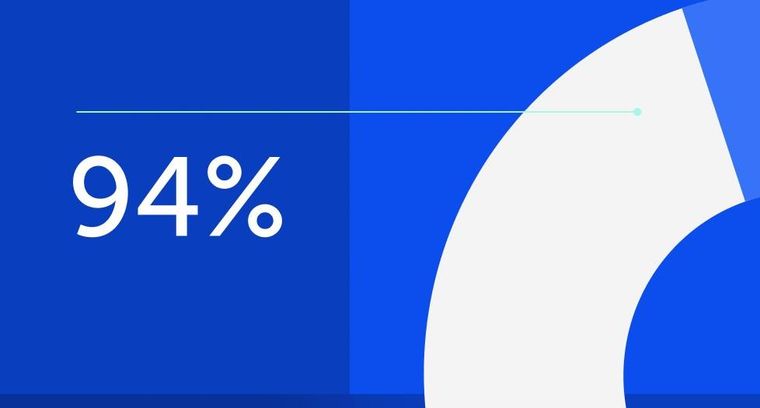
94% of researchers rate our articles as excellent or good
Learn more about the work of our research integrity team to safeguard the quality of each article we publish.
Find out more
MINI REVIEW article
Front. Immunol., 02 April 2025
Sec. Multiple Sclerosis and Neuroimmunology
Volume 16 - 2025 | https://doi.org/10.3389/fimmu.2025.1572140
This article is part of the Research TopicCentral nervous system autoimmunity and autoinflammation: insights from inborn errors of immunityView all 4 articles
Multiple sclerosis (MS) is widely acknowledged as a chronic inflammatory autoimmune disorder characterized by central nervous system (CNS) demyelination and neurodegeneration. The hyperactivation of immune and inflammatory responses is recognized as a pivotal factor contributing to the pathogenesis and progression of MS. Among various immune and inflammatory reactions, researchers have increasingly focused on the inflammasome, a complex of proteins. The initiation and activation of the inflammasome are intricately involved in the onset of MS. Notably, the NLRP3 inflammasome, the most extensively studied member of the inflammasome complex, is closely linked with MS. This review will delve into the roles of the NLRP3 inflammasome in the pathogenesis and progression of MS. Additionally, therapeutic strategies targeting the NLRP3 inflammasome for the treatment of MS, including natural compounds, autophagy regulators, and other small molecular compounds, will be detailed in this review.
Multiple Sclerosis (MS) is a chronic, progressive, and frequently disabling neurological disorder that affects the central nervous system (CNS), which comprises the brain and spinal cord (1–3). This condition is characterized by the destruction of myelin, the protective sheath surrounding nerve fibers, leading to disrupted communication between the brain and the rest of the body (4, 5). Demyelination in MS results in a diverse array of symptoms, including motor, sensory, and cognitive impairments. The etiology of MS is multifaceted, involving a complex interaction between genetic predisposition and environmental factors (6, 7). Histopathologically, MS lesions or plaques are characterized by focal demyelination, gliosis, and axonal loss (8, 9). These lesions can occur throughout the CNS but are commonly found in the periventricular regions, optic nerves, brainstem, and spinal cord (10, 11). The pathogenesis of MS involves an autoimmune and inflammatory response targeting CNS components (12–14). Therefore, modulating the overactivation of immune and inflammatory responses represents a promising therapeutic strategy against MS (15–19). In this paper, we will focus on the role of the NLRP3 inflammasome, a crucial component of the innate immune system, in the pathogenesis and progression of MS. Additionally, we will discuss the pharmacological application of agents that inhibit the NLRP3 inflammasome.
To gather relevant studies, we conducted a comprehensive literature search on PubMed (https://pubmed.ncbi.nlm.nih.gov/) and Web of Science (https://www.webofscience.com/), concentrating on publications from the past two decades. Our search terms were “NLRP3 inflammasome, multiple sclerosis, experimental autoimmune encephalomyelitis, natural compound, autophagy, small molecular compound, inflammation, mechanism, therapy”. We prioritized studies published in the last ten years. The process involved initially screening titles and abstracts, then obtaining full-text articles for in-depth analysis.
The inflammasome, a critical inducer of the innate immune response, plays a pivotal role in recognizing and targeting numerous invasive or internal pathogens (20–22). It is widely recognized that inflammasomes are primarily produced in immune and inflammatory cells, including macrophages, T lymphocytes, and natural killer (NK) cells, thereby contributing to the initiation of anti-pathogen immune inflammatory responses (23–25). To date, several types of inflammasomes have been identified, notably including NLRP1, NLRP2, NLRP3, double-stranded DNA sensors absent in melanoma 2 (AIM2), and NLRC4 inflammasomes (26–28). The NLRP3 inflammasome, compared to other inflammasomes, is the most well-studied and has been linked to various diseases, including MS (29–31). It significantly contributes to MS development through pro-inflammatory cytokine production and interactions with other immune responses. This section details the NLRP3 inflammasome’s components, activation mechanisms, and its importance in MS.
The NLRP3 inflammasome consists of three components: the NLRP3 protein, the adapter protein apoptosis-associated speck-like protein (ASC), and procaspase-1 (32, 33). In the absence of activating factors such as pathogen-associated molecular patterns (PAMPs) and danger-associated molecular patterns (DAMPs), the leucine-rich repeats (LRRs) and NACHT domain in the NLRP3 protein interact tightly, preventing the interaction between the NLRP3 protein and ASC (34–36). Upon exposure to immune stimuli, the NLRP3 protein is activated, facilitating its interaction with ASC and procaspase-1 via the pyrin domain (PYD) and caspase recruitment domain (CARD), respectively, leading to the assembly of the NLRP3 inflammasome (37–39).
