- Stantec, North Bay, ON, Canada
Environmental, social, and governance (ESG) focus continues to gain traction in the mining industry through publicly made policies, promises, and commitments. In 2022, both ESG and technological investments were identified in the top trends by Deloitte and in the top risks and opportunities by Ernst and Young. As the first step in the value chain, the mining industry sets the foundation for most industries in meeting their ESG targets. Beyond providing sustainable materials, the mining industry is required to produce the critical minerals needed for the creation of sustainable technologies. With an ongoing debate on how ESG factors should be measured and inconsistent reporting between mining companies, there remains a gap in consistent and auditable progress in ESG reporting. This study evaluates the application of a digital twin technology to bridge the gap in ESG reporting. By examining the use of digital twin technology through thirty case studies and theoretical applications across industries that share commonalities with mining, this study analyzes the opportunity to apply the technology to the mining industry. The research found that digital twin technology can be applied across all mining project phases and can provide added value to improve multiple ESG factors and measure them. Though the research identifies that there are benefits from the application of digital twin technology to all project phases, and across all three ESG dimensions, there remains challenges to implementation. Successful implementation of digital twin technology will require the right people with the right capabilities. Though suggested that the mining industry should let other industries stabilize the digital twin market due to their history and substantial investment in data systems, it is arguable through literature, case studies and leading mining companies’ investments in precursor technologies to digital twins, that solutions are available and scalable, and the time to wait is over.
1 Introduction
Environmental, social, and governance (ESG) are not new themes to the mining industry but have been gaining traction since 2015 when the United Nations released the Sustainable Development Goals (SDGs) (Leonida, 2022a). The spectrum of ESG considerations in the mining industry include, monitoring energy emissions, relations with local communities, disclosure, and reporting, among others (Baek et al., 2022), which are actively being captured into ESG plans that have become a focus for responsible investors, both in cost and benefit (Pedersen et al., 2021). In the mining industry, ESG factors not only contribute to investor evaluations, but also to obtaining social license to operate (Pennini, 2020), laying the foundation for the decision if a mining project is to proceed or not.
Prior to 2020, mining companies found themselves reacting to environmental and social issues in response to investor pressures and catastrophic events impacting their social license to operate (Leonida, 2022a). Investor pressures and increased regulations encouraged mining companies to start to change to a more proactive approach. Though ESG reporting has improved in the mining industry, it is not consistent, nor can it be replicated (Baek et al., 2022), resulting in difficulty establishing interim targets and trust in the reporting. The reporting that does exist is generally qualitative, and therefore, does not provide the quantitative metrics required to properly evaluate a mining project (Bascompta et al., 2022). Without the ability to assess a future project consistently and quantitatively, mining companies are not able to measure value propositions ESG actions would provide. Similarly, without metrics, stakeholders cannot assess one investment against another, nor can progress be measured over time. Though mining companies continue to increase the maturity of their ESG plans and have started to allocate capital to ESG actions that align with creating investor value, without the ability to quantify their reporting they risk being seen as “greenwashing” by investors and society (Leonida, 2022a). In the current environment investors are prioritizing investment in the mining industry to ESG dimensions over traditional technologies, therefore mining companies need to be utilizing technologies to optimize ESG dimensions during investment planning (Zhironkina and Zhironkin, 2023).
This paper reviews the opportunity to utilize the application of technology, specifically digital twin technology, to improve not only the communication of ESG dimension benefits to investors and society, but also the opportunity to improve progress in ESG dimensions. A digital twin is a digital representation of a physical object, process, or system, and utilizes historical data and a digital model to optimize processes and operations (Elbazi et al., 2022). Though digital twin technology aligns with the future trends needed for mining companies’ success, the use of it has not been significantly developed in the mining industry (Elbazi et al., 2022), but other industries have identified opportunities to apply digital twin technology to measure and advance towards ESG targets (Barn, 2022; Corrado et al., 2022; Shen et al., 2022; Solman et al., 2022). Barn (2022) illustrates the upmost end of digital twin technology application to ESG considerations through the EU Destination Earth, a digital twin of the Earth, that would model scenarios to meet policies and support sustainable development. The collection of data and connections between the real world and virtual, provide digital twins the ability to run future scenarios, providing the opportunity to predict and govern best sustainable practices, with potential to support the repeatable reporting and progress of ESG targets across all phases of a mining project.
2 Literature review
The bottom line for the mining industry remains unchanged - getting rocks over the line, but how mining companies accomplish this has come under scrutiny over the past few decades (Deloitte, 2022). From the initial stage of the supply chain, obtaining the resources required to supply sustainable alternative technologies, to doing so in a sustainable manner, the mining industry faces a significant requirement for change. The mining industry is at the center of opportunity for effecting change in the green energy transition, as identified in 2021 at the United Nations Climate Change Conference (COP26) (Deloitte, 2022). The World Bank Group (2020) has estimated, that a global temperature reduction of 2% could require an increase in certain minerals by 500% by 2050. The success of the mining industry in meeting their ESG goals sets the foundation for other industries to meet theirs; to produce sustainable products, the materials first need to be obtained in a sustainable manner.
With the increase of ESG awareness and the ability to fact check through the internet, stakeholders are demanding verifiable data and evidence to support ESG reporting (Saxena et al., 2023). Therefore, the challenge for mining companies is not only in reaching these targets, but also in planning, implementing, and measuring against interim targets that are needed to be successful. The solution on how to do this needs to be flexible and scalable, as sustainability targets are rapidly evolving, making them difficult to achieve, even more so when solutions and progress to those targets require capital (Popescu et al., 2022). Digital twin technology has the potential to provide reliable and consistent data in various industries and move the economy to a net-zero carbon future (Popescu et al., 2022). Though there is a need, there remains a gap in current literature regarding the application of digital twins in the mining industry to improve and report on ESG targets.
2.1 ESG in mining
Mining companies face “political, economic and social factors” (Farrelly and Davies, 2021, p. 22) that challenge greenfield projects from getting off the ground. Therefore, it is not surprising that the top ten trends identified by Deloitte (2022), for future economic success in the mining industry, are significantly influenced by ESG themes:
Trend1:Aligning capital allocation to ESG.
Trend2:Reshaping traditional value chains.
Trend3:Operating in new super-cycle.
Trend4:Embedding ESG into organizations.
Trend5:Evolving mining’s world of work.
Trend6:Establishing a new paradigm for Indigenous relations.
Trend7:Continuing the journey toward innovation-led organizations.
Trend8:Unlocking value through integrated operations.
Trend9:Closing the IT-OT vulnerability gap.
Trend10:Preparing operations for climate change.
As reported by Ernst and Young, ESG, decarbonization, and license to operate are the top three opportunities and risks for mining companies in 2022 (McQueen, 2021). These opportunities and risks align with the trends identified by Deloitte (2022), specifically with Trend 1 and Trend 4. Though ESG factors overlap between the environmental, social and governance dimensions, they do have defined elements.
2.1.1 Environmental dimensions
Environmental dimensions assess the affects a company has on the natural environment through their direct actions and behaviors (Senadheera et al., 2021). Leaders in the mining industry have been feeling the pressures from investors and other stakeholders on environmental changes to align with the Paris Agreement, and therefore, have been setting net-zero gas emissions targets to support the global reduction goals in relation to climate change (Kuykendall, 2021). Table 1 identifies the climate goals of the top ten mining and metals companies by market capitalization as identified in November 2021. Apart from Fortescue Metals Group Ltd. and Franco-Nevada Corp., these represented companies are party to a pledge to substantially reduce Scope 1, direct controllable emissions, and Scope 2, indirect emissions from purchased energy, by 2050. With further intent of tackling Scope 3, emissions derived from the upstream and downstream supply chain, in the approaching future. These goals are aggressive, and some companies have made them more so by placing interim targets or shorter timelines for full emission reduction. As Leonida (2022a) warns, mining companies cannot wait till 2050 to show they hit their targets, they will need to show progress between now and then, or risk being seen as “greenwashing”, at the potential loss of investors.
The environmental focus of mining companies goes beyond their net-zero pledges and climate change targets, it incorporates regulatory and internal metrics around pollution and waste management, resource and land use, biodiversity, and overall ecological footprint (Saxena et al., 2023). Water, for example, is an essential resource for mining operations, and the scarcity of fresh water makes the impact from mining that much more prevalent. As an industry wide challenge, the International Council on Mining and Metals has provided guidance to mining companies on water usage, including opportunities such as recycling water or the use of seawater (Cesar, 2021). Waste management is another factor that is industry wide, whether underground or open pit, extraction of ore requires effective waste management, both in lowering the ratio of waste and the negative impact (Bascompta et al., 2022).
2.1.2 Social dimensions
Social dimensions revolve around the impact a mining project will have on society, or stakeholder group. The addition of a mining project can have both positive and negative implications on local communities and can span over many years. These effects can be minimal or significant, can affect the way of life, health, culture, politics, or human rights, and can be real or perceived (Sairinen et al., 2021).
