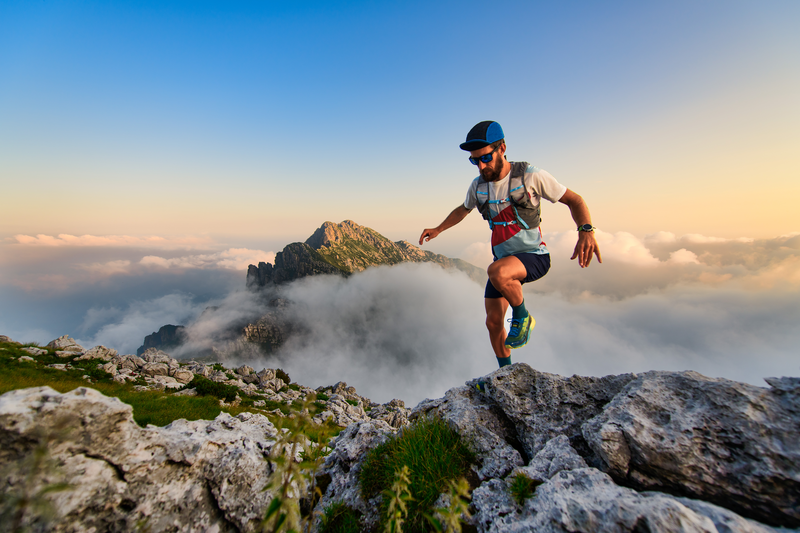
95% of researchers rate our articles as excellent or good
Learn more about the work of our research integrity team to safeguard the quality of each article we publish.
Find out more
EDITORIAL article
Front. Immunol. , 03 April 2025
Sec. Inflammation
Volume 16 - 2025 | https://doi.org/10.3389/fimmu.2025.1587075
This article is part of the Research Topic Crosstalk in Ferroptosis, Immunity & Inflammation View all 25 articles
Editorial on the Research Topic
Crosstalk in ferroptosis, immunity & inflammation
Ferroptosis, an iron-dependent form of regulated cell death characterized by iron overload and lipid peroxidation, has gained significant attention because of its involvement in various pathological conditions, including cancer, neurodegenerative diseases, and inflammatory disorders (1, 2). The intricate interplay between ferroptosis, immune responses, and inflammatory pathways remains a key area of research, with implications for therapeutic strategies targeting these mechanisms. This Research Topic, “Crosstalk in Ferroptosis, Immunity & Inflammation,” presents a collection of 24 articles that explore the multifaceted roles of ferroptosis across various disease contexts. By examining how ferroptotic processes intersect with immune responses and inflammatory pathways, these studies shed light on potential mechanisms underpinning a broad spectrum of conditions—from inflammatory and autoimmune disorders to cancer, neurodegeneration, metabolic diseases, and organ-specific injuries.
Paper in this Research Topic discuss the pivotal role of ferroptosis in the pathogenesis of inflammatory diseases such as inflammatory bowel disease (IBD) (Xie et al.), systemic lupus erythematosus (SLE) (Zhao et al.), and lupus nephritis (Fan et al.). Iron metabolism dysregulation and oxidative stress-induced cell death contribute significantly to these pathologies. Investigations into immune imbalances, particularly T-cell-mediated responses, underscore the link between ferroptotic pathways and chronic inflammation in autoimmune disorders. Understanding these mechanisms offers potential therapeutic strategies to mitigate disease progression by targeting ferroptosis-related pathways. Furthermore, oxidative stress and lipid peroxidation—hallmarks of ferroptosis—have been shown to amplify inflammatory responses in chronic diseases (3). The delicate balance between ferroptotic cell death and immune regulation is crucial for maintaining tissue homeostasis. Research suggests that inhibiting ferroptosis may protect against excessive inflammation, while the controlled induction of ferroptosis could be leveraged to eliminate hyperactive immune cells that contribute to autoimmune diseases (4).
Ferroptosis is increasingly recognized as a key factor in tumor biology (5, 6). Xia et al. have implicated ferroptosis in cancers, including colorectal cancer, hepatocellular carcinoma (Yang et al.), and lung cancer (Gao and Zhang). Regulators such as SLC7A11 in ferroptosis emerges as promising targets for tumor therapy (7) (Jiang and Sun). Ferroptosis appears to synergize with immunotherapy to enhance antitumor responses, offering new perspectives for cancer treatment. The emerging role of long noncoding RNAs (lncRNAs) in regulating ferroptosis sheds light on novel molecular interactions influencing tumor progression (Chen et al.). Additionally, ferroptosis-based therapies may overcome resistance to traditional chemotherapies and improve patient outcomes by modulating the tumor microenvironment. One of the key mechanisms by which ferroptosis influences cancer progression is through its impact on immune cell infiltration and tumor-associated macrophages. Moreover, the release of damage-associated molecular patterns (DAMPs) from ferroptotic tumor cells can activate antigen-presenting cells, thereby modulating adaptive immune response. Harnessing ferroptosis offers exciting avenues for overcoming chemoresistance and improving the efficacy of immune checkpoint inhibitors.
