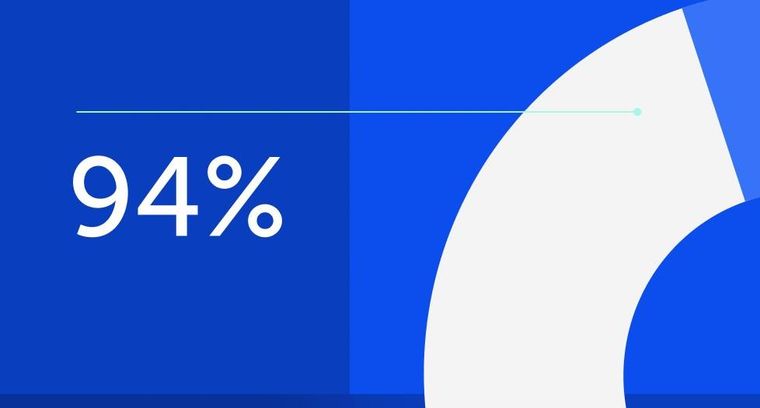
94% of researchers rate our articles as excellent or good
Learn more about the work of our research integrity team to safeguard the quality of each article we publish.
Find out more
REVIEW article
Front. Ind. Microbiol., 13 December 2023
Sec. Agriculture
Volume 1 - 2023 | https://doi.org/10.3389/finmi.2023.1308641
This article is part of the Research TopicMicrobial-based Inoculants for Agriculture: Production and Improvement of Commercial FormulationsView all 4 articles
To improve agricultural sustainability, an agriculturally productive system is required to maintain soil fertility and reduce the loss of soil biodiversity. One of the modern technologies employs microbial biostimulants that create abundant agricultural yield with high nutritional values, controlling disadvantages obtained from environmental changes. This review aimed to provide knowledge on the effects of biostimulants on microbial communities’ potential to promote agricultural production. Plant biostimulants are novel materials applied in the farming sector nowadays to improve crop yield and commonly occur in plant seeds and as an alternative to chemical derivative application on crop plants. Microbial biostimulants function as biological inputs to increase crop production and reduce the decomposition of soil. In conclusion, the application of biostimulants necessitates the accomplishment of an absolute choice of beneficial microbes as well as the potential to combat problems that will be encountered later in the agricultural sector.
According to the Food and Agriculture Organization (FAO), the soil’s potential to improve plant development as a result of making available major plant nutrients and required physical, biological, and chemical features that accommodate plant growth is known as soil fertility (Xiang et al., 2022). Recently, reports claimed that the degradation of agricultural soil has caused effectual challenges in the global agricultural sector.
Up to half the percentage of the global soil for farming activities and 24% of the farmlands across the planet have been subjected to soil fertility loss, decrease in crop production, and biodiversity (Allam et al., 2022). This process results from different abiotic factors affecting the soil, including loss of organic matter, environmental pollution, salinity, water, and wind erosion (Koza et al., 2022).
Considering the high rate of development of the universe, the decrease in cultivable area, and the reduction of genetic crops, the provision of new agricultural technologies was needed. Furthermore, reduced environmental occurrence in the agricultural system, targeted at promoting plant resilience to unfavorable environmental conditions, is becoming essential in ensuring the need for food with advanced nutritional values (Wang D. et al., 2022).
An interesting part of agriculture is its sustainability, which is the Farm-to-Fork (F2F) method, as reported by the European Union (EU) in May 2020, whose intention is to neutralize climate change by 2050 (Cué Rio et al., 2022). F2F has reported how it produces environmentally friendly healthy food by improving the change into a continuous and productive process acquired by the application and usage of chemical derivatives (pesticides) and antimicrobials, and over-fertilization is decreased (Yadav et al., 2023). Furthermore, in the organic farming system, F2F assists in the improvement of farmland to maintain the richness of the agricultural soil and inhibit biodiversity loss.
The result of the study strongly concentrated on applying agroecological knowledge to reduce possible chemical derivative applications on agricultural land and handle ecological relatedness and agro-biodiversity according to Charatsari et al. (2022). Agricultural ecology (agroecology) is supported by the improvement of biodiversity, biological process fortification, and the recycling of the biogeochemical cycle (Maity et al., 2022). With the knowledge of the agroecological system, the applications of biostimulant products can carry out specific roles including plant growth promotion and inducing resistance against disease invasion on plants directly and also maintaining agricultural production through the choice and induction of advantageous soil microbes (Feldmann et al., 2022).
Nowadays, plant growth promotion and crop production have been enhanced by plant biostimulants. Biostimulants are also categorized as biofertilizers, metabolic enhancers, and plant probiotics (Hamid et al., 2021). According to the US definition, biostimulants was defined as either organic or inorganic substances made up of beneficial microorganism that, when introduced on farmlands where crop plants were grown, promote nutrient consumption and nutrient effectiveness, tolerate abiotic stress, and improve crop quality (Benito et al., 2022). In comparison to the US definition, the EU delineated them as a unique substance in fertilizer product regulation (Kisvarga et al., 2022). Biostimulants have been applied in large-scale agriculture for several years, but nowadays, their production has reduced drastically, which makes them unavailable to farmers. Mostly used biostimulants were produced from the beneficial microbes (bacteria and fungi), chitosan, extract of seaweed, protein hydrolysates or amino acids, and organic acids (Kumari et al., 2022; Meddich, 2022), and other forms used were produced from biochar, concentrated enzymes, and microbial extracts. Biostimulants can be modified based on their composition of products and are known except for concentrated enzymes. The insufficient knowledge of the production of biological materials comprises multiple constituents, and it was speculated that the good form of the materials reveals the effect of synergies on the materials as conflicted to differentiate the materials separately (Wang Y. et al., 2022). Rasse et al. (2022) showed that the synergy among components within a product made it hard to determine the perfect methods accountable for stimulating response in crop plants, and this is the best way of identifying biostimulant activity based on the efficiency in their application. Therefore, this study concentrates on how biostimulants were employed for agricultural impingements in the production of crops and how to improve soil health for agricultural sustainability. We hypothesize that the biostimulant provides the absolute choice of beneficial microbes and also tackles challenges encountered by the invasion of phytopathogens on the crops and improves soil health in the agricultural sector.
Biostimulants are used in specialty crops, which sometimes produce tremendous income per acre, unlike row crops (Jolayemi et al., 2022). Specialty crops are known to be sensitive to environmental stresses (Aloisi et al., 2022). As a result, the potential is based on the commitment to an applied biostimulant that is higher for crops that are sensitive to climate-induced stress. The climatic condition has been a significant factor attributing to the cultivation of crops and poses a threat to food security globally (Koza et al., 2022). The application of biostimulants for agricultural practice has revealed their capacity to fight various stresses imposed by climate change like salinity, drought, extreme temperature, high rainfall, and pH of the agricultural soil (Mandal et al., 2023).
A typical example is obtained during planting that provides a chance to apply biostimulants to the crop planted on acres of farmland through seed treatment. After applying biostimulants, foliar application with chemical derivatives like herbicides, insecticides, and fungicides was applied on crop plants, and the chemical derivative invoked protection on the crops as revealed by Caicedo-Lopez et al. (2022). The study of Wozniak et al. (2020) on biostimulants reported the application of 380 plant biostimulant applications of 126 tests and revealed that 60% of the biostimulant usage was supplied as a foliar spray, 10% for seed treatment, and 30% in the form of soil. However, foliar applications are the most prevalent. When and how to apply and specific methods to employ biostimulant application depend on the materials used to produce the biostimulant and the technique of their application. Some problems experienced while producing the biostimulants are the potential to obtain compatible products, including chemical derivatives like fertilizers and pesticides. Despite many products in the market to produce biostimulants, there is less experience in research characterization required for the production of biostimulants (Fadiji et al., 2022a). Likewise, biostimulant reactions can be different after being applied on farmland for crop plant growth as a result of climate change (high temperature and precipitation) (Agbodjato et al., 2022). Applying biostimulants on farmlands requires adequate knowledge of the categories of the biostimulants to use.
One of the major elements required for living microbes is nitrogen (N), a major element needed for the biosynthesis of proteins and nucleic acids. Atmospheric nitrogen (N2) comprises 78% of air and is the most extensive storage of available N. Some microbes (diazotrophs) can convert N2 into gaseous ammonia (NH3) with the help of nitrogenase enzyme (Agbodjato et al., 2021). Nitrogenase enzyme has three types that are different in the cofactor of metal: vanadium–iron (V-Fe), iron–iron (Fe-Fe), or molybdenum–iron (Mo-Fe). The most common cofactor of nitrogenase is the Mo–Fe cofactor used by microorganisms, unlike another type of cofactor that reduces the fixation of nitrogen (Dong et al., 2022). The potential of nitrogenase enzyme is reduced by reaction with oxygen, which reveals how the bacteria perform the specific process to protect the enzyme under aerobic conditions. Bacteria do remove themselves from the oxygen environment through multiple mechanisms, with the prevalent methods for the production of a heterocyst or a nodule. The significant application of N-fixing bacteria in agriculture shows (Figure 1) the capacity of symbiotic relationship with the family of grasses (Poaceae) that has not been recognized to relate with biological nitrogen fixation (BNF) microbes for supplemental N (Scavo et al., 2022). One of the bacteria commonly used to produce BNF is Azospirillum brasilense. This bacterium has the ability to produce plant hormones and is classified as one of plant growth-promoting rhizobacteria (PGPRs). In most environments, these bacteria produce such hormones while associating with crop plants, but in an environment with high N content, N cannot be fixed (Das et al., 2022). New implements are employed to edit N-fixing microorganisms genetically to besiege the methods of modulating the N-fixation gene biological process (Figure 1). In the presence of N, BNF can be reduced, but modifying the microbe to disregard this reduction permits uninterrupted fixation of N, thereby promoting the source of N for sustainable agriculture (Yin et al., 2022). Studies have reported how endophytic symbiotes associate with crop plants intracellularly by living in the roots and/or shoots (Salhi et al., 2022; Velichko et al., 2022).
In an agricultural system, applying N-fixing microorganisms leads to an increment in the supply of N to the developing plants, which partly relieves the demand for applying N fertilizer. The important factor in improving the efficiency of the bacteria is to introduce them very close to the root of the developing plants via the in-furrow method or seed treatment (Bender et al., 2022). The relationship between bacteria and crop plants has been investigated for the Leguminosae crop plant family, including alfalfa, beans, clovers, and peas (Muresu et al., 2022). Inoculating these bacteria with soybean also revealed the prevalent function of N-fixing bacteria as biostimulants. A common example of the bacteria is Bradyrhizobium japonicum mostly employed as a result of its effectiveness and is the leading bacterium in the market (Krutyakov et al., 2022). Some studies have presented how Bradyrhizobium has been used in the USA and Argentina and showed how the production of soybeans was increased as a result of microbial inoculation (Liebrenz et al., 2022; Melissa et al., 2022; Fadel Sartori et al., 2023). Other microbes reportedly used are Azospirillum spp., which were introduced to crop plants through the co-inoculation method. The method does increase plant growth (plant root and shoot), unlike the treatment with only Bradyrhizobium, even though combined inoculation did not improve the N content in the grain.
Seaweed extracts are another biostimulant category produced from macroalgae species (seaweeds) as shown in Figure 2. These species differ in their composition and their usefulness as biostimulant effects. Macroalgae are replaceable materials that are utilized to manufacture biostimulants and closely supervised for continuous harvest to sustain the supply (Sujeeth et al., 2022). The manufactured products are produced vastly with materials used to produce them that are dependent on the species used, the state at which they were harvested, and the procedure of separation employed by the manufacturing companies. Alkaline hydrolysis is the prominent extraction process used unlike other processes, including acid hydrolysis, enzyme method, microwave method, super-critical fluid, pressurized liquid separations, ultrasound-assisted methods, and water-based methods (Samuels et al., 2022).
There are certain differences between organic products and macroalgae in their composition of carbohydrates, namely, laminarin, alginate, and fucoidan, which are greater in brown algae; ulvan is obtained from green algae and carrageen from red algae. The mentioned carbohydrate materials are sulfate compounds, and the method of extraction is a means of reducing the sulfhydryl compound production that minimizes the development of crop plants. The beneficial impacts of introducing seaweed extract to crop plants are associated with stress alleviation because they possess antioxidants that inhibit cell damage as a result of reactive oxygen species (ROS) while undergoing abiotic or biotic stress (Abideen et al., 2022; Meddich, 2022).
In the first century, the application of seaweed metabolites in the agricultural system was reported when the Roman Columella made use of organic manure and mulch treatments on agrarian fields (Armeli Minicante et al., 2022). The prevalence of the application of seaweed extracts in agricultural practice makes up foliar and has the potential to reduce abiotic stress associated with environmental factors, among which is drought tolerance (Samuels et al., 2022). Renowned concentration has been shifted to introducing biostimulants to the soil to promote the growth of plant roots and microbial functions in the rhizosphere region where plant roots are embedded (Cao et al., 2023). Certain studies have presented how seaweed extracts promote plant growth, improve abundant grain yield via phytohormones produced by the plants, control the rate of metabolism in plants, reduce stresses, and contribute to nutrient assimilation (Deolu-Ajayi et al., 2022; Rakkammal et al., 2022). No study has revealed inhibited growth and crop production, yet it does not signify that seaweed extracts constantly contribute to the wellbeing of crop plants, despite previous reports having shown dissimilarities in treated and non-treated crop plants.
