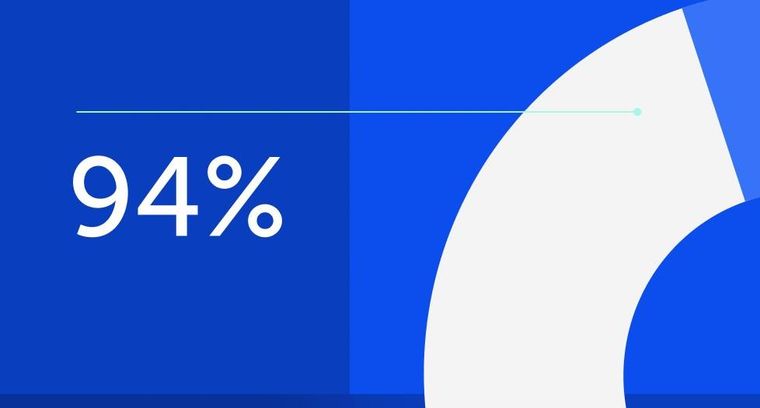
94% of researchers rate our articles as excellent or good
Learn more about the work of our research integrity team to safeguard the quality of each article we publish.
Find out more
MINI REVIEW article
Front. Immunol., 21 March 2025
Sec. Cancer Immunity and Immunotherapy
Volume 16 - 2025 | https://doi.org/10.3389/fimmu.2025.1573917
This article is part of the Research TopicHarnessing Molecular Insights for Enhanced Drug Sensitivity and Immunotherapy in CancerView all 28 articles
Colon cancer (CC) remains a primary contributor to cancer-related fatalities worldwide, driven by difficulties in early diagnosis and constrained therapeutic options. Recent studies underscore the importance of the tumor microenvironment (TME), notably tumor-associated macrophages (TAMs), in fostering malignancy progression and therapy resistance. Through their inherent plasticity, TAMs facilitate immunosuppression, angiogenic processes, metastatic spread, and drug tolerance. In contrast to M1 macrophages, which promote inflammatory and tumoricidal responses, M2 macrophages support tumor expansion and dissemination by exerting immunosuppressive and pro-angiogenic influences. Consequently, manipulating TAMs has emerged as a potential avenue to enhance treatment effectiveness. This review outlines the origins, polarization states, and functions of TAMs in CC, highlights their role in driving tumor advancement, and surveys ongoing efforts to target these cells for better patient outcomes. Emerging therapeutic strategies aimed at modulating TAM functions - including depletion strategies, reprogramming approaches that shift M2-polarized TAMs toward an M1 phenotype, and inhibition of key signaling pathways sustaining TAM-mediated immunosuppression-are currently under active investigation. These approaches hold promise in overcoming TAM - induced resistance and improving immunotherapeutic efficacy in CC.
Colon cancer (CC), a frequent malignancy of the gastrointestinal tract, has outcomes and therapeutic responses shaped by various contributing factors (1). More than one million new diagnoses emerge across the globe annually, making it the second leading contributor to cancer-associated mortality (2). Limited survival rates largely stem from obstacles in early detection and a paucity of potent treatment strategies. Current protocols primarily center on surgery, complemented by radiation and chemotherapy. As overall health standards advance, there is a pressing demand for novel therapeutic avenues and refined prognostic tools (3–5). Recent findings underscore the importance of targeting the tumor microenvironment (TME) alongside conventional interventions to bolster treatment efficacy (6–9).
Established malignancies often present a suppressive microenvironment that impairs antitumor immunity and drives cancer progression (10, 11). Within these “immune evasion mechanisms,” tumor cells circumvent normal immune processes by modifying antigen presentation, releasing suppressive signals that promote lymphocyte apoptosis, or engaging detrimental regulatory pathways (12–14). These strategies evolve over the course of tumor development, growing ever more varied and complex. Consequently, disrupting these pathways and reactivating host immunity remain significant hurdles for tumor immunotherapy. The underlying factors behind tumor evasion are highly intricate, with the TME exerting a pivotal influence. Macrophages, which can comprise up to half of the tumor’s cellular mass, are the most abundant infiltrating immune cells in the TME (15). Termed tumor-associated macrophages (TAMs), they facilitate tumor establishment, metastatic spread, immune suppression, and vascularization (16–18).
In the setting of CC, TAMs play a central role in disease onset and progression, highlighting their potential as immunotherapeutic targets. Yet, their functions are multifaceted, and either depleting these macrophages or modifying their phenotypes may boost anti-tumor immunotherapy (19). This review synthesizes current findings on TAM origins, polarization states, and overall roles. It also examines how TAMs facilitate CC initiation and progression, underscores emerging TAM-focused strategies for personalized diagnostics and treatment, and outlines prospective challenges. These insights are intended to spur the development of TAM-oriented precision medicine in CC.
Monocytes and macrophages circulating in the bloodstream represent the principal source of TAMs. Their entry into specific regions of the TME is governed by various cytokines, including C-C motif chemokine ligand 2/5/7 (CCL2/5/7), C-X-C motif chemokine ligand 8/12 (CXCL8/12), vascular endothelial growth factor (VEGF), and transforming growth factor-β (TGF-β) (20). Macrophages exhibit notable plasticity, transitioning into M1 or M2 phenotypes depending on local cues. M1 TAMs primarily initiate Th1 responses, amplify inflammation, and exert tumoricidal activity (21, 22). M2 TAMs secrete VEGF, matrix metalloproteinases (MMPs), and epidermal growth factor (EGF), displaying elevated IL-10 and reduced IL-12 levels. M2 TAMs predominantly engage in Th2-oriented immune pathways, facilitate angiogenesis and extracellular matrix remodeling, and play vital anti-inflammatory roles in wound repair. Currently, most TAMs within tumor stroma adopt the M2 phenotype, which promotes tumor initiation, disease progression, and eventual metastasis, thus serving as a prognostic marker in oncology (23, 24). TAM polarization is governed by multiple, dynamic signals from the TME. Classical M1 polarization is driven by factors such as lipopolysaccharide (LPS) and interferon-γ, which increase interleukin-2 (IL-2) while reducing IL-10 (21, 22). Conversely, M2 TAMs are polarized when exposed to IL-10, IL-4, and IL-13, displaying elevated IL-10 and reduced IL-12 levels (25).
TME is predominantly composed of immune cells, including T lymphocytes, neutrophils, natural killer (NK) cells, macrophages, dendritic cells, and myeloid-derived suppressor cells (MDSCs) (26–29). As the predominant cellular component within the TME, TAMs foster immunosuppression in CC by releasing chemokines and cytokines that compromise the local immune response (30). Chemokines produced by TAMs, such as CCL5, CCL22, and CCL20, recruit regulatory T cells (Tregs), while cytokines including IL-10 and TGF-β promote Treg differentiation, thereby driving tumorigenesis. Furthermore, TAMs suppress antitumor functions of T cells and NK cells (19, 31), and cooperate with MDSCs, tumor-associated dendritic cells, and neutrophils to intensify immunosuppression (32). These macrophages also reduce T cell activity through enzymes including nitric oxide synthase (NOS) and arginase (ARGI) (33). In addition, they display high expression of ligands for PD-1 and CTLA-4 (for example, PDL1 and B7-H1), thereby weakening the cytotoxic capacity of T cells, NKT cells, and NK cells (34–36), which further hampers the host’s ability to eliminate colorectal cancer cells. Elevated CSF-1R levels on TAMs can facilitate immune evasion via Treg recruitment, potentially accelerating colon adenocarcinoma progression (37).