As previously described by our group and other researchers, the activation of the NLRP3 inflammasome involves two distinct steps (29, 40–42). In the initial step, priming signals are triggered by specific PAMPs or DAMPs acting on Toll-like receptor 4 (TLR4), resulting in the activation of the NF-κB-mediated pathway. This activation enhances the transcription of NLRP3 inflammasome-related components, including the NLRP3 protein, pro-interleukin-1β (proIL-1β), and proIL-18. In the subsequent second step, further stimulation of immune and inflammatory cells leads to the oligomerization of the NLRP3 protein, followed by the assembly of the NLRP3 protein, ASC, and procaspase-1 into the NLRP3 inflammasome complex. The successful formation of the NLRP3 inflammasome catalyzes the conversion of procaspase-1 to caspase-1, which subsequently processes proIL-1β and proIL-18 into their mature forms, IL-1β and IL-18. These mature cytokines are secreted, initiating a cascade of immune or inflammatory reactions.
Several factors have been identified as activators of the NLRP3 inflammasome. Lipopolysaccharide (LPS) is widely recognized as a classic ligand for the activation of TLR4, initiating the first step of NLRP3 inflammasome activation (43, 44). Additionally, various factors have been shown to effectively induce the second step of NLRP3 inflammasome activation, including adenosine triphosphate (ATP, which triggers intracellular K+ efflux), PAMPs, DAMPs, silica, β-amyloid, autophagy deficiency, and factors leading to mitochondrial Ca2+ overload (45–49).
The NLRP3 inflammasome has been implicated in the pathogenesis and progression of various diseases, including cardiovascular conditions (such as atherosclerosis and myocardial infarction) (50–52), digestive disorders (such as inflammatory bowel disease and pancreatic disease) (53–55), malignancies (56–58), and metabolic diseases (such as diabetes and obesity) (59–61). In the context of multiple sclerosis (MS), the role of the NLRP3 inflammasome has been extensively studied. Elevated IL-1β levels have been identified in the serum of patients with primary progressive MS through RNA sequencing. Notably, primary progressive MS patients with high IL-1β gene expression levels in peripheral blood mononuclear cells exhibited significantly faster disease progression compared to those with low IL-1β levels (62, 63). Further studies demonstrated that the NLRP3 inflammasome was overactivated in monocytes from patients with primary progressive MS (62, 63). These findings suggested that IL-1β and the NLRP3 inflammasome could serve as prognostic biomarkers and potential therapeutic targets in primary progressive MS.
Variants in the NLRP3 inflammasome have also been associated with the susceptibility and severity of MS. Single nucleotide polymorphisms and expression levels of NLRP3 are closely related to susceptibility to relapsing-remitting MS (64–66). A pilot study reported overexpression of NLRP3 inflammasome components, including NF-κB, NLRP3, and caspase-1, in the serum during the early stages of MS (67). Moreover, bioinformatics analyses have suggested that the NLRP3 inflammasome-related NLR signaling pathway may play a critical role in COVID-19-related MS (68). These findings underscore a close relationship between the NLRP3 inflammasome and MS.
Despite the growing body of research on this topic, the mechanisms and influencing factors of the NLRP3 inflammasome in the pathogenesis and progression of MS are not fully understood. A recent study using an experimental autoimmune encephalomyelitis (EAE) mouse model, a widely accepted model for MS, demonstrated that NLRP3 exacerbated EAE severity through ROS-dependent neutrophil extracellular trap (NET) formation in the brain (69). NLRP3 facilitated NET formation in a ROS-dependent and PAD4-independent manner in brain-infiltrated neutrophils. Additionally, the NLRP3 inflammasome in microglial cells has been shown to contribute to demyelination and neurodegeneration induced by the neuronal accumulation of peroxidated lipids (70). The NLRP3 inflammasome has also been implicated in TRPM2 and IL-11-mediated neuroinflammation and cognitive deficits in a cuprizone-induced MS model (71, 72).
As discussed above, the NLRP3 inflammasome is closely related to the pathogenesis and progression of multiple sclerosis (MS), suggesting that it is a promising target for therapeutic intervention. Numerous studies have reported various agents that appear effective in attenuating MS by inhibiting the NLRP3 inflammasome. Several popular and well-studied agents will be introduced and discussed in detail in the following sections (illustrated in Figure 1 and listed in Table 1).
Figure 1. Schematic illustration of biological process of NLRP3 inflammasome and pharmacological application of NLRP3 inflammasome inhibition in multiple sclerosis treatment. Under the exposure of PAMPs/DAMPs including Epstein-Barr virus, reactive oxygen species, peroxidated lipids, and lipopolysaccharide, the NLRP3 protein as well as proIL-1β and proIL-18 production. The assembly and formation of the NLRP3 inflammasome through the combination of the NLRP3 protein, ASC and procaspase-1 were assembly, which triggered the formation and activation of the NLRP3 inflammasome. The NLRP3 inflammasome catalyzes the maturation and secretion of IL-1β and IL-18. The production and release of IL-1β and IL-18 contributed to the pathogenesis and progression of multiple sclerosis. The process of NLRP3 inflammasome activation can be blocked by several kinds of NLRP3 inflammasome inhibitors in multiple sclerosis, including natural compounds (sinomenine, mushroom natural products, emodi, and 1,2,4-trimethoxybenzene), autophagy regulators (liraglutide, AZD8055, PNU282987, and HU308), and other small molecular compounds (MCC950, lonidamine, and nebivolol), which serve as potential therapeutic strategies against multiple sclerosis.
Table 1. Potential pharmacological application of NLRP3 inflammasome inhibition in multiple sclerosis treatment.