The breakdown of organic matter by microorganisms is regarded as a complex procedure that manufactures byproducts through the process of degradation (Cayetano et al., 2022). Traditional perspectives of this route show that soil organic matter (SOM), which is the final product, comprises steady chemical materials known as humus (Figure 1). Humus is made up of recalcitrant materials tolerant to decomposition processes (Lv et al., 2022). These substances were grouped into different categories based on those soluble in alkali but insoluble in acid [humic acid (HA)], those soluble in alkali and acid [fulvic acid (FA)], and those insoluble in both alkali and acid (humin). They are all present and constitute approximately 60% of the organic matter inhabiting the soil (Pikuła and Ciotucha, 2022). These compounds were regarded to be resistant to degradation by traditional views; other views observed that decomposing organic matter is dynamic, so they have the ability to activate soil microbiome. Various compounds like peat soil, compost, and leonardite produce HA. The production and level of decomposition of HA or FA regulate their activities on crop plants, their composition, and their structure (Kou et al., 2022). Aside from alkali and acid feature differences, HA and FA also differ in makeup elements and their molecular size, which can supply knowledge of differences as biostimulants. HA is larger than FA in terms of molecular size, but FA constitutes various carboxylic groups and is therefore observed for possessing biological active sites in each molecule.
HA and FA have been employed for many years as farm inputs in the agricultural system for the production of crops and investigated for their activities on microbial communities, growth promotion in plants, and availability of nutrients (Yuan Y. et al., 2022). Both products have been applied on farmlands to improve soil fertility by aiding the assimilation of nutrients by plants. Both are obtained from different origins, and the sources greatly affect the potential of the products.
The phosphorus (P) content of the soil is almost 0.05% (w/w), while 0.1% of P is found in plants, and they are water-soluble (Enebe and Babalola, 2021; Peng et al., 2022). Due to their low availability, P fertilizer is required to satisfy the requirements for plant nutrients for particular crop production (Dong et al., 2023). Almost 90% of P fertilizer can attach to the soil when applied to the farmland, thereby making it inaccessible for plant assimilation, yet it can be found in the soil as stored P (Figure 2). Inaccessible P content can be accessible to crop plants via the activity of soil microbial communities through the process of degradation of organic P or dispensation of inorganic P (Emmanuel et al., 2021). The dispensation of inorganic P by microbial communities is prevalently carried out via the production of organic acids that promote accessibility of P by the following methods mentioned: chelation by cations, e.g., Fe2+ or Fe3+ and Ca2+, which inhibits them from fixing available P; and reduction of soil pH, which liberates mineral P complexes, especially Ca (Sible et al., 2021). Organic acid secretion among microbial species differs, but gluconic and 2-ketogluconic acids are mostly common (Uroz et al., 2022). All living things need P as a major constituent for producing nucleotides, phospholipids, nucleic acids, and enzymes. However, microbes have methods by which they can acquire P, despite some of the microbes being more effective than others. Some microbes are P solubilizers, and recent research has revealed how fungi (Aspergillus and Penicillium) and bacteria (Bacillus, Pseudomonas, and Rhizobium) are the most effective P solubilizers (Sarmah and Sarma, 2022; Masowa et al., 2023). Gram-negative bacteria solubilize P more compared to gram-positive bacteria (Alori et al., 2017). Reports have revealed how tricalcium phosphate was employed as an insoluble phosphate source to obtain phosphorus-solubilizing microorganisms (PSMs), despite that some microbes can solubilize P (Fatima et al., 2022; Tariq et al., 2022). Nevertheless, the concurrent application of multiple P sources has been advocated for better means of selecting PSMs. These sources contained FePO4 or Al2(PO4)3 for acidic soils, CaPO4 for alkaline soils, and phytates for those with abundant organic P. Another means of improving the soil state P is via organic phosphate hydrolysis by activating extracellular enzymes (Elhaissoufi et al., 2022). Although the process of P solubilization is clear, the rate of improving those methods via inoculation to promote the production of crops is unknown.
PSM has been investigated for several years, and many studies have been performed in the field and the laboratory. However, PSM has been employed recently as an agricultural implement, and the full activity of its exploitation has not been recognized (Fatima et al., 2022). The rate of the production of soil P varies from type to soil and agricultural system. The application of the essential organisms to improve the P solubilization for the specific process is demanding. Three strains of PSM were applied individually to reveal the degree to which they can promote the production of wheat by 19%–24%, while the co-inoculation of these strains revealed a 33% increase in production compared to the uninoculated plants (control) (Ahmad et al., 2023).
Bacillus megaterium M3 has been reported to be the most significant inoculant because it improves the characteristics of P cycling in the soil and increases the assimilation of P by crop plants, the potential of soil phosphatase, and multiplication of microbial communities biomass (Turan et al., 2012; Garbin et al., 2022). Different strains of B. megaterium have been investigated, contributing to the promotion of P solubilization and increased sugarcane production compared to the control (uninoculated). However, due to a 25% decrease in P fertilizer applied without lessening the production, unlike complete P application (Sundara et al., 2002; Kreutz et al., 2022), an experiment conducted in a greenhouse employing multiple strains of bacteria with and without P on maize crop plant showed how B. megaterium improved the development of the plant in unsterilized pots (Ibarra-Galeana et al., 2017; Lan et al., 2022), whereas other strains employed in sterilized pots cannot promote the development of the plant in unsterilized soils. The results revealed that B. megaterium not only displayed its potential as PSM via direct P solubilization but also promoted the potential of the soil PSM biomass.
The physiological mechanism of crop plants is influenced by microorganisms, chiefly PGPRs and arbuscular mycorrhizal fungi (AMFs), by carrying out the biostimulant potential (Emmanuel and Babalola, 2020). The characteristics of plant growth-promoting microorganisms (PGPMs) while interacting with plants revealed various ideas. However, it is not obvious if the association is parasitic or saprophytic. These microbes influenced the crop plants to secure their survival (Gupta and Nair, 2020).
The endophytic bacteria commonly found in plants and rhizosphere bacteria are found in the soil at the root region. These bacteria contribute immensely to the growth of the crop plant and are commonly referred to as PGPRs (Adedayo et al., 2022a). Aside from the potential of the bacteria to contribute to the improvement of soil productivity, they are also involved in the tolerance of abiotic stresses, thereby promoting the growth of the plants. The following are PGPR phyla in which various studies have been conducted: Acidobacter, Actinobacteria, Bacteroidetes, Chloroflexi, Firmicutes, and Proteobacteria. As a result of huge diffusion, the following PGPR genera have been investigated widely: Bacillus, Aeromonas, Clostridium, Azospirillum, Enterobacter, Pseudomonas, Azotobacter, Klebsiella, Arthrobacter, Rhizobium, Gluconacetobacter, and Serratia (Koner et al., 2022).
The fungi classified to Glomeromycota phylum are AMFs, which comprise three major classes (Archaeosporomycetes, Glomeromycetes, and Paraglomeromycetes) and five orders (Diversisporales, Glomerales, Paraglomerales, Gigasporales, and Archaeosporales). The potential of the Glomeromycota phylum is associated with the establishment of plant roots via symbiotic association of endomycorrhizae. Presently, it is revealed that 80% and above of plants can produce a beneficial relationship with AMFs (Koza et al., 2022). Despite that several AMFs have been identified, few have been utilized in the agricultural process (Khaliq et al., 2022). The obtainable inocula constitute species that belong to the genera Funneliformis and Rhizophagus, which are symbiotic and mostly inhabit soil located in a wide climate zone (Xi et al., 2022).
Even though PGPRs and AMFs have been acquiring great accomplishments in the modern agricultural system, the potential by which PGPMs beneficially influence crop plants and soil is not definite. The beneficial features count not only for microbial communities or the classification of soil but also for the stress and the form of inoculum. The potential of the microbes to contribute to plant growth are as follows: hormonal control, the promotion of nutrient utilization, equilibrium of cell oxidative nature, water use efficacy, and photosynthetic physiological response development.
Some hormones play a significant role in controlling plant physiological functions, among which is abscisic acid (ABA). ABA operates as an anti-transpiration hormone that reduces the loss of water molecules from the plant via the opening and closing of stomata (Cui et al., 2022). Accordingly, the significance of ABA is evident, particularly under various stress conditions, viz., high temperature, drought, or salinity. The biosynthesis of ABA can be impacted by several agents, comprising the existence of PGPMs in the soil (Malgioglio et al., 2022). Nonetheless, the effects associated with the changes in ABA after their introduction are considered. Some studies have revealed the introduction of a high content of ABA in crop plants planted under stress conditions (Ayaz et al., 2022). On the contrary, some studies have shown improvement in ABA concentration of crop plants planted under stress conditions and propagated with PGPRs and AMFs (Cantabella et al., 2022; Paravar et al., 2023). Under stress conditions, the tomato plants inoculated with the ABA biosynthetic gene 9-cis-epoxy carotenoid dioxygenase (SlNCED) in AMF stress conditions showed that ABA control can be controlled by the forms of stress. Chitarra et al. (2016) revealed how SlNCED activity of receptors decreases to reduce gene expression in tomato roots inhabited by Septoglomus constrictum under high-temperature conditions but stayed undisturbed under heat stress conditions and showed that the biosynthesis of ABA was likewise affected by different mycorrhizae.
Auxin (IAA) is another phytohormone and specific auxin produced by the plant. This phytohormone can control various cell processes, including the production of root hairs, root growth, and cell division and elongation. The association occurring between PGPMs with phytohormones, especially auxins (IAAs), is clear. However, IAA high stimulation was detected in plant tissues planted in soil as a result of drought or high salinity. Nevertheless, as mentioned in the literature, much more IAA stimulation was documented in plants inhabited by AMFs than under stress conditions. The morphology of root variations was also observed in some crop plants, as reported by Fadiji et al. (2022b). Such a study was also described by Liu et al. (2018), which reported the auxin pathway in plants inoculated with Funneliformis mosseae grown under drought conditions.
The technological facts propose that AMF stimulates the secretion of IAAs and exaggerates the plants’ physiological effects on abiotic stress through the production of morphological modifications of the plant root. Some PGPMs do produce IAAs, so they are regarded as the source of IAAs from the outer part of crop plants (Fadiji et al., 2022b). The potential to induce and secrete various materials that are utilizable to the crop plant is infinite to the IAA biosynthesis.
Some PGPR strains can produce an enzyme ACC deaminase that catalyzes the transformation of ACC to ammonia and α-ketobutyrate, the product of ethylene. Accordingly, the production of ACC deaminase contributes to reducing the degree of ethylene in plants and soil, with a subsequent decrease in environmental stresses on crop plants (Adedayo et al., 2022c). When PGPRs stimulate IAAs and ACC deaminase, an interference effect takes place. However, IAAs induce the development of the plant, while ACC deaminase reduces the production of plant ethylene. Certain PGPR-producing ACC deaminase regulates the growth of plants by improving the levels of stress-related hormones like jasmonic acid (JA) and salicylic acid (SA) (Gowtham et al., 2022). JA is an endogenic control hormone that performs a significant function in contrast to development procedures since it partakes in major signaling routes of biotic or abiotic responses. Studies have evaluated the biosynthesis of JA rigorously corresponding to AMF symbiosis (Diksha and Parul, 2023). Therefore, the introduction of AMF promotes the level of JA in cucumber plants, durum wheat, and maize, in both standard and stressful states (Fiorilli et al., 2022).
Meanwhile, SA stimulates stress-related gene expression to regulate membrane stability and avoid oxidative modifications. However, SA functions as a significant factor in the control of the disease signaling routes (Ayyaz et al., 2022). SA likewise partakes in the beneficial association between PGPMs and plants as reported by Tyśkiewicz et al. (2022).
The introduction of PGPMs has been demonstrated to contribute to mineral assimilation, phosphate solubilization, siderophore production, and atmospheric nitrogen fixation, therefore impacting nutrient use efficiency (NUE) (Malgioglio et al., 2022). Major elements are essentially required in mineral uptake in plants. Such elements include Ca, Cu, Fe, Mg, Mn, K, and Zn and are better absorbed, but Na is egested because it is a deleterious element (Yuan S. et al., 2022). Several studies have associated the promotion of mineral assimilation with the increase in the morphology of plant roots upon PGPM inoculation (Ali F. et al., 2022; De Palma et al., 2022). AMF inocula produced extraradical hyphae to help plant roots increase the soil volume exploitation, hence increasing the nutrient assimilation potential (Chandra et al., 2022). This process could also be defined by the overexpression of exclusive ion channels, which results in the increment of the K+/Na+ ratio, leading to an effective technique that promotes plant tolerance to salt concentration environment (Karimi et al., 2022).