Siglec9 has been recognized as a crucial immune checkpoint molecule expressed on TAMs, significantly contributing to the development of an immunosuppressive TME (38–40). Studies indicate that Siglec9+ TAMs drive immune evasion in CC by facilitating suppressive cell infiltration and compromising CD8+ T-cell function (41). Leukemia inhibitory factor (LIF), a pleiotropic cytokine, is robustly produced in tumor-associated macrophages, and its administration endows them with potent immunosuppressive traits. MSC-1 interferes with LIF signaling by engaging epitopes overlapping the gp130 receptor binding region on LIF, prompting TAMs to adopt antitumor, proinflammatory attributes in a syngeneic CC mouse model (42). Additionally, heightened metastasis-associated protein 1 (MTA1) expression in CC fosters an immunosuppressive milieu exhibiting abundant CD8+ T cells but lacking classical macrophages (43). Moreover, eight pivotal enzymes governing amino acid metabolism in colon TAMs-ACADM, ACADS, GPX4, GSR, HADH, HMGCL, HMGCS1, and IDH1-trigger pyroptotic cell death in these macrophages, thereby potentially bolstering immune evasion in CC (44).
In addition to secreting cytokines and chemokines, TAMs mediate immune suppression through diverse receptor-ligand interactions and intracellular signaling cascades that affect T cells, NK cells, dendritic cells, and B cells. For instance, Tim-4+ macrophages in tumor cavities suppress CD8+ T cell anti-tumor responses, inhibiting cytotoxic immunity (45). Furthermore, TAM-derived PGE2 and TGF-β, along with various chemokines, disrupt dendritic cell (DC) maturation. This disruption destabilizes the equilibrium between innate and adaptive immune responses, while concurrently impairing the effector functions of both T cells and NK cells (46). Indoleamine 2,3-dioxygenase (IDO)-expressing TAMs metabolize tryptophan to kynurenine, an aryl hydrocarbon receptor (AHR) ligand that drives Treg differentiation while suppressing Th17 development and T-cell proliferation (47–50). As a consequence, TAMs establish an immune-privileged niche that enables CC cells to escape immunosurveillance and thrive within the TME.
The vascular network sustaining tumor cells is pivotal in tumorigenesis and metastatic dissemination, making angiogenesis a critical process in tumor progression. Earlier investigations posited that this neovascularization was driven exclusively by tumor cells (51). However, emerging evidence shows that TAMs are major contributors to angiogenic regulation. Analyses of human CC tissues revealed that central angiogenic mediators-including S100A4, SPP1, and SPARC-are largely produced in stromal regions, particularly by TAMs, and strongly correlate with macrophage infiltration levels (52). Through immunohistochemical staining and image-based morphometry, Badawi et al. (53) examined microvascular density and macrophage presence in CC biopsy specimens and 15 benign adenomatous polyps, finding a marked link between elevated macrophage numbers and increased microvessel density in malignant tumors. Moreover, Luput et al. (54) demonstrated in an animal model that co-culturing C26 murine CC cells with TAMs significantly heightened total angiogenic protein levels in cell lysates. They also observed an inverse relationship between NF-κB activity and VEGF expression in C26 cells, indicating that TAMs boost angiogenesis by downregulating NF-κB. Additionally, CXCL1 is markedly upregulated in colorectal tumors and promotes neovascularization (55), while VEGF released by Siglec9+ TAMs further drives angiogenesis in CC (41).
Over 90% of cancer-related deaths are attributed to metastasis (56, 57). TAMs play essential roles in this process, contributing to epithelial-mesenchymal transition (EMT), local invasion, intravasation, systemic dissemination, extravasation, and final colonization that results in metastatic outgrowth (58–60). Within CC, TAMs facilitate metastatic progression by secreting various chemokines, proinflammatory molecules, and growth factors (61, 62). Jinushi and colleagues (63) reported that metastatic spread largely originates from tumor cells responding to cytokines released by TAMs, thus guiding migratory behavior. Furthermore, Xu et al. (64) observed that elevated Six1 protein levels in a murine colorectal cancer model foster TAM accumulation, which in turn amplifies metastatic potential. Moreover, TAMs increase EMR1/ADGRE1 expression in CC cells, potentially driving lymph node metastasis and disease progression through JAK2/STAT1,3 signaling (65). In colon adenocarcinoma (COAD), heightened CCL3 levels in both TAMs and tumor cells, acting via the CCL3-CCR5 axis, boost Akt-mediated migration and invasiveness (66). Additionally, CXCL1 fosters CC cell proliferation, enhances angiogenesis, and promotes M2 TAM recruitment through NF-κB/P300, ultimately advancing CC progression (55). The compound phenylhydrazine and pyrrolidinone sulfate 1 (PHPS1), identified as a potential phosphotyrosine inhibitor, can modulate M2-polarized TAMs and exosome secretion through the PI3K–AKT axis by downregulating SHP-2, thereby bolstering CC cell migration and invasion (67). Meanwhile, TAM-derived TGF-β1 instigates motility, invasiveness, and EMT in CC cells. Besides, the progression of EMT is facilitated by TAMs through activation of the CCL2/AKT/β-catenin signaling pathway (68). IL-6 secreted by TAMs interacts with the receptor complex involving glycoprotein 130 (gp130), thereby activating the JAK/STAT3 pathway in CC cells. This signaling cascade promotes EMT and chemoresistance (69, 70). Through heightened RBP-Jκ expression, CC cells release CXCL11, which elevates TGF-β1 levels in TAMs, further driving metastatic spread (71). Moreover, CCL14 fortifies M1 macrophage–mediated inhibition of CC cell proliferation and invasion, while negating the tumor-promoting influence of M2 macrophages (72).
Macrophages, often referred to as TAMs, can hinder the success of chemotherapy. Numerous in vivo and in vitro investigations have shown their tumor-protective influence under various chemotherapeutic conditions (73, 74). Following chemotherapy, TAMs stimulate tumor advancement through a range of mechanisms, including increased recruitment of immunosuppressive macrophages, pro-tumor polarization, a Th17 response that favors malignancy, and heightened anti-apoptotic signaling in cancer cells (74). In particular, M2 macrophages are instrumental in driving tumor progression by sustaining an immunosuppressive local milieu (62). Moreover, a fraction of tumor cells known as cancer stem cells (CSCs) holds self-renewal potential and can produce malignant offspring. Due to their notable resilience to chemotherapy, CSCs serve as critical contributors to tumor recurrence (75–77). TAMs influence both CSC self-renewal and therapy resistance by controlling an intricate network of cytokines, chemokines, growth factors, and extracellular matrix molecules. Research indicates that TAMs induce chemoresistance by providing survival cues or activating anti-apoptotic pathways within malignant cells (62). This process often relies on macrophage-derived soluble mediators, although it may also involve extracellular matrix remodeling or direct cellular contacts (61). Inflammatory factors significantly influence the efficacy of therapies (78–81). Chemoresistance in CC cells frequently relies on the activation of STAT3, often driven by macrophage-derived IL-6 or other factors produced by macrophages (82). Moreover, FJX1 expression is positively associated with TAM infiltration and immune-related genes, such as TGFB1 and IL-10, as well as with immune-suppressive pathways involving TGFB1 and WNT1. By contrast, FJX1 demonstrates an inverse correlation with CD8+ T-cell abundance. Elevated FJX1 also diminishes immunotherapy efficacy while exacerbating therapy resistance (83). In parallel, hypoxia prompts HIF2α-dependent upregulation of dihydropyrimidine dehydrogenase in TAMs, thereby driving 5-FU resistance in CC (84). Studies have demonstrated a correlation between EMT and the development of resistance to chemotherapy (85). TAMs have been found to facilitate this transition through multiple mechanisms, including IL-8 (86), CCL20 (87), and the toll-like receptor 4/interleukin-10 (TLR4/IL-10) signaling cascade (88).
