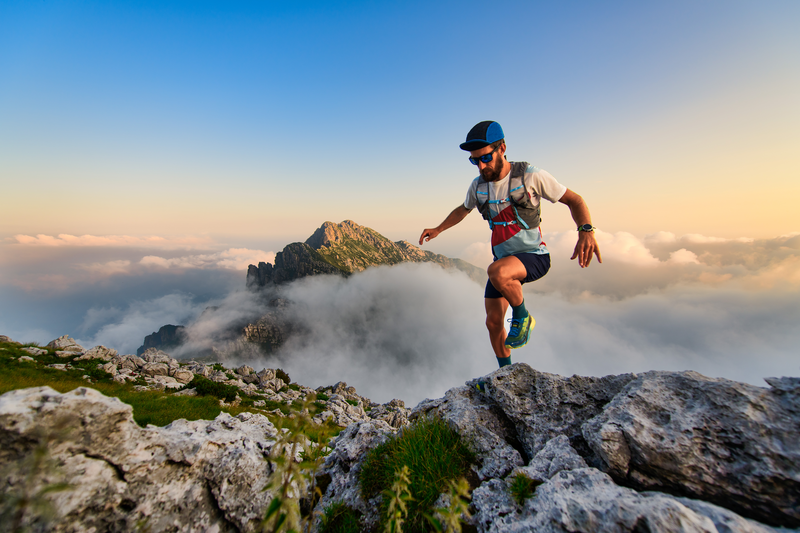
94% of researchers rate our articles as excellent or good
Learn more about the work of our research integrity team to safeguard the quality of each article we publish.
Find out more
EDITORIAL article
Front. Immunol.
Sec. Microbial Immunology
Volume 16 - 2025 | doi: 10.3389/fimmu.2025.1582489
This article is part of the Research Topic Nontuberculous Mycobacterial Pulmonary Disease: Immunopathogenesis and Immunological Risk Factors View all 6 articles
The final, formatted version of the article will be published soon.
You have multiple emails registered with Frontiers:
Please enter your email address:
If you already have an account, please login
You don't have a Frontiers account ? You can register here
Mycobacteria are comprised of Mycobacterium tuberculosis complex, Mycobacterium leprae, and non-tuberculous mycobacteria (NTM). While NTM contain approximately 200 distinct species and subspecies, diseases in humans are predominantly caused by three main groups: (i) Mycobacterium avium complex (which is comprised of several members, with the most clinically relevant being M. avium subsp hominissuis, M. intracellulare subsp intracellulare, and M. intracellulare subsp chimaera);(ii) Mycobacterium abscessus group (which consists of three subspecies: abscessus, massiliense, and bolletii); and (iii) Mycobacterium kansasii complex. NTM are ubiquitous in natural and built environs, especially in freshwater, soil, and biofilms, making NTM infections environmentally-acquired.Upon encountering a patient with an NTM infection, four elements should be determined: the specific NTM responsible, how the infection was contracted, the location and extent of the infection, and the presence of any host risk factors. The last two elements are interrelated because the degree of underlying host risk factors often dictates the scope of the infection. Thus, it is useful to categorize the NTM infection into one of four main types: (i) skin, soft tissue, and osteoarticular infections, which are typically due to accidental trauma or medical procedures; (ii) isolated infection of the head and neck lymph nodes (also known as cervical lymphadenitis or "scrofula"); (iii) isolated NTM lung disease; (iv) Hicks and colleagues (https://doi.org/10.3389/fimmu.2023.1078976) reported three infants with complete DiGeorge anomaly (cDGA) -a genetic condition resulting in the complete absence of the thymus resulting in severe T cell deficiency -all with disseminated NTM infections. These cases are rare, likely due to the highly infrequent intersection of two uncommon conditions (DGA and NTM infection). The authors recommend that DGA should be considered in the differential diagnoses of children with disseminated NTM infections. Currently, mutations of genes that encode for elements of the interferon-gamma (IFNg)-interleukin-12 (IL-12) axis should be suspected in very young children with disseminated NTM disease. In adults with disseminated NTM infections, acquired immune disorders like AIDS, anti-IFNg antibody syndrome, or use of immunosuppressive drugs are more likely risk factors.disseminatedVarious mouse strains have been used to explore host susceptibility to NTM infections. However, most murine infection models result in systemic NTM infection rather than exhibit isolated NTM lung disease, the latter characterized by bronchiolitis and bronchiectasis in humans. Yang and colleagues (https://doi.org/10.3389/fimmu.2023.1277745) developed a mouse model using intratracheal infection with M. abscessus embedded in agar beads, which better simulates airway infection. The infected mice developed granulomas, a common feature M. abscessus infection in humans. Others have also employed a fibrin plug model where M. abscessus is suspended in thrombin and fibrinogen, trapping the bacteria in the airways as the plugs coagulate (1). Models such as these intuitively more closely replicate isolated NTM lung disease. Future studies could investigate whether such inoculation methods also enhance NTM biofilm formation in the airways, a barrier known to resist penetration by immune cells and antibiotics.The co-evolution of humans and mycobacteria has enabled reciprocal adaptation in a mutual struggle for dominance. A recurring example is that the same host factors or mycobacterial components can be used by either side to gain an advantage, analogous to a "tug o' war" event. Wang and colleagues (https://doi.org/10.3389/fimmu.2024.1413947) reviewed the role of CD36, a cell surface scavenger receptor which mycobacteria have hijacked for their own advantage. Although their focus was on M. tuberculosis, similar mechanisms may also be involved in the pathogenesis of NTM disease. They discussed that M. tuberculosis engages CD36 leading to: (i) increased fatty acid uptake by host cells, aiding bacillary persistence; (ii) monocyte differentiation into macrophages with an immunosuppressive phenotype; and (iii) enhanced uptake of surfactant lipids by macrophages, supporting mycobacterial