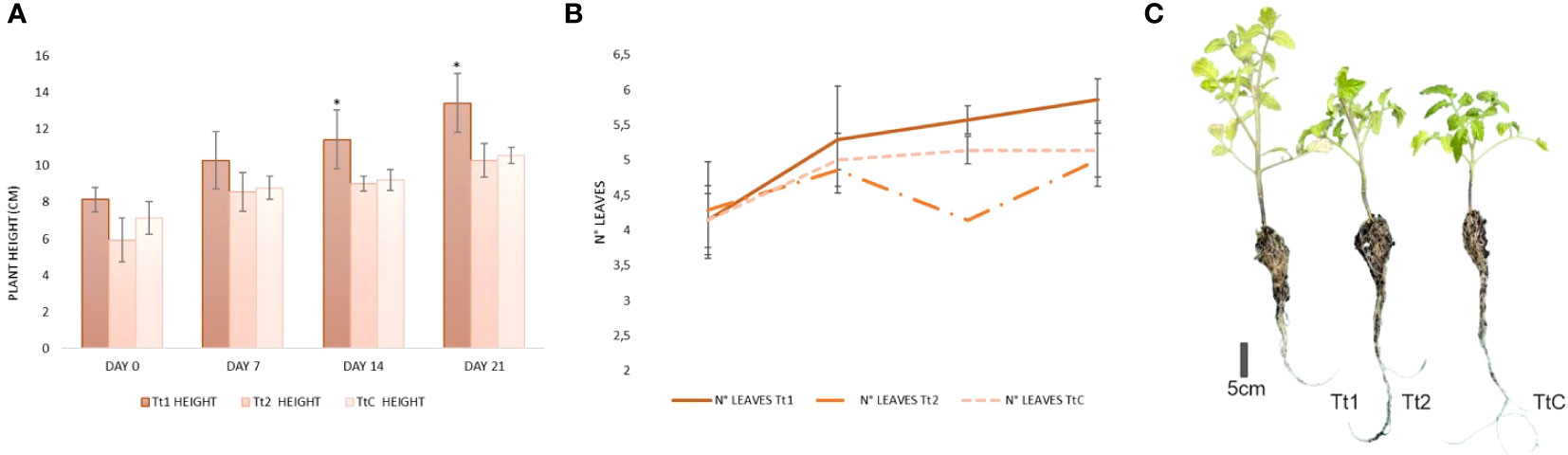
Figure 5 (A) Comparison of tomato height growth between the yeast biofertilizer and commercial fertilizer (*indicates significant difference) (B) Comparison of tomato number of leaves growth between the yeast biofertilizer and commercial fertilizer. (C) Effects of plant growth-promoting yeasts on tomato development after 21 days of in vivo trials (Tt1: 107 cel mL −1; Tt2: organic commercial fertilizer and TtC: tomato without treatment).
Table 4 shows that Tt1 presented the highest values (p<0.05) for fresh and dry weight in the above-ground part, aligning with the recorded values of higher leaf count reflected in Figure 5B. Nevertheless, both fertilized treatments (Tt1 and Tt2) showed chlorophyll values with no significant differences. Meanwhile, in TtC the lowest chlorophyll SPAD value was recorded (31.66 ± 1.23). On the other hand, it was noted that unfertilized plants (TtC) had longer root lengths (p<0.05) compared to fertilized plants (Tt1 and Tt2) (Figure 5C). However, Tt2 plants exhibited significantly higher fresh and dry weight of the roots, while TtC showed the lowest values (p<0.05).
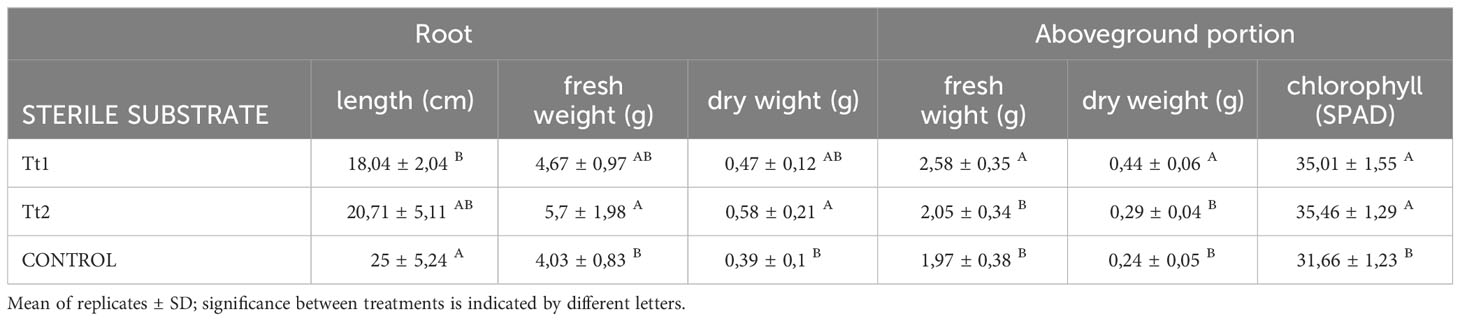
Table 4 Influence in the root and aboveground portion of yeast biofertilizer and commercial fertilizer in tomato plants.