Mounting evidence suggests that TAMs foster oncogenesis, and their increased numbers correlate with greater malignancy and worse patient outcomes across various cancers. Recent investigations underscore how TAMs remodel the TME. In CC, they primarily stimulate tumor progression by promoting angiogenesis, immune evasion, metastatic spread, and chemoresistance-partly through expanding cancer stem cell populations. During immunotherapeutic interventions, TAMs can also reshape the microenvironment to enable anti-tumor responses. Accordingly, emerging clinical data indicate that therapeutic strategies directed at TAMs-such as limiting their infiltration, altering M2/M1 polarization, or inhibiting key signaling pathways linked to TAM-associated antigen functions-may provide a promising route to enhance tumor immunotherapy (Figure 1).
Elevated levels of TAMs in tumors are strongly correlated with the progression of colorectal cancer (89). Current therapeutic strategies aim to restrict the infiltration of macrophage precursors and eliminate existing TAM populations within the TME. This process is facilitated by several chemokines, including CCL3, CCL4, CCL5, CCL22, and CXCL8, which are highly expressed in various tumors and promote TAM migration (90–92). Additionally, cytokines such as CSF-1 and EMAPII (93, 94), as well as growth factors like VEGF, endothelin-2, and PDGF, further enhance the recruitment of monocytes and macrophages (95–98). In hypoxic regions of tumors, HIF-1α plays a critical role in reprogramming TAMs toward a protumor phenotype by altering gene expression (99, 100). HIF-1α upregulates CXCR4 and induces CXCL12, which drives the recruitment of monocytes and macrophages into these oxygen-deprived areas (101, 102). It also increases VEGF-A expression in TAMs, creating a self-sustaining cycle that reinforces their survival and persistence (103). Meanwhile, hypoxia triggers MKP-1, which curbs ERK1/2 and p38 MAPK activity, restricting TAM mobility and causing them to remain under hypoxic stress (104). Additionally, hypoxia downregulates CCR2 and CCR5 on macrophages, reducing their chemotactic responsiveness (105). Targeting TAMs has emerged as a promising strategy to combat cancer progression. For instance, in an orthotopic CC xenograft mouse model, the CSF-1R inhibitor PLX3397 effectively reduced M2 macrophage populations, and its combination with anti-PD-1 therapy significantly suppressed tumor growth (106). Furthermore, FAP-modified, tumor-derived exosome-like vesicles (eNVs-FAP) demonstrated potent anticancer vaccine activity by inducing robust tumor-specific cytotoxic T lymphocyte (CTL) responses. This approach also reduced immunosuppressive components, including M2-TAMs, in the CC model (107).
In CC, TAMs typically adopt an M2-oriented phenotype, contrasting with M1 macrophages, which display a classically activated profile. These cells actively collaborate with tumor elements, primarily via the TME driving them toward an immunosuppressive M2 state that promotes vascularization, tumor expansion, invasion, and metastatic spread (36). Notably, M1 and M2 macrophages can switch between phenotypes under changing conditions. According to Georgoudaki et al. (108), immune checkpoint treatments in a mouse MC38 colorectal cancer model strengthened antitumor defenses by shifting TAMs toward an M1 phenotype and elevating tumor immunogenicity. Stimulating the STING pathway within TAMs presents a potential approach for managing CC. Recent data indicate that the prodrug STING agonist GB2 redirects TAM polarization by targeting TREM2 within malignant tissue, thereby enhancing CC immunotherapy (109). Moreover, inhibiting phosphoglycerate mutase 1 can diminish M2 polarization in CC cells, thereby restricting TAM-driven disease progression (110). Blue light exposure was further shown to impede M2 polarization in TAMs during CC (111). Curcumin mitigates malignant features of CC cells by controlling TAM M2 polarization and metastasis-associated colon cancer 1 (MACC1) levels (112). Through dual targeting of M2-oriented TAMs and TGF-β1-dependent positive feedback mechanisms, paclitaxel hinders CC advancement (113). Tasquinimod modulates myeloid cell recruitment in CC, inducing a shift from immunosuppressive M2-like TAMs with pro-angiogenic traits toward M1-like macrophages, consistent with its documented effects on vascularization, immune evasion, and metastatic activity (114). Within peptide-guided cancer therapy, recent investigations examined IFN-γ liposomes engineered with an M2-targeting peptide, selectively adhering to anti-inflammatory TAMs. Findings revealed improved uptake and antitumor activity of liposomal IFN-γ in C26 CC mouse models (115). Mesobuthus eupeus venom also shows promise against tumor expansion and metastasis by redirecting macrophages from M2 to M1 polarity (116). Furthermore, inhibiting CD39 with polyoxotungstate (POM-1) curbed CC tumor burden in mice and was associated with an upsurge of M1-like TAMs in tumor tissue (117).
In CT26 CC models, HDAC6 inhibitor AVS100 increased pro-inflammatory tumor-infiltrating macrophages and CD8 effector T cells with inflammatory gene profiles (118). Recently, AVS100’s favorable safety profile led to FDA IND approval, enabling clinical trials (118). A Phase I trial of Trebananib (Angiopoietin 1/2 inhibitor) with Pembrolizumab in CC showed reduced T cells and increased immunosuppressive macrophages post-resistance (119). Zhang et al. identified CD155 expressing on M2 type TAMs as therapeutic target in digestive cancers, with CD155-directed CAR-T cells showing promise in clinical evaluations, including CC (120). These findings underscore the translational potential of targeting TAMs to enhance immunotherapy (Table 1).
TAMs are central to colon cancer progression by fostering immune evasion, neovascularization, metastasis, and resistance to chemotherapy. The predominance of M2-oriented TAMs correlates with unfavorable clinical outcomes. Efforts to minimize their infiltration, alter their polarization, or disrupt their immunosuppressive traits may greatly enhance the efficacy of established colon cancer interventions. Approaches that focus on TAMs-used individually or in conjunction with existing immunotherapies-could form promising, patient-specific strategies. While obstacles remain in effectively neutralizing TAMs, continued investigations reveal new therapeutic prospects that hold potential for significantly influencing colon cancer management. Future directions for TAM research should include (1) leveraging advanced molecular and imaging techniques (e.g., single-cell RNA sequencing, multiplex immunofluorescence) to dissect TAM heterogeneity within the tumor microenvironment; (2) exploring combinatorial therapeutic strategies that integrate TAM modulation (via polarization reprogramming or depletion) with immune checkpoint inhibitors or targeted therapies; (3) identifying novel biomarkers that can accurately assess TAM activity and predict patient response to treatment; and (4) elucidating the role of the gut microbiome and other systemic factors in regulating TAM function. By pursuing these multidisciplinary approaches, researchers may uncover innovative interventions that enhance antitumor immunity and substantially improve clinical outcomes in CC.
JX: Writing – original draft. JW: Writing – original draft. HX: Writing – original draft. CH: Writing – original draft. CW: Writing – original draft. LZ: Writing – original draft. CQ: Writing – original draft, Writing – review & editing. DX: Writing – original draft, Writing – review & editing.