Natural compounds, also known as natural extracts, are substances isolated or derived from organisms such as plants, animals, microorganisms, and humans (73–75). Some of these compounds have demonstrated potential in alleviating MS symptoms by targeting the NLRP3 inflammasome. For example, Kiasalari et al. (76) revealed that sinomenine, a natural alkaloid with various therapeutic benefits including anti-inflammatory and immunosuppressive activities, decreased EAE severity. This effect was attributed to its reduction of microglial and astrocytic activation, demyelination, and axonal damage, along with its suppression of neuroinflammation. Additionally, various mushroom-derived natural products have been shown to exert neuroprotective effects in MS through novel high-throughput screening methods by suppressing NLRP3 inflammasome activation and oligomerization (77). Cui et al. (78) demonstrated that emodin, a compound extracted from herbs such as rhubarb, could improve symptoms of experimental autoimmune encephalomyelitis, potentially by regulating the silent information regulator of transcription 1 (SIRT1)/peroxisome proliferator-activated receptor gamma coactivator 1-alpha (PGC-1α)/NLRP3 signaling pathway and inhibiting microglial inflammation. Furthermore, 1,2,4-trimethoxybenzene, an active ingredient in essential oils, significantly ameliorated EAE progression and demyelination by inhibiting ASC and the protein-protein interaction between NLRP3 and ASC (79). However, despite the promising potential of these natural compounds in preclinical studies, few have been successfully applied in clinical practice for the treatment of MS. Therefore, further research is needed to explore and validate the therapeutic efficacy of natural compounds targeting the NLRP3 inflammasome in MS.
Autophagy is a fundamental catabolic cellular process responsible for degrading protein aggregates and damaged organelles into metabolic components through lysosomal recycling, thereby maintaining cellular homeostasis and vitality (75, 80–82). This process is ubiquitously present in virtually all cell types and is evolutionarily conserved from yeast to mammals (83, 84). In recent years, extensive research has explored the role of autophagy in inflammation- and immune-related diseases through its regulatory effects on inflammatory and immune responses (85–87). Several autophagy regulators have shown potential in alleviating MS by modulating the NLRP3 inflammasome. Liraglutide, a glucagon-like peptide-1 receptor (GLP-1R) agonist, has been shown to ameliorate central nervous system demyelination and inflammation in EAE models by regulating the pAMPK and AMPK/SIRT1 signaling pathways (88, 89). Additionally, He et al. (90) reported that AZD8055, an autophagy activator, could reduce EAE severity through anti-inflammatory and anti-pyroptotic effects via the mammalian target of rapamycin (mTOR)/ROS/NLRP3 pathway. Our previous studies have further elucidated the role of autophagy in MS. We demonstrated that activating the α7 nicotinic acetylcholine receptor (α7nAChR) with PNU282987 could alleviate neuroinflammation in EAE models by enhancing monocyte/microglia autophagy, thereby inhibiting the NLRP3 inflammasome (91). Another study conducted by our group revealed that activation of cannabinoid receptor 2 (CB2R) with HU308 protected against neuroinflammation through autophagy-mediated suppression of the NLRP3 inflammasome. This effect was mediated via the autophagy-related gene 5 (ATG5)-dependent signaling pathway (92).
In addition to natural compounds and autophagy regulators, several other small molecular compounds have been demonstrated to effectively alleviate MS by inhibiting the NLRP3 inflammasome. For instance, MCC950, a potent and selective small-molecule inhibitor of NLRP3, was first reported in 2015 to alleviate MS, as well as type 2 diabetes, Alzheimer’s disease, atherosclerosis, and cryopyrin-associated periodic syndrome (CAPS), by blocking both canonical and noncanonical NLRP3 activation at nanomolar concentrations (93). Subsequent studies have shown that MCC950 can reduce neurological impairment in EAE models by inhibiting the NLRP3 inflammasome, thereby suppressing glial cell activation and preventing the polarization of microglia to the pro-inflammatory M1 phenotype (94–96). Additionally, lonidamine (LND), a small-molecule inhibitor of glycolysis used as an antineoplastic drug, has been evidenced to have anti-inflammatory effects. LND was reported to alleviate EAE by directly binding to the NLRP3 inflammasome component ASC and inhibiting its oligomerization (97). Another small molecular compound shown to alleviate MS through NLRP3 inflammasome inhibition is nebivolol, which has been found to modulate M1/M2 microglial polarization (98). These findings highlight the therapeutic potential of small molecular compounds in targeting the NLRP3 inflammasome for the treatment of MS. Further research is warranted to explore the clinical applications and efficacy of these agents in MS patients.
In conclusion, recent studies have highlighted the critical roles of the NLRP3 inflammasome in the pathogenesis and progression of MS (Figure 1). We are fortunate to have a variety of inhibitors targeting NLRP3 inflammasome activation that have demonstrated efficacy in alleviating MS symptoms. These include natural compounds, autophagy regulators, and other small molecular compounds. Despite progress, significant hurdles remain in applying NLRP3 inflammasome-targeted therapies clinically, especially concerning long-term safety and side effects. Few agents have been successfully used in MS treatment due to insufficient evidence for long-term safety and potential risks of side effects associated with prolonged use of such agents. Therefore, further research is essential to develop effective MS therapies by targeting the NLRP3 inflammasome.
W-GZ: Writing – original draft. X-RZ: Writing – original draft. YY: Writing – original draft. W-JS: Writing – original draft. B-ZS: Writing – review & editing.
The author(s) declare that financial support was received for the research and/or publication of this article. This work was supported by grants from Beijing Natural Science Foundation Program (No. 7244297 and 7234412) and National Natural Science Foundation of China (No. 82204483).
The authors declare that the research was conducted in the absence of any commercial or financial relationships that could be construed as a potential conflict of interest.