Another way that PGPMs can assist plantations is to assimilate nutritive substances, revealing their capability to add acid to the soil. Some PGPMs have been reported to manufacture organic compounds and release them into the soil, hence alleviating the inorganic phosphate solubilization (Pi) and K (De Palma et al., 2022). Moreover, recent research has displayed how they can produce acid phosphatases and phytases, thereby increasing the mineralization of P (Saranya et al., 2022; Zhao et al., 2022). In addition, hydrogen cyanide (HCN)-stimulating bacteria partake in accelerating the accessibility of P and the utilization of heavy metals with advantageous results for PGPRs and crop plants (Elnahal et al., 2022).
Bioinoculation also promotes the efficiency of nitrogen use with the aid of microorganisms that can fix N. A common PGPR is the genus Rhizobium, which fixes atmospheric nitrogen gas (N2) to produce NH3. However, the genera Azotobacter and Azospirillum also show their potential to fix N2 in non-leguminous plants via a beneficial or free-living association (Patra and Mandal, 2022). Moreover, Wang H.-R. et al. (2022) reported how AMFs were introduced into tobacco plants and further revealed the association between improved N assimilation and upregulation of nitrate reductase (NR) potential. The greater the NR activity granted for a better N uptake, the greater the increase in the production of amino acid and protein biosynthesis (Bandyopadhyay et al., 2022).
One of the significant features of PGPMs is their capacity to make ion-chelating substances, called siderophores. Although Fe is one of the elements existing in the abundant form in the soil, it exists as an impure state Fe3+, which is insoluble and not readily bioavailable. Accordingly, some microorganisms produce siderophores to clean the iron from the mineral phases and ingest the Fe. Therefore, plants can gain from the siderophore that the bacteria produce to assimilate Fe required for several mechanisms like photosynthesis (Dhankhar et al., 2022).
Under stress conditions, ROS and reactive nitrogen species (RNS) are formed to control the diversity of physiological methods to ensure the survival of plants (Adedeji et al., 2020). Several biomolecules, including nucleic acids and lipids, are sensitive to their strong oxidative potential because ROS and RNS are highly reactive molecules. Therefore, unmaintainable harm can be introduced to cell membranes, DNA, enzymes, and RNA, causing cell death. Some researchers have reported how plants inoculated with PGPMs had huge cleansing potential against ROS and RNS (Singh et al., 2021). In comparing the tissue of inoculated plants to non-inoculated plants, lesser quantities of H2O2 and malondialdehyde were confirmed.
The decrease in the level of ROS stages under various stress conditions could be demonstrated by the rise in the potential of antioxidant enzymes in crop plants already inoculated with PGPM (Castiglione et al., 2021). Under abiotic stress conditions, ascorbate peroxidase (APX), peroxidase (POD), catalase (CAT), superoxide dismutase (SOD), glutathione reductase (GR), and glutathione peroxidase (GPX) activities are considerably promoted in colonized plants. The inhibited potential of GR, SOD, and POD in the mycorrhizal roots of the drought-sensitive wheat plant and digit grass was confirmed (Faria et al., 2022). However, some reports revealed that PGPR treatments reduced CAT, SOD, GR, and GPX potentials when introduced to crop plants in comparison to uninoculated ones (Castiglione et al., 2021). Camaille et al. (2021) proposed that IAA-producing bacteria promoted the aggregation of osmolytes and decreased enzymatic antioxidant potential by speeding up the transition of the biochemical reactions occurring in wheat plants.
In contrast, non-enzymatic antioxidants, including carotenoids, glutathione, organic acids, polyphenols, and vitamins, take part in the activity of PGPMs’ relationship with crop plants, causing their effects on oxidative stress. A case study revealed the collection of glycine betaine and proline used for preventing cellular oxidative impairment in crop plants already inoculated with AMFs and PGPRs. An exclusion was proclaimed by Zhao et al. (2023), who provided inconsistent data concerning rhizobacteria that produce proline in plant tissues in comparison with inoculated crop plants grown in the drought-stress region. Ali and Khan (2021) explained how inoculation could credibly activate early proline collection in plantations by decreasing the choice of late collection and encouraging the adaptation of the crop plant under drought-stress conditions. Moreover, the plant growth stage was also revealed to perform a significant function in proline accumulation.
Many studies on PGPM have described that its application can influence the relationship of plant and water features by improving the activity of plant leaf water, water content, stomatal conductance, transpiration process, or other features that can specify affirmative results on the utilization of water.
AMF-inoculated tomato and maize plants under irrigation and drought-stress conditions revealed an essential promotion of the specific apoplastic flow of water, unlike as described in control plants by Castiglione et al. (2021). AMFs possess the potential to control the shift between cell-to-cell and apoplastic water transport, resulting in higher flexibility in the process of plant effects under stress conditions. The condition can be the result of positive control of aquaporin genes that significantly promote the water activity in the leaf, thereby proposing that AMF plants control water content in cells. From another point of view, the sustenance of a high stomatal conductance permits the assimilation of CO2 during the process of photosynthesis in plants. A huge amount of CO2 that occurs intracellularly piled up under the nature of stress negatively impacts the absorption of sunlight in Ricinus communis. However, it has been explained that the existence of AMFs decreases the concentration of intracellular CO2, relieving the decrease in the process of photosynthesis as a result of stress conditions (Koza et al., 2022). The symbiotic activity of PGPM on plant photosynthesis was confirmed by the promotion of photosynthetic processes under drought salt stress and other optimal conditions (Maitra et al., 2022). The accumulation of increased photosynthetic pigment content was found in various plant species. Most importantly, the green pigment of the leaves (chlorophyll) appeared to be modified. It has been considered that the symbiosis association of PGPM with plants negates the impact of stress, antagonizing the breaking down of chlorophyll and improving the quantum product of open photosystem II.
Some studies have used native or allochthonous PGPMs, and their ability as biostimulants was compared (Kashyap et al., 2023). In most cases, plants are influenced positively by inoculants, which promote the ability to assimilate nutrients and reduce oxidative stress (Emmanuel and Babalola, 2020). To obtain the best inoculation methods for planting and growing vegetation in degraded ecosystems, autochthonous and allochthonous microbial communities were assessed (Ojuederie and Babalola, 2017). Some studies have explained the activity of autochthonous microbes that are adapted to the stress conditions and physiologically and genetically of the referenced region, unlike allochthonous ones (Ojuederie and Babalola, 2017; Enebe and Babalola, 2018). Mainly, since autochthonous microbes can quickly and efficiently infect the root of a plant, they, therefore, show suitability to antagonize the negative factors obtained from stressed environments to promote the assimilation and transportation of P and to improve plant growth compared to those obtained from the collections. Nevertheless, other reports likewise revealed how PGPMs are required not as biostimulants when compared to non-native microbes.
Pseudomonas putida allochthonous strain is a typical bacterium that is effective as a phosphate-solubilizing bacterium (Khajeeyan et al., 2022). This is equivalent to significant characteristics since the speedy attraction of P in a fixed state is unavailable for assimilation by plants. However, from the soil samples obtained in the agricultural soil, Bacillus spp. were isolated and used to improve the development of maize, tomato, and Arabidopsis plants in a greenhouse (Olowe et al., 2022).
Some microbes isolated from desert and salinity soils were evaluated. Studies have revealed how PGPMs isolated from decomposed matter applied with high salt concentration and drought could promote tolerance of plants to high temperatures and salinity (Munir et al., 2022). The investigations propose that the effectiveness of biostimulants does not inevitably correspond to the origin of the inoculant but primarily to its internal features by which their relationship with the host is satisfactory and advantageous. Orozco-Mosqueda et al. (2022) agreed with the proposition that revealed the introduction of some bacteria, chiefly Herbaspirillum sp. and Rhizobium sp., on two major plants’ hosts and the different results they produced. Most especially, they discovered the growth of the plant in ryegrass inoculated with Rhizobium sp. and the highest growth improvement in red clover inoculated with Herbaspirillum sp. under a P-deficient soil stress environment. Furthermore, two different methods of promoting the availability of P were suggested and explained based on the inoculum and plant relationship. The same knowledge was used to explain the factors obtained from the introduction of three different PGPR halotolerant variants, namely, Exiguobacterium aurantiacum, Bacillus pumilus, and Pseudomonas fluorescens, in salinity-tolerant and wheat cultivars sensitive to salinity (Sayahi et al., 2022). It was reported that B. pumilus and E. aurantiacum showed more results on the salt-resistant genotype, in contrast to the development of promotion of the genotype sensitive to salinity conducted by P. fluorescens. As a result, the outcome revealed the assortment of potential variants to be introduced in the soil and the greatest significant influence to improve the accomplishment of bioinoculation under stressful environment. The accomplishment of abundant yield and presentable attribute may pose unusual choices, particularly the type of microorganism introduced when considering the purpose of making salt environment and high-temperature soils fertile, as compared to the restoration (replanting) of a forest that had been reduced by fire or cutting and restoration of plants in dissolved soil. Therefore, the idea of applying microbial biostimulants is more efficient than others.
Considering the major facts, it is relevant to choose available PGPMs and symbiotic associations under the most deviated environmental conditions. Nevertheless, as a result of the increased genetic attributes of PGPMs, further studies need to identify the features of the bioinoculants and the potential of using various PGPM genera as plant biostimulants to acquire the essential multispecies variants or single strains based on the selected purpose.
Biostimulants improve the rate of assimilation of nutrients in crop plants. However, the major mechanisms resulting from the improved nutrient assimilation and availability are not primarily known. The major potential of biostimulants on available nutrients is via modifications in enzyme action due to the catalysis of hydrolytic and oxidative breakdown of organic materials by soil enzymes (Sible et al., 2021). These changes take place in multi-step methods with particular enzymes changing the stages, making it hard to observe specifically where the action of the biostimulant takes place. However, these mechanisms include multiple steps that change one after the other by the action of a specific protein. The alignment of enzyme-mediated degradation stages is made up of the initial (first) and terminal (last) steps (Lopez-Cantu et al., 2022).
Terminal-step enzymes are effective in treatments as a result of their involvement in the catalytic modification of food material to bioavailable products. Therefore, the last stage in the nutrient product is an indirect means of making available the nutrient and renders perceptivity, as a biostimulant can produce nutrients. Agricultural soil is made up of soil organic matter that consists richly of nutrients, C, N, P, and S (Vermeiren et al., 2022) and, likewise, enzymes associated with the nutrients mineralization, which is a major signal for clarifying biostimulant mechanisms associated with nutrient availability. They function in the recurrences of SOM that subject soil enzyme potentials as signs of healthy soil, thereby producing a major usage of biostimulants as soil health materials (Asghar et al., 2022). The following are primary terminal-step enzymes.
It is involved in the catalytic hydrolysis of complex sugar glycosidic bonds to produce glucose (Samanta, 2022). The glycosidic bonds are a crucial factor in the cell wall, and this bond decomposition is interceded by bacteria and fungi (Pourjafar et al., 2022). The variable activity of β-glucosidase shows the distinguishing factor in breaking down residue and soil C accumulation and has been employed by the U.S. Department of Agriculture (USDA) Natural Resources Conservation Service (NRCS) Soil Health Division as a soil biological health indicator.
These enzymes act on proteins. They are specific components of organic N embedded in the soil and are, therefore, expected to be the rate-restrictive state in the process of mineralization of N from SOM (Tang et al., 2022). In the hydrolysis of proteins, the protease cleaves the peptide bonds in amino acid molecules, producing peptide materials that are worked upon by aminopeptidases to liberate available N and amino acids. Variability in protease activity showed microbial biomass or breakdown of residue as a result of activity in the crop plant root and nutrient cycling (Hakimi et al., 2022).
Amino acid residues produced from this enzyme are the N-terminus of proteins and peptide chains. Many enzymes take part in the decomposition of proteins, majorly in the peptide bonds. Nevertheless, leucine aminopeptidase is common in soils and is otherwise referred to as a good indicator of protein degradation (Gong et al., 2022). Together with other aminopeptidases, leucine aminopeptidase carries out an important function in the release of organic N as the terminal stage of protein decomposition into amino acids (Ghifari et al., 2022). Higher stages of leucine aminopeptidase potential show improved mineralization of organic N, and therefore, N is released from SOM upon the organic process of deaminase.
These enzymes, composed of phosphomonoesterases and phosphodiesterases, are included in the production of orthophosphate from organic P (Richardson et al., 2022). Organic phosphates do occur as a single-ester or double-ester bond that is joined by unique organic catalysts, phosphomonoesterases or phosphodiesterases. Microbes multiply to occupy their ecosystems and are involved in the phosphomonoesterase tract, while others are involved in the phosphodiesterase tract (Bauters et al., 2022). The measurement of the activity of both P-producing enzymes provided a broad range classification of P activities. Similar to the mineralization of N obtained via SOM, the two P enzymes worked hand in hand to make crop-available P from its organic form.