The author(s) declare that financial support was received for the research and/or publication of this article. This work was financially supported by the Natural Science Foundation of Chongqing (CSTB2024NSCQ-KJFZZDX0042), Science and Technology Research Project of Chongqing Education Commission (KJZD-M202400101), the Talents Project of Chongqing (CSTC2022YCJH-BGZXM0032) and the Fundamental Research Funds for the Central Universities (2022CDJYGRH-006, 2022CDJYGRH-018).
We thank the Affiliated Hospital of Southwest Medical University and Chongqing University Jiangjin Hospital for supporting this study.
The authors declare that the research was conducted in the absence of any commercial or financial relationships that could be construed as a potential conflict of interest.
The author(s) declare that no Generative AI was used in the creation of this manuscript.
All claims expressed in this article are solely those of the authors and do not necessarily represent those of their affiliated organizations, or those of the publisher, the editors and the reviewers. Any product that may be evaluated in this article, or claim that may be made by its manufacturer, is not guaranteed or endorsed by the publisher.
1. Li S, Tian J, Zhang H, Zhou S, Wang X, Zhang L, et al. Down-regulating IL-6/GP130 targets improved the anti-tumor effects of 5-fluorouracil in colon cancer. Apoptosis. (2018) 23:356–74. doi: 10.1007/s10495-018-1460-0
2. Bray F, Laversanne M, Sung H, Ferlay J, Siegel RL, Soerjomataram I, et al. Global cancer statistics 2022: GLOBOCAN estimates of incidence and mortality worldwide for 36 cancers in 185 countries. CA Cancer J Clin. (2024) 74:229–63. doi: 10.3322/caac.21834
3. Shen K, Wang H, Xue S, Wang L, Ren M, Gao Z, et al. Genome-wide screening and immune landscape suggest a potential-m6A-related lncRNA risk signature for predicting prognosis of melanoma. Ann Transl Med. (2022) 10:241. doi: 10.21037/atm-21-4402
4. Wang Y, Wang J, Liu J, Zhu H. Immune-related diagnostic markers for benign prostatic hyperplasia and their potential as drug targets. Front Immunol. (2024) 15:1516362. doi: 10.3389/fimmu.2024.1516362
5. Wang Y, Zhu H, Wang X. Prognosis and immune infiltration analysis of endoplasmic reticulum stress-related genes in bladder urothelial carcinoma. Front Genet. (2022) 13:965100. doi: 10.3389/fgene.2022.965100
6. Zhang J, Peng G, Chi H, Yang J, Xie X, Song G, et al. CD8 + T-cell marker genes reveal different immune subtypes of oral lichen planus by integrating single-cell RNA-seq and bulk RNA-sequencing. BMC Oral Health. (2023) 23:464. doi: 10.1186/s12903-023-03138-0
7. Zhang X, Zhuge J, Liu J, Xia Z, Wang H, Gao Q, et al. Prognostic signatures of sphingolipids: Understanding the immune landscape and predictive role in immunotherapy response and outcomes of hepatocellular carcinoma. Front Immunol. (2023) 14:1153423. doi: 10.3389/fimmu.2023.1153423
8. Ren Q, Zhang P, Lin H, Feng Y, Chi H, Zhang X, et al. A novel signature predicts prognosis and immunotherapy in lung adenocarcinoma based on cancer-associated fibroblasts. Front Immunol. (2023) 14:1201573. doi: 10.3389/fimmu.2023.1201573
9. Chi H, Gao X, Xia Z, Yu W, Yin X, Pan Y, et al. FAM family gene prediction model reveals heterogeneity, stemness and immune microenvironment of UCEC. Front Mol Biosci. (2023) 10:1200335. doi: 10.3389/fmolb.2023.1200335
10. Hui L, Chen Y. Tumor microenvironment: Sanctuary of the devil. Cancer Lett. (2015) 368:7–13. doi: 10.1016/j.canlet.2015.07.039
11. Xia Z, Chen S, He M, Li B, Deng Y, Yi L, et al. Editorial: Targeting metabolism to activate T cells and enhance the efficacy of checkpoint blockade immunotherapy in solid tumors. Front Immunol. (2023) 14:1247178. doi: 10.3389/fimmu.2023.1247178
12. Finke J, Ferrone S, Frey A, Mufson A, Ochoa A. Where have all the T cells gone? Mechanisms of immune evasion by tumors. Immunol Today. (1999) 20:158–60. doi: 10.1016/S0167-5699(98)01435-2
13. Drake CG, Jaffee E, Pardoll DM. Mechanisms of immune evasion by tumors. Adv Immunol. (2006) 90:51–81. doi: 10.1016/S0065-2776(06)90002-9
14. Gong X, Chi H, Strohmer DF, Teichmann AT, Xia Z, Wang Q. Exosomes: A potential tool for immunotherapy of ovarian cancer. Front Immunol. (2022) 13:1089410. doi: 10.3389/fimmu.2022.1089410
15. Tu E, Chia PZ, Chen W. TGFbeta in T cell biology and tumor immunity: Angel or devil? Cytokine Growth Factor Rev. (2014) 25:423–35. doi: 10.1016/j.cytogfr.2014.07.014
16. Ge Z, Ding S. The crosstalk between tumor-associated macrophages (TAMs) and tumor cells and the corresponding targeted therapy. Front Oncol. (2020) 10:590941. doi: 10.3389/fonc.2020.590941
17. Yang Q, Guo N, Zhou Y, Chen J, Wei Q, Han M. The role of tumor-associated macrophages (TAMs) in tumor progression and relevant advance in targeted therapy. Acta Pharm Sin B. (2020) 10:2156–70. doi: 10.1016/j.apsb.2020.04.004
18. Malekghasemi S, Majidi J, Baghbanzadeh A, Abdolalizadeh J, Baradaran B, Aghebati-Maleki L. Tumor-associated macrophages: protumoral macrophages in inflammatory tumor microenvironment. Adv Pharm Bull. (2020) 10:556–65. doi: 10.34172/apb.2020.066
19. Pan Y, Yu Y, Wang X, Zhang TJ. Tumor-associated macrophages in tumor immunity. Front Immunol. (2020) 11:583084. doi: 10.3389/fimmu.2020.583084
20. Weigert A, Strack E, Snodgrass RG, Brune B. mPGES-1 and ALOX5/-15 in tumor-associated macrophages. Cancer Metastasis Rev. (2018) 37:317–34. doi: 10.1007/s10555-018-9731-3
21. Martinez FO, Helming L, Milde R, Varin A, Melgert BN, Draijer C, et al. Genetic programs expressed in resting and IL-4 alternatively activated mouse and human macrophages: similarities and differences. Blood. (2013) 121:e57–69. doi: 10.1182/blood-2012-06-436212
22. Suzuki H, Hisamatsu T, Chiba S, Mori K, Kitazume MT, Shimamura K, et al. Glycolytic pathway affects differentiation of human monocytes to regulatory macrophages. Immunol Lett. (2016) 176:18–27. doi: 10.1016/j.imlet.2016.05.009
23. Sousa S, Brion R, Lintunen M, Kronqvist P, Sandholm J, Monkkonen J, et al. Human breast cancer cells educate macrophages toward the M2 activation status. Breast Cancer Res. (2015) 17:101. doi: 10.1186/s13058-015-0621-0