growth. Additionally, they highlighted studies showing CD36 expression in various cell types that are involved in granuloma formation, including macrophages, granulocytes, lymphocytes, and fibroblasts. It was previously demonstrated that the M. avium complex manipulates macrophage lipid metabolism, leading to the formation of lipid-laden foamy macrophages that create a favorable environment for mycobacterial growth (2). Recent studies using a mouse model demonstrated that CD36 expression is elevated on macrophages during M. avium infection (3). In line with this, inhibiting CD36 has been shown to reduce the growth of M. avium. Choi et al (4) confirmed that blocking CD36 leads to decreased growth of M. avium, which aligns with earlier research. These findings highlight the crucial role of CD36 in the pathology of mycobacterial diseases as well as a potential therapeutic target.Bronchiectasis, a common feature of chronic NTM lung disease, is characterized by repeated cycles of infection and inflammation. Airway injury may be exacerbated by NTM-generated biofilms, a complex matrix of extracellular polymeric substances -comprised of DNA, proteins, polysaccharides, and lipids -released from live and dead microbes (5)(6)(7)(8)(9). NTM lung cavity walls have also been demonstrated to contain biofilms (10). This biofilm matrix provides protection and nutrients for the microbes (11). Keefe and colleagues (https://doi.org/10.3389/fimmu.2025.1508584) identified gene products from M. abscessus in biofilms using a transposon library of M. abscessus along with surface proteomic analysis. They uncovered specific proteins related to the mycobacteria's ability to detach and establish new biofilms as well as attach to and infect both epithelial cells and macrophages. The primary treatment for NTM lung disease involves antibiotics, airway clearance, and targeted therapies for underlying conditions, e.g., anti-retroviral drugs for HIV infection, modulators for defective cystic fibrosis transmembrane conductance regulator, and alpha-1-antitrypsin (AAT) replacement therapy for those with AAT deficiency. For those with disseminated NTM disease, host-directed therapies may include IFNg replacement therapy for subjects with defects in the IFNg-IL-12 axis, anti-B cell therapy for those with anti-IFNg autoantibodies, and immune checkpoint inhibitors for individuals with severe T cell immunosuppression (12,13), though the lattermost is controversial (13,14). Adjunct therapies directed more specifically against the mycobacteria include inhibitors of M. abscessus blactamase for those on b-lactam antibiotics (15), efflux pump inhibitors (16), and biofilm disrupters (8).Mediaas and colleagues (https://doi.org/10.3389/fimmu.2024.1463224) found that metformin, commonly used to treat diabetes mellitus, significantly reduced M. avium burden in mice. One mechanism for this salubrious effect was by enhancing the ability of macrophages to kill or control the infection by activating the AMPK (5' adenosine monophosphate-activated protein kinase) pathway, increasing mitochondrial reactive oxygen species and enhancing phagosome maturation and acidification.In summary, the five articles featured in this special issue enhance our understanding of the pathogenesis of NTM infections. This increase in knowledge is crucial since we are all likely exposed to NTM and yet NTM infections occur in relatively few individuals. The interplay between specific host susceptibility and NTM virulence factors is likely critical in the development of NTM disease. Each of these papers contributes to our collective understanding of this relationship. Ongoing research into innovative strategies and therapies, as highlighted by these five papers, is essential for improving outcomes for patients with NTM disease.
Keywords: NTM (nontuberculous mycobacteria), NTM infections, NTM disease, NTM host interaction, NTM therapy
Received: 24 Feb 2025; Accepted: 10 Mar 2025.
Copyright: © 2025 Chan and Schmitz. This is an open-access article distributed under the terms of the Creative Commons Attribution License (CC BY). The use, distribution or reproduction in other forums is permitted, provided the original author(s) or licensor are credited and that the original publication in this journal is cited, in accordance with accepted academic practice. No use, distribution or reproduction is permitted which does not comply with these terms.
* Correspondence:
Edward Chan, Department of Academic Affairs, National Jewish Health, Denver, Colorado, United States
Disclaimer: All claims expressed in this article are solely those of the authors and do not necessarily represent those of their affiliated organizations, or those of the publisher, the editors and the reviewers. Any product that may be evaluated in this article or claim that may be made by its manufacturer is not guaranteed or endorsed by the publisher.
Research integrity at Frontiers
Learn more about the work of our research integrity team to safeguard the quality of each article we publish.