Most mining companies have corporate responsibility, ethical, and sustainability statements on their websites. When there are breaches in these practices or lack of commitment to promised ESG actions, they face more than reputational risk. In 2020, the mining company Rio Tinto blasted an aboriginal archaeological site at Juukan Gorge, Australia, that dated back 46,000 years. The site was above an iron ore body that had an estimated value of $104 million, and as such, Rio Tinto proceeded as planned, with knowledge of the archaeological site but still operating within the limits of Australian law (BBC News, 2021; Butron, 2022). In 2021, shareholders voted against the remuneration package proposed by Rio Tinto for executives that were stepping down from their positions due to stakeholder pressure for action. The shareholder vote was only advisory, and the board looked to proceed with the payouts, risking their potential removal from the board by shareholders (BBC News, 2021). The end results for Rio Tinto were the loss of three senior leaders, two of their board members, remuneration costs, funding a legacy foundation and there are ongoing discussions regarding the restoration of the site (Butron, 2022). This event shows the power society and shareholders can have regarding enforcement of ESG compliance to mining companies. Rio Tinto was compliant as per the law, but expectation is greater than compliance. The results Rio Tinto faced, align with Trend 6, identified by Deloitte (2022), mining companies need to go beyond creating partnerships with Indigenous communities that go above traditional agreements, to developing relationships and mutual economic models that result in long term benefit for all parties. To compete for investment, mining companies will need to go beyond regulations, they need to mitigate negative impacts, and optimize stakeholder benefit (Pennini, 2020).
2.1.3 Governance dimensions
Though environmental and social dimensions have been transforming the way the mining sector conducts business, the governance dimension has not been as relevant (Woźniak et al., 2022). Governance dimensions are defined by the organizational and management processes that provide control over company actions (Saxena et al., 2023). These dimensions can vary between regions and countries, each presenting their own risks (Leonida, 2022b). Domínguez-Gómez and González-Gómez (2021), identify four variations of understanding when it comes to governance in the mining sector. These variants are not siloed, but rather are connected and overlap within shared concepts (Domínguez-Gómez and González-Gómez, 2021).
The first understanding of governance in the mining industry, corporate governance, is internal. It defines governance by means of corporate management and organization, and good governance is evaluated against strong financial performance, transparency, and participation. The second variation, contextual governance, is fundamentally based on the external context, considering both social and environmental risk, and measures good governance like the first variation (Domínguez-Gómez and González-Gómez, 2021). This variation is measured by communities and other non-government stakeholders. Though these stakeholders do not have the same level of influence, the sheer size of the network creates influence as can be seen in the Rio Tinto case. Domínguez-Gómez and González-Gómez (2021) identify that these first two variations contribute to 61.5% of references in their data and conclude that these concepts define the core understanding of governance in mining industry literature.
The third variance of governance in the mining industry, metagovernance, bases governance as a value, and evaluates good performance against good values, such as ethics, sustainability, transparency, and respect. Unlike the first two variances, the government is a key stakeholder in the third variation and acts as an arbitrator of good governance (Domínguez-Gómez and González-Gómez, 2021). The fourth variation looks to governance as a key contributor for efficiency and effective management, acting as a “strategic tool” (Domínguez-Gómez and González-Gómez, 2021, p. 9). Like the discussion of “greenwashing”, Domínguez-Gómez and González-Gómez (2021) state the need for further research on the regulation of governance in the mining industry to go beyond “ethical washing” the industry’s image.
2.1.4 ESG targets in mining
It has become stakeholder expectation of the mining industry, that mining companies are managing the risk of ESG impacts (Woźniak et al., 2022). To maintain the trust with stakeholders and avoid “greenwashing” and “ethical washing”, mining companies need to report both financial and non-financial data that are reliable and available to the public (Pimonenko et al., 2020). Consistent and publicly available data, that is supported by transparent audits, certificates, and reports, will help reduce the image of “greenwashing” (Pimonenko et al., 2020). Though social and environmental reporting has become more sophisticated, there remains inconsistency amongst the top mining companies. The increase in yearly reporting does provide the opportunity to investigate trends in mining companies’ disclosures and as mergers and acquisitions occur the overall reporting does continue to improve (Jenkins and Yakovleva, 2006). Beyond the inconsistency between companies’ reporting, the accuracy, consistency, and adequacy of the data used in the reporting affects the trustworthiness of the reports (Saxena et al., 2023), further expanding the gap for stakeholders and investors to assess mining companies or projects.
Through their research, Bascompta et al. (2022), explore the various methods and indicators to assess environmental and social dimensions through a corporate social responsibility (CSR) lens. Their research identifies the importance of rankings in improving behaviors and governance, reducing poor practices, and providing transparency to stakeholders, overall influencing mining companies’ access to financing. Bascompta et al. (2022) found that most of the tools available have a foundation on qualitative data instead on quantitative data, lack technical input, or are too general and cannot be applied to a specific mining project. Therefore, Bascompta et al. (2022), proposed a simple and scalable index to evaluate the CSR state of mine sites. The proposed CSR index focuses on the social and environmental dimensions of ESG, though the authors acknowledge that the governance dimension could be added in future work. The CSR index proposed by Bascompta et al. (2022) moves reporting on social and environmental dimensions to a qualitative approach, but there remains the challenge of obtaining the data consistently in a way that can be audited, repeated, and verified.
2.2 Digital twins
Over the last 2 decades, leading mining companies have been exploring and implementing technologies to improve their digitalization through various stages of the mining lifecycle, from exploration to delivery (Farrelly and Davies, 2021). Though the detailed definition of a digital twin varies, the following definitive points tend to be consistent, and are what this paper refers to as a digital twin (Bhatti et al., 2021; Farrelly and Davies, 2021; Baek et al., 2022; Corrado et al., 2022; Elbazi et al., 2022; Newrzella et al., 2022; Popescu et al., 2022; Zekri et al., 2022).
• A digital twin is a virtual representation of a physical object or process,
• Once the physical is constructed, data is pushed from the physical to the virtual representation over the course of its lifetime,
• The virtual model incorporates historical data from the physical, and
• The data collected through digital twins are used to optimize the physical operations.
The concept of the digital twin has been developed through various industries, including manufacturing, aerospace, defense, shopping centers, process industries, networked infrastructure, and was originally proposed to the mining industry in 2009 as the Common Mine Model (Farrelly and Davies, 2021). This paper also refers to digital twin technologies throughout, to include precursor virtual models that exist before the physical twin is constructed.
Elbazi et al. (2022) proposed a conceptual architecture for a digital twin for a mine. The first layer represents the physical object or process which pushes data to the virtual model. The second layer proposed by Elbazi et al. (2022) is the data preprocessing layer. This layer is required to format and fill in the gaps that are found in the preliminary data gathered from the physical, and to reduce the data to a simplified form. The third layer, edge computing, is a local application layer where real-time monitoring occurs. The final proposed layer is the cloud layer, which hosts the virtual mine database. This is where optimization and predictive applications occur, combining the various models that exist in the edge computing layer and provides a backup of the data. The optimized models in the cloud layer get pushed back to the edge computing layer models regularly so the real-time models maintain the optimized data.
Hazrathosseini and Moradi Afrapoli (2023) used Elbazi et al.’s (2022) four-layer representation of a virtual mine along with other existing frameworks and lessons learned to derive a six-layer digital twin architecture: physical space, Internet of Things (IoT) gateway, cloud repository, virtual space, cognition, and briefing. The briefing layer takes the framework beyond that proposed by Elbazi et al.’s (2022), providing a layer that accounts for further manipulation of the data with meaningful outputs that can feed into informed decision making, strategic planning, and external reporting.
2.3 Digital twin development and implementation
Mining companies have faced several challenges that have slowed adoption of technology, including accountability and commitment, poor change management, and lack of interoperability between platforms and data (Farrelly and Davies, 2021). Newrzella et al. (2022) recognize that there are plenty of positive case studies of digital twins across various industries, but identify the challenge in establishing a starting point, the value proposition to stakeholders and estimating the effort to implement a digital twin. They utilized their research on existing models to develop a two-stage methodology that aids in application of digital twin technologies. The first step in their methodology is to evaluate if a digital twin application is the best solution, compared to other technology. The second step is to evaluate and identify data sources that provide the most value, but the least amount of effort to implement (Newrzella et al., 2022).
It is important to understanding the desired outcome of the innovation to determine prioritized development (Ulwick, 2002), this is fundamental to Newrzella et al.’s methodology in contributing not only to the value proposition of a digital twin but ensuring alignment with stakeholders’ satisfaction on the outcome. Though Newrzella et al.’s methodology was validated on a product development case, their research was guided by application in various areas, including process and engineering. Though Farrelly and Davies (2021) suggest that the majority in the mining industry should let other industries stabilize the digital twin market due to their history and substantial investment in data systems, it is arguable through literature, case studies and leading mining companies’ investments in predecessors to digital twins, that solutions are scalable and the time to wait is over.
2.4 Digital twin opportunities in relation to mining ESG targets
The opportunity for the digital twin to be more than a model or simulation, is founded in the ability to communicate in real time with the real physical environment (Popescu et al., 2022). This allows for corrective and preventative actions to improve the system immediately (Popescu et al., 2022). This allows for opportunity to plan, implement, and improve sustainability strategy and practices using a digital twin. Farrelly and Davies (2021) describe this flow of information as a “loop cycle of decision making: observe, orient, decide, act”, giving the ability to run simulations and then make changes in the real environment, ultimately improving decision making (Farrelly and Davies, 2021, p. 25).
Zhironkina and Zhironkin (2023) propose the mining industry is headed in the direction of Mining 5.0 as the next evolution of technology application in mining. The evolution of the mining technology paradigms, as defined by Zhironkina and Zhironkin (2023), are:
Mining 1.0: Mechanisation of the auxiliary mine process
Mining 2.0: Mechanisation of key processes of mining and mineralization
Mining 3.0: High powered equipment, digital computing and control systems and analog telemetry
Mining 4.0: Systematic optimization of the mining sector through digital and cyber-physical technologies
Mining 5.0: Synergy of humans and autonomous machines
The shift proposed by Zhironkina and Zhironkin (2023) into Mining 5.0, will focus on circular production and prioritization of life and health of workers, and will require the adaption of digital twins and other technologies.