Ferroptosis has emerged as a key player in neurodegenerative diseases, contributing to neuronal damage through iron accumulation and oxidative stress. In Alzheimer’s disease and Parkinson’s diseases, iron accumulation and lipid peroxidation contribute to neuronal loss and cognitive decline. Additionally, ferroptosis is involved in neuroinflammation by modulating microglial activation, which in turn influences neuronal survival and disease progression. Emerging therapeutic strategies—ranging from antioxidant-based approaches to iron chelation—are being explored to protect neuronal integrity by targeting ferroptotic pathways. In addition to classical neurodegenerative disorders, ferroptosis also plays a critical role in sepsis-associated encephalopathy (SAE), a severe neurological complication of sepsis. Microglia-derived CXCL2 has been shown to promote neuronal ferroptosis in SAE by activating Jun, exacerbating cognitive dysfunction and neuronal loss. Inhibiting CXCL2 or Jun has demonstrated neuroprotective effects, suggesting that targeting ferroptosis could be a novel therapeutic strategy for mitigating SAE-induced brain injury (Yang et al.).
Chronic metabolic disorders, including diabetes and obesity, are often accompanied by ferroptosis-driven tissue damage. Hyperglycemia-induced oxidative stress can lead to ferroptotic cell death in renal and vascular tissues, contributing to diabetic nephropathy and atherosclerosis. Understanding the metabolic regulation of ferroptosis could help in designing effective interventions for metabolic syndrome and related disorders (8). Additionally. Liu et al. have identified key ferroptosis-related genes involved in endometriosis pathogenesis, highlighting the potential for ferroptosis-targeting therapies to mitigate disease progression and immune dysfunction.
Kidney diseases is a typical metabolic disorder. Dysregulation of iron homeostasis during ferroptotic processes significantly influences progression of kidney diseases, including acute kidney injury and renal fibrosis (9, 10) (Long et al.). While alterations in lipid metabolism also modulate ferroptotic cell death. These findings highlight the need for further mechanistic studies to fully understand these pathways. In renal ischemia–reperfusion (I/R) injury, ferroptosis contributes to kidney damage, which is characterized by increased collagen deposition, α-SMA expression, and oxidative stress. Fer-1 treatment or ATF3 knockdown alleviated these changes while modulating Nrf2/HO-1 signaling. Additionally, exosomal miR-1306-5p release under hypoxia-reoxygenation promoted M2 macrophage polarization, linking ferroptosis to immune regulation in renal fibrosis (Tang et al.). Moreover, Li et al. ‘s study investigating the role of ferroptosis in acute kidney injury (AKI) revealed that GSTT1 and GSTM1 were significantly downregulated in the renal tubular cells of AKI patients and in cisplatin-treated mice. Notably, Gstm1/Gstt1 double knockout (DKO) mice, while phenotypically normal under baseline conditions, presented exacerbated kidney dysfunction, increased ROS levels, and severe ferroptosis following cisplatin administration, underscoring the contribution of GST enzymes to ferroptotic resistance in renal injury.
In chronic obstructive pulmonary disease (COPD), ferroptosis-mediated alveolar epithelial cell death exacerbates disease progression. Exposure to electronic nicotine delivery systems (ENDS) has been linked to worsening COPD features, including emphysema, mucus accumulation, inflammation, and fibrosis, all of which are associated with lipid dysregulation and ferroptotic injury(Han et al.). Beyond pulmonary diseases, ferroptosis also plays a crucial role in I/R injury, a pathological process affecting multiple organ systems, including the heart, brain, lung and kidneys. Ferroptotic cell death contributes to endothelial dysfunction, which in turn promotes vascular inflammation and atherosclerosis. In cardiovascular conditions such as heart failure and myocardial infarction, oxidative stress-induced ferroptosis of cardiomyocytes accelerates disease progression, particularly in cases involving iron overload. Additionally, ferroptosis has been implicated in cerebral I/R injury, where it exacerbates neuronal damage and neuroinflammation (He et al.), further linking ferroptotic mechanisms to both cardiovascular and neurological pathologies. Given its broad impact, targeting ferroptosis represents a promising therapeutic strategy for mitigating I/R injury, reducing vascular complications, and improving outcomes in cardiopulmonary diseases.
The rapid evolution of ferroptosis research has opened new therapeutic frontiers across various medical disciplines. Studies exploring anastasis—the recovery from ferroptotic stress—could unveil novel mechanisms of cellular resilience. Advanced bioinformatics analyses have identified key molecular signatures linking ferroptosis with immune infiltration, further reinforcing the importance of computational approaches in this field. Looking ahead, the development of reliable biomarkers, targeted inhibitors, and inducers of ferroptosis will be crucial. Moreover, integrating ferroptosis modulation with immunotherapy holds significant promise for clinical translation across a range of conditions, particularly in oncology and inflammatory diseases.