24. Lee S, Lee E, Ko E, Ham M, Lee HM, Kim ES, et al. Tumor-associated macrophages secrete CCL2 and induce the invasive phenotype of human breast epithelial cells through upregulation of ERO1-alpha and MMP-9. Cancer Lett. (2018) 437:25–34. doi: 10.1016/j.canlet.2018.08.025
25. Ghebremedhin A, Athavale D, Zhang Y, Yao X, Balch C, Song S. Tumor-associated macrophages as major immunosuppressive cells in the tumor microenvironment. Cancers (Basel). (2024) 16:3410. doi: 10.3390/cancers16193410
26. Iriondo O, Liu Y, Lee G, Elhodaky M, Jimenez C, Li L, et al. TAK1 mediates microenvironment-triggered autocrine signals and promotes triple-negative breast cancer lung metastasis. Nat Commun. (2018) 9:1994. doi: 10.1038/s41467-018-04460-w
27. Deng Y, Shi M, Yi L, Naveed Khan M, Xia Z, Li X. Eliminating a barrier: Aiming at VISTA, reversing MDSC-mediated T cell suppression in the tumor microenvironment. Heliyon. (2024) 10:e37060. doi: 10.1016/j.heliyon.2024.e37060
28. Shen K, Wang Q, Wang L, Yang Y, Ren M, Li Y, et al. Prediction of survival and immunotherapy response by the combined classifier of G protein-coupled receptors and tumor microenvironment in melanoma. Eur J Med Res. (2023) 28:352. doi: 10.1186/s40001-023-01346-6
29. Wang Y, Li C, He J, Zhao Q, Zhou Y, Sun H, et al. Multi-omics analysis and experimental validation of the value of monocyte-associated features in prostate cancer prognosis and immunotherapy. Front Immunol. (2024) 15:1426474. doi: 10.3389/fimmu.2024.1426474
30. Chen L, Wang S, Wang Y, Zhang W, Ma K, Hu C, et al. IL-6 influences the polarization of macrophages and the formation and growth of colorectal tumor. Oncotarget. (2018) 9:17443–54. doi: 10.18632/oncotarget.24734
31. Han Q, Shi H, Liu F. CD163(+) M2-type tumor-associated macrophage support the suppression of tumor-infiltrating T cells in osteosarcoma. Int Immunopharmacol. (2016) 34:101–6. doi: 10.1016/j.intimp.2016.01.023
32. Lohela M, Casbon AJ, Olow A, Bonham L, Branstetter D, Weng N, et al. Intravital imaging reveals distinct responses of depleting dynamic tumor-associated macrophage and dendritic cell subpopulations. Proc Natl Acad Sci U.S.A. (2014) 111:E5086–5095. doi: 10.1073/pnas.1419899111
33. Lu T, Ramakrishnan R, Altiok S, Youn JI, Cheng P, Celis E, et al. Tumor-infiltrating myeloid cells induce tumor cell resistance to cytotoxic T cells in mice. J Clin Invest. (2011) 121:4015–29. doi: 10.1172/JCI45862
34. Andrews LP, Yano H, Vignali DAA. Inhibitory receptors and ligands beyond PD-1, PD-L1 and CTLA-4: breakthroughs or backups. Nat Immunol. (2019) 20:1425–34. doi: 10.1038/s41590-019-0512-0
35. Zhou J, Zhang S, Guo CJII. Crosstalk between macrophages and natural killer cells in the tumor microenvironment. Int Immunopharmacol. (2021) 101:108374. doi: 10.1016/j.intimp.2021.108374
36. Noy R, Pollard JW. Tumor-associated macrophages: from mechanisms to therapy. Immunity. (2014) 41:49–61. doi: 10.1016/j.immuni.2014.06.010
37. Wang X, Zhang J, Hu B, Qian F. High expression of CSF-1R predicts poor prognosis and CSF-1R(high) tumor-associated macrophages inhibit anti-tumor immunity in colon adenocarcinoma. Front Oncol. (2022) 12:850767. doi: 10.3389/fonc.2022.850767
38. Laubli H, Pearce OM, Schwarz F, Siddiqui SS, Deng L, Stanczak MA, et al. Engagement of myelomonocytic Siglecs by tumor-associated ligands modulates the innate immune response to cancer. Proc Natl Acad Sci U.S.A. (2014) 111:14211–6. doi: 10.1073/pnas.1409580111
39. Wang Y, He M, Zhang C, Cao K, Zhang G, Yang M, et al. Siglec-9(+) tumor-associated macrophages delineate an immunosuppressive subset with therapeutic vulnerability in patients with high-grade serous ovarian cancer. J Immunother Cancer. (2023) 11:e007099. doi: 10.1136/jitc-2023-007099
40. Mei Y, Wang X, Zhang J, Liu D, He J, Huang C, et al. Siglec-9 acts as an immune-checkpoint molecule on macrophages in glioblastoma, restricting T-cell priming and immunotherapy response. Nat Cancer. (2023) 4:1273–91. doi: 10.1038/s43018-023-00598-9
41. Chang J, Feng Q, Mao Y, Zhang Z, Xu Y, Chen Y, et al. Siglec9 + tumor-associated macrophages predict prognosis and therapeutic vulnerability in patients with colon cancer. Int Immunopharmacol. (2024) 130:111771. doi: 10.1016/j.intimp.2024.111771
42. Hallett RM, Bonfill-Teixidor E, Iurlaro R, Arias A, Raman S, Bayliss P, et al. Therapeutic targeting of LIF overcomes macrophage-mediated immunosuppression of the local tumor microenvironment. Clin Cancer Res. (2023) 29:791–804. doi: 10.1158/1078-0432.CCR-21-1888
43. Zhou Y, Nan P, Li C, Mo H, Zhang Y, Wang H, et al. Upregulation of MTA1 in colon cancer drives A CD8(+) T cell-rich but classical macrophage-lacking immunosuppressive tumor microenvironment. Front Oncol. (2022) 12:825783. doi: 10.3389/fonc.2022.825783
44. Jiang M, Cui H, Liu Z, Zhou X, Zhang L, Cao L, et al. The role of amino acid metabolism of tumor associated macrophages in the development of colorectal cancer. Cells. (2022) 11:4106. doi: 10.3390/cells11244106
45. Chow A, SChad S, Green MD, Hellmann MD, Allaj V, Ceglia N, et al. Tim-4(+) cavity-resident macrophages impair anti-tumor CD8(+) T cell immunity. Cancer Cell. (2021) 39:973–988.e979. doi: 10.1016/j.ccell.2021.05.006
46. Wang J, Li D, Cang H, Guo B. Crosstalk between cancer and immune cells: Role of tumor-associated macrophages in the tumor microenvironment. Cancer Med. (2019) 8:4709–21. doi: 10.1002/cam4.v8.10
47. Opitz CA, Litzenburger UM, Sahm F, Ott M, Tritschler I, Trump S, et al. An endogenous tumour-promoting ligand of the human aryl hydrocarbon receptor. Nature. (2011) 478:197–203. doi: 10.1038/nature10491
48. Mezrich JD, Fechner JH, Zhang X, Johnson BP, Burlingham WJ, Bradfield CA. An interaction between kynurenine and the aryl hydrocarbon receptor can generate regulatory T cells. J Immunol. (2010) 185:3190–8. doi: 10.4049/jimmunol.0903670
49. Stephens GL, Wang Q, Swerdlow B, Bhat G, Kolbeck R, Fung M. Kynurenine 3-monooxygenase mediates inhibition of Th17 differentiation via catabolism of endogenous aryl hydrocarbon receptor ligands. Eur J Immunol. (2013) 43:1727–34. doi: 10.1002/eji.201242779