2.4.1 Conceptual, study, and engineering phases
During the conceptual, design, and engineering project lifecycle phases, models and simulations are precursors to a digital twin as the physical twin does not exist yet. Depending on the outcome of the project, conceptual models may never develop into a digital twin, but these project lifecycle phases are still relevant to this research for planning and scenario purposes. Parallels between the research conducted by Corrado et al. (2022), in applying a digital twin technology to cities for sustainability planning and governance, can be made in the mining industry. Like cities, mining sites are built up of complex systems and processes. Commonalities include water management, vehicle maintenance and routing, infrastructure design and engineering, climate change effects and more. Corrado et al.’s (2022) research supported their development of a framework for sustainability planning for urban development and governance by bringing multiple digital twins together to study multiple green metrics. This allows planners to make decisions that lead to positive sustainable outcomes (Corrado et al., 2022). Design models can also provide the opportunity to improve safety early in the project. During the design phase, rule-based validation can be applied to models to improve spatial constraints and virtual walkthroughs can improve hazard identification and risk mitigation (Hoeft and Trask, 2022). The exploration of the virtual model can improve communication and trust between stakeholders and can support the efficiency of constructability and risk assessments (Hoeft and Trask, 2022).
2.4.2 Construction phase
The construction phase of a project is where the virtual model begins to transition into a digital twin as the physical twin begins to take shape. During this transition, the model can still be used to simulate future activities such as construction sequencing, laydown areas, and task planning. During construction, the model can also use real data from the field through sensors marking material locations, vehicle paths, and personnel through wearable technology. This data can be used to reduce collisions, support evacuation plans, alert of environmental factors, and identify high hazard zones, improving the over safety of the construction site (Hoeft and Trask, 2022).
Plachinda et al. (2023), share the benefits that digital twins can minimize greenhouse gas emissions and energy consumption during construction by optimizing schedule and performance. They argue that there is a lack of visualization of the related data though and propose the net-zero engineering support tool (NEST), that was developed by the Idaho National Laboratory (INL) campus, as a tool to help stakeholders visualize carbon emissions, energy consumption, and cost in construction and into operations.
2.4.3 Operational phase
The operational phase implements the full understanding of a digital twin, as real data gets pushed from the physical twin. This application is illustrated through Elbazi et al.’s (2022) case study. Their case study resulted in a preliminary digital twin that embodies the mechanisms and automation of a stacker machine in an open pit mining environment, creating the ability to test the design and control of the real system in a virtual environment.
Baek et al. (2022) presented an application of digital twin technology using mobile light detection and ranging (LiDAR) mapping paired with object location through point cloud registration, which resulted in a 3D global localization method. Though not able to operate in real time, the research by Baek et al. (2022) contributes to digital twin realization in underground mining by adding improved spatial accuracy where satellite coordinate systems are not available. Through the application of the 3D global localization method to a digital twin, Baek et al. (2022) believe there is opportunity to improve prediction in operation, production, and safety through geotechnical analysis and the accuracy of autonomous driving vehicles.
Beyond the safety measures identified in the construction phase, the full digital twin can bring information into a visualized virtual replica of the mine during operations. Combining this visualization with maintenance standards and standard operating procedures can centralize the information for access to everyone and trends can be identified through accumulation of historical data (Hoeft and Trask, 2022). These trends can then be corrected or mitigated, improving the safety of the operation.
2.4.4 Closure phase
The closure phase of a mining project is another transitional phase of a digital twin, either during demolition or asset transformation. As identified by Deloitte (2022), mining companies need to partner with Indigenous communities to create mutual economic models that create long term benefit. Deloitte (2022) indicates that sustainable projects consider the entire life cycle of the mine and would continue to add value to the local communities after mine closure. Digital twin technology can run simulations that could provide mutual benefit for remote communities, such as transportation infrastructure or utility facilities prior to and during operations. Beyond that, a digital twin can assist in presenting various asset transformations to support a community rather than a path to closure.
3 Materials and methods
This research is focused on the challenge of measuring progress toward ESG targets in the mining industry consistently using digital twin technology. This paper does not introduce new technology or a new theory but seeks to evaluate the application of existing technologies and theories presented in other industries that utilize digital twin technology to measure and improve ESG dimensions to the mining industry. The structure of this research was initiated through a detailed literature review in response of the primary research questions.
• Why are ESG considerations important to the mining industry?
• How does the mining industry define ESG dimensions, targets, and their progress in meeting them?
• What is digital twinning technology?
• How are digital twins supporting ESG targets in other industries?
To address the focus of this paper, “How can the mining industry apply digital twins to measure and advance their ESG goals?”, the data obtained in the literature review was coded against ESG dimensions and applicability to the phases of a mining project. The research for this paper was gathered through secondary data sources: academic journals, mining company websites and other public facing sources. Though the focus of this research is the mining industry, data was pulled from journals in various industries that have commonalities with mining or presented a case study that could be applicable to mining. Table 2 identifies the key journals used for gathering data and Table 3 identifies the key search words and phrases used. As advancements in mine digitalization technology and ESG trends are rapidly evolving, the focus of the research is from the last 5 years, earlier documentation is the exception. The data collection for this paper was limited by the gap in research in digital twin application in the mining industry. Though this paper looks to address this gap through the application of digital twins in other industries, some activities in mining do not align with other industries, such as tailings management. Another limitation to the research is the relative newness of the development of digital twin technology and the tested application in measuring ESG dimensions and the need of mining companies measuring and reporting on their ESG plans. There also remains a lack of guidance for ESG reporting in the industry.
A total of thirty studies were evaluated as part of this research and are listed in Table 4. Each study was evaluated against the application of digital twin technology, the industry it applied to, whether there was a supporting case study or if it was theoretical application, and the main application to ESG dimensions. The following discussion provides a detailed analysis of the results in Table 4 against the project phases of mine project development to explore how the mining industry can apply digital twins in new ways to measure and advance their ESG goals.
4 Discussion
The benefit of applying digital twin technology to a mine site can be evaluated against the various project phases and ESG. This evaluation results in Table 5, illustrating the applicability of digital twin technology to ESG dimensions at various project phases of a mine site. The sources presented in Table 5 support the application of digital twin technology to the referenced ESG dimension and are further defined in the following sub-sections. Table 5 methodology can be applied to a greenfield build, or a modification project of a brownfield operation. Many ESG dimensions span through various project phases, providing mining companies various vectors to manage ESG targets. The shaded cells in Table 5 represent theoretical application of a digital twin during that project phase for that ESG dimension on a mining project. Cells with an ‘x’ represent areas that a proof of concept has been completed. The following sections review each of the ESG dimensions and associated case studies and theories, presented in Table 5, in detail.
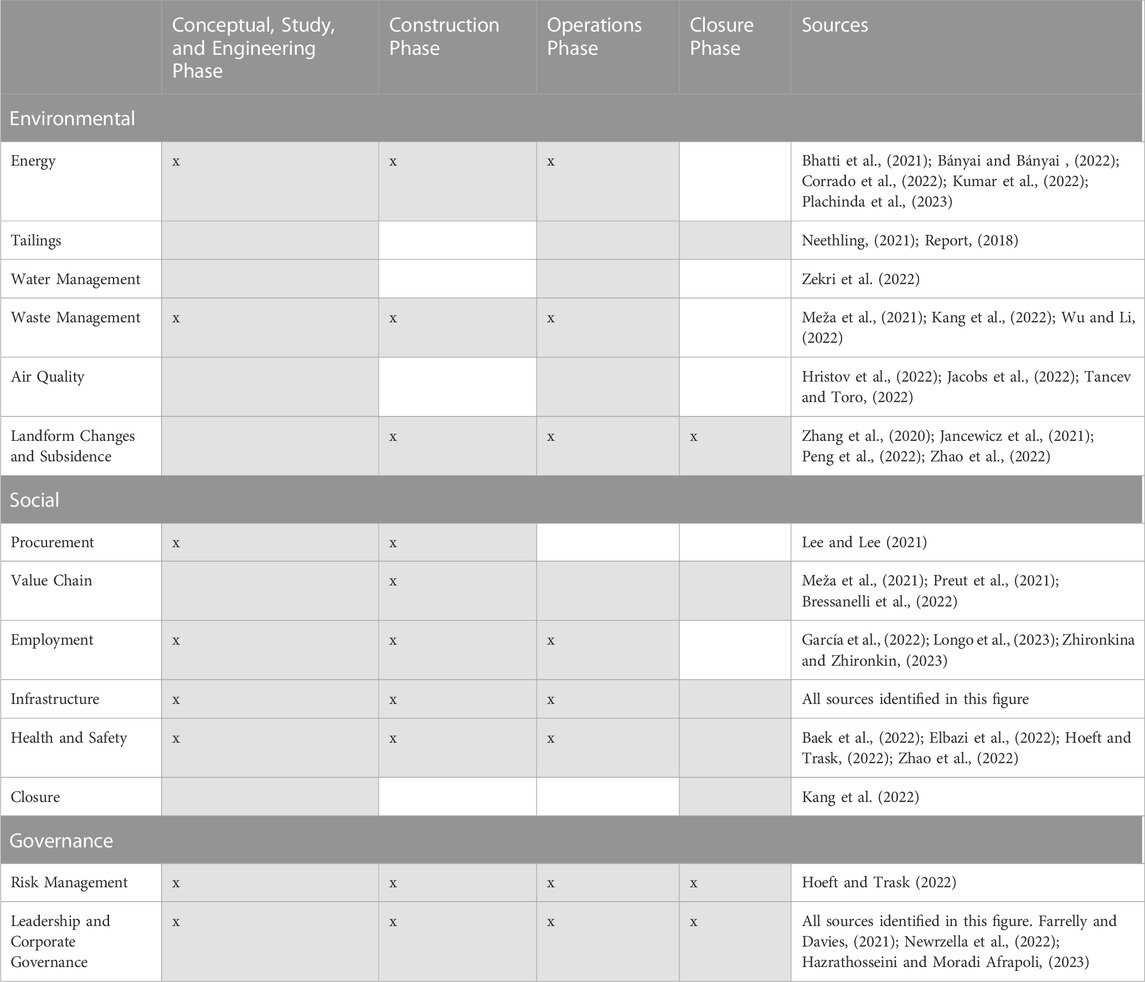
TABLE 5. Potential application of digital twin technology to ESG dimensions and mining project phases.