4 Discussion
In recent years, the application of microorganisms as promoters of plant growth has gained significance in the pursuit of sustainable agriculture (Mahanty et al., 2017; Mahapatra et al., 2022). Biofertilizers commonly used across different continents typically involve bacteria and filamentous fungi (Mącik et al., 2020), with limited information available on yeasts as bioinoculant agents (Fu et al., 2016; De Souza et al., 2019). Given the extensive genetic diversity within this microbial group, comprehensive studies are crucial to ascertain their potential as Plant Growth-Promoting Yeasts (PGPY). Saccharomyces cerevisiae, in particular, plays a prominent role in various biotechnological processes (Parapouli et al., 2020). This yeast specie has the ability to modulate plant growth and development through the biological synthesis of various components, as well as its contribution of macro and micronutrients, organic matter, carbohydrates, organic acids, and vitamins (Bekatorou et al., 2006; El-Motty et al., 2010; El-Yazied and Mady, 2012). Additionally, it has the potential to increase soil porosity and improve moisture content (Xi et al., 2019).
Various inoculation methods for PGPY exist, such as seed, root, soil, and foliar inoculation (Nimsi et al., 2023). Regarding seed application, it involves applying a known dose of microbial biomass directly onto the seeds through spraying or immersion. In the present study, when assessing the effect of the direct application of growing doses of yeast biomass on seeds, it was observed that higher doses (107 and 108 cell mL−1) might be linked to a greater germination and cotyledon expansion percentage, as well as enhanced shoot length and fresh weight (p<0,05). This suggests that the direct application of these doses on seeds may be advisable to promote germination and plant growth. However, it is necessary to explore different seed application strategies, such as inoculation rate, formulation matrix, among others. It’s worth noting that the yeast residue at these doses has shown non-phytotoxic properties in lettuce seeds, emphasizing its potential as a safe and effective bioinoculant for seed treatment.
A study reported by Lonhienne et al. (2014) indicates that both live and dead yeast can enhance plant growth and development. The viability assessment on the yeast biomass in the present study was 49.7%, indicating the presence of a portion of viable or metabolically active biomass. Under these conditions, S. cerevisiae can increase nutrient availability, synthesize substances, and phytohormones necessary for plant growth (Boraste et al., 2009).
The ability of microorganisms to solubilize phosphorus and zinc contributes to better nutrient absorption by the plant (Saravanan et al., 2011; Rawat et al., 2020). In this study, it was determined that S. cerevisiae has solubilizing capacity, showcasing its potential as a growth promoter. The phosphorus solubilization efficiency value was 3.34, higher than those recorded by Fu et al. (2016), where the inoculum of S. cerevisiae was adjusted to an optical density of 0.10 at 600 nm. Conversely, the zinc solubilization efficiency (SE=2.58) was high but lower than those recorded in various strains of S. cerevisiae (108 cell mL−1) by Fernandez-San Millan et al., 2020. It is important to note that the solubilization of these compounds is associated with factors such as substrate concentration, medium pH, and temperature (Xiao et al., 2013; Nutaratat et al., 2014; Kuo et al., 2018). Therefore, it is advisable to take into account these potential discrepancies when comparing and analyzing reports on the solubilization of the compounds in question. Thus, further research on the variability of these determining factors is necessary.
A growing number of studies indicate that plant root growth may be directly or indirectly enhanced by yeasts in the rhizosphere (Medina et al., 2004; Nassar et al., 2005; El-Tarabily and Sivasithamparam, 2006; Cloete et al., 2009). Ditengou et al. (2008) and Laskowski et al. (2008) demonstrated the fundamental role of IAA in stimulating lateral root elongation. The production of this plant hormone by S. cerevisiae can occur using tryptophan as a biochemical precursor or independently of this amino acid (Rao et al., 2010; Fu et al., 2015). The values obtained in this study for S. cerevisiae in the presence and absence of L-Trp were 10.89 and 6.55 µg mL−1, respectively. This suggests that the L-Trp-dependent pathway is the mechanism through which the brewing yeast residue synthesizes a greater amount of indoleacetic acid. These results align with reports by Fu et al. (2016) and Fernandez-San Millan et al. (2020). It is important to note that the production of this phytohormone by yeasts exhibits interspecific variability and is dependent on environmental factors, as demonstrated by Sun et al. (2014). In the in vitro experiments conducted in this study, seedlings treated with the YPD + L-Trp supernatant exhibited superior development of root hairs compared to those treated with YPD without L-Trp (Figure 3). These findings were confirmed in the in vivo trials, where root expression was higher in treatments with increasing biomass applications ranging from 5 x 105 to 5 x 107 cells mL−1 compared to the control. Similarly, Shalaby and El-Nady (2008) demonstrated that the application of increasing yeast doses in sugar beet plants was proportionally correlated with root growth. Based on the aforementioned, these effects on rootlet production could be mediated by auxins. However, the heightened root density could also be attributed to the fact that the roots did not need to extend in search of nutrients, as they were readily available in the inoculation zone, consistent with observations by Robinson (1994) and Forde and Lorenzo (2001) regarding root growth response to nutrient availability. Nevertheless, under conditions evaluated in this study, the treatment with an application of 5 x 108 cells mL−1 of yeast (T4) led to a significant decrease (p<0.05) in root biomass in lettuce seedlings. This could be associated with the high cell density of the inoculated dose, which remained on the substrate surface, causing root asphyxiation and waterlogging. The effects of low root oxygen availability on nutrient absorption and growth have been addressed by Morard and Silvestre (1996) and Smith and Gianinazzi-Pearson (1988).