50. Wang Y, Wang D, Yang L, Zhang Y. Metabolic reprogramming in the immunosuppression of tumor-associated macrophages. Chin Med J (Engl). (2022) 135:2405–16. doi: 10.1097/CM9.0000000000002426
51. Bruno A, Bassani B, D’Urso DG, Pitaku I, Cassinotti E, Pelosi G, et al. Angiogenin and the MMP9-TIMP2 axis are up-regulated in proangiogenic, decidual NK-like cells from patients with colorectal cancer. FASEB J. (2018) 32:5365–77. doi: 10.1096/fj.201701103R
52. Kazakova E, Rakina M, Sudarskikh T, Iamshchikov P, Tarasova A, Tashireva L, et al. Angiogenesis regulators S100A4, SPARC and SPP1 correlate with macrophage infiltration and are prognostic biomarkers in colon and rectal cancers. Front Oncol. (2023) 13:1058337. doi: 10.3389/fonc.2023.1058337
53. Badawi MA, Abouelfadl DM, El-Sharkawy SL, El-Aal WE, Abbas NF. Tumor-associated macrophage (TAM) and angiogenesis in human colon carcinoma. Open Access Maced J Med Sci. (2015) 3:209–14. doi: 10.3889/oamjms.2015.044
54. Luput L, Licarete E, Sesarman A, Patras L, Alupei MC, Banciu M. Tumor-associated macrophages favor C26 murine colon carcinoma cell proliferation in an oxidative stress-dependent manner. Oncol Rep. (2017) 37:2472–80. doi: 10.3892/or.2017.5466
55. Zhuo C, Ruan Q, Zhao X, Shen Y, Lin R. CXCL1 promotes colon cancer progression through activation of NF-kappaB/P300 signaling pathway. Biol Direct. (2022) 17:34. doi: 10.1186/s13062-022-00348-4
56. Shen K, Song W, Wang H, Wang L, Yang Y, Hu Q, et al. Decoding the metastatic potential and optimal postoperative adjuvant therapy of melanoma based on metastasis score. Cell Death Discovery. (2023) 9:397. doi: 10.1038/s41420-023-01678-6
57. Wang JF, Wang JS, Liu Y, Ji B, Ding BC, Wang YX, et al. Knockdown of integrin beta1 inhibits proliferation and promotes apoptosis in bladder cancer cells. Biofactors. (2025) 51:e2150. doi: 10.1002/biof.2150
58. Correia AL, Bissell MJ. The tumor microenvironment is a dominant force in multidrug resistance. Drug Resist Update. (2012) 15:39–49. doi: 10.1016/j.drup.2012.01.006
59. Wang X, Zhao Y, Strohmer DF, Yang W, Xia Z, Yu C. The prognostic value of MicroRNAs associated with fatty acid metabolism in head and neck squamous cell carcinoma. Front Genet. (2022) 13:983672. doi: 10.3389/fgene.2022.983672
60. Zhang P, Pei S, Wu L, Xia Z, Wang Q, Huang X, et al. Integrating multiple machine learning methods to construct glutamine metabolism-related signatures in lung adenocarcinoma. Front Endocrinol (Lausanne). (2023) 14:1196372. doi: 10.3389/fendo.2023.1196372
61. Castells M, Thibault B, Delord JP, Couderc B. Implication of tumor microenvironment in chemoresistance: tumor-associated stromal cells protect tumor cells from cell death. Int J Mol Sci. (2012) 13:9545–71. doi: 10.3390/ijms13089545
62. Larionova I, Cherdyntseva N, Liu T, Patysheva M, Rakina M, Kzhyshkowska J. Interaction of tumor-associated macrophages and cancer chemotherapy. Oncoimmunology. (2019) 8:. doi: 10.1080/2162402X.2019.1596004
63. Jinushi M, Chiba S, Yoshiyama H, Masutomi K, Kinoshita I, Dosaka-Akita H, et al. Tumor-associated macrophages regulate tumorigenicity and anticancer drug responses of cancer stem/initiating cells. Proc Natl Acad Sci U.S.A. (2011) 108:12425–30. doi: 10.1073/pnas.1106645108
64. Xu H, Zhang Y, Peña MM, Pirisi L, Creek KE. Six1 promotes colorectal cancer growth and metastasis by stimulating angiogenesis and recruiting tumor-associated macrophages. Carcinogenesis. (2017) 38:281–92. doi: 10.1093/carcin/bgw121
65. Akter R, Park R, Lee SK, Han EJ, Park KS, Park J, et al. Upregulation of EMR1 (ADGRE1) by tumor-associated macrophages promotes colon cancer progression by activating the JAK2/STAT1,3 signaling pathway in tumor cells. Int J Mol Sci. (2024) 25:4388. doi: 10.3390/ijms25084388
66. Guan B, Li H, Yao J, Guo J, Yu F, Li G, et al. CCL3-CCR5 axis promotes cell migration and invasion of colon adenocarcinoma via Akt signaling pathway. Environ Toxicol. (2023) 38:172–84. doi: 10.1002/tox.23675
67. Yuan K, Zhao S, Ye B, Wang Q, Liu Y, Zhang P, et al. A novel T-cell exhaustion-related feature can accurately predict the prognosis of OC patients. Front Pharmacol. (2023) 14:1192777. doi: 10.3389/fphar.2023.1192777
68. Chen X, Yang M, Yin J, Li P, Zeng S, Zheng G, et al. Tumor-associated macrophages promote epithelial-mesenchymal transition and the cancer stem cell properties in triple-negative breast cancer through CCL2/AKT/beta-catenin signaling. Cell Commun Signal. (2022) 20:92. doi: 10.1186/s12964-022-00888-2
69. Zhong Q, Fang Y, Lai Q, Wang S, He C, Li A, et al. CPEB3 inhibits epithelial-mesenchymal transition by disrupting the crosstalk between colorectal cancer cells and tumor-associated macrophages via IL-6R/STAT3 signaling. J Exp Clin Cancer Res. (2020) 39:132. doi: 10.1186/s13046-020-01637-4
70. Wang L, Shen K, Gao Z, Ren M, Wei C, Yang Y, et al. Melanoma derived exosomes amplify radiotherapy induced abscopal effect via IRF7/I-IFN axis in macrophages. Adv Sci (Weinh). (2024) 11:e2304991. doi: 10.1002/advs.202304991
71. Liu M, Fu X, Jiang L, Ma J, Zheng X, Wang S, et al. Colon cancer cells secreted CXCL11 via RBP-Jkappa to facilitated tumour-associated macrophage-induced cancer metastasis. J Cell Mol Med. (2021) 25:10575–90. doi: 10.1111/jcmm.v25.22
72. Li N, Liang X, Li J, Zhang D, Li T, Guo Z. C-C motif chemokine ligand 14 inhibited colon cancer cell proliferation and invasion through suppressing M2 polarization of tumor-associated macrophages. Histol Histopathol. (2021) 36:743–52. doi: 10.14670/HH-18-348
73. Dijkgraaf EM, Heusinkveld M, Tummers B, Vogelpoel LT, Goedemans R, Jha V, et al. Chemotherapy alters monocyte differentiation to favor generation of cancer-supporting M2 macrophages in the tumor microenvironment. Cancer Res. (2013) 73:2480–92. doi: 10.1158/0008-5472.CAN-12-3542
74. Mantovani A, Allavena P. The interaction of anticancer therapies with tumor-associated macrophages. J Exp Med. (2015) 212:435–45. doi: 10.1084/jem.20150295