4.1 Environmental and social
Environmental and social dimensions evaluate the impacts that mining sites have on the natural environment and on society, respectively, through the mining companies’ direct actions and behaviors (Senadheera et al., 2021).
4.1.1 Energy
Energy consumption and greenhouse gas emissions are the forefront of promised targets of mining companies (Kuykendall, 2021). Corrado et al.’s (2022) research provides a framework and basis for the application of digital twin technology supporting complex systems in the planning stages. Though their research is based on cities, the surface infrastructure of a mine site is comparable to that of a city. They found that digital twins assist with optimization of operational processes during design, resulting in conservation, efficient monitoring, and predictive processes (Corrado et al., 2022). Though Plachinda et al. (2023) have presented the NEST tool for the visualization of energy and emission related data, they do identify a benefit of digital twin technology application during construction to reduce energy consumption and greenhouse gas emission through schedule and performance optimization.
Bányai and Bányai’s (2022) research applied digital twin technology to improve the operational and maintenance processes of manufacturing system. Their research found that the optimization of the real-time maintenance policy through the data provided by the digital twin, decreased the energy consumption, energy cost and greenhouse gas emissions by approximately 21%. Greenhouse gas emissions can also be reduced using an electric fleet (Bascompta et al., 2022). Bhatti et al. (2021), explore the application of digital twin technology, and highlight the benefit of using the technology to monitor vehicle health, battery management, and autonomous control. In another study, Kumar et al. (2022), identify the opportunity for machine learning through digital twin technology, to create data driven models that can predict electrical vehicles’ battery management.
4.1.2 Tailings
In 2019, a tailings dam failure occurred in Brazil at a Vale iron ore mine Córrego do Feijão. After the failure, the slurry covered 8 km of farmland and communities, killing over 250 people (Morse, 2020). Despite the importance of tailings management in the mining industry, this research found no case studies, peer reviewed articles or academic papers on applying digital twin technology to tailings management. Though there is little academic data on documented findings, there does appear to be future opportunity. In 2018, the software developers, Dassault Systemes, announced a partnership with ATC Williams, tailings consultants. The goal of the partnership was to utilize Dassault Systemes’ software platform to develop a study level digital twin solution to simulate complex water recovery processes (Report, 2018). Peter Neethling (2021), a Director from Seequent, published an article in the Canadian Mining Journal, that shared Seequant’s vision of a Tailing Storage Solution. This solution is a combination of multiple software offerings that contribute to a cloud-based digital twin of a tailings facility. This solution would provide real-time data to various stakeholders to make informed decisions through operations and maintenance (Neethling, 2021).
The articles advertising software solutions for tailings management suggest that digital twin technologies will be applicable to manage tailings facilities through predictive analysis during planning, operations and into maintenance. Though there will likely always be a need for tailings management facility, Bascompta et al. (2022) argue that the sustainable goal should be to reprocess the tailings into a product or use tailings as a construction material, resulting in zero tailings.
4.1.3 Water management
Water access and usage continues to be an industry wide challenge. The scarcity of fresh water makes the impact from mining that much more prevalent. Digital twin technology in water management is currently limited, though Zekri et al. (2022) propose a framework for the application. Their prototype provided status of smart water meters, delivering the user feedback on water consumption (Zekri et al., 2022). Though the prototype presented by Zekri et al. (2022) did not engage the full digital twin infrastructure, their research did provide examples of digital twin technology being used to acquire real-time data, provide simulations, and device monitoring, that could be applicable during operations.
4.1.4 Waste management
When considering waste management as an ESG dimension, the focus is on the elimination or reduction of the waste. Meža et al. (2021) explored the use of secondary raw material, such as mine waste, to road development. They first created a two-dimensional design and then turned it into a three-dimensional model, which acted as the precursor of the digital twin. During initial construction, sensors were placed throughout the section of road and their data was then integrated with the digital model. The key values from the data gathered from the physical twin were uploaded to the digital twin and provided status of the secondary raw materials, and therefore the structural stability of the road.
When waste cannot be eliminated or reused, it must be managed. Wu and Li (2022) created a digital model of a hazardous waste landfill system. Their digital twin was fed by real-time data from the automated guided vehicle sensors and other environmental sensors that enable the system to identify the best driving path. They found that the application of the digital twin technology enabled them to monitor the landfill in real-time and to use the digital twin for off-line simulations. The opportunity for this technology in the mining industry is comparable to material handling and managing large amounts of waste.
Kang et al. (2022) examined the applicability of digital twin technology to the demolition of residential buildings and waste management. They propose a three-layer framework that begins with collecting data from surveying the building and multiple sensors in the first layer. In the second layer data is stored, cleaned, and processed. The second layer supports decision making and operations of the demolition. The final layer uses the data from the first two layers to manage collaboration with waste trading, supply change management and treatment management (Kang et al., 2022). Kang et al.’s (2022) case study and cost-benefit analysis demonstrated that digital twin technology shows a cost benefit. Though this case study was completed on a residential building, it can serve as a proof of concept for the demolition of infrastructure of a mine site.
4.1.5 Air quality
Tancev and Toro (2022) proposed a digital twin model to monitor air pollution, with a focus on reducing maintenance costs and metrological traceability. The technology, tools and sensors required to measure air quality to support a digital twin already exist, but they raised concerns, such as data quality, computational limitations, and ability to provide return on investment (Tancev and Toro, 2022). Hristov et al. (2022) present a living lab that is part of an initiative to develop a digital twin of the city Sofia, Bulgaria. One of their analyses of the data collected in the living lab is on air pollution. The immediate benefit realized for the monitoring of air quality is identifying and reviewing of trends. The system will ultimately be able to gather real time data and produce predictions, this will allow users to analyze the success of air management practices (Hristov et al., 2022).
The considerations for air quality in the mining industry do not only need to take in consideration emissions created by the mine, but they also need to consider the air quality within the mine. Jacobs et al. (2022) study how digital twin technology can aid in ventilation planning for deep mines. They identify that the ventilation challenges in deep mines are: the high temperatures, air pollutants from dust and emissions from the mining equipment. To mitigate these risks and hazards, ventilation systems need to be reviewed and revised on a regular basis to ensure safe working conditions. Jacobs et al.’s (2022) case study identify the opportunity to apply digital twin technology to a mine’s ventilation system in an existing mine. Utilizing information from the current operations they were able to derive a digital twin that was calibrated to the physical twin. Using the digital twin, they were able to identify areas in the planned mine design that were at risk for high temperatures and low airflow. Having this information available at the planning stage allowed for mitigation, and in some cases, elimination of risk (Jacobs et al., 2022).
4.1.6 Landform changes and subsidence
Landform changes created by mining operations are any changes that change the natural topography of the land. The changes can be visual, affect the ecosystem (Bascompta et al., 2022), and can be both planned (ex. excavation, waste piles) or unplanned (ex. subsidence) (Jancewicz et al., 2021). Jancewicz et al. (2021) present the development of a map that identifies the landform changes of the city of Walbrzych, which has been substantially impacted by coal mining activities. Using LiDAR technology, they were able to model and identify various changes to the topography of the area (Jancewicz et al., 2021). The efforts carried out by Peng et al. (2022) to create a digital twin to analysis the geological and geomorphic conditions of Beijing, illustrate the opportunity to monitor landform changes from mining activities in a similar manner. Peng et al. (2022) utilized drone technology with tilt arial photogrammetry capabilities to develop a digital twin of the forested mountain terrain of Beijing. This technology captured geological hazard points that are in difficult to access terrains, which is common in mining sites. The model provided not only visualization of the terrain, but also high precision measurement and ability to analysis and trace hidden hazards, providing a foundation for early warning of geological risks (Peng et al., 2022).
Beyond the planned landform changes from mining activities, there is the risk of ground subsidence. Surface deformations can be caused by underground mining, and can threaten what is on the surface, whether it is an ecosystem or city development. To measure ground subsidence caused by underground mining activities, Zhang et al. (2020) utilize Interferometric Synthetic Aperture Radar (InSAR) technology and were able to identify rapid surface deformation. Their conclusions included that InSAR technology could be combined with a virtual model to create a three-dimensional monitoring network, used for rapid and slow rate subsidence, and could provide data for land restoration.
4.1.7 Procurement
Supply chain management has been an area of focus for digital twin technology, specifically in relations to coordinating various components including equipment, transportation, storage, and workers (Lee and Lee, 2021). Lee and Lee (2021) share their research on the use of three-dimensional modelling to define modules for streamlined construction. Modular construction refers to the fabrication of a modules of a building being assembled offsite while foundations are being constructed on site. The virtual model of the building allows the design team to design the modules to fit within transportation size and weight constraints and can also simulate the construction sequence and timing (Lee and Lee, 2021). Modular design on its own can benefit sustainable design by streamlining materials, assembly onsite, and transportation costs. Lee and Lee’s (2021) research provides a framework and a case study to take the modular model to a full digital twin with real time feedback from the supply chain and construction site. They used the digital twin to create “what-if” scenarios with the transportation routing scenarios to identify and predict risks and suggest alternatives. In addition to the transportation routes, the digital twin can identify the estimate time of arrival in real time, providing construction crews the ability to streamline the construction schedule based on delays to minimize downtime. Their case study found the application of the digital twin reduced idle construction time by 157.5 h.