This enzyme helps in the production of inorganic S (SO42−) by linking the S ester linkage to the OM (Silva et al., 2022). Approximately 98% of soil S can be found in the SOM, and 35%–74% of this S content is attributed to the S esters (Kumar et al., 2022). For proper assimilation of S by crop plants, the potential level of arylsulfatase can be derived from the organic S. Therefore, the estimation of soil enzyme potentials as characterized by biostimulants can suggest how the biostimulant affects nutrient transportation in the rhizosphere soil of crop plants. However, as a result of β-glucosidase, it acts coincidentally as an effect produced on soil health.
Any initiation of enzymatic procedures due to biostimulant introduction will be triggered by plant–microbe activities except if the biostimulant is an enzyme. Abundant enzyme activities do take place via higher production of enzymes in plant–microbe interaction (Ayangbenro et al., 2022) or by improving microbial biomass or crop growth, thereby producing abundant enzymes. The biomass of microorganisms and the diversity of the microbes can be traced to the potential methods and activity of biostimulants applied to agricultural soil (Table 1). Moreover, the evaluation of microbial communities inhabiting tomato plant soil is conducted to confirm the quality of the soil and its potential for agricultural management practices, generating information on the attributes and health status of the soil (Adedayo et al., 2022b). Several procedures were employed to evaluate microbial communities, total microbial biomass, and microbial respiration due to their utilization of C substrates to increase their biomass in the soil. These procedures include Sanger sequencing [16S rRNA and internal transcribed spacer (ITS)] analysis, whole genome sequencing, phospholipid fatty acid (PLFA) analysis, and metagenomic sequencing analysis and are mostly adopted nowadays to reveal the diversity of the microbes inhabiting various soils (Adedayo et al., 2022c). These procedures are likewise employed to confirm the persistence of applied microbial communities and microbial functional diversities in the rhizosphere soil when analyzed with the control soil samples. Despite the analysis of microbial communities’ diversity to derive biostimulants and their environmental challenges as a result of their introduction being expensive to investigate, the potential of microbial diversity analysis makes available functional features for assessing biostimulants.
The idea of the health status of soil has been in existence for a long time in the field of agricultural systems, and it was regarded as soil quality and tilth (Liptzin et al., 2022). Attention to environmental challenges of improper, unsuitable management leads to higher soil management resulting in erosion of nutrients into surrounding bodies of water, causing pollution to the waterways and a rise in the idea of soil health. Multiple features are characterized by soil health, which is biologically mediated and promoted by the introduction of biostimulants. The USDA NRCS is conducting thorough research with scientists in the USA to set up consistent methods for testing and observing the health indicators of soil and to choose some enzymes produced by microbes inhabiting the soil, detect soil respiration levels, and confirm the total soil organic C (Gutknecht et al., 2022). These features can likewise be signals of biostimulant potential in crops, thereby providing knowledge for their introduction to control the development of the crop and the health status of the soil as observed in Table 1.
Farmers employed biostimulants for adequate production of yield in the growing season with little concentration for long-period impacts on the soils they have inoculated over time. Although biostimulants do not produce immediate effect upon its application on plants, yet, improvement of soil health and production of abundant yield will be achieved overtime. Nevertheless, measuring biostimulants in soils by employing long-term methods is unsatisfactory. The direct effect on soil bioactivities promotes soil health as the potential of soil microbes to utilize C substrate. Biochar, a typical example of a biostimulant, when added as a C substrate to the agricultural field, is resistant to decomposition (Xin et al., 2022). It has been proposed that the reiterated introduction of biostimulants can improve the rates of soil C and agricultural yield, resulting in abundant production of seasonal crops and biological C utilization (Zanli et al., 2022). The continuous increase of C can transform the ratio of C:N in the soils, which can control more N and reduce plant activities. These require the employment of an extended study of a biostimulant’s attribute on the cycling of nutrients in the soil, soil C, and improving soil health (van der Sloot et al., 2022).
Green Deal intentions, in addition to the rules of the European Regulation 2019/1009, proposed to decrease the application of chemical fertilizer through various methods. The methods, including the promotion of biostimulant efficacy, were brought to attention. To obtain the necessary tools that can be employed to exert the efficient biostimulant potential in plants, various reports concentrated on the association of plants and microorganisms, including PGPRs and AMFs (Bartucca et al., 2022).
The advantageous factor of the accumulation of various forms of microbes employed as plant biofertilizers may hinge upon their potentiality under unfavorable conditions of the environments. Studies have revealed the activity of biostimulants when different materials were added together, describing synergistic interactions (Montoneri et al., 2022; Navarro-León et al., 2022; Canellas et al., 2023). This review portrays how biostimulants (microbial-based) can be produced employing the following methods: AMF/PGPR multispecies consortia, a single PGPM strain, and aggregation of PGPMs with inorganic and organic chemicals.
PGPRs may behave not only as a biostimulant in the presence of AMFs but also act as a mycorrhizal supporter, mostly when rigorously related to their mycelium and spores (Pandit et al., 2022). In other words, AMFs can improve the potential of solubilizing phosphorus and fixing nitrogen. Mycorrhizal supporter potential can be demonstrated under concurrent introduction with PGPRs and AMFs. The inhabitation of plant roots by PGPRs may improve their potential to stimulate cell wall-destructing enzymes that alleviate AMF formation. Furthermore, PGPRs do produce enormous secondary metabolites that can improve root exudation, producing higher cell porosity and hyphal growth (Koza et al., 2022).
The application of co-inoculation of AMFs and PGPRs not only improves plant growth but also has an advantageous status for AMFs and PGPRs. Accordingly, the application of combined PGPRs and AMFs as biostimulants has been inquired about in various reports. The efficacy of a biostimulant made up of both bacteria assisting plants and AMFs was observed in various plants, contributing to their growth characteristics. Improvement is obtained in the plant via microbial multiplication, and the production of abundant crops follows as a result of PGPR and AMF introduction, unlike uninoculated plants or plants introduced with only a PGPR or AMF strain alone (Cantabella et al., 2022). Moreover, the research investigating wheat plants by Zhang L.-L. et al. (2022) showed an absolute improvement of particular gluten protein fractions included in dough intensity and its elastic nature.
To improve the effectiveness of microbial biostimulants, various preparations constituting PGPMs and bioactive materials, like composts, protein hydrolysates, humic acids, plant exudates, agronomic or industrial materials produced, sewage, algae, and/or their extracts, were estimated.
Compost and compost products (vermicompost) are regarded as significant unprocessed materials for the production of biostimulants. Though they are considered biostimulants, the biologically active materials can be acquired from amino acids, phytohormones, humic substances, etc., which reveal fascinating features (Figure 1). Microbial communities inhabiting the soil are employed for producing biostimulants, and such examples of microbes include PGPRs and plant growth-promoting fungi (PGPFs), which may be either living or dead microbes, and the metabolites produced (Adedayo and Babalola, 2023). They can also be manufactured from extracts obtained from food wastes, aquaculture waste, composts, vermicompost, manure, animal wastes, etc. (Tolisano and Del Buono, 2023). Some ideas have been described that when PGPMs and compost were combined, they showed the potential to ensure a positive synergistic effect on the development of the plant (Table 2). A typical example of an advantageous factor was observed in plant growth in which upon introducing a halotolerant bacterial strain (Dietzia natronolimnaea) and AMFs, Glomus intraradices improved with compost product (Hossain et al., 2022). This effect has also been employed upon inoculation of AMF to green waste compost; after observation, a salinity tolerance on palm tree was observed, as verified by the improved K+/Na+ and Ca2+/Na+ proportion (Bello et al., 2021). The advantageous factors for plant growth were proposed and could be connected to the more effective acquisition of nutrients relinquished by compost as a result of AMF presence. Therefore, the development of nutrient uptake (K, Na, Ca, Mg, Al, and N) was observed when AMFs were introduced with compost but were less apparent once the plants were inoculated with compost or AMFs.
The formulation of biostimulants situated on amino acids is considered for their advantageous potential on the development of plants and crop yield levels, most importantly when stress occurs in the environment (Franzoni et al., 2022). Among the several features of amino acids are metal ion chelators, promoters of photosynthesis activity, anti-stress components, and metabolism of the hormone. Through the process of chemical or enzymatic hydrolysis, amino acids can be acquired from proteins of plants and/or animals.
Gowtham et al. (2022) explained that the application of PGPR strains (Bacillus cereus, B. pumilus, and Pseudomonas sp.) obtained from non-moisturized regions produces IAA, gibberellin (GA), and ABA content and reduced relative water content (RWC) (Malik et al., 2022). This process, promoted by the introduction of l-tryptophan, permits the preservation of a phytohormone proportion in dried environments, reducing the harmful activities of abiotic stress (Adeleke et al., 2022). Moreover, conservation of water was detected in the treatments in which PGPRs obtained from moisturized environments were introduced concerning tryptophan. Consequently, the dry weight of the root and shoot of the inoculated plants was greater, thereby proposing the development of these organs.
Biostimulant improvement activities on plants stimulated via synergistic association were likewise confirmed with AMFs and inorganic minor elements. An example is observed in rice plants; a greater yield was observed after the introduction of Rhizophagus clarus and silicon (Si) under normal and high-temperature environments (Etesami et al., 2022). The Si improved the colonization of AMF and the production of fungal structures; likewise, AMF improved the assimilation of Si by the plants. However, the high rate of Zn and Fe assimilation improved the formulation of the gene sequencing for enzymes included in the antioxidative defense system. Also, the assimilation of water and water use efficiency (WUE) may be associated with the activity of AMF and Si (Akensous et al., 2022). Most importantly, the assimilation of Fe and Zn can be affected by Si inoculation and can stimulate the upregulation of genes coding for IRT1 and IRT2, regarded as the Fe transporters classified to the Zrt/IRT-like protein (ZIP) family (Israel et al., 2022), which comprises Zn transporters. In contrast, the cellular oxidative condition can likewise be changed. This advantageous potential could result from the potential of Si to improve the stimulation of secondary metabolites or from the potential of the enzymes or gene expression of antioxidant enzymes, such as catalase, glutathione peroxidase, and superoxide dismutase.
The major problem encountered in the biostimulant market is unlimited usage. Despite agronomic inputs comprising a method of choosing, the proposed reason for biostimulant usage is sometimes direct. Chemical derivatives have been employed in the inhibition and total eradication of phytopathogens. The fertilizer market has a large number of alternatives to fertilizer accessible to farmers. Irrespective of the chemical fertilizer production site and selected means of usage, biostimulants are intensively employed to provide needed nutrients to encourage the developing plants to an ambitious stage of crop growth. Biostimulants are known to be different from other agronomic inputs and are in individual products irrespective of the desired response. Biostimulant application, for example, seaweed extract, to crop plants can introduce the microbial communities at the point of inoculation. In contrast, foliar application to vegetative growth stages is aimed at stimulating signal tracts to reduce abiotic stresses. Using biostimulants to promote the chosen product is another potential factor that farmers take. Chemical-derived fertilizers and pesticide markets are likewise gaining product options, and the major difference between the chemical derivatives and biostimulants is in its undefined lane. There are three features of product skillfulness, and they include little knowledge of the composition of the product and associated potential mechanisms and acquirable alternatives that left the biostimulant sale disorganized and modified for row crops. Moreover, the actual variability in season products enables farmers’ hesitance, and the successful application of biostimulants to farmland currently allows an instruction method that enables multiple seasons of fine-tuning for the successful employment of novel patterns.
The variability of information and demand for agricultural land observation is feasible. Biostimulants can promote crop and soil systems to adequately improve crop productivity. Attention has shifted to the potential of the fertilizer, which is presently the primary research method for biostimulants employed in crop systems, with the ability to increase the planting process and crop productivity, which is a result of effective nutrient utilization. Although some biostimulants are aimed at the application to the plantation of crops to improve their production, some products obtain these effects through their interaction with soils and their association with the plant root. Comparative observation of biostimulant impacts on the quality of soil and biological signals may show previously unidentified advantages of their introduction. The public authorities’ efforts are on orienting the public on agricultural systems and their potential to characterize the quality of water and manage nutrients, the application of biostimulants act as an alternative to greater sustainability of agriculture systems, and the promotion of soil quality that allows a feasible choice despite the lack of increased crop production.
AA: Data curation, Methodology, Writing – original draft, Writing – review & editing. OB: Conceptualization, Funding acquisition, Project administration, Supervision, Writing – review & editing.
The author(s) declare financial support was received for the research, authorship, and/or publication of this article. We acknowledge the National Research Foundation of South Africa grant (UID132595) for the funding support granted to OB.
The authors declare that the research was conducted in the absence of any commercial or financial relationships that could be construed as a potential conflict of interest.
All claims expressed in this article are solely those of the authors and do not necessarily represent those of their affiliated organizations, or those of the publisher, the editors and the reviewers. Any product that may be evaluated in this article, or claim that may be made by its manufacturer, is not guaranteed or endorsed by the publisher.