The author(s) declare that no Generative AI was used in the creation of this manuscript.
All claims expressed in this article are solely those of the authors and do not necessarily represent those of their affiliated organizations, or those of the publisher, the editors and the reviewers. Any product that may be evaluated in this article, or claim that may be made by its manufacturer, is not guaranteed or endorsed by the publisher.
1. Hauser SL, Cree BAC. Treatment of multiple sclerosis: A review. Am J Med. (2020) 133:1380–1390 e2. doi: 10.1016/j.amjmed.2020.05.049
2. Compston A, Coles A. Multiple sclerosis. Lancet. (2008) 372:1502–17. doi: 10.1016/S0140-6736(08)61620-7
3. Lublin FD, Haring DA, Ganjgahi H, Ocampo A, Hatami F, Cuklina J, et al. How patients with multiple sclerosis acquire disability. Brain. (2022) 145:3147–61. doi: 10.1093/brain/awac016
4. Younger DS. Multiple sclerosis: Motor dysfunction. Handb Clin Neurol. (2023) 196:119–47. doi: 10.1016/B978-0-323-98817-9.00016-8
5. Christopher A, Kraft E, Olenick H, Kiesling R, Doty A. The reliability and validity of the Timed Up and Go as a clinical tool in individuals with and without disabilities across a lifespan: a systematic review. Disabil Rehabil. (2021) 43:1799–813. doi: 10.1080/09638288.2019.1682066
6. Ward M, Goldman MD. Epidemiology and pathophysiology of multiple sclerosis. Continuum (Minneap Minn). (2022) 28:988–1005. doi: 10.1212/CON.0000000000001136
7. Stoiloudis P, Kesidou E, Bakirtzis C, Sintila SA, Konstantinidou N, Boziki M, et al. The role of diet and interventions on multiple sclerosis: A review. Nutrients. (2022) 14(6):1150. doi: 10.3390/nu14061150
8. Bagnato F, Sati P, Hemond CC, Elliott C, Gauthier SA, Harrison DM, et al. Imaging chronic active lesions in multiple sclerosis: a consensus statement. Brain. (2024) 147(9):2913–33. doi: 10.1093/brain/awae013
9. Oechtering J, Stein K, Schaedelin SA, Maceski AM, Orleth A, Meier S, et al. Complement activation is associated with disease severity in multiple sclerosis. Neurol Neuroimmunol Neuroinflamm. (2024) 11:e200212. doi: 10.1212/NXI.0000000000200212
10. Lin JP, Brake A, Donadieu M, Lee A, Kawaguchi R, Sati P, et al. A 4D transcriptomic map for the evolution of multiple sclerosis-like lesions in the marmoset brain. bioRxiv. (2023) 2023.09.25.559371. doi: 10.1101/2023.09.25.559371
11. Wiggermann V, Endmayr V, Hernandez-Torres E, Hoftberger R, Kasprian G, Hametner S, et al. Quantitative magnetic resonance imaging reflects different levels of histologically determined myelin densities in multiple sclerosis, including remyelination in inactive multiple sclerosis lesions. Brain Pathol. (2023) 33:e13150. doi: 10.1111/bpa.13150
12. Perez CA, Cuascut FX, Hutton GJ. Immunopathogenesis, diagnosis, and treatment of multiple sclerosis: A clinical update. Neurol Clin. (2023) 41:87–106. doi: 10.1016/j.ncl.2022.05.004
13. Rodriguez Murua S, Farez MF, Quintana FJ. The immune response in multiple sclerosis. Annu Rev Pathol. (2022) 17:121–39. doi: 10.1146/annurev-pathol-052920-040318
14. Kukanja P, Langseth CM, Rubio-Rodriguez-Kirby LA, Agirre E, Zheng C, Raman A, et al. Cellular architecture of evolving neuroinflammatory lesions and multiple sclerosis pathology. Cell. (2024) 187:1990–2009.e19. doi: 10.1016/j.cell.2024.02.030
15. McGinley MP, Goldschmidt CH, Rae-Grant AD. Diagnosis and treatment of multiple sclerosis: A review. JAMA. (2021) 325:765–79. doi: 10.1001/jama.2020.26858
16. Kuhlmann T, Moccia M, Coetzee T, Cohen JA, Correale J, Graves J, et al. Multiple sclerosis progression: time for a new mechanism-driven framework. Lancet Neurol. (2023) 22:78–88. doi: 10.1016/S1474-4422(22)00289-7
17. Ruiz F, Vigne S, Pot C. Resolution of inflammation during multiple sclerosis. Semin Immunopathol. (2019) 41:711–26. doi: 10.1007/s00281-019-00765-0
18. Dendrou CA, Fugger L, Friese MA. Immunopathology of multiple sclerosis. Nat Rev Immunol. (2015) 15:545–58. doi: 10.1038/nri3871
19. Klotz L, Antel J, Kuhlmann T. Inflammation in multiple sclerosis: consequences for remyelination and disease progression. Nat Rev Neurol. (2023) 19:305–20. doi: 10.1038/s41582-023-00801-6
20. Islamuddin M, Mustfa SA, Ullah S, Omer U, Kato K, Parveen S. Innate immune response and inflammasome activation during SARS-CoV-2 infection. Inflammation. (2022) 45:1849–63. doi: 10.1007/s10753-022-01651-y
21. Wei L, Wang X, Zhou H. Interaction among inflammasome, PANoptosise, and innate immune cells in infection of influenza virus: Updated review. Immun Inflammation Dis. (2023) 11:e997. doi: 10.1002/iid3.997