4.1.8 Value chain
Mining is often the first step in value chain creation, with the extraction of raw material, but mining sites can also see sustainable benefit through more cyclic use of materials. The circular economy paradigm provides a sustainable approach to the value chain, often with a basis in the 3 R’s: reduce, reuse, and recycle. The “R” framework has been explored and expanded on by many researchers, expanding the concept to include more granular levels including rethink, repair, repurpose, recover, and remanufacture (Bressanelli et al., 2022). Bressanelli et al. (2022) presented a framework for the use of digital technologies in application to the circular economy, including: Internet of Thing (IoT), big data and analytics, three-dimensional printing, blockchain, and augmented and virtual reality. Though not identified as a specific technology, Bressanelli et al. (2022) applied their framework to the article Digital Twins for the Circular Economy, by Preut et al. (2021). Preut et al. (2021) theorized through their research, that digital twin technology has the potential to add to the circularity of materials and products. Bressanelli et al. (2022) connected these findings to support design, manufacturing, distribution, usage, and end of life, overall adding to the circular value chain. The applicability of this research in the mining industry is tied to procurement of materials, equipment, parts and to the lifecycle of these items during and post operation.
Another opportunity to add to the value chain in mining can be applied by the research conducted by Meža et al. (2021) on the use of digital twin technology on road construction. Not only does their research apply to the infrastructure piece of road construction for mine sites, but their research also included the use of secondary raw materials in road construction, for example, the use of mining waste. Meža et al. (2021) were able to create a proof of concept through their digital twin prototype that used secondary raw materials in road construction.
4.1.9 Employment
When considering employment as a social dimension, it is based on the quality of the employment. This can vary between the diversity of the staff, the level of skill required, to salary disparity (Bascompta et al., 2022). Digital twins can contain data to report on these elements, but this evaluation is in the benefit of improving the quality of employment tasks. Longo et al. (2023), explored the use of digital twin technology as means of a training tool, using offshore oil rig activities as a proof of concept. Though not a replacement for current onboarding training, the “training on-the-go” strategy presented, compliments productivity and safety requirements. This case study identified learner flaws, assumptions, and the opportunity to improve the learning process. The “training on-the-go” strategy provides the ability to train workers on a particular skill remotely and get familiar with the content or a new upcoming task (Longo et al., 2023).
García et al. (2022) also explored the opportunity of applying digital twin technology to learning. Through their research they propose a three-layer Digital Twin Learning Ecosystem. The ecosystem utilizes human-machine collaboration and infaces through digital twin data streams, models, and knowledge models. This ecosystem resulted in “an augmented physical-digital way of bidirectional interaction, understanding and learning, between workers, systems and process in a framework integrated by virtual and real Intelligent Manufacturing Ecosystems” (García et al., 2022, p. 7).
Beyond the use of digital twin technology for training and learning to improve the employment experience there is opportunity to connect virtual environments with robotics in the physical world. Hoeft and Trask (2022) identified opportunity to use the model for linkage to robotic construction, and Zhironkina and Zhironkin (2023) present the use of digital twin along with machine learning and vision, automation and other technologies continue to move the mining industry into Industry 4.0, with the opportunity to completely exclude human workers from hazardous areas.
4.1.10 Infrastructure
Infrastructure that mining sites create can have a substantial impact to the local communities. The scale of this can range from roads and railways to schools and airports. Like other social dimensions, the creation of infrastructure can be positive and/or negative if not planned well (Bascompta et al., 2022). Through the analysis of other environmental and social dimensions, the research has shown applicability of digital twin technology to infrastructure in all project phases, whether it is buildings, roads, and/or systems.
4.1.11 Health and safety
Health and safety are prevalent topics in the mining industry. The Prevention Through Design (PtD) program by the National Institute for Occupational Health and Safety (NIOSH), is a program that is used throughout mining and other industries. The intent of the program is to aid in designing hazards out of products, operations, and processes. One of the tools that NIOSH uses is the Hierarchy of Controls, which has five levels: elimination, substitution, engineering controls, administrative controls, and personal protective equipment. The first level of elimination is the most effective, and each subsequent level is less effective. Virtual models of mine sites have been used to guide safety programs during the engineering and design phases through visualization, hazard identification, code checking and scheduling (Hoeft and Trask, 2022). Utilizing the concept of PtD, with the aid of three-dimensional models, rule-based design and visualization can support the elimination or substitution of hazards during the engineering phases. Hoeft and Trask (2022) reviewed literature on using three-dimensional modelling for hazard identification and mitigation throughout the life cycle of buildings. Throughout the project phases, their research showed hazard identification of strikes, injury, fire and “caught betweens”, with mitigating solutions being rule-based design checking and validation, task planning, temporary structures, safety training, monitoring, learning and documentation. The deconstruction phase remained a gap in their research, as they were not able to identify applicable literature. Their research found that the models were typically used to support the engineering phase, through aiding risk assessments and design reviews, or through simulations. They also identified opportunity to use the model for linkage to robotic construction, which could reduce human exposure and therefore risk (Hoeft and Trask, 2022).
Zhao et al. (2022) challenge traditional approaches to construction safety assessments and suggest that a digital twin can offer a solution to the dynamic nature of the construction process. Zhao et al. (2022) present a construction safety assessment framework based on digital twins. Their framework has three layers: the entity layer (the physical twin), the digital layer (the digital twin) and the functional layer (predictive functions). The functional layer is where the safety management process occurs, which then guides construction. During the construction phase there are challenges to represent the physical state, and therefore scanning technology can be used to feed data and accuracy back to the model (Zhao et al., 2022). Zhao et al. (2022) conducted a case study to test their framework, using the construction of a tunnel equipped with smart devices. Their conclusion was that utilizing a digital twin, evaluation and prediction of construction elements can provide more intelligent safety management than traditional processes, maintaining a consistent safe state.
During operations, digital twins continue to apply to safety planning through fire safety management and various maintenance activities (Hoeft and Trask, 2022). Beyond the operational planning aspects, the real-time feedback ability can provide awareness of equipment status and immediate actionability, adding to maintenance safety. Elbazi et al. (2022) caution that though digital twins can add the dimension of safety, more effort needs to occur on ensuring that the gathering of data for the digital twin is done safely.
4.1.12 Closure
Though there is little documented use of digital twin technology in deconstruction, simulations have been used to visualize sequencing and scheduling with various stakeholders (Hoeft and Trask, 2022). The residential deconstruction case study by Kang et al.’s (2022) does provide a proof of concept and direction on how digital twins can be used for closure and managing and reusing waste. Though there is no case study that was reviewed on using digital twin technology for the closure of a mine, it can be theorized that the application of the technology for planning and construction could be inversely applied.
4.2 Governance
Governance aligns and overlaps with environmental and social dimensions. Specifically, to mining, governance is crucial to the impact to both society and the environment and therefore, to the economics of the mining project. As a result, governance entails both internal and external management, while maintaining a focus on economic effectiveness (Domínguez-Gómez and González-Gómez, 2021). Though the applicability of digital twin technology may not be as apparently tactile to governance dimensions as it is to environmental and social dimensions, the overall development of the concept of digital twins was derived from systems engineering for the purpose to support in the design and governance of complex systems (Corrado et al., 2022). Therefore, the digital twin within itself, is a tool of governance. Providing visualization for communication with stakeholders and real-time data for informed decision making and transparent consistent reporting.
4.2.1 Risk management
Risk assessments and overall risk management often drives decisions in the mining industry. A risk matrix is typically used to evaluate various types of risks through their likelihood of happening and the severity of their impact if they do. The definition of severity and likelihood will change depending on the type of risk you are evaluating. Once a risk is identified and assessed, stakeholders mitigate the risk to bring it into a lower risk threshold, or preferably eliminating the risk, as per the Hierarchy of Controls.
The continuous and dynamic nature of risk management means it occurs through all the project phases. The risk identification and analysis processes are often driven by subject matter experts and can be time consuming and expensive (Wang, 2022). During the engineering and construction phases of a project, the three-dimensional model can use rule-based validation, layout and task planning, and serve as a visual communication tool for all stakeholders (Hoeft and Trask, 2022). During construction and into the operational phase, the digital twin is supported by real-time data, which in turn, can support real-time hazard identification (Hoeft and Trask, 2022). By building rule-based checking into the model, hazards are quicker to identify and then the focus of the subject matter experts is on the mitigation of the risk, improving the overall efficiency of the process. Beyond design risk, there is opportunity to apply digital twins to improve schedule risks in procurement, construction and demolition, and personal risk through virtual training.
4.2.2 Leadership and corporate governance
When measuring ESG dimensional impacts, there tends to be a lack of consistency and impartiality. Implementation of technology can add uniformity to the collected data (Saxena et al., 2023), minimizing the argument of “greenwashing” or “ethical washing”. Beyond the collection of data during operations, the virtual model can support communication with various stakeholders by visual means (Hoeft and Trask, 2022). This can happen throughout the life of the project, building overall trust through transparency, an essential item in getting social license to operate. Though there was not a specific case study that identified the use of a digital twin for corporate governance, the application of the technology provides a form of governance through every analyzed case study related to each identified ESG dimension.
4.3 Limitations and implementation risks
This research did not evaluate the feasibility of implementing digital twin technology or the profitability and return on investment, but rather the value proposition in making progress towards and measuring against ESG targets. Throughout the literature review and analysis there are identifications of cost savings, schedule improvements, and other efficiencies, but for the purpose of this research these applications were evaluated on the value they added to ESG dimensions.