Abideen Z., Waqif H., Munir N., El-Keblawy A., Hasnain M., Radicetti E., et al. (2022). Algal-mediated nanoparticles, phycochar, and biofertilizers for mitigating abiotic stresses in plants: A review. Agron 12, 1788. doi: 10.3390/agronomy12081788
Adedayo A. A., Babalola O. O. (2023). Fungi that promote plant growth in the rhizosphere boost crop growth. J. Fungi 9, 239. doi: 10.3390/jof9020239
Adedayo A. A., Babalola O. O., Prigent-Combaret C., Cruz C., Stefan M., Kutu F., et al. (2022a). The application of plant growth-promoting rhizobacteria in Solanum lycopersicum production in the agricultural system: a review. PeerJ 10, e13405. doi: 10.7717/peerj.13405
Adedayo A. A., Fadiji A. E., Babalola O. O. (2022b). The effects of plant health status on the community structure and metabolic pathways of rhizosphere microbial communities associated with Solanum lycopersicum. Horticulturae 8, 404. doi: 10.3390/horticulturae8050404
Adedayo A. A., Fadiji A. E., Babalola O. O. (2022c). Plant health status affects the functional diversity of the rhizosphere microbiome associated with Solanum lycopersicum. Front. Sustain Food Syst. 6. doi: 10.3389/fsufs.2022.894312
Adedeji A. A., Häggblom M. M., Babalola O. O. (2020). Sustainable agriculture in Africa: Plant growth-promoting rhizobacteria (PGPR) to the rescue. Sci. Afr 9, e00492. doi: 10.1016/j.sciaf.2020.e00492
Adeleke B. S., Ayangbenro A. S., Babalola O. O. (2022). In vitro screening of sunflower associated endophytic bacteria with plant growth-promoting traits. Front. Sustain Food Syst. 6. doi: 10.3389/fsufs.2022.903114
Agbodjato N. A., Adoko M. Y., Babalola O. O., Amogou O., Badé F. T., Noumavo P. A., et al. (2021). Efficacy of biostimulants formulated with Pseudomonas putida and clay, peat, clay-peat binders on maize productivity in a farming environment in Southern Benin. Front. Sustain Food Syst. 5, 1–13. doi: 10.3389/fsufs.2021.666718
Agbodjato N. A., Assogba S. A., Babalola O. O., Koda A. D., Aguégué R. M., Sina H., et al. (2022). Formulation of biostimulants based on arbuscular mycorrhizal fungi for maize growth and yield. Front. Agron. 4, 1–15. doi: 10.3389/fagro.2022.894489
Ahmad A., Moin S. F., Liaqat I., Saleem S., Muhammad F., Mujahid T., et al. (2023). Isolation, solubilization of inorganic phosphate, and production of organic acids by individual and co-inoculated microorganisms. Geomicrobiol J. 40, 111–121. doi: 10.1080/01490451.2022.2124329
Akensous F.-Z., Anli M., Meddich A. (2022). Biostimulants as Innovative Tools to Boost Date Palm (Phoenix dactylifera L.) Performance under Drought, Salinity, and Heavy Metal (Oid)s ’ Stresses: A Concise Review. Sustainability 14, 15984. doi: 10.3390/su142315984
Ali S., Khan N. (2021). Delineation of mechanistic approaches employed by plant growth promoting microorganisms for improving drought stress tolerance in plants. Microbiol. Res. 249, 126771. doi: 10.1016/j.micres.2021.126771
Ali S., Moon Y.-S., Hamayun M., Khan M. A., Bibi K., Lee I.-J. (2022). Pragmatic role of microbial plant biostimulants in abiotic stress relief in crop plants. J. Plant Interact. 17, 705–718. doi: 10.1080/17429145.2022.2091801
Ali O., Ramsubhag A., Farrell A. D., Jayaraman J. (2022). Foliar application of seaweed extracts influences the phytomicrobiome dynamics in tomato and sweet pepper plants. J. Appl. Phycol 34, 3219–3235. doi: 10.1007/s10811-022-02823-x
Ali F., Wei X., Siddiqui Z. S., Chen J., Ansari H. H., Wajid D., et al. (2022). Scrutinizes the sustainable role of halophilic microbial strains on oxygen-evolving complex, specific energy fluxes, energy flow and nitrogen assimilation of sunflower cultivars in a suboptimal environment. Front. Plant Sci. 13. doi: 10.3389/fpls.2022.913825
Allam M., Radicetti E., Quintarelli V., Petroselli V., Marinari S., Mancinelli R. (2022). Influence of organic and mineral fertilizers on soil organic carbon and crop productivity under different tillage systems: A meta-analysis. Agriculture 12, 464. doi: 10.3390/agriculture12040464
Aloisi I., Piccini C., Cai G., Del Duca S. (2022). Male fertility under environmental stress: do polyamines act as pollen tube growth protectants? Int. J. Mol. Sci. 23, 1874. doi: 10.3390/ijms23031874
Alori E. T., Glick B. R., Babalola O. O. (2017). Microbial phosphorus solubilization and its potential for use in sustainable agriculture. Front. Microbiol. 8, 1–8. doi: 10.3389/fmicb.2017.00971
Ansari M., Devi B. M., Sarkar A., Chattopadhyay A., Satnami L., Balu P., et al. (2023). Microbial exudates as biostimulants: role in plant growth promotion and stress mitigation. J. Xenobiot 13, 572–603. doi: 10.3390/jox13040037
Armeli Minicante S., Bongiorni L., De Lazzari A. (2022). Bio-based products from mediterranean seaweeds: italian opportunities and challenges for a sustainable blue economy. Sustainability 14, 5634. doi: 10.3390/su14095634
Asghar W., Akça M. O., Akça H., Tarf O. J., Kataoka R., Turgay O. C. (2022). “Chapter 1 - Alternative strategies to synthetic chemical fertilizers: revitalization of soil quality for sustainable agriculture using organic-based approaches,” in New and future developments in microbial biotechnology and bioengineering. Eds. Singh H. B., Vaishnav A. (Elsevier), 1–30. doi: 10.1016/B978-0-323-85581-5.00003-3
Ayangbenro A. S., Chukwuneme C. F., Ayilara M. S., Kutu F. R., Khantsi M., Adeleke B. S., et al. (2022). Harnessing the rhizosphere soil microbiome of organically amended soil for plant productivity. Agron. 12, 3179. doi: 10.3390/agronomy12123179
Ayaz M., Ali Q., Jiang Q., Wang R., Wang Z., Mu G., et al. (2022). Salt tolerant bacillus strains improve plant growth traits and regulation of phytohormones in wheat under salinity stress. Plants 11, 2769. doi: 10.3390/plants11202769
Ayyaz A., Shahzadi A. K., Fatima S., Yasin G., Zafar Z. U., Athar H.-U.-R., et al. (2022). Uncovering the role of melatonin in plant stress tolerance. Theor. Exp. Plant Physiol. 34, 335–346. doi: 10.1007/s40626-022-00255-z
Bandyopadhyay P., Yadav B. G., Kumar S. G., Kumar R., Kogel K.-H., Kumar S. (2022). Piriformospora indica and Azotobacter chroococcum Consortium Facilitates Higher Acquisition of N, P with Improved Carbon Allocation and Enhanced Plant Growth in Oryza sativa. J. Fungi 8, 453. doi: 10.3390/jof8050453
Bartucca M. L., Cerri M., Del Buono D., Forni C. (2022). Use of biostimulants as a new approach for the improvement of phytoremediation performance & mdash; A review. Plants 11, 1946. doi: 10.3390/plants11151946
Bastianelli C., Pesante G., Ambrosini S., Zamboni A., Frison N. (2023). Upcycling of PHA-producing bacteria for biostimulants production and polyhydroxyalkanoates recovery. Sci. Total Environ. 888, 164238. doi: 10.1016/j.scitotenv.2023.164238
Bauters M., Janssens I. A., Wasner D., Doetterl S., Vermeir P., Griepentrog M., et al. (2022). Increasing calcium scarcity along Afrotropical forest succession. Nat. Ecol. Evol. 6, 1122–1131. doi: 10.1038/s41559-022-01810-2
Bello S. K., Alayafi A. H., Al-Solaimani S. G., Abo-Elyousr K. (2021). Mitigating soil salinity stress with gypsum and bio-organic amendments: A review. Agron 11, 1735. doi: 10.3390/agronomy11091735
Bender F. R., Alves L. C., Da Silva J. F. M., Ribeiro R. A., Pauli G., Nogueira M. A., et al. (2022). Microbiome of nodules and roots of soybean and common bean: searching for differences associated with contrasting performances in symbiotic nitrogen fixation. Int. J. Mol. Sci. 23, 12035. doi: 10.3390/ijms231912035
Benito P., Ligorio D., Bellón J., Yenush L., Mulet J. M. (2022). A fast method to evaluate in a combinatorial manner the synergistic effect of different biostimulants for promoting growth or tolerance against abiotic stress. Plant Methods 18, 111. doi: 10.1186/s13007-022-00943-6
Caicedo-Lopez L. H., Guevara-Gonzalez R. G., Ramirez-Jimenez A. K., Feregrino-Perez A. A., Contreras-Medina L. M. (2022). Eustress application trough-controlled elicitation strategies as an effective agrobiotechnology tool for capsaicinoids increase: a review. Phytochem. Rev. 21, 1941–1968. doi: 10.1007/s11101-022-09818-z
Camaille M., Fabre N., Clément C., Ait Barka E. (2021). Advances in wheat physiology in response to drought and the role of plant growth promoting rhizobacteria to trigger drought tolerance. Microorganisms 9, 687. doi: 10.3390/microorganisms9040687
Canellas L. P., Canellas N. O. A., Da Silva R. M., Spaccini R., Mota G. P., Olivares F. L. (2023). Biostimulants Using Humic Substances and Plant-Growth-Promoting Bacteria: Effects on Cassava (Manihot esculentus) and Okra (Abelmoschus esculentus) Yield. Agron 13, 80. doi: 10.3390/agronomy13010080
Cantabella D., Dolcet-Sanjuan R., Teixidó N. (2022). Using plant growth-promoting microorganisms (PGPMs) to improve plant development under in vitro culture conditions. Planta 255, 117. doi: 10.1007/s00425-022-03897-0
Cao M., Narayanan M., Shi X., Chen X., Li Z., Ma Y. (2023). Optimistic contributions of plant growth-promoting bacteria for sustainable agriculture and climate stress alleviation. Environ. Res. 217, 114924. doi: 10.1016/j.envres.2022.114924
Cardarelli M., Woo S. L., Rouphael Y., Colla G. (2022). Seed treatments with microorganisms can have a biostimulant effect by influencing germination and seedling growth of crops. Plants 11, 259. doi: 10.3390/plants11030259
Castiglione A. M., Mannino G., Contartese V., Bertea C. M., Ertani A. (2021). Microbial biostimulants as response to modern agriculture needs: composition, role and application of these innovative products. Plants 10, 1533. doi: 10.10.3390/plants10081533
Cayetano R. D. A., Kim G.-B., Park J., Yang Y.-H., Jeon B.-H., Jang M., et al. (2022). Biofilm formation as a method of improved treatment during anaerobic digestion of organic matter for biogas recovery. Bioresour Technol. 344, 126309. doi: 10.1016/j.biortech.2021.126309
Chandra P., Singh A., Prajapat K., Rai A. K., Yadav R. K. (2022). Native arbuscular mycorrhizal fungi improve growth, biomass yield, and phosphorus nutrition of sorghum in saline and sodic soils of the semi–arid region. Environ. Exp. Bot. 201, 104982. doi: 10.1016/j.envexpbot.2022.104982
Charatsari C., Lioutas E. D., Papadaki-Klavdianou A., Koutsouris A., Michailidis A. (2022). Experiential, social, connectivist, or transformative learning? Farm advisors and the construction of agroecological knowledge. Sustainability 14, 2426. doi: 10.3390/su14042426
Chitarra W., Pagliarani C., Maserti B., Lumini E., Siciliano I., Cascone P., et al. (2016). Insights on the impact of arbuscular mycorrhizal symbiosis on tomato tolerance to water stress. Plant Physiol. 171, 1009–1023. doi: 10.1104/pp.16.00307
Costa O. Y. A., Pijl A., Houbraken J., Van Lith W., Kuramae E. E. (2023). Soil substrate source drives the microbes involved in the degradation of gelatin used as a biostimulant. Appl. Soil Ecol. 189, 104906. doi: 10.1016/j.apsoil.2023.104906
Cué Rio M., Bovenkerk B., Castella J.-C., Fischer D., Fuchs R., Kanerva M., et al. (2022). The elephant in the room is really a cow: using consumption corridors to define sustainable meat consumption in the European Union. Sustain Sci., 1–19. doi: 10.1007/s11625-022-01235-7
Cui X., Zhang P., Chen C., Zhang J. (2022). VyUSPA3, a universal stress protein from the Chinese wild grape Vitis yeshanensis, confers drought tolerance to transgenic V. vinifera. Plant Cell Rep. 42, 181–196. doi: 10.1007/s00299-022-02943-1