22. Yu G, Choi YK, Lee S. Inflammasome diversity: exploring novel frontiers in the innate immune response. Trends Immunol. (2024) 45:248–58. doi: 10.1016/j.it.2024.02.004
23. Wang L, Cai J, Zhao X, Ma L, Zeng P, Zhou L, et al. Palmitoylation prevents sustained inflammation by limiting NLRP3 inflammasome activation through chaperone-mediated autophagy. Mol Cell. (2023) 83:281–297.e10. doi: 10.1016/j.molcel.2022.12.002
24. Li W, Cao T, Luo C, Cai J, Zhou X, Xiao X, et al. Crosstalk between ER stress, NLRP3 inflammasome, and inflammation. Appl Microbiol Biotechnol. (2020) 104:6129–40. doi: 10.1007/s00253-020-10614-y
25. Kong P, Cui ZY, Huang XF, Zhang DD, Guo RJ, Han M. Inflammation and atherosclerosis: signaling pathways and therapeutic intervention. Signal Transduct Target Ther. (2022) 7:131. doi: 10.1038/s41392-022-00955-7
26. Yan Z, Qi Z, Yang X, Ji N, Wang Y, Shi Q, et al. The NLRP3 inflammasome: Multiple activation pathways and its role in primary cells during ventricular remodeling. J Cell Physiol. (2021) 236:5547–63. doi: 10.1002/jcp.30285
27. Jiang Q, Wang X, Xu X, Hu L, Zhou G, Liu R, et al. Inflammasomes in rheumatoid arthritis: a pilot study. BMC Rheumatol. (2023) 7:39. doi: 10.1186/s41927-023-00353-8
28. Chiarini A, Armato U, Gui L, Dal Pra I. Other than NLRP3” Inflammasomes: multiple roles in brain disease. Neuroscientist. (2024) 30:23–48. doi: 10.1177/10738584221106114
29. Fu J, Wu H. Structural mechanisms of NLRP3 inflammasome assembly and activation. Annu Rev Immunol. (2023) 41:301–16. doi: 10.1146/annurev-immunol-081022-021207
30. Toldo S, Mezzaroma E, Buckley LF, Potere N, Di Nisio M, Biondi-Zoccai G, et al. Targeting the NLRP3 inflammasome in cardiovascular diseases. Pharmacol Ther. (2022) 236:108053. doi: 10.1016/j.pharmthera.2021.108053
31. Olona A, Leishman S, Anand PK. The NLRP3 inflammasome: regulation by metabolic signals. Trends Immunol. (2022) 43:978–89. doi: 10.1016/j.it.2022.10.003
32. McManus RM, Latz E. NLRP3 inflammasome signalling in Alzheimer’s disease. Neuropharmacology. (2024) 252:109941. doi: 10.1016/j.neuropharm.2024.109941
33. Balci CN, Acar N. NLRP3 inflammasome pathway, the hidden balance in pregnancy: A comprehensive review. J Reprod Immunol. (2024) 161:104173. doi: 10.1016/j.jri.2023.104173
34. Zhao C, Zhao W. NLRP3 inflammasome-A key player in antiviral responses. Front Immunol. (2020) 11:211. doi: 10.3389/fimmu.2020.00211
35. Napodano C, Carnazzo V, Basile V, Pocino K, Stefanile A, Gallucci S, et al. NLRP3 inflammasome involvement in heart, liver, and lung diseases-A lesson from cytokine storm syndrome. Int J Mol Sci. (2023) 24(23):16556. doi: 10.3390/ijms242316556
36. Jiang H, Gong T, Zhou R. The strategies of targeting the NLRP3 inflammasome to treat inflammatory diseases. Adv Immunol. (2020) 145:55–93. doi: 10.1016/bs.ai.2019.11.003
37. Shao BZ, Cao Q, Liu C. Targeting NLRP3 inflammasome in the treatment of CNS diseases. Front Mol Neurosci. (2018) 11:320. doi: 10.3389/fnmol.2018.00320
38. Di Q, Zhao X, Tang H, Li X, Xiao Y, Wu H, et al. USP22 suppresses the NLRP3 inflammasome by degrading NLRP3 via ATG5-dependent autophagy. Autophagy. (2023) 19:873–85. doi: 10.1080/15548627.2022.2107314
39. Vande Walle L, Lamkanfi M. Drugging the NLRP3 inflammasome: from signalling mechanisms to therapeutic targets. Nat Rev Drug Discovery. (2024) 23:43–66. doi: 10.1038/s41573-023-00822-2
40. Shao BZ, Xu ZQ, Han BZ, Su DF, Liu C. NLRP3 inflammasome and its inhibitors: a review. Front Pharmacol. (2015) 6:262. doi: 10.3389/fphar.2015.00262
41. Huang Y, Xu W, Zhou R. NLRP3 inflammasome activation and cell death. Cell Mol Immunol. (2021) 18:2114–27. doi: 10.1038/s41423-021-00740-6
42. Kodi T, Sankhe R, Gopinathan A, Nandakumar K, Kishore A. New insights on NLRP3 inflammasome: mechanisms of activation, inhibition, and epigenetic regulation. J Neuroimmune Pharmacol. (2024) 19:7. doi: 10.1007/s11481-024-10101-5
43. Liu Y, Dai Y, Li Q, Chen C, Chen H, Song Y, et al. Beta-amyloid activates NLRP3 inflammasome via TLR4 in mouse microglia. Neurosci Lett. (2020) 736:135279. doi: 10.1016/j.neulet.2020.135279
44. Zhang Y, Ren X, Zhang L, Sun X, Li W, Chen Y, et al. Hydrogen gas inhalation ameliorates LPS-induced BPD by inhibiting inflammation via regulating the TLR4-NFkappaB-IL6/NLRP3 signaling pathway in the placenta. Eur J Med Res. (2024) 29:285. doi: 10.1186/s40001-024-01874-9