Parameters evaluated in the aerial zone of lettuce seedlings showed that T3 (5 x 107 cells mL−1) recorded greater height development, leaf number, and consequently, dry and fresh weight (p<0.05). Additionally, chlorophyll measurements for this treatment were significantly higher compared to the other treatments and the control. This could also be attributed to auxin production by the yeast. These hormones promote plant growth (Leopold, 2022) and play a crucial role in the production of photosynthetic pigments, as established by Dao et al. (2018) and Zuo et al. (2019). Moreover, various studies suggest that auxins are directly or indirectly involved in chloroplast development (Kobayashi et al., 2017; Xu et al., 2018; Lupi et al., 2019). On the other hand, the higher chlorophyll levels could be associated with the ability of S. cerevisiae to produce cytokinins (Amer, 2004; Streletskii et al., 2019). Shalaby and El-Nady (2008) and Złotek and Świeca (2016) confirmed that chlorophyll production in plants treated with S. cerevisiae would increase with an escalating inoculation dose, linking this effect to cytokinin production by the yeast. Also, these chlorophyll levels could be associated with a higher available nitrogen content (Table 1), as pointed out by Rodríguez et al. (1998). In addition to the aforementioned, the percentage of non-vital biomass can release beneficial nutrients into the rhizosphere through lysis, promoting plant development (Lonhienne et al., 2014). In this context, the recorded values of NPK and organic matter could be responsible for the increased growth detected with the rising yeast dose applied to lettuce seedlings.
Based on the obtained results, it was determined that the most effective bio-stimulating dose in lettuce seedlings was 5 x 107 cells mL−1 in a 30 mL irrigation application in the seedling state with emerging roots. Comparing this dose with the commercial organic fertilizer Hampi in tomato seedlings revealed that both the number of leaves and the dry and fresh weight of the aerial zone were favored in the yeast application treatment compared to the control and the commercial treatment. Similar to lettuce, root length was greater in treatments without fertilizer application, but fresh and dry weight were higher in Tt1 and Tt2. This reinforces the hypotheses proposed in the lettuce seedling trial regarding the yeast’s effect on plant growth. Additionally, these results suggest that fertilization with this yeast dose has similar effectiveness to the commercial fertilizer used in this study.
Finally, it should be considered that the persistence and effectiveness of microorganism-based biostimulants are conditioned by various environmental factors that may affect their metabolic activity. Yeasts can exhibit different defense mechanisms against stress factors (Li et al., 2022). In the face of oxidative and hyperosmotic stress in yeast cells, antioxidants such as glutathione (GSH), superoxide dismutase (SOD), and catalase (CAT) play a fundamental role in survival (Lu et al., 2005). In this study, it was observed that the yeast exhibited catalase activity, suggesting its ability to cope with oxidative stress conditions. This finding aligns with the report by Izawa et al. (1996), who investigated the role of catalase in tolerance and the adaptive response to hydrogen peroxide (H2O2) stress in S. cerevisiae.
5 Conclusion
Sustainable resource management, waste reduction, and the promotion of responsible agricultural practices contribute to a more sustainable and equitable global development. The findings reported in this study indicate that brewer’s yeast residue is a biological agent with biofertilizing potential. The possibility of reusing this byproduct has a positive impact that can be reflected in: a) reducing its discharge into sewage water and revaluing the residue; b) incorporating a biostimulant into the agricultural sector, thus reducing the application of chemically synthesized products.
To the best of our knowledge, there are few studies that propose a comprehensive evaluation of yeast residue with minimal intervention, both as a PGP in vitro and in vivo. The observed results in lettuce (both in seed and seedling application) and tomato were consistent. Furthermore, the residue used as a biofertilizer showed comparable, and even superior, performance to that of the commercial fertilizer. Therefore, the results obtained here lay the groundwork for further exploration of the application potential of these yeasts. In future studies, it is essential to emphasize the assessment of various formulation strategies, as well as extrapolation to other substrates and crops. The study group plans to conduct future studies to assess the application of this technology in hydroponics and greenhouse settings.
Data availability statement
The original contributions presented in the study are included in the article/supplementary material. Further inquiries can be directed to the corresponding author.