Das P. P., Singh K. R. B., Nagpure G., Mansoori A., Singh R. P., Ghazi I. A., et al. (2022). Plant-soil-microbes: A tripartite interaction for nutrient acquisition and better plant growth for sustainable agricultural practices. Environ. Res. 214, 113821. doi: 10.1016/j.envres.2022.113821
Deolu-Ajayi A. O., van der Meer I. M., van der Werf A., Karlova R. (2022). The power of seaweeds as plant biostimulants to boost crop production under abiotic stress. Plant Cell Environ. 45, 2537–2553. doi: 10.1111/pce.14391
De Palma M., Scotti R., D’agostino N., Zaccardelli M., Tucci M. (2022). Phyto-friendly soil bacteria and fungi provide beneficial outcomes in the host plant by differently modulating its responses through (In)Direct mechanisms. Plants 11, 2672. doi: 10.3390/plants11202672
Dhankhar R., Gupta S., Gulati P. (2022). Insights on plant–microbe interactions in soil in relation to iron dynamics. Vegetos 36, 750–767. doi: 10.1007/s42535-022-00467-3
Díaz M., Bach T., González Anta G., Agaras B., Wibberg D., Noguera F., et al. (2022). Agronomic efficiency and genome mining analysis of the wheat-biostimulant rhizospheric bacterium Pseudomonas pergaminensis sp. nov. strain 1008(T). Front. Plant Sci. 13. doi: 10.3389/fpls.2022.894985
Diksha V., Parul C. (2023). “Types and function of phytohormone and their role in stress,” in Plant stress responses and defense mechanisms. Eds. Saddam H., Hussain A. T., Ejaz W., Masood Iqbal A. (Rijeka: IntechOpen). Ch. 1. doi: 10.5772/intechopen.109325
Dong L., He Z., Wu J., Zhang K., Zhang D., Pan X. (2023). Remediation of uranium-contaminated alkaline soil by rational application of phosphorus fertilizers: Effect and mechanism. Environ. Res. 220, 115172. doi: 10.1016/j.envres.2022.115172
Dong X., Zhang C., Peng Y., Zhang H.-X., Shi L.-D., Wei G., et al. (2022). Phylogenetically and catabolically diverse diazotrophs reside in deep-sea cold seep sediments. Nat. Commun. 13, 4885. doi: 10.1038/s41467-022-32503-w
Elhaissoufi W., Ghoulam C., Barakat A., Zeroual Y., Bargaz A. (2022). Phosphate bacterial solubilization: A key rhizosphere driving force enabling higher P use efficiency and crop productivity. J. Adv. Res. 38, 13–28. doi: 10.1016/j.jare.2021.08.014
Elnahal A. S. M., El-Saadony M. T., Saad A. M., Desoky E.-S. M., El-Tahan A. M., Rady M. M., et al. (2022). The use of microbial inoculants for biological control, plant growth promotion, and sustainable agriculture: A review. Eur. J. Plant Pathol. 162, 759–792. doi: 10.1007/s10658-021-02393-7
Emmanuel O. C., Akintola O. A., Tetteh F. M., Babalola O. O. (2021). Combined application of inoculant, phosphorus and potassium enhances cowpea yield in savanna soils. Agron 11, 15. doi: 10.3390/agronomy11010015
Emmanuel O. C., Babalola O. O. (2020). Productivity and quality of horticultural crops through co-inoculation of arbuscular mycorrhizal fungi and plant growth promoting bacteria. Microbiol. Res. 239, 126569. doi: 10.1016/j.micres.2020.126569
Enebe M. C., Babalola O. O. (2018). The influence of Plant Growth-Promoting Rhizobacteria in Plant tolerance to Abiotic Stress: A survival strategy. Appl. Microbiol. Biotechnol. 102, 7821–7835. doi: 10.1007/s00253-018-9214-z
Enebe M. C., Babalola O. O. (2021). The influence of soil fertilization on the distribution and diversity of phosphorus cycling genes and microbes community of maize rhizosphere using shotgun metagenomics. Genes 12, 1022. doi: 10.3390/genes12071022
Etesami H., Jeong B. R., Glick B. R. (2023). Potential use of Bacillus spp. as an effective biostimulant against abiotic stresses in crops—A review. Curr. Res. Biotechnol. 5, 100128. doi: 10.1016/j.crbiot.2023.100128
Etesami H., Li Z., Maathuis F. J. M., Cooke J. (2022). The combined use of silicon and arbuscular mycorrhizas to mitigate salinity and drought stress in rice. Environ. Exp. Bot. 201, 104955. doi: 10.1016/j.envexpbot.2022.104955
Fadel Sartori F., Dieminger Engroff T., Godoy Sanches T. H., Soave J. M., Victório Pessotto M., Felisberto G., et al. (2023). Potentially harmful effects of seed treatment and pre-inoculation on soybean biological nitrogen fixation and yield. Eur. J. Agron. 142, 126660. doi: 10.1016/j.eja.2022.126660
Fadiji A. E., Babalola O. O., Santoyo G., Perazzolli M. (2022a). The potential role of microbial biostimulants in the amelioration of climate change-associated abiotic stresses on crops. Front. Microbiol. 12, 1–17. doi: 10.3389/fmicb.2021.829099
Fadiji A. E., Santoyo G., Yadav A. N., Babalola O. O. (2022b). Efforts towards overcoming drought stress in crops: Revisiting the mechanisms employed by plant growth-promoting bacteria. Front. Microbiol. 13. doi: 10.3389/fmicb.2022.962427
Faria J. M. S., Pinto A. P., Teixeira D., Brito I., Carvalho M. (2022). Diversity of native arbuscular mycorrhiza extraradical mycelium influences antioxidant enzyme activity in wheat grown under Mn toxicity. Bull. Environ. Contam Toxicol. 108, 451–456. doi: 10.1007/s00128-021-03240-5
Fatima F., Ahmad M. M., Verma S. R., Pathak N. (2022). Relevance of phosphate solubilizing microbes in sustainable crop production: a review. Int. J. Environ. Sci. Technol. 19, 9283–9296. doi: 10.1007/s13762-021-03425-9
Feldmann F., Jehle J., Bradáčová K., Weinmann M. (2022). Biostimulants, soil improvers, bioprotectants: promoters of bio-intensification in plant production. J. Plant Dis. Prot 129, 707–713. doi: 10.1007/s41348-022-00567-x
Fiorilli V., Maghrebi M., Novero M., Votta C., Mazzarella T., Buffoni B., et al. (2022). Arbuscular mycorrhizal symbiosis differentially affects the nutritional status of two durum wheat genotypes under drought conditions. Plants 11, 804. doi: 10.3390/plants11060804
Franzoni G., Cocetta G., Prinsi B., Ferrante A., Espen L. (2022). Biostimulants on crops: their impact under abiotic stress conditions. Horticulturae 8, 189. doi: 10.3390/horticulturae8030189
Ganugi P., Fiorini A., Tabaglio V., Capra F., Zengin G., Bonini P., et al. (2023). The functional profile and antioxidant capacity of tomato fruits are modulated by the interaction between microbial biostimulants, soil properties, and soil nitrogen status. Antioxidants 12, 520. doi: 10.3390/antiox12020520
Garbin E., Cargnelutti D., Engel C. L., De Sá K. R., Galon L., Müller C., et al. (2022). Morphophysiological aspects of bean plants cultivated with natural reactive phosphate and solubilizing and growth promoting microorganisms. Sci. Electron Arch. 15 (9), 1–8. doi: 10.36560/15920221583
Ghifari A. S., Teixeira P. F., Kmiec B., Singh N., Glaser E., Murcha M. W. (2022). The dual-targeted prolyl aminopeptidase PAP1 is involved in proline accumulation in response to stress and during pollen development. J. Exp. Bot. 73, 78–93. doi: 10.1093/jxb/erab397
Gong J., Zhang Z., Zhu C., Shi J., Zhang W., Song L., et al. (2022). The response of litter decomposition to phosphorus addition in typical temperate grassland in Inner Mongolia. J. Arid Environ. 197, 104677. doi: 10.1016/j.jaridenv.2021.104677
Gowtham H. G., Singh S. B., Shilpa N., Aiyaz M., Nataraj K., Udayashankar A. C., et al. (2022). Insight into Recent Progress and Perspectives in Improvement of Antioxidant Machinery upon PGPR Augmentation in Plants under Drought Stress: A Review. Antioxidants 11, 1763. doi: 10.3390/antiox11091763
Gupta A., Nair S. (2020). Dynamics of insect–microbiome interaction influence host and microbial symbiont. Front. Microbiol. 11, 1357. doi: 10.3389/fmicb.2020.01357
Gutknecht J., Journey A., Peterson H., Blair H., Cates A. (2022). Cover crop management practices to promote soil health and climate adaptation: Grappling with varied success from farmer and researcher observations. J. Environ. Qual, 1–17. doi: 10.1002/jeq2.20383
Hakimi S., Kari N. M., Ismail N., Ismail M. N., Ahmad F. (2022). Evaluation of taste active peptides and amino acids from anchovy proteins in fish sauce by in silico approach. Food Sci. Biotechnol. 31, 767–785. doi: 10.1007/s10068-022-01097-w
Hamid B., Zaman M., Farooq S., Fatima S., Sayyed R. Z., Baba Z. A., et al. (2021). Bacterial plant biostimulants: A sustainable way towards improving growth, productivity, and health of crops. Sustainability 13, 2856. doi: 10.3390/su13052856
Hao J., Tan J., Zhang Y., Wang S., Zhang X., Wang Z., et al. (2023). Metabolomics reveals the molecular mechanism of sewage sludge-derived nutrients and biostimulants stimulating resistance enhancement and the redistribution of carbon and nitrogen metabolism in pakchoi cabbage. Sci. Total Environ. 891, 164330. doi: 10.1016/j.scitotenv.2023.164330
Hossain A., Ali M. E., Maitra S., Bhadra P., Rahman M. M. E., Ali S., et al. (2022). “Chapter 13 - The role of soil microorganisms in plant adaptation to abiotic stresses: Current scenario and future perspectives,” in Plant perspectives to global climate changes. Eds. Aftab T., Roychoudhury A. (Switzerland: Academic Press), 233–278. doi: 10.1016/B978-0-323-85665-2.00001-7
Ibarra-Galeana J. A., Castro-Martínez C., Fierro-Coronado R. A., Armenta-Bojórquez A. D., Maldonado-Mendoza I. E. (2017). Characterization of phosphate-solubilizing bacteria exhibiting the potential for growth promotion and phosphorus nutrition improvement in maize (Zea mays L.) in calcareous soils of Sinaloa, Mexico. Ann. Microbiol. 67, 801–811. doi: 10.1007/s13213-017-1308-9
Israel A., Langrand J., Fontaine J., Lounès-Hadj Sahraoui A. (2022). Significance of arbuscular mycorrhizal fungi in mitigating abiotic environmental stress in medicinal and aromatic plants: A review. Foods 11, 2591. doi: 10.3390/foods11172591
Johnson R., Joel J. M., Puthur J. T. (2023). Biostimulants: the futuristic sustainable approach for alleviating crop productivity and abiotic stress tolerance. J. Plant Growth Regul., 1–16. doi: 10.1007/s00344-023-11144-3
Jolayemi O. L., Malik A. H., Ekblad T., Fredlund K., Olsson M. E., Johansson E. (2022). Protein-based biostimulants to enhance plant growth—State-of-the-art and future direction with sugar beet as an example. Agron 12, 3211. doi: 10.3390/agronomy12123211
Karimi G., Pourakbar L., Siavash Moghaddam S., Rezaee Danesh Y., Popovi´C-Djordjevi´ C. ,. J. (2022). Effectiveness of fungal bacterial biofertilizers on agrobiochemical attributes of quinoa (Chenopodium quinoa willd.) under salinity stress. Int. J. Environ. Sci. Technol. 19, 11989–12002. doi: 10.1007/s13762-022-04427-x
Kashyap S., Barman R., Nath M., Agarwala N. (2023). “16 - Microbial symbionts for alleviation of heavy metal toxicity in crop plants,” in Biostimulants in alleviation of metal toxicity in plants. Eds. Gill S. S., Tuteja N., Khan N. A., Gill R. (Switzerland: Academic Press), 371–400. doi: 10.1016/B978-0-323-99600-6.00009-8
Khajeeyan R., Salehi A., Movahhedi Dehnavi M., Farajee H., Kohanmoo M. A. (2022). Growth parameters, water productivity and aloin content of Aloe vera affected by mycorrhiza and PGPR application under different irrigation regimes. S Afr J. Bot. 147, 1188–1198. doi: 10.1016/j.sajb.2021.02.026
Khaliq A., Perveen S., Alamer K. H., Zia Ul Haq M., Rafique Z., Alsudays I. M., et al. (2022). Arbuscular mycorrhizal fungi symbiosis to enhance plant–soil interaction. Sustainability 14, 7840. doi: 10.3390/su14137840
Kisvarga S., Farkas D., Boronkay G., Neményi A., Orlóci L. (2022). Effects of biostimulants in horticulture, with emphasis on ornamental plant production. Agron 12, 1043. doi: 10.3390/agronomy12051043