45. Zhou H, Zhang Q, Huang W, Zhou S, Wang Y, Zeng X, et al. NLRP3 inflammasome mediates silica-induced lung epithelial injury and aberrant regeneration in lung stem/progenitor cell-derived organotypic models. Int J Biol Sci. (2023) 19:1875–93. doi: 10.7150/ijbs.80605
46. Zhu M, Liu Y, Chen C, Chen H, Ni W, Song Y, et al. TLR4/rac1/NLRP3 pathway mediates amyloid-beta-induced neuroinflammation in alzheimer’s disease. J Alzheimers Dis. (2024) 99:911–25. doi: 10.3233/JAD-240012
47. Huang S, Dong W, Lin X, Xu K, Li K, Xiong S, et al. Disruption of the Na(+)/K(+)-ATPase-purinergic P2X7 receptor complex in microglia promotes stress-induced anxiety. Immunity. (2024) 57:495–512.e11. doi: 10.1016/j.immuni.2024.01.018
48. Shi W, Liu T, Yang H, Zhao J, Wei Z, Huang Y, et al. Isomaculosidine facilitates NLRP3 inflammasome activation by promoting mitochondrial reactive oxygen species production and causes idiosyncratic liver injury. J Ethnopharmacol. (2024) 319:117063. doi: 10.1016/j.jep.2023.117063
49. Jiang H, Xie Y, Lu J, Li H, Zeng K, Hu Z, et al. Pristimerin suppresses AIM2 inflammasome by modulating AIM2-PYCARD/ASC stability via selective autophagy to alleviate tendinopathy. Autophagy. (2024) 20:76–93. doi: 10.1080/15548627.2023.2249392
50. Toldo S, Abbate A. The role of the NLRP3 inflammasome and pyroptosis in cardiovascular diseases. Nat Rev Cardiol. (2024) 21:219–37. doi: 10.1038/s41569-023-00946-3
51. Ding P, Song Y, Yang Y, Zeng C. NLRP3 inflammasome and pyroptosis in cardiovascular diseases and exercise intervention. Front Pharmacol. (2024) 15:1368835. doi: 10.3389/fphar.2024.1368835
52. Zhong Y, Li XY, Liang TJ, Ding BZ, Ma KX, Ren WX, et al. Effects of NLRP3 inflammasome mediated pyroptosis on cardiovascular diseases and intervention mechanism of Chinese medicine. Chin J Integr Med. (2024) 30:468–79. doi: 10.1007/s11655-024-3655-2
53. Qiang R, Li Y, Dai X, Lv W. NLRP3 inflammasome in digestive diseases: From mechanism to therapy. Front Immunol. (2022) 13:978190. doi: 10.3389/fimmu.2022.978190
54. Wen J, Xuan B, Liu Y, Wang L, He L, Meng X, et al. NLRP3 inflammasome-induced pyroptosis in digestive system tumors. Front Immunol. (2023) 14:1074606. doi: 10.3389/fimmu.2023.1074606
55. Zhou L, Qiu X, Meng Z, Liu T, Chen Z, Zhang P, et al. Hepatic danger signaling triggers TREM2(+) macrophage induction and drives steatohepatitis via MS4A7-dependent inflammasome activation. Sci Transl Med. (2024) 16:eadk1866. doi: 10.1126/scitranslmed.adk1866
56. Li Z, Yang F, Zhu X, Zhou B, Jin K, Dai J, et al. Effect of NLRP3 inflammasome on lung cancer immune microenvironment activation and its mechanism. Altern Ther Health Med. (2024) 30(11):86–91.
57. Cheng Z, Huang M, Li W, Hou L, Jin L, Fan Q, et al. HECTD3 inhibits NLRP3 inflammasome assembly and activation by blocking NLRP3-NEK7 interaction. Cell Death Dis. (2024) 15:86. doi: 10.1038/s41419-024-06473-4
58. Feng SH, Zhao B, Zhan X, Li RH, Yang Q, Wang SM, et al. Quercetin-induced pyroptosis in colon cancer through NEK7-mediated NLRP3 inflammasome-GSDMD signaling pathway activation. Am J Cancer Res. (2024) 14:934–58. doi: 10.62347/MKAN3550
59. Zhu W, Zhang H, Niu T, Liu K, Fareeduddin Mohammed Farooqui H, Sun R, et al. Microglial SCAP deficiency protects against diabetes-associated cognitive impairment through inhibiting NLRP3 inflammasome-mediated neuroinflammation. Brain Behav Immun. (2024) 119:154–70. doi: 10.1016/j.bbi.2024.03.051
60. Zhong C, Xie Y, Wang H, Chen W, Yang Z, Zhang L, et al. Berberine inhibits NLRP3 inflammasome activation by regulating mTOR/mtROS axis to alleviate diabetic cardiomyopathy. Eur J Pharmacol. (2024) 964:176253. doi: 10.1016/j.ejphar.2023.176253
61. Vandanmagsar B, Youm YH, Ravussin A, Galgani JE, Stadler K, Mynatt RL, et al. The NLRP3 inflammasome instigates obesity-induced inflammation and insulin resistance. Nat Med. (2011) 17:179–88. doi: 10.1038/nm.2279
62. Malhotra S, Costa C, Eixarch H, Keller CW, Amman L, Martinez-Banaclocha H, et al. NLRP3 inflammasome as prognostic factor and therapeutic target in primary progressive multiple sclerosis patients. Brain. (2020) 143:1414–30. doi: 10.1093/brain/awaa084
63. Lempriere S. NLRP3 inflammasome activity as biomarker for primary progressive multiple sclerosis. Nat Rev Neurol. (2020) 16:350. doi: 10.1038/s41582-020-0366-y
64. Soares JL, Oliveira EM, Pontillo A. Variants in NLRP3 and NLRC4 inflammasome associate with susceptibility and severity of multiple sclerosis. Mult Scler Relat Disord. (2019) 29:26–34. doi: 10.1016/j.msard.2019.01.023