Author contributions
MV: Conceptualization, Investigation, Methodology, Writing – original draft, Writing – review & editing. MM: Conceptualization, Investigation, Methodology, Writing – original draft, Writing – review & editing, Funding acquisition, Project administration, Supervision, Validation, Visualization. CV: Investigation, Writing – review & editing. PM: Investigation, Validation, Writing – review & editing. DP: Investigation, Methodology, Writing – review & editing. VP: Investigation, Methodology, Writing – review & editing. FV: Funding acquisition, Investigation, Visualization, Writing – review & editing.
Funding
The author(s) declare financial support was received for the research, authorship, and/or publication of this article. This work was supported by grants from: PDTS National University of San Juan - Argentina- SECITI [1499-23 R] and PIBBA National Council for Scientific and Technical Research [2872021010 0017CO].
Acknowledgments
We are grateful to Cerveza Ancestral SRL, for the residual yeasts provided. During the preparation of this work the authors used ChatGPT 3.5 in order to improve readability and language. After using this tool, the authors reviewed and edited the content as needed and take full responsibility for the content of the publication.
Conflict of interest
The authors declare that the research was conducted in the absence of any commercial or financial relationships that could be construed as a potential conflict of interest.
Publisher’s note
All claims expressed in this article are solely those of the authors and do not necessarily represent those of their affiliated organizations, or those of the publisher, the editors and the reviewers. Any product that may be evaluated in this article, or claim that may be made by its manufacturer, is not guaranteed or endorsed by the publisher.
References
Agamy R., Hashem M., Alamri S. (2012). Effect of soil amendment with yeasts as bio-fertilizers on the growth and productivity of sugar beet. Afr. J. Agric. Res. 8, 46–56. doi: 10.5897/AJAR12.1989
Amer S. (2004). Growth, green pods yield and seeds yield of common bean (Phaseolus vulgaris L.) as affected by active dry as affected by active dry yeasts, salicylic acid and their interaction. J. Agric. Sci. Mansoura Univ. 29, 1407–1422. doi: 10.21608/jpp.2004.238589
Amprayn K. O., Rose M. T., Kecskés M., Pereg L., Nguyen H. T., Kennedy I. R. (2012). Plant growth promoting characteristics of soil yeast (Candida tropicalis HY) and its effectiveness for promoting rice growth. Appl. Soil Ecol. 61, 295–299. doi: 10.1016/j.apsoil.2011.11.009
Bekatorou A., Psarianos C., Koutinas A. A. (2006). Production of food grade yeasts. Food Technol. Biotechnol. 44, 407–415.
Boraste A., Vamsi K. K., Jhadav A., Khairnar Y., Gupta N., Trivedi S. (2009). Biofertilizers: A novel tool for agriculture. Int. J. Microbiol. Res. 1, 23–31. doi: 10.9735/0975-5276.1.2.23-31
Bradstreet R. B. (1954). Kjeldahl method for organic nitrogen. Anal. Chem. 26, 185–187. doi: 10.1021/ac60085a028
Briggs D. E. (2004). Brewing: science and practice Vol. 108 (Cambridge, England: Woodhead Publishing).
Castro-Criado D., Abdullah J. A. A., Romero A., Jiménez-Rosado M. (2023). Stabilization and valorization of beer bagasse to obtain bioplastics. Polymers 15, 1877. doi: 10.3390/polym15081877
Cloete K. J., Valentine A. J., Stander M. A., Blomerus L. M., Botha A. (2009). Evidence of symbiosis between the soil yeast Cryptococcus laurentii and a sclerophyllous medicinal shrub, Agathosma betulina (Berg.) Pillans. Microbial. Ecol. 57, 624–632. doi: 10.1007/s00248-008-9457-9
Coelho A. R., Celli M. G., Ono E. Y. S., Wosiacki G., Hoffmann F. L., Pagnocca F. C. (2007). Penicillium expansum versus antagonist yeasts and patulin degradation in vitro. Braz. Arch. Biol. Technol. 50, 725–733. doi: 10.1590/S1516-89132007000400019
Dao G. H., Wu G. X., Wang X. X., Zhuang L. L., Zhang T. Y., Hu H. Y. (2018). Enhanced growth and fatty acid accumulation of microalgae Scenedesmus sp LX1 by two types of auxin. Bioresour. Technol 247, 561–567. doi: 10.1016/j.biortech.2017.09.079
De Souza C., Christofoleti-Furlan R. M., de Souza Miranda Muynarsk. E., Vinicius de Melo Pereira G., Lucas D. L., Basso L. C. (2019). “Biotechnological applications of non-conventional Yeasts,” in Yeasts in biotechnology. Ed. Basso T. P. (London, United Kingdom: Book Metrics Overview, Intechopen), 1–27. doi: 10.5772/intechopen.83035
Ditengou F. A., Teale W. D., Kochersperger P., Flittner K. A., Kneuper I., van der Graaff E. (2008). Mechanical induction of lateral root initiation in Arabidopsis thaliana. Proc. Natl. Acad. Sci. 105, 18818–18823. doi: 10.1073/pnas.0807814105
dos Santos M. ,. T. R., de Mello P. P. M., Sérvulo E. F. C. (2014). Solid wastes in brewing process: A review. J. Brew. Distill 5, 1–19. doi: 10.5897/JBD2014.0043
Droby S., Vinokur V., Weiss B., Cohen L., Daus A., Goldschmidt E. E. (2002). Induction of resistance to Penicillium digitatum in grapefruit by the yeast biocontrol agent Candida oleophila. Phytopathology 92, 393–399. doi: 10.1094/PHYTO.2002.92.4.393
EL-Ghamring E. A., Arish H. M., Nour K. A. (1999). Studies on tomato flowering, fruit set, yield, and quality in the summer season. I. Spraying with thiamine, ascorbic acid, and yeast. Zagazig. J. Agric. Res. 26, 1345–1364.
