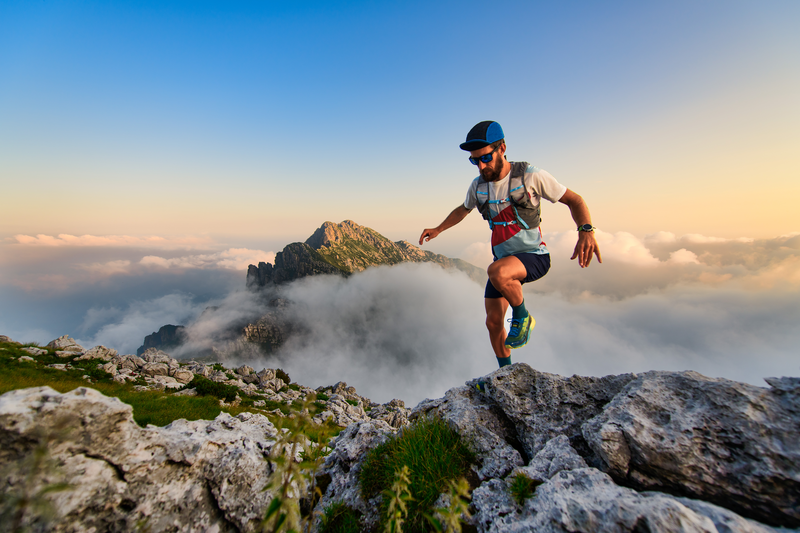
94% of researchers rate our articles as excellent or good
Learn more about the work of our research integrity team to safeguard the quality of each article we publish.
Find out more
ORIGINAL RESEARCH article
Front. Immunol.
Sec. Molecular Innate Immunity
Volume 16 - 2025 | doi: 10.3389/fimmu.2025.1567522
The final, formatted version of the article will be published soon.
You have multiple emails registered with Frontiers:
Please enter your email address:
If you already have an account, please login
You don't have a Frontiers account ? You can register here
Background: Jing-Yin-Gu-Biao formula (JYGBF) is a Chinese medicine derived from Yupingfeng power, Huoxiangzhengqi powder and Yinqiao powder, and has been widely used to treat acute respiratory infections. This study aims to observe the effects of JYGBF against postinfluenza Staphylococcus aureus (S. aureus) infection. Purpose and study design: A mouse model of secondary S. aureus infection following PR8 infection was established to evaluate the protective effects of JYGBF against postinfluenza Staphylococcus aureus (S. aureus) infection and related mechanisms were validated in vivo and in vitro. Results: The administration of JYGBF significantly ameliorated acute lung injury (ALI) and inhibited overactivated inflammatory response (MIP-2, IL-6, etc.) in mice with postinfluenza S. aureus infection. Single cell RNA-sequencing (scRNA-seq) data indicated that neutrophils had the highest cytokine score in lungs and JYGBF inhibited neutrophil chemotaxis, reactive oxygen species (ROS) biosynthesis and ERK1/2 cascades in neutrophils. Meanwhile, JYGBF inhibited the formation of neutrophil extracellular traps (NETs) in lungs, which is characterized by the production of ROS, peptidyl arginine deiminase 4 (PAD4), citrullinated histone H3 (CitH3), and myeloperoxidase (MPO), neutrophil elastase (NE), S100A8/A9 and MPO-CitH3 colocalization. Moreover, JYGBF decreased platelet counts and the expression of its activated markers (CD62P and αIIbβ3) accompanied by the drop of fibrinogen (FIB) and fibrin degradation product (FDP), accounting for alleviating hypercoagulable state. JYGBF inhibited ERK1/2 phosphorylation in neutrophils and in lungs of flu mice. Acacetin, a critical compound from JYGBF, inhibited NET formation via downregulating ERK/ROS axis. Conclusions: These results indicated that JYGBF inhibited NET formation and overactivated inflammatory response by suppressing ERK/ROS axis in neutrophils, thereby mitigating ALI and improving the hypercoagulable state during postinfluenza S. aureus infection. JYGBF could be considered a potent therapeutic agent for the prevention and treatment of postinfluenza bacterial infection.
Keywords: Jing-Yin-Gu-Biao formula, postinfluenza Staphylococcus aureus infection, Neutrophil extracellular trap, Platelet Activation, ERK/ROS axis
Received: 27 Jan 2025; Accepted: 19 Feb 2025.
Copyright: © 2025 Biao, Mu, Xu, Yang, Huang, Hu, Liu, Cheng, Ma, Liang, Xu, Lin, Zhou, Zhou, Wei and Zheng. This is an open-access article distributed under the terms of the Creative Commons Attribution License (CC BY). The use, distribution or reproduction in other forums is permitted, provided the original author(s) or licensor are credited and that the original publication in this journal is cited, in accordance with accepted academic practice. No use, distribution or reproduction is permitted which does not comply with these terms.
* Correspondence:
Chunxian Zhou, Shanghai University of Traditional Chinese Medicine, Shanghai, 201203, Shanghai Municipality, China
Zhang Wei, Shanghai University of Traditional Chinese Medicine, Shanghai, 201203, Shanghai Municipality, China
Yuejuan Zheng, Shanghai University of Traditional Chinese Medicine, Shanghai, 201203, Shanghai Municipality, China
Disclaimer: All claims expressed in this article are solely those of the authors and do not necessarily represent those of their affiliated organizations, or those of the publisher, the editors and the reviewers. Any product that may be evaluated in this article or claim that may be made by its manufacturer is not guaranteed or endorsed by the publisher.
Research integrity at Frontiers
Learn more about the work of our research integrity team to safeguard the quality of each article we publish.