Overall, the analysis shows opportunity to apply digital twin technology to mining projects to measure ESG targets and advance them. Though there is opportunity, there are still challenges and risks to implementation. From a governance perspective, there is still lack of standardize quantitative ESG metrics (Bascompta et al., 2022) and a lack of regulatory standards for modelling (Hoeft and Trask, 2022). Although many of the reviewed papers have proposed frameworks and indexes to address this gap, none have been fully adopted by the mining industry, and therefore pose a risk for implementation. In the meantime, if implemented consistently, a framework or index could provide the opportunity for consistency within the life of a mining project or across an owner portfolio, and therefore auditability and transparency for stakeholders.
The technology itself also proposes a challenge to implementing a complete digital twin, as there is not a singular product offering that can address the complexity of a full mine operation (Farrelly and Davies, 2021). Systems are currently siloed and have minimal interoperability between platforms (Pileggi et al., 2020; Farrelly and Davies, 2021). The complexity of proprietary expertise of service providers, along with the various initiatives to standardize interoperability in the mining industry, leaves the mining industry at a disadvantage for implementing digital twin technologies (Farrelly and Davies, 2021).
For successful implementation of digital twin technologies, you need the right people, with the right capability, or risk failure (Farrelly and Davies, 2021). Review of the top five global mining engineering academic institutions (Philip, 2020), showed that there is minimal, if any, required courses related to ESG or digital twin technology in their curriculums, refer to Table 6. For the mining industry, implementation of digital twin technology is still in its infancy and the pressure to not only achieve but also show measurable progress to ESG targets is also new. This means that the current workforce did not study these topics in school. Though many of the institutes listed in Table 6 do offer electives related to ESG or emergent technologies, for the most part they are not required courses in the curriculum, which is only growing the gap in these knowledge areas.
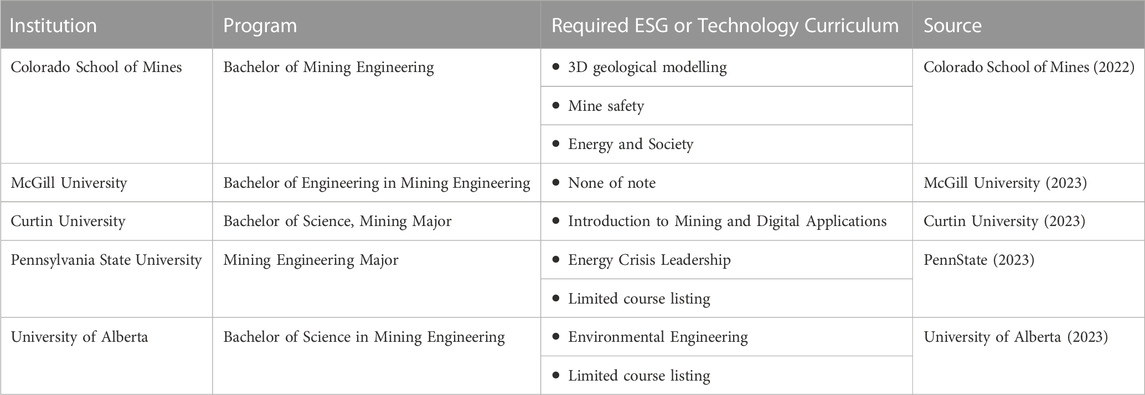
TABLE 6. Evaluation of the mining curriculum of the “top 5 best mining engineering schools in the world—2020” (source: Philip, 2020).
Although the research did identify applications of digital twin technology being utilized to measure and improve ESG dimensions in other industries that were applicable to the mining industry, there remains the challenge that there are certain ESG dimensions that are unique to the mining industry and therefore cannot be proven through applications in other industries. In some cases, such as landform changes due to mining activities, there were proof of concepts available in academic literature. In other cases, such as tailings management, there was no academic research to pull from.
As seen in Table 5, there is opportunity to use digital twin technology to impact multiple ESG dimensions across all project phases. The analysis does show that utilizing a three-dimensional model during the early stages of engineering, supports planning and creation of simulations to improve the most ESG dimensions compared to the use of a digital twin technology in other project phases.
5 Recommendations
The research and analysis show that digital twin technology can be applied across all phases of a mining project and provide value added in improving ESG dimensions and measuring them. Although there can be added benefit, it does not mean that implementation is feasible, possible, or desired. When considering the recommendations derived from this research, they need to be shaped by not only their practicality in relation to feasibility and ability, but also in alignment with stakeholder satisfaction, which is critical to measure success (Newrzella et al., 2022).
5.1 Mining companies and mining service industry
In this context, mining companies are the operators of the mine sites and owners of the project. Mining companies are accountable for the execution of the projects to their stakeholders, and therefore are the decision makers in the execution of projects and implementations of technologies. Though these recommendations are targeted to mining companies they are also applicable to the mining services industry, referring specifically to the services that would directly impact the use of digital twin technology in relation to measuring against and improving ESG dimensions in the mining industry: engineering services, project management, procurement, construction, and suppliers.
5.2 Strategy, influence and change
Leadership is key to successful implementation of both digital twin technologies and progressing ESG targets (Farrelly and Davies, 2021; Leonida, 2022a). Successful transformational change will not happen by hiring a single subject matter expert, there needs to be a sense of urgency created throughout the managerial team, a change coalition, and an overall strong vision (Kotter, 1995). Not only do mining companies need to be getting the right people with the right skills sets within their teams, but they also need to ensure that the one leading the project is a change agent (Kotter, 1995). Leadership will not be successful at executing a vision alone, change agents throughout the team are key to restructuring power balances and identifying resistance (Manning, 2003). Therefore, it is recommended that mining companies and the service industries evaluate their teams, intentionally hire change agents throughout their organization, specifically within leadership, and develop a change management-based strategy for both ESG and implementation of technology. As the influencers for the industry, mining companies need to be clear on the vision and demand compliance and alignment not only from their partners and service providers, but also from academia and education institutions to support them in the execution of their vision.
5.3 Knowledge gap
Though ESG measurements and digital twin technologies are not new, they do not have a long history either, even more so within the mining industry. Consequently, unless they have sought it out through continuous education, many leaders in the mining industry do not have the skill set or knowledge of these topics. Table 6 identifies that there is still a gap when it comes to both ESG and digital twin technologies in the required education for mining engineers in the leading global programs. Though there are available electives that are related to ESG and technologies, if they are not mandatory the knowledge gap will continue to expand. Therefore, it is recommended that mining companies evaluate the skills and knowledge that they will need in the future and use their influence to change the curriculums of their feeder schools so that new graduates are ready to be change agents the industry needs. There is also opportunity for mining companies to ensure their current talent take courses to understand what is required for technological and ESG change.
5.4 Culture and behaviour creation
Nitlarp and Kiattisin (2022) found that technology can benefit ESG dimensions and production, but implementation requires a positive culture of adoption. Culture is heavily tied to the first recommendation regarding leadership and change management. One of Kotter’s (1995) top errors for transformational change, is not integrating the change into the culture. A transformational cultural shift previously seen in the mining industry can be seen through the shift in the safety culture. Over the last 2 decades, there has been significant growth in the mining safety culture throughout Canada (Braden, 2016). Safety has been embedded into each employee’s daily tasks and they have accountability to it, but zero tolerance is driven from the top (Braden, 2016).
The growth of the safety culture globally is entrenched in several safety related activities, practices, and programs. Some of these tools were previously explored in relations to health and safety and risk assessment, The Hierarchy of Controls, and Risk Assessment Matrix. These tools are common in the industry, are already embedded into the safety culture, and are often used with digital twin technologies. Therefore, it is recommended that the mining industry utilize these existing tools to effect change with ESG dimensions. With a slight modification to The Hierarchy of Controls, changing the last control, Personal Protective Equipment (PPE) to personal protection, a tool that is already well known to the industry, can be made applicable to controlling ESG dimensions.
The first layer, which is the most effective layer, is Elimination. In the traditional tool, elimination refers only to the elimination of hazards. Reframing this to consider the reduction or elimination of an impact to an ESG dimension, allows the industry to broaden the use of the tool. Elimination can still be a hazard, but through this lens it could also be unethical behaviour, biases, inequality, harassment, etc. The next layer, Substitution, traditionally refers to replacing a hazard, in an ESG context it could be repurposing material or using a more sustainable product. Engineering Controls are typically used to isolate an individual from a hazard, but engineering solutions offer significant opportunity to control the impact on ESG dimensions. Pulling from the research by Bressanelli et al. (2022), in relations to the circular economy and ‘R’ strategies through: reduce, repurpose, rethink, repairing, remanufacturing, and refurbishing, the Engineering Controls can be broadened towards environmental and social dimensions. Behavioral changes are driven by Administrative Controls, and similar to how they can reduce hazards by directing the way people work, they have the same control over ESG dimensions. The final control, Personal Protection, is a bit broader than the original PPE, going beyond the use of equipment and including elements such as, media communication training, cultural understanding, and other items that protect individuals from impacts.
The other tool that is already part of the mining industry that can be utilized to support ESG targets is the risk identification process. Risk identification can utilize three-dimensional models during risk review workshops to assess various risks. This generally applies to financial risks and hazards, which are then mitigated through The Hierarchy of Controls and brought to a lower risk threshold. It is recommended to adapt these tools to work with the indexing work done by Bascompta et al. (2022), to include all ESG risks into risk assessments and mitigate the impacts. The transformational change to the safety culture has taken over a decade to result in tangible results, therefore, it is recommended to use these tools that have already become part of culture to facilitate quicker adoption and integration into the mining industry to drive an ESG focused culture.