El-Motty E. Z. A., Shahin M. F. M., El-Shiekh M. H., El-Abd-Migeed M. M. M. (2010). Effect of algae extract and yeast application on growth, nutritional status, yield and fruit quality of Keitte mango trees. Agr. Biol. J. N. Am. 1, 421–429. doi: 10.5251/abjna.2010.1.3.421.429
El-Tarabily K. A., Sivasithamparam K. (2006). Potential of yeasts as biocontrol agents of soil-borne fungal plant pathogens and as plant growth promoters. Mycoscience 47, 25–35. doi: 10.1007/S10267-005-0268-2
El-Yazied A. A., Mady M. A. (2012). Effect of boron and yeast extract foliar application on growth, pod setting and both green pod and seed yield of broad bean (Vicia faba L.). J. Am. Sci. 8, 517–533.
El-Zohri M., Medhat N., Saleh F. E. M., El-Maraghy S. S. (2017). Some biofertilizers relieved the stressful drawbacks of calcareous soil upon black seed (Nigella sativa L.) through inhibiting stress markers and antioxidant enzymes with enhancing plant growth. Egypt. J. Bot. 57, 75–92. doi: 10.21608/ejbo.2017.262.1006
Fernandez-San Millan A., Farran I., Larraya L., Ancin M., Arregui L. M., Veramendi J. (2020). Plant growth-promoting traits of yeasts isolated from Spanish vineyards: Benefits for seedling development. Microbiol. Res. 237, 126480. doi: 10.1016/j.micres.2020.126480
Ferreira I. M. P. L. V. O., Pinho O., Vieira E., Tavarela J. G. (2010). Brewer’s Saccharomyces yeast biomass: characteristics and potential applications. Trends Food Sci. Technol. 21, 77–84. doi: 10.1016/j.tifs.2009.10.008
Forde B., Lorenzo H. (2001). The nutritional control of root development. Plant Soil 232, 51–68. doi: 10.1023/A:1010329902165
Fu S. F., Sun P. F., Lu H. Y., Wei J. Y., Xiao H. S., Fang W. T. (2016). Plant growth-promoting traits of yeasts isolated from the phyllosphere and rhizosphere of Drosera spatulata Lab. Fungal Biol. 120, 433–448. doi: 10.1016/j.funbio.2015.12.006
Fu S.-F., Wei J.-Y., Chen H.-W., Liu Y.-Y., Lu H.-Y., Chou J.-Y. (2015). Indole-3-acetic acid: a widespread physiological code in interactions of fungi with other organisms. Plant Signal Behav. 10 (8), e1048052. doi: 10.1080/15592324.2015.1048052
Galanakis C. (2020). Food waste valorization opportunities for different food industries, The interaction of food industry and environment (Cambridge, Massachusetts, United States: Academic Press), 341–422. doi: 10.1016/B978-0-12-816449-5.00011-4
Gordon S. A., Weber R. P. (1951). Colorimetric estimation of indoleacetic acid. Plant Physiol. 26 (1), 192–195.
Heldrich K. (1990). Official methods of analysis of the association of official analytical chemists (Virginia, U.S: Association of Official Analytical Chemists).
