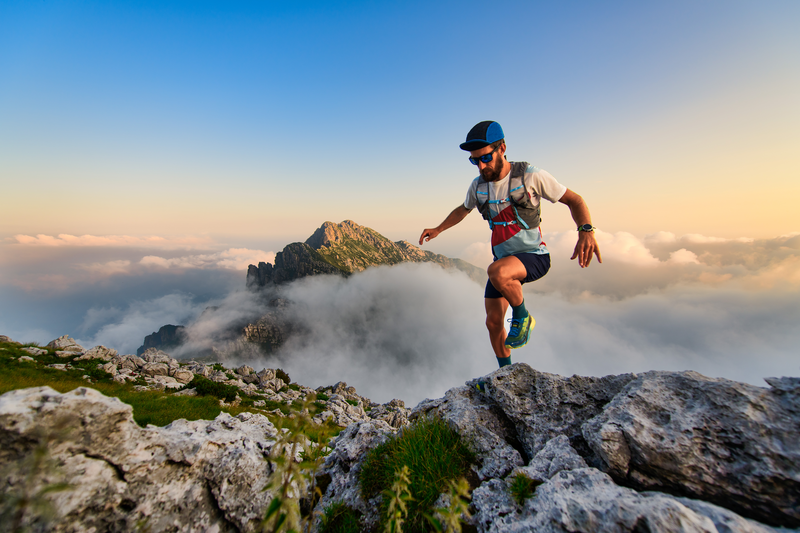
94% of researchers rate our articles as excellent or good
Learn more about the work of our research integrity team to safeguard the quality of each article we publish.
Find out more
ORIGINAL RESEARCH article
Front. Immunol.
Sec. Alloimmunity and Transplantation
Volume 16 - 2025 | doi: 10.3389/fimmu.2025.1568862
The final, formatted version of the article will be published soon.
You have multiple emails registered with Frontiers:
Please enter your email address:
If you already have an account, please login
You don't have a Frontiers account ? You can register here
Post-transplant cyclophosphamide (PT-CY) has been pivotal in controlling graftversus-host disease (GvHD) following T-cell-replete haploidentical hematopoietic cell transplantation (haplo-HCT). However, the widely adopted regimen is associated with high relapse rates, particularly in patients without GvHD. Our preclinical studies indicate that pre-or post-transplant bendamustine (PT-BEN) may reduce GvHD, enhance graft-versus-leukemia (GvL) effects, and induce significant alterations in the proportion, phenotype, and function of various immune cell subsets. We initiated a Phase Ia/Ib, single-center trial with a standard 3+3 dose-escalation design, sequentially replacing post-transplant (PT)-CY with BEN (PT-CY/BEN). Multi-parameter flow cytometry and TCR β sequencing of genomic DNA was performed on isolated PBMCs on days +30, +60, +100, +180, and +365. Overall, the PT-CY/BEN (n=14) regimen was associated with earlier neutrophil and platelet engraftment, reduced transfusion requirements, and comparable clinical outcomes to PT-CY (n=10), including survival and relapse rates. PT-CY/BEN patients exhibited distinct immune reconstitution patterns, characterized by earlier CD4+ T-cell recovery, impaired CD8+ T-cell engraftment, and reduced NK-cell counts. Notably there were no significant changes in B-cells, Tregs, or MDSCs. Enhanced T-cell repertoire diversity in the PT-CY/BEN cohort was associated with improved CMV control. In conclusion, our Phase Ia findings demonstrate the well-tolerability of PT-CY/BEN and its association with early engraftment, a more diverse T-cell repertoire, and earlier CD4+ T-cell reconstitution. Future studies are warranted to confirm our findings and investigate potential additional benefits of PT-CY/BEN over PT-CY alone.
Keywords: bendamustine, Cyclophosphamide, Hematopoietic Cell Transplantation, TCR-β sequencing, T-cells, Cytomegalovirus
Received: 30 Jan 2025; Accepted: 06 Mar 2025.
Copyright: © 2025 Baker, Stokes, Cracchiolo, Davini, Simpson and Katsanis. This is an open-access article distributed under the terms of the Creative Commons Attribution License (CC BY). The use, distribution or reproduction in other forums is permitted, provided the original author(s) or licensor are credited and that the original publication in this journal is cited, in accordance with accepted academic practice. No use, distribution or reproduction is permitted which does not comply with these terms.
* Correspondence:
Emmanuel Katsanis, University of Arizona, Tucson, United States
Disclaimer: All claims expressed in this article are solely those of the authors and do not necessarily represent those of their affiliated organizations, or those of the publisher, the editors and the reviewers. Any product that may be evaluated in this article or claim that may be made by its manufacturer is not guaranteed or endorsed by the publisher.
Research integrity at Frontiers
Learn more about the work of our research integrity team to safeguard the quality of each article we publish.