5.5 Partnerships
The analysis in Table 5, identifies the largest opportunity to influence change on ESG dimensions in the mining industry through digital twin technology is during the conceptual, study and engineering phases. This is related to the ability to run simulations to optimize results before construction and the use of the visual model to communicate to all stakeholders. During the design phases, data is provided from various services. The challenge with bringing the content from various providers together is the lack of interoperability between different platforms. Farrelly and Davies (2021) suggest that technological transformation will require software vendors to move beyond service providers and become partners with mining companies, and as such this would need to extend beyond mining companies to service providers partnering with other service providers. Therefore, it is recommended that service providers consider and evaluate partnerships to not only to improve collaboration through technology with mining companies, but also with other service providers to expand their offering.
6 Conclusion
The mining industry is not only the first step in the value chain, setting the foundation for all industries to meet their ESG goals, but mining is required for the increase in critical minerals needed to meet the sustainable technology demands to reduce the global temperature (The World Bank Group, 2020). Investors and stakeholders are demanding change, even when regulation and law are not keeping up, the expectation is greater than compliance. Therefore, to compete for investment, mining companies will need to go beyond regulations, they will need to optimize benefits while mitigating any negative impacts (Pennini, 2020). As the industry shifts from a reactive state to ESG dimensions to a proactive one, mining companies must illustrate shared value for both investors and society (Leonida, 2022a), and require the tools to do that.
This study has shown, through the review of case studies and theoretical application, that utilizing digital twin technologies could provide consistent measurement towards ESG targets and assist in advancing ESG goals through all project phases in mining. Table 5 demonstrates the potential to use digital twin technology to impact multiple ESG dimensions across mining project phases. The analysis conducted on this research shows that the early stages of engineering and planning of a project would have a greater opportunity to improve ESG dimensions through the use of three-dimensional models and simulations compared to the use of digital twin technology in other project phases.
Though the technology exists, application to the complexity and scale of a full mining site has not been proven. To drive this kind of transformational change in both the technological implementation and ESG advancement, strong leadership and the right talent will be critical to success. Utilizing tools that already exist in the mining industry for hazard identification and mitigation, could assist in streamlining the implementation and shortening the transition to an ESG driven culture, and provide much needed short-term wins. For both ESG dimensions and technological change to be successful, the mining industry will require a unified vision and short-term wins, but in the end the changes need to be institutionalized into the behaviors and culture of the industry (Kotter, 1995).
Data availability statement
The original contributions presented in the study are included in the article/supplementary material, further inquiries can be directed to the corresponding author.
Author contributions
RC conducted research, wrote, and edited the manuscript revision, and approved the submitted version.
Acknowledgments
Thank you to A. Khare for the encouragement and constructive feedback.
Conflict of interest
Author RC was employed by the company Stantec.
Publisher’s note
All claims expressed in this article are solely those of the authors and do not necessarily represent those of their affiliated organizations, or those of the publisher, the editors and the reviewers. Any product that may be evaluated in this article, or claim that may be made by its manufacturer, is not guaranteed or endorsed by the publisher.
References
Baek, J., Park, J., Cho, S., and Lee, C. (2022). 3D global localization in the underground mine environment using mobile LiDAR mapping and point cloud registration. Sensors 22 (8), 2873. doi:10.3390/s22082873
Bányai, Á., and Bányai, T. (2022). Real-time maintenance policy optimization in manufacturing systems: An energy efficiency and emission-based approach. Sustainability 14 (17), 10725. doi:10.3390/su141710725
Barn, B. S. (2022). “The sociotechnical sigital twin: on the gap between social and technical feasibility,” in 2022 IEEE 24th conference on business informatics (CBI), 11–20. doi:10.1109/CBI54897.2022.00009
Bascompta, M., Sanmiquel, L., Vintró, C., and Yousefian, M. (2022). Corporate social responsibility index for mine sites. Sustainability 14 (20), 13570. doi:10.3390/su142013570
BBC News (2021). Juukan Gorge: Rio Tinto investors in pay revolt over sacred cave blast. London: BBC News.
Bhatti, G., Mohan, H., and Raja Singh, R. (2021). “Towards the future of smart electric vehicles: Digital twin technology,” in Renewable and sustainable energy reviews (Germany: Elsevier Ltd), 141. doi:10.1016/j.rser.2021.110801
Bressanelli, G., Adrodegari, F., Pigosso, D. C. A., and Parida, V. (2022). Towards the smart circular economy paradigm: A definition, conceptualization, and research agenda. Sustainability 14 (9), 4960. doi:10.3390/su14094960
Butron, M. (2022). Rio Tinto reaches historic agreement with Juukan Gorge group. United Kingdom, Reuters. Available at: https://www.reuters.com/world/asia-pacific/rio-tinto-reaches-historic-agreement-with-juukan-gorge-group-2022-11-28/.
Cesar, S. (2021). Corporate social responsibility fit helps to earn the social license to operate in the mining industry. Resour. Policy 74, 101814. doi:10.1016/j.resourpol.2020.101814
Colorado School of Mines (2022). Mining engineering. Available at: https://catalog.mines.edu/undergraduate/programs/miningengineering/#coursestext.
Corrado, C. R., DeLong, S. M., Holt, E. G., Hua, E. Y., and Tolk, A. (2022). Combining green metrics and digital twins for sustainability planning and governance of smart buildings and cities. Sustainability 14 (20), 12988. doi:10.3390/su142012988
Curtin University (2023). Mining major (BSc science). Available at: https://handbook.curtin.edu.au/courses/course-ug-mining-major-bsc-science--mjru-mnengv1.
Deloitte (2022). Tracking the trends 2022 redefining mining. Available at: https://www.deloitte.com/global/en/Industries/mining-metals/analysis/tracking-the-trends-mining-industry.html?icid=globalReportEN#.
Domínguez-Gómez, J. A., and González-Gómez, T. (2021). Governance in mining: Management, ethics, sustainability and efficiency. Extr. Industries Soc. 8 (Issue 3), 100910. doi:10.1016/j.exis.2021.100910
Elbazi, N., Mabrouki, M., Chebak, A., and Hammouch, F. E. (2022). Digital twin architecture for mining industry: Case study of a stacker machine in an experimental open-pit mine. 2022 4th Glob. Power, Energy Commun. Conf. (GPECOM), 232–237. doi:10.1109/GPECOM55404.2022.9815618
Farrelly, C. T., and Davies, J. (2021). Interoperability, integration, and digital twins for mining—Part 1: Pathways to the network-centric mine. IEEE Ind. Electron. Mag. 15 (3), 13–21. doi:10.1109/MIE.2020.3029391
García, Á., Bregon, A., and Martínez-Prieto, M. A. (2022). Towards a connected digital twin learning ecosystem in manufacturing: Enablers and challenges. Comput. andIndustrial Eng. 171, 108463. doi:10.1016/j.cie.2022.108463
Hazrathosseini, A., and Moradi Afrapoli, A. (2023). The advent of digital twins in surface mining: Its time has finally arrived. Resour. Policy 80, 103155. doi:10.1016/j.resourpol.2022.103155
Hoeft, M., and Trask, C. (2022). Safety built right in: Exploring the occupational health and safety potential of BIM-based platforms throughout the building lifecycle. Sustainability 14 (10), 6104. doi:10.3390/su14106104
Hristov, P. O., Petrova-Antonova, D., Ilieva, S., and Rizov, R. (2022). Enabling city digital twins through urban living labs. Int. Archives Photogrammetry, Remote Sens. Spatial Inf. Sci. 43 (B1-2022), 151–156. doi:10.5194/isprs-archives-XLIII-B1-2022-151-2022
Jacobs, D. R., Laar, J. H., and Schutte, C. S. L. (2022). Strategy to identify and mitigate hazards in deep-level mine ventilation systems using a calibrated digital twin. South Afr. J. Industrial Eng. 33 (3), 204–217. doi:10.7166/33-3-2795
Jancewicz, K., Traczyk, A., and Migoń, P. (2021). Landform modifications within an intramontane urban landscape due to industrial activity, Wałbrzych, SW Poland. J. Maps 17 (4), 194–201. doi:10.1080/17445647.2020.1805805
Jenkins, H., and Yakovleva, N. (2006). Corporate social responsibility in the mining industry: Exploring trends in social and environmental disclosure. J. Clean. Prod. 14 (3–4), 271–284. doi:10.1016/j.jclepro.2004.10.004
Kang, K., Besklubova, S., Dai, Y., and Zhong, R. Y. (2022). Building demolition waste management through smart bim: A case study in Hong Kong. Waste Manag. 143, 69–83. doi:10.1016/j.wasman.2022.02.027
Kotter, J. P. (1995). Leading change: Why transformation efforts fail. Harv. Bus. Rev., 59–67. doi:10.1007/978-1-137-16511-4_7
Kumar, V. S., Begum, A. Y., Moniruzzaman, M., Sagar, K. V. D., Rao, L. M., and Santhosh, P. (2022). Battery management in electrical vehicles using machine learning techniques. J. Pharm. Negat. Results 13, 3213–3222. doi:10.47750/pnr.2022.13.S06.434
Kuykendall, T. (2021). Path to net-zero: Drive to lower emissions pays in metals, mining sector. SandP Global Market Intelligence. Available at: https://www.spglobal.com/marketintelligence/en/news-insights/latest-news-headlines/path-to-net-zero-drive-to-lower-emissions-pays-in-metals-mining-sector-67951431.
Lee, D., and Lee, S. (2021). Digital twin for supply chain coordination in modular construction. Appl. Sci. 11 (13), 5909. doi:10.3390/app11135909
Leonida, C. (2022a). Building better mining companies through ESG. Engineering and Mining Journal. Available at: https://www.e-mj.com/features/building-better-mining-companies-through-esg/.