Hernández-Fernández M., Cordero-Bueso G., Ruiz-Muñoz M., Cantoral J. M. (2021). Culturable yeasts as biofertilizers and biopesticides for a sustainable agriculture: A comprehensive review. Plants 10, 822. doi: 10.3390/plants10050822
Hesham A. E. L., Mohamed H. M. (2011). Molecular genetic identification of yeast strains isolated from Egyptian soils for solubilization of inorganic phosphates and growth promotion of corn plants. J. Microbiol. Biotechnol. 21, 55–61. doi: 10.4014/jmb.1006.06045
Ibrahim H. A., El-Fiki I. A. I. (2019). Study on the effect of yeast in compost tea efficiency in controlling chocolate leaf spot disease in broad bean (Vicia faba). Org. Agric. 9, 175–188. doi: 10.1007/s13165-018-0221-2
Izawa S., Inoue Y., Kimura A. (1996). Importance of catalase in the adaptive response to hydrogen peroxide: analysis of acatalasaemic Saccharomyces cerevisiae. Biochem. J. 320, 61–67. doi: 10.1042/bj3200061
Kamal A. H. M., Komatsu S. (2016). Jasmonic acid induced protein response to biophoton emissions and flooding stress in soybean. J. Proteomics 133, 33–47. doi: 10.1016/j.jprot.2015.12.004
Kerby C., Vriesekoop F. (2017). An overview of the utilization of brewery by-products as generated by British craft breweries. Beverages 3, 24. doi: 10.3390/beverages3020024
Khedkar R., Singh K. (2018). Food industry waste: A panacea or pollution hazard? Paradigms pollut. Prev., 35–47. doi: 10.1007/978-3-319-58415-7_3
Kobayashi K., Ohnishi A., Sasaki D., Fujii S., Iwase A., Sugimoto K. (2017). Shoot removal induces chloroplast development in roots via cytokinin signaling. Plant Physiol. 173, 2340–2355. doi: 10.1104/pp.16.01368
Kuo C., Fu S., Chou F., Chen R., Chou J. (2018). Phosphate-solubilizing characteristics of yeasts. Mycosphere 9, 1117–1131. doi: 10.5943/mycosphere/9/6/4
Laskowski M., Grieneisen V. A., Hofhuis H., Hove C. A. T., Hogeweg P.and Marée A. F. M. (2008). Root system architecture from coupling cell shape to auxin transport. PloS Biol. 6, e307. doi: 10.1371/journal.pbio.0060307
Leopold A. C. (2022). Auxins and plant growth (California, United States: California University Press).
Li B., Liu N., Zhao X. (2022). Response mechanisms of Saccharomyces cerevisiae to the stress factors present in lignocellulose hydrolysate and strategies for constructing robust strains. Biotechnol. Biofuels 15, 28. doi: 10.1186/s13068-022-02127-9
Lin C. S. K., Koutinas A. A., Stamatelatou K., Mubofu E. B., Matharu A. S., Kopsahelis N. (2014). Current and future trends in food waste valorization for the production of chemicals, materials and fuels: a global perspective. Biofuels Bioprod. Biorefining 8, 686–715. doi: 10.1002/bbb.1506
Lonhienne T., Mason M. G., Ragan M. A., Hugenholtz P., Schmidt S., Paungfoo-Lonhienne C. (2014). Yeast as a biofertilizer alters plant growth and morphology. Crop Sci. 54, 785–790. doi: 10.2135/cropsci2013.07.0488
Lu F., Wang Y., Bai D., Du L. (2005). Adaptive response of Saccharomyces cerevisiae to hyperosmotic and oxidative stress. Process Biochem. 40, 3614–3618. doi: 10.1016/j.procbio.2005.03.061
Lupi A. C. D., Lira B. S., Gramegna G., Trench B., Alves F. R. R., Demarco D. (2019). Solanum lycopersicum GOLDEN 2-LIKE 2 transcription factor affects fruit quality in a light-and auxin-dependent manner. PloS One 14, e0212224. doi: 10.1371/journal.pone.0212224
Mącik M., Gryta A., Frąc M. (2020). Biofertilizers in agriculture: An overview on concepts, strategies and effects on soil microorganisms. Adv. Agronomy 162, 31–87.
Mahanty T., Bhattacharjee S., Goswami M., Bhattacharyya P., Das B., Ghosh A. (2017). Biofertilizers: a potential approach for sustainable agriculture development. Environ. Sci. pollut. Res. 24, 3315–3335. doi: 10.1007/s11356-016-8104-0
Mahapatra D. M., Satapathy K. C., Panda B. (2022). Biofertilizers and nanofertilizers for sustainable agriculture: Phycoprospects and challenges. Sci. Total Environ. 803, 149990. doi: 10.1016/j.scitotenv.2021.149990
Medani R. A., Ragab S. T. (2015). Improving Growth and Yield of Caraway (Carum carvi L.), Plants by Decapitation and/or Active Dry Yeast Application. Int. J. Curr. Microbiol. App. Sci. 4, 47–60.
Medina A., Vassileva M., Caravaca F., Roldán A., Azcón R. (2004). Improvement of soil characteristics and growth of Dorycnium pentaphyllum by amendment with agrowastes and inoculation with AM fungi and/or the yeast Yarowia lipolytica. Chemosphere 56, 449–456. doi: 10.1016/j.chemosphere.2004.04.003
Morard P., Silvestre J. (1996). Plant injury due to oxygen deficiency in the root environment of soilless culture: A review. Plant Soil 184, 243–254. doi: 10.1007/BF00010453
Nagodawithana T. W. (1991). “Products and uses of yeast and yeastlike fungi,” in Handbook of applied mycology, vol. 3. (Boca Ratón, Florida, Estados Unidos: CRC Press), 553–603.