75. Clarke MF. Neurobiology: at the root of brain cancer. Nature. (2004) 432:281–2. doi: 10.1038/432281a
76. Dick JE. Stem cell concepts renew cancer research. Blood. (2008) 112:4793–807. doi: 10.1182/blood-2008-08-077941
77. Zhang X, Zhang P, Cong A, Feng Y, Chi H, Xia Z, et al. Unraveling molecular networks in thymic epithelial tumors: deciphering the unique signatures. Front Immunol. (2023) 14:1264325. doi: 10.3389/fimmu.2023.1264325
78. Zhai X, Zhang H, Xia Z, Liu M, Du G, Jiang Z, et al. Oxytocin alleviates liver fibrosis via hepatic macrophages. JHEP Rep. (2024) 6:101032. doi: 10.1016/j.jhepr.2024.101032
79. Xiao J, Lin H, Liu B, Xia Z, Zhang J, Jin J. Decreased S1P and SPHK2 are involved in pancreatic acinar cell injury. biomark Med. (2019) 13:627–37. doi: 10.2217/bmm-2018-0404
80. Xiao J, Huang K, Lin H, Xia Z, Zhang J, Li D, et al. Mogroside II(E) inhibits digestive enzymes via suppression of interleukin 9/interleukin 9 receptor signalling in acute pancreatitis. Front Pharmacol. (2020) 11:859. doi: 10.3389/fphar.2020.00859
81. Zhang H, Xia T, Xia Z, Zhou H, Li Z, Wang W, et al. KIF18A inactivates hepatic stellate cells and alleviates liver fibrosis through the TTC3/Akt/mTOR pathway. Cell Mol Life Sci. (2024) 81:96. doi: 10.1007/s00018-024-05114-5
82. Ruffell B, Coussens LM. Macrophages and therapeutic resistance in cancer. Cancer Cell. (2015) 27:462–72. doi: 10.1016/j.ccell.2015.02.015
83. Huang M, Guo T, Meng Y, Zhou R, Xiong M, Ding J, et al. Comprehensive analysis of the prognosis and immune effect of the oncogenic protein Four Jointed Box 1. Front Oncol. (2023) 13:170482. doi: 10.3389/fonc.2023.1170482
84. Malier M, Gharzeddine K, Laverriere MH, Marsili S, Thomas F, Decaens T, et al. Hypoxia drives dihydropyrimidine dehydrogenase expression in macrophages and confers chemoresistance in colorectal cancer. Cancer Res. (2021) 81:5963–76. doi: 10.1158/0008-5472.CAN-21-1572
85. Kuwada K, Kagawa S, Yoshida R, Sakamoto S, Ito A, Watanabe M, et al. The epithelial-to-mesenchymal transition induced by tumor-associated macrophages confers chemoresistance in peritoneally disseminated pancreatic cancer. J Exp Clin Cancer Res. (2018) 37:307. doi: 10.1186/s13046-018-0981-2
86. Chen SJ, Lian GD, Li JJ, Zhang QB, Zeng LJ, Yang KG, et al. Tumor-driven like macrophages induced by conditioned media from pancreatic ductal adenocarcinoma promote tumor metastasis via secreting IL-8. Cancer Med. (2018) 7:5679–90. doi: 10.1002/cam4.2018.7.issue-11
87. Liu B, Jia Y, Ma J, Wu S, Jiang H, Cao Y, et al. Tumor-associated macrophage-derived CCL20 enhances the growth and metastasis of pancreatic cancer. Acta Biochim Biophys Sin (Shanghai). (2016) 48:1067–74. doi: 10.1093/abbs/gmw101
88. Liu CY, Xu JY, Shi XY, Huang W, Ruan TY, Xie P, et al. M2-polarized tumor-associated macrophages promoted epithelial-mesenchymal transition in pancreatic cancer cells, partially through TLR4/IL-10 signaling pathway. Lab Invest. (2013) 93:844–54. doi: 10.1038/labinvest.2013.69
89. Kang JC, Chen JS, Lee CH, Chang JJ, Shieh YS. Intratumoral macrophage counts correlate with tumor progression in colorectal cancer. J Surg Oncol. (2010) 102:242–8. doi: 10.1002/jso.v102:3
90. Hao NB, Lu MH, Fan YH, Cao YL, Zhang ZR, Yang SM. Macrophages in tumor microenvironments and the progression of tumors. Clin Dev Immunol. (2012) 2012:948098. doi: 10.1155/2012/948098
91. Wu Y, Li YY, Matsushima K, Baba T, Mukaida N. CCL3-CCR5 axis regulates intratumoral accumulation of leukocytes and fibroblasts and promotes angiogenesis in murine lung metastasis process. J Immunol. (2008) 181:6384–93. doi: 10.4049/jimmunol.181.9.6384
92. Milliken D, Scotton C, Raju S, Balkwill F, Wilson J. Analysis of chemokines and chemokine receptor expression in ovarian cancer ascites. Clin Cancer Res. (2002) 8:1108–14.
93. Lin EY, Nguyen AV, Russell RG, Pollard JW. Colony-stimulating factor 1 promotes progression of mammary tumors to Malignancy. J Exp Med. (2001) 193:727–40. doi: 10.1084/jem.193.6.727
94. Kao J, Houck K, Fan Y, Haehnel I, Libutti SK, Kayton ML, et al. Characterization of a novel tumor-derived cytokine. Endothelial-monocyte activating polypeptide II. J Biol Chem. (1994) 269:25106–19. doi: 10.1016/S0021-9258(17)31505-3
95. Balkwill F. Cancer and the chemokine network. Nat Rev Cancer. (2004) 4:540–50. doi: 10.1038/nrc1388
96. Murdoch C, Giannoudis A, Lewis CE. Mechanisms regulating the recruitment of macrophages into hypoxic areas of tumors and other ischemic tissues. Blood. (2004) 104:2224–34. doi: 10.1182/blood-2004-03-1109
97. Solinas G, Germano G, Mantovani A, Allavena P. Tumor-associated macrophages (TAM) as major players of the cancer-related inflammation. J Leukoc Biol. (2009) 86:1065–73. doi: 10.1189/jlb.0609385
98. Zhao Y, Wei K, Chi H, Xia Z, Li X. IL-7: A promising adjuvant ensuring effective T cell responses and memory in combination with cancer vaccines? Front Immunol. (2022) 13:1022808. doi: 10.3389/fimmu.2022.1022808
99. Burke B, Tang N, Corke KP, Tazzyman D, Ameri K, Wells M, et al. Expression of HIF-1alpha by human macrophages: implications for the use of macrophages in hypoxia-regulated cancer gene therapy. J Pathol. (2002) 196:204–12. doi: 10.1002/path.v196:2
100. Talks KL, Turley H, Gatter KC, Maxwell PH, Pugh CW, Ratcliffe PJ, et al. The expression and distribution of the hypoxia-inducible factors HIF-1alpha and HIF-2alpha in normal human tissues, cancers, and tumor-associated macrophages. Am J Pathol. (2000) 157:411–21. doi: 10.1016/S0002-9440(10)64554-3
101. Schioppa T, Uranchimeg B, Saccani A, Biswas SK, Doni A, Rapisarda A, et al. Regulation of the chemokine receptor CXCR4 by hypoxia. J Exp Med. (2003) 198:1391–402. doi: 10.1084/jem.20030267
102. Ceradini DJ, Kulkarni AR, Callaghan MJ, Tepper OM, Bastidas N, Kleinman ME, et al. Progenitor cell trafficking is regulated by hypoxic gradients through HIF-1 induction of SDF-1. Nat Med. (2004) 10:858–64. doi: 10.1038/nm1075
103. Eubank TD, Roda JM, Liu H, O’Neil T, Marsh CB. Opposing roles for HIF-1alpha and HIF-2alpha in the regulation of angiogenesis by mononuclear phagocytes. Blood. (2011) 117:323–32. doi: 10.1182/blood-2010-01-261792