65. Imani D, Azimi A, Salehi Z, Rezaei N, Emamnejad R, Sadr M, et al. Association of nod-like receptor protein-3 single nucleotide gene polymorphisms and expression with the susceptibility to relapsing-remitting multiple sclerosis. Int J Immunogenet. (2018) 45:329–36. doi: 10.1111/iji.12401
66. Vidmar L, Maver A, Drulovic J, Sepcic J, Novakovic I, Ristic S, et al. Multiple Sclerosis patients carry an increased burden of exceedingly rare genetic variants in the inflammasome regulatory genes. Sci Rep. (2019) 9:9171. doi: 10.1038/s41598-019-45598-x
67. Tamam Y, Yu H, du B, Sen M, Ulusoy C, Tuzun E. Serum levels of inflammasome pathway factors in clinically isolated syndrome and multiple sclerosis patients: a pilot study. Cent Eur J Immunol. (2020) 45:237–40. doi: 10.5114/ceji.2020.96877
68. Qiu D, Zhang D, Yu Z, Jiang Y, Zhu D. Bioinformatics approach reveals the critical role of the NOD-like receptor signaling pathway in COVID-19-associated multiple sclerosis syndrome. J Neural Transm (Vienna). (2022) 129:1031–8. doi: 10.1007/s00702-022-02518-0
69. Byun DJ, Lee J, Ko K, Hyun YM. NLRP3 exacerbates EAE severity through ROS-dependent NET formation in the mouse brain. Cell Commun Signal. (2024) 22:96. doi: 10.1186/s12964-023-01447-z
70. Wang G, Yin W, Shin H, Tian Q, Lu W, Hou SX. Neuronal accumulation of peroxidated lipids promotes demyelination and neurodegeneration through the activation of the microglial NLRP3 inflammasome. Nat Aging. (2021) 1:1024–37. doi: 10.1038/s43587-021-00130-7
71. Shao Y, Chen C, Zhu T, Sun Z, Li S, Gong L, et al. TRPM2 contributes to neuroinflammation and cognitive deficits in a cuprizone-induced multiple sclerosis model via NLRP3 inflammasome. Neurobiol Dis. (2021) 160:105534. doi: 10.1016/j.nbd.2021.105534
72. Seyedsadr M, Wang Y, Elzoheiry M, Shree Gopal S, Jang S, Duran G, et al. IL-11 induces NLRP3 inflammasome activation in monocytes and inflammatory cell migration to the central nervous system. Proc Natl Acad Sci U.S.A. (2023) 120:e2221007120. doi: 10.1073/pnas.2221007120
73. Zhao XY, Wang JQ, Neely GG, Shi YC, Wang QP. Natural compounds as obesity pharmacotherapies. Phytother Res. (2024) 38:797–838. doi: 10.1002/ptr.8083
74. Song Z, Li W, He Q, Xie X, Wang X, Guo J. Natural products - Dawn of keloid treatment. Fitoterapia. (2024) 175:105918. doi: 10.1016/j.fitote.2024.105918
75. Mizushima N, Komatsu M. Autophagy: renovation of cells and tissues. Cell. (2011) 147:728–41. doi: 10.1016/j.cell.2011.10.026
76. Kiasalari Z, Afshin-Majd S, Baluchnejadmojarad T, Azadi-Ahmadabadi E, Fakour M, Ghasemi-Tarie R, et al. Sinomenine alleviates murine experimental autoimmune encephalomyelitis model of multiple sclerosis through inhibiting NLRP3 inflammasome. J Mol Neurosci. (2021) 71:215–24. doi: 10.1007/s12031-020-01637-1
77. Abitbol A, Mallard B, Tiralongo E, Tiralongo J. Mushroom natural products in neurodegenerative disease drug discovery. Cells. (2022) 11(23):3938. doi: 10.3390/cells11233938
78. Cui YR, Bu ZQ, Yu HY, Yan LL, Feng J. Emodin attenuates inflammation and demyelination in experimental autoimmune encephalomyelitis. Neural Regener Res. (2023) 18:1535–41. doi: 10.4103/1673-5374.358612
79. Pan RY, Kong XX, Cheng Y, Du L, Wang ZC, Yuan C, et al. 1,2,4-Trimethoxybenzene selectively inhibits NLRP3 inflammasome activation and attenuates experimental autoimmune encephalomyelitis. Acta Pharmacol Sin. (2021) 42:1769–79. doi: 10.1038/s41401-021-00613-8
80. Zhou Y, Manghwar H, Hu W, Liu F. Degradation mechanism of autophagy-related proteins and research progress. Int J Mol Sci. (2022) 23(13):7301. doi: 10.3390/ijms23137301
81. Chen X, Yu C, Kang R, Kroemer G, Tang D. Cellular degradation systems in ferroptosis. Cell Death Differ. (2021) 28:1135–48. doi: 10.1038/s41418-020-00728-1
82. Paudel RR, Lu D, Roy Chowdhury S, Monroy EY, Wang J. Targeted protein degradation via lysosomes. Biochemistry. (2023) 62:564–79. doi: 10.1021/acs.biochem.2c00310
83. Rashid S, Dimitriadi M. Autophagy in spinal muscular atrophy: from pathogenic mechanisms to therapeutic approaches. Front Cell Neurosci. (2023) 17:1307636. doi: 10.3389/fncel.2023.1307636
84. Kurusu R, Morishita H, Komatsu M. Vault-phagy: a phase-separation-mediated selective autophagy of vault, a non-membranous organelle. Autophagy. (2024) 20:441–2. doi: 10.1080/15548627.2023.2266996
85. Deretic V. Autophagy in inflammation, infection, and immunometabolism. Immunity. (2021) 54:437–53. doi: 10.1016/j.immuni.2021.01.018