Nassar A. H., El-Tarabily K. A., Sivasithamparam K. (2005). Promotion of plant growth by an auxin-producing isolate of the yeast Williopsis saturnus endophytic in maize (Zea mays L.) roots. Biol. Fertil. Soils 42, 97–108. doi: 10.1007/s00374-005-0008-y
Nimsi K. A., Manjusha K., Kathiresan K., Arya H. (2023). Plant growth-promoting yeasts (PGPY), the latest entrant for use in sustainable agriculture: A review. J. App. Microbiol. 134, lxac088. doi: 10.1093/jambio/lxac088
Nutaratat P., Srisuk N., Arunrattiyakorn P., Limtong S. (2014). Plant growth-promoting traits of epiphytic and endophytic yeasts isolated from rice and sugarcane leaves in Thailand. Fungal Biol. 118, 683–694. doi: 10.1016/j.funbio.2014.04.010
Olajire A. A. (2020). The brewing industry and environmental challenges. J. Clean. Prod. 256, 102817. doi: 10.1016/j.jclepro.2012.03.003
Pandi R., Velu G., Dananjeyan B. (2019). Isolation and screening of soil yeasts for plant growth promoting traits. Madras Agric. J. 106, 439–443. doi: 10.29321/MAJ.2019.000289
Parapouli M., Vasileiadis A., Afendra A. S., Hatziloukas E. (2020). Saccharomyces cerevisiae and its industrial applications. AIMS. Microbiol 6, 1. doi: 10.3934/microbiol.2020001
Pimenta R. S., Silva J. F. M., Coelho C. M., Morais P. B., Rosa C. A. (2010). Integrated control of Penicillium digitatum by the predacious yeast Saccharomycopsis crataegenesis and sodium bicarbonate on oranges. Braz. J. Microbiol. 41, 404–4410. doi: 10.1590/S1517-83822010000200022
Poloni V., Salvato L., Pereyra C., Oliveira A., Rosa C., Cavaglieri L. (2017). Bakery by-products based feeds borne-Saccharomyces cerevisiae strains with probiotic and antimycotoxin effects plus antibiotic resistance properties for use in animal production. Food Chem. Toxicol. 107, 630–636. doi: 10.1016/j.fct.2017.02.040
Qazanfarzadeh Z., Ganesan A. R., Mariniello L., Conterno L., Kumaravel V. (2023). Valorization of brewer’s spent grain for sustainable food packaging. J. Clean. Prod 385, 135726. doi: 10.1016/j.jclepro.2022.135726
Radić D., Karličić V., Đorđević J., Jovičić-Petrović J., Kljujev I., Lalević B. (2022). Soil yeasts promoting plant growth: benefits for the development of common wheat and white mustard. Zemdirbyste 109, 27–34. doi: 10.13080/z-a.2022.109.004
Rao R. P., Hunter A., Kashpur O., Normanly J. (2010). Aberrant synthesis of indole-3-acetic acid in Saccharomyces cerevisiae triggers morphogenic transition, a virulence trait of pathogenic fungi. Genetics 185, 211–220. doi: 10.1534/genetics.109.112854
Rawat P., Shankhdhar D., Shankhdhar S. C. (2020). “Plant growth-promoting rhizobacteria: A booster for ameliorating soil health and agriculture production,” in Soil health. Ed. Varma B. G. A. (Springer, Nature, Switzerland), 47–68. doi: 10.1007/978-3-030-44364-1
Robinson D. (1994). The responses of plants to non-uniform supplies of nutrients. New Phytol. 127, 635–674. doi: 10.1111/j.1469-8137.1994.tb02969.x
Rodríguez M., Alcántar G., Aguilar A., Etchevers J., Santizó J. (1998). Estimation of nitrogen and chlorophyll status of tomato with a portable chlorophyll meter. Terra Latinoamericana 16, 135–141.
Rokhbakhsh-Zamin F., Sachdev D., Kazemi-Pour N., Engineer A., Pardesi K. R., Zinjarde S. (2011). Characterization of plant-growth-promoting traits of Acinetobacter species isolated from rhizosphere of Pennisetum glaucum. J. Microbiol. Biotechnol. 21, 556–566. doi: 10.4014/jmb.1012.12006
Saravanan V. S., Kumar M. R., Sa T. M. (2011). “Microbial zinc solubilization and their role on plants, Bacteria in agrobiology,” in plant nutrient management. Ed. Maheshwari D. K. (Springer, Berlin, Heidelberg), 47–63. doi: 10.1007/978-3-642-21061-7_3
Sgroi M., Vagliasindi F. G., Snyder S. A., Roccaro P. (2018). N-Nitrosodimethylamine (NDMA) and its precursors in water and wastewater: A review on formation and removal. Chemosphere 191, 685–703. doi: 10.1016/j.chemosphere.2017.10.089
Shalaby M. E., El-Nady M. F. (2008). Application of Saccharomyces cerevisiae as a biocontrol agent against Fusarium infection of sugar beet plants. Acta Biologica Szegediensis 52, 271–275.