104. Grimshaw MJ, Balkwill FR. Inhibition of monocyte and macrophage chemotaxis by hypoxia and inflammation–a potential mechanism. Eur J Immunol. (2001) 31:480–9. doi: 10.1002/1521-4141(200102)31:2<480::AID-IMMU480>3.0.CO;2-L
105. Sica A, Saccani A, Bottazzi B, Bernasconi S, Allavena P, Gaetano B, et al. Defective expression of the monocyte chemotactic protein-1 receptor CCR2 in macrophages associated with human ovarian carcinoma. J Immunol. (2000) 164:733–8. doi: 10.4049/jimmunol.164.2.733
106. Shimizu D, Yuge R, Kitadai Y, Ariyoshi M, Miyamoto R, Hiyama Y, et al. Pexidartinib and immune checkpoint inhibitors combine to activate tumor immunity in a murine colorectal cancer model by depleting M2 macrophages differentiated by cancer-associated fibroblasts. Int J Mol Sci. (2024) 25:7001. doi: 10.3390/ijms25137001
107. Hu S, Ma J, Su C, Chen Y, Shu Y, Qi Z, et al. Engineered exosome-like nanovesicles suppress tumor growth by reprogramming tumor microenvironment and promoting tumor ferroptosis. Acta Biomater. (2021) 135:567–81. doi: 10.1016/j.actbio.2021.09.003
108. Georgoudaki AM, Prokopec KE, Boura VF, Hellqvist E, Sohn S, Ostling J, et al. Reprogramming tumor-associated macrophages by antibody targeting inhibits cancer progression and metastasis. Cell Rep. (2016) 15:2000–11. doi: 10.1016/j.celrep.2016.04.084
109. Deng A, Fan R, Hai Y, Zhuang J, Zhang B, Lu X, et al. A STING agonist prodrug reprograms tumor-associated macrophage to boost colorectal cancer immunotherapy. Theranostics. (2025) 15:277–99. doi: 10.7150/thno.101001
110. Wang C, Zhang M, Li S, Gong M, Luo MY, Zhang MC, et al. A phosphoglycerate mutase 1 allosteric inhibitor restrains TAM-mediated colon cancer progression. Acta Pharm Sin B. (2024) 14:4819–31. doi: 10.1016/j.apsb.2024.09.007
111. Yoshimoto T, Nishi M, Okikawa S, Yoshikawa K, Tokunaga T, Nakao T, et al. Blue light irradiation inhibits the M2 polarization of the cancer-associated macrophages in colon cancer. BMC Cancer. (2024) 24:664. doi: 10.1186/s12885-024-12440-1
112. Ge S, Sun X, Sang L, Zhang M, Yan X, Ju Q, et al. Curcumin inhibits Malignant behavior of colorectal cancer cells by regulating M2 polarization of tumor-associated macrophages and metastasis associated in colon cancer 1 (MACC1) expression. Chem Biol Drug Des. (2023) 102:1202–12. doi: 10.1111/cbdd.v102.5
113. Chiou YS, Lan YM, Lee PS, Lin Q, Nagabhushanam K, Ho CT, et al. Piceatannol prevents colon cancer progression via dual-targeting to M2-polarized tumor-associated macrophages and the TGF-beta1 positive feedback signaling pathway. Mol Nutr Food Res. (2022) 66:e2200248. doi: 10.1002/mnfr.202200248
114. Olsson A, Nakhle J, Sundstedt A, Plas P, Bauchet AL, Pierron V, et al. Tasquinimod triggers an early change in the polarization of tumor associated macrophages in the tumor microenvironment. J Immunother Cancer. (2015) 3:53. doi: 10.1186/s40425-015-0098-5
115. Kateh Shamshiri M, Vakili-Ghartavol R, Aiyelabegan HT, Asvar Z, Zare Marzouni H, Matbou Riahi M, et al. M2 macrophage-targeting peptide-modified liposomes enhance the uptake and antitumor efficacy of liposomal IFN-gamma in mice with C26 colon carcinoma. Cytokine. (2025) 187:156860. doi: 10.1016/j.cyto.2025.156860
116. Sadeghi M, Amari A, Asadirad A, Nemati M, Khodadadi A. F1 fraction isolated from Mesobuthus eupeus scorpion venom induces macrophage polarization toward M1 phenotype and exerts anti-tumoral effects on the CT26 tumor cell line. Int Immunopharmacol. (2024) 132:111960. doi: 10.1016/j.intimp.2024.111960
117. Park HJ, Seo EH, Piao L, Park ST, Lee MK, Koh SE, et al. The preventive effect of the phenotype of tumour-associated macrophages, regulated by CD39, on colon cancer in mice. Int J Mol Sci. (2021) 22:7478. doi: 10.3390/ijms22147478
118. Kovalovsky D, NooNepalle S, Suresh M, Kumar D, Berrigan M, Gajendran N, et al. The HDAC6 inhibitor AVS100 (SS208) induces a pro-inflammatory tumor microenvironment and potentiates immunotherapy. Sci Adv. (2024) 10:eadp3687. doi: 10.1126/sciadv.adp3687
119. Huffman BM, Rahma OE, Tyan K, Li YY, Giobbie-Hurder A, Schlechter BL, et al. A phase I trial of trebananib, an angiopoietin 1 and 2 neutralizing peptibody, combined with pembrolizumab in patients with advanced ovarian and colorectal cancer. Cancer Immunol Res. (2025) 13:9–22. doi: 10.1158/2326-6066.CIR-23-1027
Keywords: colon cancer, tumor-associated macrophages, M1 macrophages, immune suppression, metastasis, chemokines
Citation: Xiang J, Wang J, Xiao H, Huang C, Wu C, Zhang L, Qian C and Xiang D (2025) Targeting tumor-associated macrophages in colon cancer: mechanisms and therapeutic strategies. Front. Immunol. 16:1573917. doi: 10.3389/fimmu.2025.1573917
Received: 10 February 2025; Accepted: 10 March 2025;
Published: 21 March 2025.
Edited by:
Minghua Ren, First Affiliated Hospital of Harbin Medical University, ChinaReviewed by:
Kangjie Shen, Fudan University, ChinaCopyright © 2025 Xiang, Wang, Xiao, Huang, Wu, Zhang, Qian and Xiang. This is an open-access article distributed under the terms of the Creative Commons Attribution License (CC BY). The use, distribution or reproduction in other forums is permitted, provided the original author(s) and the copyright owner(s) are credited and that the original publication in this journal is cited, in accordance with accepted academic practice. No use, distribution or reproduction is permitted which does not comply with these terms.
*Correspondence: Debing Xiang, eGRiODZAY3F1LmVkdS5jbg==; Chenyuan Qian, NDkxMDY3NDlAcXEuY29t
Disclaimer: All claims expressed in this article are solely those of the authors and do not necessarily represent those of their affiliated organizations, or those of the publisher, the editors and the reviewers. Any product that may be evaluated in this article or claim that may be made by its manufacturer is not guaranteed or endorsed by the publisher.
Research integrity at Frontiers
Learn more about the work of our research integrity team to safeguard the quality of each article we publish.