Leonida, C. (2022b). Riding the risks and ppportunities of ESG in mining. Eng. Min. J., 40–45. Available at: www.e-mj.com.
Longo, F., Padovano, A., de Felice, F., Petrillo, A., and Elbasheer, M. (2023). From “prepare for the unknown” to “train for what’s coming”: A digital twin-driven and cognitive training approach for the workforce of the future in smart factories. J. Industrial Inf. Integration 32, 100437. doi:10.1016/j.jii.2023.100437
Manning, S. (2003). How do you create lasting organizational change? You must first slay grendel’s mother. Organ. Change Dev. 14, 195–224. doi:10.1016/s0897-3016(03)14083-9
McGill University (2023). Mining engineering. Available at: https://www.mcgill.ca/study/2022-2023/faculties/engineering/undergraduate/programs/bachelor-engineering-beng-mining-engineering.
McQueen, V. (2021). ESG concerns grow as miners rank environment, social issues and decarbonization as top risks for 2022. Ernst and Young Global Limited. Available at: https://www.ey.com/en_ca/news/2021/10/esg-concerns-grow-as-miners-rank-environment-social-issues-and-decarbonization-as-top-risks-for-2022.
Meža, S., Mauko Pranjić, A., Vezočnik, R., Osmokrović, I., and Lenart, S. (2021). Digital twins and road construction using secondary raw materials. J. Adv. Transp., 2021, 1, 12. doi:10.1155/2021/8833058
Morse, I. (2020). Mining industry releases first standard to improve safety of waste storage. Mongabay Series: Land Rights and Extractives. Available at: https://news.mongabay.com/2020/08/industry-releases-first-standard-to-improve-safety-of-mine-waste-storage/#:∼:text=Released%20Aug.%205%2C%20the%20Global%20Industry%20Standard%20on,the%20safe%20disposal%20of%20mine%20waste%2C%20called%20tailings.
Neethling, P. (2021). How digital twins can help safeguard tailings facilities. Can. Min. J., 36–38. Available at: https://www.canadianminingjournal.com/featured-article/how-digital-twins-can-help-safeguard-tailings-facilities/.[
Newrzella, S. R., Franklin, D. W., and Haider, S. (2022). Methodology for digital twin use cases: Definition, prioritization, and implementation. IEEE Access 10, 75444–75457. doi:10.1109/ACCESS.2022.3191427
Nitlarp, T., and Kiattisin, S. (2022). The Impact factors of industry 4.0 on ESG in the energy sector. Sustainability 14 (15), 9198. doi:10.3390/su14159198
Peng, B., Xiao, X., Zhao, B., and Li, H. (2022). Application of digital twins in high precision geological hazard survey and prevention in Beijing. The International Archives of the Photogrammetry. Remote Sens. Spatial Inf. Sci. W1, 39–44. doi:10.5194/isprs-archives-xlviii-3-w1-2022-39-2022
Pennini, A. (2020). The ESG bar has just been raised. Who will get over it? Min. J. Available at: https://www.mining-journal.com/partners/partner-content/1383066/the-esg-bar-has-just-been-raised-who-will-get-over-it.
PennState (2023). Mining engineering major. Available at: https://view.officeapps.live.com/op/view.aspx?src=https%3A%2F%2Fwww.eme.psu.edu%2Fsites%2Fwww.eme.psu.edu%2Ffiles%2Fdocuments%2Fundergraduate%2Fmnge_sp_2023_cos.docxandwdOrigin=BROWSELINK.
Philip, O. (2020). 12 best mining engineering schools in the world 2020. Available at: https://collegereporters.com/mining-engineering-schools-world/.
Pileggi, P., Lazovik, E., Broekhuijsen, J., Borth, M., and Verriet, J. (2020). “Lifecycle governance for effective digital twins: a joint systems engineering and IT perspective,” in 2020 IEEE International Systems Conference (SysCon), Montreal, QC, Canada, 2020, 1–8. doi:10.1109/SysCon47679.2020.9275662
Pimonenko, T., Bilan, Y., Horák, J., Starchenko, L., and Gajda, W. (2020). Green brand of companies and greenwashing under sustainable development goals. Sustainability 12 (4), 1679. doi:10.3390/su12041679
Plachinda, P., Morgan, J., and Coelho, M. (2022). Towards net zero: Modeling approach to the right-sized facilities. Sustainability 15 (1), 163. doi:10.3390/su15010163
Popescu, D., Dragomir, M., Popescu, S., and Dragomir, D. (2022). Building better digital twins for production systems by incorporating environmental related functions—literature analysis and determining alternatives. Appl. Sci. 12 (17), 8657. doi:10.3390/app12178657
Preut, A., Kopka, J. P., and Clausen, U. (2021). Digital twins for the circular economy. Sustainability 13 (18), 10467. doi:10.3390/su131810467
Report (2018). Dassault partners with ATC Williams for digital twin. Eng. Min. J. 98. Available at: www.e-mj.com.
Sairinen, R., Sidorenko, O., and Tiainen, H. (2021). A research framework for studying social impacts: Application to the field of mining. Environ. Impact Assess. Rev. 86, 106490. doi:10.1016/j.eiar.2020.106490
Saxena, A., Singh, R., Gehlot, A., Akram, S. V., Twala, B., Singh, A., et al. (2022). Technologies empowered environmental, social, and governance (ESG): An industry 4.0 landscape. Sustainability 15 (1), 309. doi:10.3390/su15010309
Senadheera, S. S., Withana, P. A., Dissanayake, P. D., Sarkar, B., Chopra, S. S., Rhee, J. H., et al. (2021). Scoring environment pillar in environmental, social, and governance (ESG) assessment. Sustain. Environ. 7 (1). doi:10.1080/27658511.2021.1960097
Shen, K., Ding, L., and Wang, C. C. (2022). Development of a framework to support whole-life-cycle net-zero-carbon buildings through integration of building information modelling and digital twins. Buildings 12 (10). doi:10.3390/buildings12101747
Solman, H., Kirkegaard, J. K., Smits, M., van Vliet, B., and Bush, S. (2022). Digital twinning as an act of governance in the wind energy sector. Environ. Sci. Policy 127, 272–279. doi:10.1016/j.envsci.2021.10.027
Tancev, G., and Toro, F. G. (2022). Towards a digital twin for air quality monitoring networks in smart cities. ISC2 2022 - 8th IEEE International Smart Cities Conference. 26-29 September 2022, Cyprus, IEEE, doi:10.1109/ISC255366.2022.9921782
The World Bank Group (2020). Climate-smart mining: Minerals for climate action. The World Bank. Available at: https://www.worldbank.org/en/topic/extractiveindustries/brief/climate-smart-mining-minerals-for-climate-action.
Ulwick, A. W. (2002). Turn customer input into innovation. Harv. Bus. Rev. 80, 91–126. Available at: https://hbr.org/2002/01/turn-customer-input-into-innovation#:∼:text=Turn%20Customer%20Input%20into%20Innovation%201%20Step%201%3A,5%3A%20Use%20the%20outcomes%20to%20jump-start%20innovation.%20.
University of Alberta (2023). Mining engineering. Available at: https://www.ualberta.ca/index.html.
Wang, X. (2022). Risk assessment of smart coal mine safety mining based on BIM. 2022 World Autom. Congr. (WAC) 2022, 548–552. doi:10.23919/WAC55640.2022.9934120
Woźniak, J., Pactwa, K., Szczęśniewicz, M., and Ciapka, D. (2022). Declaration of the sustainable development goals of mining companies and the effect of their activities in selected areas. Sustainability 14 (24), 16422. doi:10.3390/su142416422
Wu, Y., and Li, Y. (2022). Digital twin-driven performance optimization for hazardous waste landfill systems. Math. Problems Eng., 2022, 1, 10. doi:10.1155/2022/7778952
Zekri, S., Jabeur, N., and Gharrad, H. (2022). Smart water management using intelligent digital twins. Comput. Inf. 41, 135–153. doi:10.31577/cai_2022_1_135
Zhang, L., Ge, D., Guo, X., Liu, B., Li, M., and Wang, Y. (2020). InSAR monitoring surface deformation induced by underground mining using Sentinel-1 images. Proc. Int. Assoc. Hydrological Sci. 382, 237–240. doi:10.5194/piahs-382-237-2020
Zhao, Y., Wang, N., and Liu, Z. (2022). An established theory of digital twin model for tunnel construction safety assessment. Appl. Sci. 12 (23), 12256. doi:10.3390/app122312256
Keywords: digital twins, mining industry, sustainability, environmental, social, governance
Citation: Cranford R (2023) Conceptual application of digital twins to meet ESG targets in the mining industry. Front. Ind. Eng. 1:1223989. doi: 10.3389/fieng.2023.1223989
Received: 17 May 2023; Accepted: 07 July 2023;
Published: 21 July 2023.
Edited by:
Abu Haddud, University of Southern Mississippi, United StatesReviewed by:
Annamária Behúnová, Technical University of Košice, SlovakiaIonica Oncioiu, Titu Maiorescu University, Romania
Copyright © 2023 Cranford. This is an open-access article distributed under the terms of the Creative Commons Attribution License (CC BY). The use, distribution or reproduction in other forums is permitted, provided the original author(s) and the copyright owner(s) are credited and that the original publication in this journal is cited, in accordance with accepted academic practice. No use, distribution or reproduction is permitted which does not comply with these terms.
*Correspondence: Rachel Cranford, cmFjaGVsLmNyYW5mb3JkQG91dGxvb2suY29t