86. Zuo H, Chen C, Sa Y. Therapeutic potential of autophagy in immunity and inflammation: current and future perspectives. Pharmacol Rep. (2023) 75:499–510. doi: 10.1007/s43440-023-00486-0
87. Goswami AB, Karadarevic D, Castano-Rodriguez N. Immunity-related GTPase IRGM at the intersection of autophagy, inflammation, and tumorigenesis. Inflammation Res. (2022) 71:785–95. doi: 10.1007/s00011-022-01595-x
88. Song S, Guo R, Mehmood A, Zhang L, Yin B, Yuan C, et al. Liraglutide attenuate central nervous inflammation and demyelination through AMPK and pyroptosis-related NLRP3 pathway. CNS Neurosci Ther. (2022) 28:422–34. doi: 10.1111/cns.13791
89. Ammar RA, Mohamed AF, Kamal MM, Safar MM, Abdelkader NF. Neuroprotective effect of liraglutide in an experimental mouse model of multiple sclerosis: role of AMPK/SIRT1 signaling and NLRP3 inflammasome. Inflammopharmacology. (2022) 30:919–34. doi: 10.1007/s10787-022-00956-6
90. He M, Wu DM, Zhao YY, Yu Y, Deng SH, Liu T, et al. AZD8055 ameliorates experimental autoimmune encephalomyelitis via the mTOR/ROS/NLRP3 pathway. Biochem Biophys Res Commun. (2021) 573:27–34. doi: 10.1016/j.bbrc.2021.08.010
91. Shao BZ, Ke P, Xu ZQ, Wei W, Cheng MH, Han BZ, et al. Autophagy plays an important role in anti-inflammatory mechanisms stimulated by alpha7 nicotinic acetylcholine receptor. Front Immunol. (2017) 8:553. doi: 10.3389/fimmu.2017.00553
92. Shao BZ, Wei W, Ke P, Xu ZQ, Zhou JX, Liu C. Activating cannabinoid receptor 2 alleviates pathogenesis of experimental autoimmune encephalomyelitis via activation of autophagy and inhibiting NLRP3 inflammasome. CNS Neurosci Ther. (2014) 20:1021–8. doi: 10.1111/cns.12349
93. Coll RC, Robertson AA, Chae JJ, Higgins SC, Munoz-Planillo R, Inserra MC, et al. A small-molecule inhibitor of the NLRP3 inflammasome for the treatment of inflammatory diseases. Nat Med. (2015) 21:248–55. doi: 10.1038/nm.3806
94. Hou B, Yin J, Liu S, Guo J, Zhang B, Zhang Z, et al. Inhibiting the NLRP3 inflammasome with MCC950 alleviates neurological impairment in the brain of EAE mice. Mol Neurobiol. (2024) 61:1318–30. doi: 10.1007/s12035-023-03618-y
95. Xu L, Zhang C, Jiang N, He D, Bai Y, Xin Y. Rapamycin combined with MCC950 to treat multiple sclerosis in experimental autoimmune encephalomyelitis. J Cell Biochem. (2019) 120:5160–8. doi: 10.1002/jcb.27792
96. Bakhshi S, Shamsi S. MCC950 in the treatment of NLRP3-mediated inflammatory diseases: Latest evidence and therapeutic outcomes. Int Immunopharmacol. (2022) 106:108595. doi: 10.1016/j.intimp.2022.108595
97. Chen C, Zhou Y, Ning X, Li S, Xue D, Wei C, et al. Directly targeting ASC by lonidamine alleviates inflammasome-driven diseases. J Neuroinflamm. (2022) 19:315. doi: 10.1186/s12974-022-02682-w
Keywords: multiple sclerosis, NLRP3 inflammasome, inflammation, experimental autoimmune encephalomyelitis, autophagy
Citation: Zhang W-G, Zheng X-R, Yao Y, Sun W-J and Shao B-Z (2025) The role of NLRP3 inflammasome in multiple sclerosis: pathogenesis and pharmacological application. Front. Immunol. 16:1572140. doi: 10.3389/fimmu.2025.1572140
Received: 20 February 2025; Accepted: 21 March 2025;
Published: 02 April 2025.
Edited by:
Luisa María Villar, Ramón y Cajal University Hospital, SpainReviewed by:
Yuki Yokota, Nihon University, JapanCopyright © 2025 Zhang, Zheng, Yao, Sun and Shao. This is an open-access article distributed under the terms of the Creative Commons Attribution License (CC BY). The use, distribution or reproduction in other forums is permitted, provided the original author(s) and the copyright owner(s) are credited and that the original publication in this journal is cited, in accordance with accepted academic practice. No use, distribution or reproduction is permitted which does not comply with these terms.
*Correspondence: Bo-Zong Shao, c2hhb2Jvem9uZ0AxMjYuY29t; Wei-Jia Sun, bml1bml1MTk4NDIwMDZAMTYzLmNvbQ==
†These authors have contributed equally to this work
Disclaimer: All claims expressed in this article are solely those of the authors and do not necessarily represent those of their affiliated organizations, or those of the publisher, the editors and the reviewers. Any product that may be evaluated in this article or claim that may be made by its manufacturer is not guaranteed or endorsed by the publisher.
Research integrity at Frontiers
Learn more about the work of our research integrity team to safeguard the quality of each article we publish.