Shannon M. A., Bohn P. W., Elimelech M., Georgiadis J. G., Mariñas B. J., Mayes A. M. (2008). Science and technology for water purification in the coming decades. Nature 452, 301–310. doi: 10.1038/nature06599
Simate G. S., Cluett J., Iyuke S. E., Musapatika E. T., Ndlovu S., Walubita L. F. (2011). The treatment of brewery wastewater for reuse: State of the art. Desalination 273, 235–247. doi: 10.1016/j.desal.2011.02.035
Smith S. E., Gianinazzi-Pearson V. (1988). Physiological interactions between symbionts in vesicular-arbuscular mycorrhizal plants. Annu. Rev. Plant Physiol. Plant Mol. 39, 221–244. doi: 10.1146/annurev.pp.39.060188.001253
Streletskii R. A., Kachalkin A. V., Glushakova A. M., Yurkov A. M., Demin V. V. (2019). Yeasts producing zeatin. PeerJ 7, e6474. doi: 10.7717/peerj.6474
Sujanya S., Chandra S. (2011). Effect of part replacement of chemical fertilizers with organic and bio-organic agents in ground nut, Arachis hypogea. J. Algal Biomass Util 2, 38–41.
Sun P. H., Fang W. T., Shin L. Y., Wei J. Y., Fu S. F., Chou J. Y. (2014). Indole-3-acetic acid-producing yeasts in the phyllosphere of the carnivorous plant Drosera indica L. PloS one 9 (12), e114196.
Tartoura K. A. H. (2001). Effect of abscisic acid on endogenous IAA, auxin protector levels and peroxidase activity during adventitious root initiation in Vigna radiata cuttings. Acta Physiol. Plant 23, 149–156. doi: 10.1007/s11738-001-0002-4
Thomas K. R., Rahman P. (2006). Brewery wastes. Strategies for sustainability. A review. Asp. Appl. Biol. 80.
Tschoeke I. C. P., Fraga T. J. M., da Silva M. P., Souza T. P. C., Chinelate IG. C. B. (2023). Biogas production from malt bagasse from craft beer industry: kinetic modeling and process simulation. J. Mat. Cycles Waste Manag 25, 2809–2821. doi: 10.1007/s10163-023-01715-7
Walkley A., Black I. A. (1934). An examination of the Degtjareff method for determining soil organic matter, and a proposed modification of the chromic acid titration method. Soil Sci. 37, 29–38. doi: 10.1097/00010694-193401000-00003
White C., Zainasheff J. (2010). Yeast: The Practical Guide to Beer Fermentation; Kent, OH, USA : Brewers Publications.
Xi Q., Lai W., Cui Y., Wu H., Zhao T. (2019). BEffect of yeast extract on seedling growth promotion and soil improvement in afforestation in a semiarid chestnut soil area. Forests 10 (1), 76.
Xiao C., Chi R., Pan X., Liu F., He J. (2013). Rock phosphate solubilization by four yeast strains. Ann. Microbiol. 63, 173–178. doi: 10.1007/s13213-012-0458-z
Xu H., Zhang L., Li R., Wang X., Liu S., Liu X. (2018). SKL1 is essential for chloroplast development in Arabidopsis. Front. Plant Sci. 9. doi: 10.3389/fpls.2018.00179
Zaidi S., Usmani S., Singh B. R., Musarrat J. (2006). Significance of Bacillus subtilis strain SJ-101 as a bioinoculant for concurrent plant growth promotion and nickel accumulation in Brassica juncea. Chemosphere 64, 991–997. doi: 10.1016/j.chemosphere.2005.12.057
Złotek U., Świeca M. (2016). Elicitation effect of Saccharomyces cerevisiae yeast extract on main health-promoting compounds and antioxidant and anti-inflammatory potential of butter lettuce (Lactuca sativa L.). J. Sci. Food Agric. 96, 2565–2572. doi: 10.1002/jsfa.7377
Keywords: yeast biofertilizer, Saccharomyces cerevisiae, plant growth-promoting yeast, circular economy, sustainable agriculture
Citation: Vargas MF, Mestre MV, Vergara C, Maturano P, Petrignani D, Pesce V and Vazquez F (2024) Residual brewer’s Saccharomyces cerevisiae yeasts as biofertilizers in horticultural seedlings: towards a sustainable industry and agriculture. Front. Ind. Microbiol. 2:1360263. doi: 10.3389/finmi.2024.1360263
Received: 22 December 2023; Accepted: 16 February 2024;
Published: 11 March 2024.
Edited by:
Marika Pellegrini, University of L’Aquila, ItalyReviewed by:
Sumit K. Soni, Central Institute for Subtropical Horticulture (ICAR), IndiaWilgince Apollon, National Polytechnic Institute (IPN), Mexico
Copyright © 2024 Vargas, Mestre, Vergara, Maturano, Petrignani, Pesce and Vazquez. This is an open-access article distributed under the terms of the Creative Commons Attribution License (CC BY). The use, distribution or reproduction in other forums is permitted, provided the original author(s) and the copyright owner(s) are credited and that the original publication in this journal is cited, in accordance with accepted academic practice. No use, distribution or reproduction is permitted which does not comply with these terms.
*Correspondence: María Victoria Mestre, victoria.mestref@gmail.com
†These authors have contributed equally to this work and share first authorship