This Research Topic provides valuable insights into the crosstalk between ferroptosis, immunity, and inflammation across diverse disease models. By understanding its molecular mechanisms and interactions with immune responses, inflammation, and metabolic pathways, we can identify novel therapeutic targets and improve treatment strategies for a wide range of conditions. Future research should focus on ferroptosis-modulating strategies to enhance therapeutic efficacy, minimize disease progression, and improve patient outcomes. The findings presented in this Research Topic pave the way for future investigations and potential clinical applications, emphasizing the critical role of ferroptosis in immune regulation and disease pathogenesis.
YL: Investigation, Resources, Writing – original draft, Writing – review & editing. GW: Investigation, Writing – original draft. LF: Investigation, Writing – original draft. QF: Conceptualization, Visualization, Writing – review & editing. HZ: Conceptualization, Investigation, Writing – review & editing.
The authors declare that the research was conducted in the absence of any commercial or financial relationships that could be construed as a potential conflict of interest.
The author(s) declare that no Generative AI was used in the creation of this manuscript.
All claims expressed in this article are solely those of the authors and do not necessarily represent those of their affiliated organizations, or those of the publisher, the editors and the reviewers. Any product that may be evaluated in this article, or claim that may be made by its manufacturer, is not guaranteed or endorsed by the publisher.
1. Jiang X, Stockwell BR, Conrad M. Ferroptosis: mechanisms, biology and role in disease. Nat Rev Mol Cell Biol. (2021) 22:266–82. doi: 10.1038/s41580-020-00324-8
2. Xu L, Liu Y, Chen X, Zhong H, Wang Y. Ferroptosis in life: To be or not to be. BioMed Pharmacother. (2023) 159:114241. doi: 10.1016/j.biopha.2023.114241
3. Tang D, Chen X, Kang R, Kroemer G. Ferroptosis: molecular mechanisms and health implications. Cell Res. (2021) 31:107–25. doi: 10.1038/s41422-020-00441-1
4. Lai B, Wu CH, Wu CY, Luo SF, Lai JH. Ferroptosis and autoimmune diseases. Front Immunol. (2022) 13:916664. doi: 10.3389/fimmu.2022.916664
5. Liu Y, Lu S, Wu LL, Yang L, Yang L, Wang J. The diversified role of mitochondria in ferroptosis in cancer. Cell Death Dis. (2023) 14:519. doi: 10.1038/s41419-023-06045-y
6. Gu X, Liu Y, Dai X, Yang YG, Zhang X. Deciphering the potential roles of ferroptosis in regulating tumor immunity and tumor immunotherapy. Front Immunol. (2023) 14:1137107. doi: 10.3389/fimmu.2023.1137107
7. Wang X, Chen Y, Wang X, Tian H, Wang Y, Jin J, et al. Stem cell factor SOX2 confers ferroptosis resistance in lung cancer via upregulation of SLC7A11. Cancer Res. (2021) 81:5217–29. doi: 10.1158/0008-5472.CAN-21-0567
8. Ajoolabady A, Aslkhodapasandhokmabad H, Libby P, Tuomilehto J, Lip GYH, Penninger JM, et al. Ferritinophagy and ferroptosis in the management of metabolic diseases. Trends Endocrinol Metab. (2021) 32:444–62. doi: 10.1016/j.tem.2021.04.010
9. Feng Q, Yang Y, Ren K, Qiao Y, Sun Z, Pan S, et al. Broadening horizons: the multifaceted functions of ferroptosis in kidney diseases. Int J Biol Sci. (2023) 19:3726–43. doi: 10.7150/ijbs.85674
Keywords: ferroptosis, immunity, inflammation, cancer, other diseases
Citation: Liu Y, Wu G, Feng L, Feng Q and Zhong H (2025) Editorial: Crosstalk in ferroptosis, immunity & inflammation. Front. Immunol. 16:1587075. doi: 10.3389/fimmu.2025.1587075
Received: 03 March 2025; Accepted: 17 March 2025;
Published: 03 April 2025.
Edited and Reviewed by:
Pietro Ghezzi, Brighton and Sussex Medical School, United KingdomCopyright © 2025 Liu, Wu, Feng, Feng and Zhong. This is an open-access article distributed under the terms of the Creative Commons Attribution License (CC BY). The use, distribution or reproduction in other forums is permitted, provided the original author(s) and the copyright owner(s) are credited and that the original publication in this journal is cited, in accordance with accepted academic practice. No use, distribution or reproduction is permitted which does not comply with these terms.
*Correspondence: Hua Zhong, aHpob25nQGFjYy5oYXdhaWkuZWR1; Qi Feng, ZmVuZ3FpMjAxOUB6enUuZWR1LmNu; Yu’e Liu, WXVlLmxpdUBjaGlsZHJlbnMuaGFydmFyZC5lZHU=
†These authors have contributed equally to this work
Disclaimer: All claims expressed in this article are solely those of the authors and do not necessarily represent those of their affiliated organizations, or those of the publisher, the editors and the reviewers. Any product that may be evaluated in this article or claim that may be made by its manufacturer is not guaranteed or endorsed by the publisher.
Research integrity at Frontiers
Learn more about the work of our research integrity team to safeguard the quality of each article we publish.