Koner S., Tsai H.-C., Chen J.-S., Hussain B., Rajendran S. K., Hsu B.-M. (2022). Exploration of pristine plate-tectonic plains and mining exposure areas for indigenous microbial communities and its impact on the mineral-microbial geochemical weathering process in ultramafic setting. Environ. Res. 214, 113802. doi: 10.1016/j.envres.2022.113802
Kou B., Hui K., Miao F., He Y., Qu C., Yuan Y., et al. (2022). Differential responses of the properties of soil humic acid and fulvic acid to nitrogen addition in the North China Plain. Environ. Res. 214, 113980. doi: 10.1016/j.envres.2022.113980
Koza N. A., Adedayo A. A., Babalola O. O., Kappo A. P. (2022). Microorganisms in plant growth and development: roles in abiotic stress tolerance and secondary metabolites secretion. Microorganisms 10, 1528. doi: 10.3390/microorganisms10081528
Kreutz G. F., Bhadha J. H., Sandoya G. V. (2022). Examining phosphorus use efficiency across different lettuce (Lactuca sativa L.) accessions. Euphytica 218, 28. doi: 10.1007/s10681-022-02973-6
Krutyakov Y. A., Mukhina M. T., Shapoval O. A., Zargar M. (2022). Effect of foliar treatment with aqueous dispersions of silver nanoparticles on legume-rhizobium symbiosis and yield of soybean (Glycine max L. Merr.). Agron 12, 1473. doi: 10.3390/agronomy12061473
Kumar U., Cheng M., Islam M. J., Maniruzzaman M., Nasreen S. S., Haque M. E., et al. (2022). Long-term Conservation Agriculture increases sulfur pools in soils together with increased soil organic carbon compared to conventional practices. Soil Tillage Res. 223, 105474. doi: 10.1016/j.still.2022.105474
Kumari M., Swarupa P., Kesari K. K., Kumar A. (2022). Microbial inoculants as plant biostimulants: A review on risk status. Life 13, 12. doi: 10.3390/life13010012
Lan Y., Liao L., Yao X., Ye S. (2022). Synergistic effects of nitrogen and plant growth-promoting rhizobacteria inoculation on the growth, physiological traits and nutrient absorption of intercropped Eucalyptus urophylla × Eucalyptus grandis and Dalbergia odorifera. Trees 37, 319–330. doi: 10.1007/s00468-022-02350-9
Lee J. W., Bhagwat S. S., Kuanyshev N., Cho Y. B., Sun L., Lee Y.-G., et al. (2023). Rewiring yeast metabolism for producing 2,3-butanediol and two downstream applications: Techno-economic analysis and life cycle assessment of methyl ethyl ketone (MEK) and agricultural biostimulant production. J. Chem. Eng. 451, 138886. doi: 10.1016/j.cej.2022.138886
Liebrenz K., Gómez C., Brambilla S., Frare R., Stritzler M., Maguire V., et al. (2022). Whole-genome resequencing of spontaneous oxidative stress-resistant mutants reveals an antioxidant system of Bradyrhizobium japonicum involved in soybean colonization. Microb. Ecol. 84, 1133–1140. doi: 10.1007/s00248-021-01925-2
Liptzin D., Norris C. E., Cappellazzi S. B., Bean G. M., Cope M., Greub K. L. H., et al. (2022). An evaluation of carbon indicators of soil health in long-term agricultural experiments. Soil Biol. Biochem. 172, 108708. doi: 10.1016/j.soilbio.2022.108708
Liu C.-Y., Zhang F., Zhang D.-J., Srivastava A. K., Wu Q.-S., Zou Y.-N. (2018). Mycorrhiza stimulates root-hair growth and IAA synthesis and transport in trifoliate orange under drought stress. Sci. Rep. 8, 1978. doi: 10.1038/s41598-018-20456-4
Lopez-Cantu D. O., González-González R. B., Melchor-Martínez E. M., Martínez S., Araújo R. G., Parra-Arroyo L., et al. (2022). Enzyme-mimicking capacities of carbon-dots nanozymes: Properties, catalytic mechanism, and applications – A review. Int. J. Biol. Macromol 194, 676–687. doi: 10.1016/j.ijbiomac.2021.11.112
Lv J., Huang Z., Luo L., Zhang S., Wang Y. (2022). Advances in molecular and microscale characterization of soil organic matter: current limitations and future prospects. Environ. Sci. Technol. 56, 12793–12810. doi: 10.1021/acs.est.2c00421
Mackiewicz-Walec E., Olszewska M. (2023). Biostimulants in the production of forage grasses and turfgrasses. Agriculture 13, 1796. doi: 10.3390/agriculture13091796
Magnabosco P., Masi A., Shukla R., Bansal V., Carletti P. (2023). Advancing the impact of plant biostimulants to sustainable agriculture through nanotechnologies. Chem. Biol. Technol. Agric. 10, 117. doi: 10.1186/s40538-023-00491-8
Maitra S., Brestic M., Bhadra P., Shankar T., Praharaj S., Palai J. B., et al. (2022). Bioinoculants—Natural biological resources for sustainable plant production. Microorganisms 10, 51. doi: 10.10.3390/microorganisms10010051
Maity D., Gupta U., Saha S. (2022). Biosynthesized metal oxide nanoparticles for sustainable agriculture: next-generation nanotechnology for crop production, protection and management. Nanoscale 14, 13950–13989. doi: 10.1039/d2nr03944c
Malgioglio G., Rizzo G. F., Nigro S., Lefebvre Du Prey V., Herforth-Rahmé J., Catara V., et al. (2022). Plant-microbe interaction in sustainable agriculture: the factors that may influence the efficacy of PGPM application. Sustainability 14, 2253. doi: 10.3390/su14042253
Malik A., Punia H., Singh N., Singh P. (2022). “Chapter 3 - Bionanomaterials-mediated seed priming for sustainable agricultural production,” in Bionanotechnology : emerging applications of bionanomaterials. Eds. Barhoum A., Jeevanandam J., Danquah M. K. (Elsevier), 77–99. doi: 10.1016/B978-0-12-823915-5.00008-3
Mandal S., Anand U., López-Bucio J., Radha, Kumar M., Lal M. K., et al. (2023). Biostimulants and environmental stress mitigation in crops: A novel and emerging approach for agricultural sustainability under climate change. Environ. Res. 233, 116357. doi: 10.1016/j.envres.2023.116357
Masowa M. M., Dlamini P., Babalola O. O., Mulidzi A. R., Kutu F. R. (2023). In-field assessment of soil pH and mineralization of phosphorus and potassium following the application of composted winery solid waste in sandy loam Ferric Luvisol. Emir J. Food Agric. 35. doi: 10.9755/ejfa.2023.3120
Mattedi A., Sabbi E., Farda B., Djebaili R., Mitra D., Ercole C., et al. (2023). Solid-state fermentation: applications and future perspectives for biostimulant and biopesticides production. Microorganisms 11. doi: 10.3390/microorganisms11061408
Meddich A. (2022). Biostimulants for resilient agriculture—Improving plant tolerance to abiotic stress: A concise review. Gesunde Pflanzen 75, 709–727. doi: 10.1007/s10343-022-00784-2
Melissa O., Cristian A., Sheila C., Vanina M., Daniela T., Gabriela P., et al. (2022). Evaluation of nitrous oxide emission by soybean inoculated with Bradyrhizobium strains commonly used as inoculants in South America. Plant Soil 472, 311–328. doi: 10.1007/s11104-021-05242-y
Missaoui T., Boughdiri N., Chemingui H., Al Sobeai S. M., Smiri M. (2023). Growth and metabolism of pea (Pisum sativum) via biostimulants based on greener ZnO nanoparticles. Int. J. Phytoremediation, 1–9. doi: 10.1080/15226514.2023.2265504
Montoneri E., Baglieri A., Fascella G. (2022). Biostimulant effects of waste derived biobased products in the cultivation of ornamental and food plants. Agriculture 12(7), 944. doi: 10.3390/agriculture12070994
Munir N., Hanif M., Abideen Z., Sohail M., El-Keblawy A., Radicetti E., et al. (2022). Mechanisms and strategies of plant microbiome interactions to mitigate abiotic stresses. Agron 12(9), 2069. doi: 10.3390/agronomy12092069
Muresu R., Porceddu A., Concheri G., Stevanato P., Squartini A. (2022). Legumes of the sardinia island: knowledge on symbiotic and endophytic bacteria and interactive software tool for plant species determination. Plants 11, 1521. doi: 10.3390/plants11111521
Naderi K., Etesami H., Alikhani H. A., Mosleh Arani A. (2022). Potential use of endophytic and rhizosheath bacteria from the desert plant Stipagrostis pennata as biostimulant against drought in wheat cultivars. Rhizosphere 24, 100617. doi: 10.1016/j.rhisph.2022.100617
Navarro-León E., Borda E., Marín C., Sierras N., Blasco B., Ruiz J. M. (2022). Application of an enzymatic hydrolysed L-& alpha;-amino acid based biostimulant to improve sunflower tolerance to imazamox. Plants 11, 2761. doi: 10.3390/plants11202761
Ojuederie O. B., Babalola O. O. (2017). Microbial and plant-assisted bioremediation of heavy metal polluted environments: A review. Int. J. Environ. Res. Public Health 14, 1504. doi: 10.3390/ijerph14121504
Olowe O. M., Nicola L., Asemoloye M. D., Akanmu A. O., Babalola O. O. (2022). Trichoderma: Potential bio-resource for the management of tomato root rot diseases in Africa. Microbiol. Res. 257, 126978. doi: 10.1016/j.micres.2022.126978
Orozco-Mosqueda M. D. C., Fadiji A. E., Babalola O. O., Glick B. R., Santoyo G. (2022). Rhizobiome engineering: Unveiling complex rhizosphere interactions to enhance plant growth and health. Microbiol. Res. 263, 127137. doi: 10.1016/j.micres.2022.127137
Pandit A., Johny L., Srivastava S., Adholeya A., Cahill D., Brau L., et al. (2022). Recreating in vitro tripartite mycorrhizal associations through functional bacterial biofilms. Appl. Microbiol. Biotechnol. 106, 4237–4250. doi: 10.1007/s00253-022-11996-x
Paravar A., Piri R., Balouchi H., Ma Y. (2023). Microbial seed coating: An attractive tool for sustainable agriculture. Biotechnol. Rep. 37, e00781. doi: 10.1016/j.btre.2023.e00781
Patra D., Mandal S. (2022). Non-rhizobia are the alternative sustainable solution for growth and development of the nonlegume plants. Biotechnol. Genet. Eng. Rev., 1–30. doi: 10.1080/02648725.2022.2152623
Peng Y., Zhang B., Guan C.-Y., Jiang X., Tan J., Li X. (2022). Identifying biotic and abiotic processes of reversing biochar-induced soil phosphorus leaching through biochar modification with MgAl layered (hydr)oxides. Sci. Total Environ. 843, 157037. doi: 10.1016/j.scitotenv.2022.157037
Pikuła D., Ciotucha O. (2022). The Composition of the Organic Matter Fractions of Loamy Sand after Long-Term FYM Application without Liming. Agron 12, 2385. doi: 10.3390/agronomy12102385
Pourjafar H., Ansari F., Sadeghi A., Samakkhah S. A., Jafari S. M. (2022). Functional and health-promoting properties of probiotics’ exopolysaccharides; isolation, characterization, and applications in the food industry. Crit. Rev. Food Sci. Nutr., 8194–8225. doi: 10.1080/10408398.2022.2047883
Prabhukarthikeyan S. R., Keerthana U., Baite M. S., Panneerselvam P., Mitra D., Naveen Kumar R., et al. (2022). “CHAPTER 3 - Bacillus rhizobacteria: A versatile biostimulant for sustainable agriculture,” in New and future developments in microbial biotechnology and bioengineering. Eds. Singh H., Vaishnav A. (Elsevier), 33–44. doi: 10.1016/B978-0-323-85163-3.00009-0
Rakkammal K., Maharajan T., Ceasar S. A., Ramesh M. (2022). Biostimulants and their role in improving plant growth under drought and salinity. Cereal Res. Commun. 51, 61–74. doi: 10.1007/s42976-022-00299-6
Rasse D. P., Weldon S., Joner E. J., Joseph S., Kammann C. I., Liu X., et al. (2022). Enhancing plant N uptake with biochar-based fertilizers: limitation of sorption and prospects. Plant Soil 475, 213–236. doi: 10.1007/s11104-022-05365-w
Renaud C., Leys N., Wattiez R. (2023). Photosynthetic microorganisms, an overview of their biostimulant effects on plants and perspectives for space agriculture. J. Plant Interact. 18, 2242697. doi: 10.1080/17429145.2023.2242697
Richardson A. E., George T. S., Hens M., Delhaize E., Ryan P. R., Simpson R. J., et al. (2022). Organic anions facilitate the mobilization of soil organic phosphorus and its subsequent lability to phosphatases. Plant Soil 476, 161–180. doi: 10.1007/s11104-022-05405-5
Sakthieaswari P., Kannan A., Baby S. (2022). “Chapter 14 - Role of mycorrhizosphere as a biostimulant and its impact on plant growth, nutrient uptake and stress management,” in New and future developments in microbial biotechnology and bioengineering. Eds. Singh H. B., Vaishnav A. (Elsevier), 319–336. doi: 10.1016/B978-0-323-85577-8.00010-X
Salhi L. N., Bustamante Villalobos P., Forget L., Burger G., Lang B. F. (2022). Endosymbionts in cranberry: Diversity, effect on plant growth, and pathogen biocontrol. PLANTS PEOPLE PLANET 4, 511–522. doi: 10.1002/ppp3.10290
Samanta S. (2022). Structural and catalytical features of different amylases and their potential applications. Jordan J. Biol. Sci. 15, 311–337. doi: 10.54319/jjbs/150220
Samuels L. J., Setati M. E., Blancquaert E. H. (2022). Towards a better understanding of the potential benefits of seaweed based biostimulants in Vitis vinifera L. Cultivars. Plants 11, 348. doi: 10.3390/plants11030348
Saranya K., Sundaramanickam A., Manupoori S., Kanth S. V. (2022). Screening of multi-faceted phosphate-solubilising bacterium from seagrass meadow and their plant growth promotion under saline stress condition. Microbiol. Res. 261, 127080. doi: 10.1016/j.micres.2022.127080
Sarmah R., Sarma A. K. (2022). Phosphate solubilizing microorganisms: A review. Commun. Soil Sci. Plant Anal., 1306–1315. doi: 10.1080/00103624.2022.2142238
Savarese C., Cozzolino V., Verrillo M., Vinci G., De Martino A., Scopa A., et al. (2022). Combination of humic biostimulants with a microbial inoculum improves lettuce productivity, nutrient uptake, and primary and secondary metabolism. Plant Soil, 1306–1315. doi: 10.1007/s11104-022-05634-8
Sayahi N., Djemal R., Ben Merdes K., Saidii M. N., Yengui M., Gdoura R., et al. (2022). Characterization of siccibacter sp. Strain C2 a novel rhizobacterium that enhances tolerance of barley to salt stress. Curr. Microbiol. 79, 239. doi: 10.1007/s00284-022-02930-5
Scavo A., Fontanazza S., Restuccia A., Pesce G. R., Abbate C., Mauromicale G. (2022). The role of cover crops in improving soil fertility and plant nutritional status in temperate climates. A review. Agron. Sustain Dev. 42, 93. doi: 10.1007/s13593-022-00825-0
Schmitzer V., Stampar F., Turk A., Jakopic J., Hudina M., Veberic R., et al. (2023). Before or after Planting? Mycorrhizal and Bacterial Biostimulants and Extracts in Intense Strawberry (Fragaria × ananassa Duch.) Production. Horticulturae 9, 769. doi: 10.3390/horticulturae9070769
Sible C. N., Seebauer J. R., Below F. E. (2021). Plant biostimulants: A categorical review, their implications for row crop production, and relation to soil health indicators. Agron 11, 1297. doi: 10.3390/agronomy11071297
Silva C. R., Miller R. M., Pereira B. C., Aveleda L., Marin V. A. (2022). Genomic analysis and plant growth-promoting potential of a Serratia marcescens isolated from food. Res. Soc. Dev. 11, e29611124799–e29611124799. doi: 10.33448/rsd-v11i1.24799
Singh H., Bhat J. A., Singh V. P., Corpas F. J., Yadav S. R. (2021). Auxin metabolic network regulates the plant response to metalloids stress. J. Hazard Mater 405, 124250. doi: 10.1016/j.jhazmat.2020.124250
Sujeeth N., Petrov V., Guinan K. J., Rasul F., O’sullivan J. T., Gechev T. S. (2022). Current insights into the molecular mode of action of seaweed-based biostimulants and the sustainability of seaweeds as raw material resources. Int. J. Mol. Sci. 23, 7654. doi: 10.3390/ijms23147654
Sundara B., Natarajan V., Hari K. (2002). Influence of phosphorus solubilizing bacteria on the changes in soil available phosphorus and sugarcane and sugar yields. Field Crops Res. 77, 43–49. doi: 10.1016/S0378-4290(02)00048-5
Tang Y., Zhang X., Wang H., Meng S., Yang F., Chen F., et al. (2022). Warming causes variability in SOM decomposition in N-and P-fertiliser-treated soil in a subtropical coniferous forest. Eur. J. Soil Sci. 73, e13320. doi: 10.1111/ejss.13320
Tariq M. R., Shaheen F., Mustafa S., Ali S., Fatima A., Shafiq M., et al. (2022). Phosphate solubilizing microorganisms isolated from medicinal plants improve growth of mint. PeerJ 10, e13782. doi: 10.7717/peerj.13782
Tolisano C., Del Buono D. (2023). Biobased: Biostimulants and biogenic nanoparticles enter the scene. Sci. Total Environ. 885, 163912. doi: 10.1016/j.scitotenv.2023.163912
Turan M., Gulluce M., Von Wirén N., Sahin F. (2012). Yield promotion and phosphorus solubilization by plant growth–promoting rhizobacteria in extensive wheat production in Turkey. J. Plant Nutr. Soil Sci. 175, 818–826. doi: 10.1002/jpln.201200054
Tyśkiewicz R., Nowak A., Ozimek E., Jaroszuk-Ściseł J. (2022). Trichoderma: the current status of its application in agriculture for the biocontrol of fungal phytopathogens and stimulation of plant growth. Int. J. Mol. Sci. 23, 2329. doi: 10.3390/ijms23042329
Uroz S., Picard L., Turpault M.-P. (2022). Recent progress in understanding the ecology and molecular genetics of soil mineral weathering bacteria. Trends Microbiol. 30, 882–897. doi: 10.1016/j.tim.2022.01.019
van der Sloot M., Kleijn D., De Deyn G. B., Limpens J. (2022). Carbon to nitrogen ratio and quantity of organic amendment interactively affect crop growth and soil mineral N retention. Crop Environ. 1, 161–167. doi: 10.1016/j.crope.2022.08.001
Velichko N. S., Bagavova A. R., Burygin G. L., Baymiev A. K., Pylaev T. E., Fedonenko Y. P. (2022). In situ localization and penetration route of an endophytic bacteria into roots of wheat and the common bean. Rhizosphere 23, 100567. doi: 10.1016/j.rhisph.2022.100567
Vermeiren C., Kerckhof P., Reheul D., Smolders E. (2022). Increasing soil organic carbon content can enhance the long-term availability of phosphorus in agricultural soils. Eur. J. Soil Sci. 73, e13191. doi: 10.1111/ejss.13191
Wang D., Saleh N. B., Byro A., Zepp R., Sahle-Demessie E., Luxton T. P., et al. (2022). Nano-enabled pesticides for sustainable agriculture and global food security. Nat. Nanotechnol 17, 347–360. doi: 10.1038/s41565-022-01082-8
Wang Y., Sun Q., Liu J., Wang L., Wu X., Zhao Z., et al. (2022). Suaeda salsa Root-Associated Microorganisms Could Effectively Improve Maize Growth and Resistance under Salt Stress. Microbiol. Spectr. 10, e01349–e01322. doi: 10.1128/spectrum.01349-22
Wang H.-R., Zhao X.-Y., Zhang J.-M., Lu C., Feng F.-J. (2022). Arbuscular mycorrhizal fungus regulates cadmium accumulation, migration, transport, and tolerance in Medicago sativa. J. Hazard Mater 435, 129077. doi: 10.1016/j.jhazmat.2022.129077
Wood M. J., Kortsinoglou A. M., Khoja S., Kouvelis V. N., Myrta A., Midthassel A., et al. (2022). Metarhizium brunneum (Hypocreales: clavicipitaceae) and its derived volatile organic compounds as biostimulants of commercially valuable angiosperms and gymnosperms. J. Fungi 8, 1052. doi: 10.3390/jof8101052
Wozniak E., Blaszczak A., Wiatrak P., Canady M. (2020). Biostimulant mode of action: impact of biostimulant on whole-plant level. Chem. Biol. Plant Biostimulants, 205–227. doi: 10.1002/9781119357254.ch8
Xi M., Deyett E., Ginnan N., Ashworth V. E. T. M., Dang T., Bodaghi S., et al. (2022). Geographic location, management strategy, and huanglongbing disease affect arbuscular mycorrhizal fungal communities across US citrus orchards. Phytobiomes J. 6, 342–353. doi: 10.1094/PBIOMES-03-22-0014-R
Xiang L., Harindintwali J. D., Wang F., Redmile-Gordon M., Chang S. X., Fu Y., et al. (2022). Integrating biochar, bacteria, and plants for sustainable remediation of soils contaminated with organic pollutants. Environ. Sci. Technol. 56, 16546–16566. doi: 10.1021/acs.est.2c02976
Xin Y., Fan Y., Babalola O. O., Zhang X., Yang W. (2022). Legacy effects of biochar and compost addition on arbuscular mycorrhizal fungal community and co-occurrence network in black soil. Microorganisms 10, 2137. doi: 10.10.3390/microorganisms10112137
Yadav A., Yadav K., Abd-Elsalam K. A. (2023). Nanofertilizers: types, delivery and advantages in agricultural sustainability. Agrochemicals 2, 296–336. doi: 10.3390/agrochemicals2020019
Yin J., Sui Z., Yuan L., Huang J. (2022). A profitable function of Ceriporia lacerata HG2011 on nodulation and biological nitrogen fixation of green bean (Phaseolus vulgaris L.). J. Appl. Microbiol. 134(1), lxac025. doi: 10.1093/jambio/lxac025
Yuan Y., Gai S., Tang C., Jin Y., Cheng K., Antonietti M., et al. (2022). Artificial humic acid improves maize growth and soil phosphorus utilization efficiency. Appl. Soil Ecol. 179, 104587. doi: 10.1016/j.apsoil.2022.104587
Yuan S., Huang J., Jiang X., Huang Y., Zhu X., Cai Z. (2022). Environmental fate and toxicity of sunscreen-derived inorganic ultraviolet filters in aquatic environments: A review. Nanomaterials 12, 104587. doi: 10.3390/nano12040699
Zanli B. L. G. L., Gbossou K. C., Tang W., Kamoto M., Chen J. (2022). A review of biochar potential in Cote d’Ivoire in light of the challenges facing Sub-Saharan Africa. Biomass Bioenergy 165, 106581. doi: 10.1016/j.biombioe.2022.106581
Zhang Q., Kingsley K. L., White J. F. (2022). Endophytic Pseudomonas sp. from Agave palmeri Participate in the Rhizophagy Cycle and Act as Biostimulants in Crop Plants. Biology 11. doi: 10.3390/biology11121790
Zhang L.-L., Li M.-M., Guan E.-Q., Yang Y.-L., Zhang T.-J., Liu Y.-X., et al. (2022). Interactions between wheat globulin and gluten under alkali or salt condition and its effects on noodle dough rheology and end quality. Food Chem. 382, 132310. doi: 10.1016/j.foodchem.2022.132310
Zhao D., Ding Y., Cui Y., Zhang Y., Liu K., Yao L., et al. (2022). Isolation and genome sequence of a novel phosphate-solubilizing rhizobacterium Bacillus altitudinis GQYP101 and its effects on rhizosphere microbial community structure and functional traits of corn seedling. Curr. Microbiol. 79, 249. doi: 10.1007/s00284-022-02944-z
Keywords: abiotic stress, environment, soil degradation, microbial biostimulant, PGPM, soil biodiversity, synergism
Citation: Adedayo AA and Babalola OO (2023) The potential of biostimulants on soil microbial community: a review. Front. Ind. Microbiol. 1:1308641. doi: 10.3389/finmi.2023.1308641
Received: 06 October 2023; Accepted: 24 November 2023;
Published: 13 December 2023.
Edited by:
Marcelo Carvalho Minhoto Teixeira Filho, São Paulo State University, BrazilReviewed by:
Rihab Djebaili, Consorzio Interuniversitario Nazionale per le Scienze Ambientali, ItalyCopyright © 2023 Adedayo and Babalola. This is an open-access article distributed under the terms of the Creative Commons Attribution License (CC BY). The use, distribution or reproduction in other forums is permitted, provided the original author(s) and the copyright owner(s) are credited and that the original publication in this journal is cited, in accordance with accepted academic practice. No use, distribution or reproduction is permitted which does not comply with these terms.
*Correspondence: Olubukola Oluranti Babalola, b2x1YnVrb2xhLmJhYmFsb2xhQG53dS5hYy56YQ==
†ORCID: Afeez Adesina Adedayo, orcid.org/0000-0001-5388-2877
Olubukola Oluranti Babalola, orcid.org/0000-0003-4344-1909
Disclaimer: All claims expressed in this article are solely those of the authors and do not necessarily represent those of their affiliated organizations, or those of the publisher, the editors and the reviewers. Any product that may be evaluated in this article or claim that may be made by its manufacturer is not guaranteed or endorsed by the publisher.
Research integrity at Frontiers
Learn more about the work of our research integrity team to safeguard the quality of each article we publish.