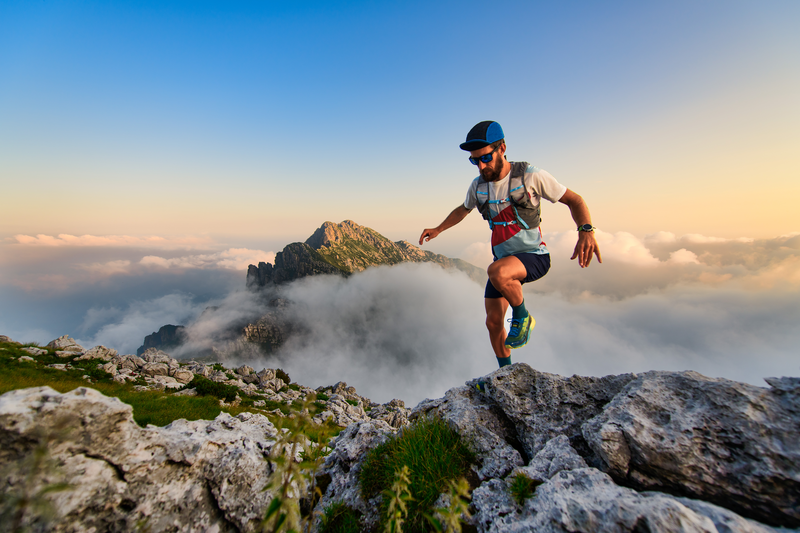
95% of researchers rate our articles as excellent or good
Learn more about the work of our research integrity team to safeguard the quality of each article we publish.
Find out more
ORIGINAL RESEARCH article
Front. Immunol. , 31 March 2025
Sec. Comparative Immunology
Volume 16 - 2025 | https://doi.org/10.3389/fimmu.2025.1566496
This article is part of the Research Topic Molecular and Cellular Insights into Fish Immunity: Adaptations to Pathogens and Environmental Challenges View all articles
Introduction: Diclofenac (DCF) is a commonly utilized medication in the non-steroidal anti-inflammatory drug category that is released into aquatic systems in significant amounts. Chlorella vulgaris (C. vulgaris) is rich in active phytochemicals known for their haemato-immunological boosting properties.
Methods: Our objective was to investigate the haemato-immunological protective properties of Chlorella in mitigating the toxic effects of DCF. Five groups of Clarias gariepinus, each comprising 36 fish, were assigned over a two-week period. The groups were assigned as follows: control group, which received a basal diet only; DCF1 group, which received a basal diet and was exposed to 20 μg/L of DCF; DCF2 group, which received a basal diet and was exposed to 10 mg/L of DCF; and Chlorella +DCF1 and Chlorella+DCF2 groups, which were exposed to the same DCF doses as Groups 2 and 3, respectively, while also being fed a diet containing 25% Chlorella.
Results: Exposure to both doses of DCF significantly decreased erythrocyte count, hemoglobin content, white blood cell count, phagocytic index, and lysozyme activity, while increased eosinophil and neutrophil % in an equipotent manner. The low dose caused a more pronounced reduction in packed cell volume (PCV)% and large lymphocyte% compared to the high dose. A significant decline in platelet count was observed only with the low DCF dose, while the high dose led to a decrease in monocyte%. DCF intoxication led to a dose-related decrease in small lymphocyte% and an increase in erythrocyte morphological alterations and interleukin (IL)-6 levels. The DCF2 group exhibited a higher increase in apoptotic RBCs than the DCF1 group. Intervention with Chlorella alongside the two DCF doses significantly normalized RBC count and eosinophil %, increased PCV% and small lymphocyte%, and decreased erythrocyte abnormalities to an equal extent. Large lymphocyte% in the Chlorella+DCF1 group was successfully restored to normal levels. Phagocytic index and lysozyme activity in the supplemented groups were lower, while IL-6 levels were higher than in the DCF groups. The percentage of apoptotic cells decreased with Chlorella administration, with the Chlorella+DCF1 group showing fewer apoptotic cells than the Chlorella+DCF2 group. Histopathological deterioration and excessive collagen deposition were observed in the spleen of DCF groups, while notable improvements were seen following C. vulgaris supplementation.
Conclusion: These findings suggest that dietary inclusion of C. vulgaris may antagonize the haemato-cytological abnormalities induced by DCF intoxication.
Pharmaceuticals encompass a wide range of remedial substances utilized for the prevention, diagnosis, treatment, and cure of diseases in both humans and animals, with their global consumption being substantial (1). The extensive use of medications inevitably results in their introduction into the environment, primarily through excretions in either their unchanged form or as metabolites (2). Other significant sources include discharges from manufacturing plants, hospitals, and households, as well as improper disposal in landfills (3). Over the past two decades, numerous studies have reported trace levels of pharmaceuticals in subterranean and surface-level water waters, wastewater treatment plant effluents, and even potable drinking water due to their ability to bypass treatment processes (4, 5). From ecological standpoint, over-the-counter prescription drugs like non-steroidal anti-inflammatory drugs (NSAIDs), commonly used for their pain-relieving, fever-reducing, and inflammation-reducing properties, have a considerable likelihood of becoming widespread in ecosystems because of their easy availability and potential for misuse (6). Diclofenac (DCF) is one of the widely used NSAIDs. In Germany, its annual consumption is 1033 mg per person (7). In Turkey, it was 950 mg per person in 2009 and increased to 985 mg per person in 2013 (8). In the Netherlands, the consumption rate is 440 mg per person per year (9). However, the consumption rate of DCF in developing countries is expected to rise, where self-medication is prevalent due to inadequate pharmaceutical care (10). DCF, even at low exposure levels, can exhibit ecotoxicological concerns on various non-target organisms like fish, owing to its ability to penetrate cellular biological barriers (11). DCF has been identified in surface and groundwater because conventional activated sludge processes are unable to fully eliminate these contaminants (12, 13).
African catfish (Clarias gariepinus) was chosen as the model organism for this study due to its widespread cultivation, abundance in Africa and other tropical regions, nutritional importance, and adaptability to laboratory conditions. It is valued for its year-round availability and ability to thrive across diverse environments, making it suitable for ecotoxicological research (14, 15). Despite typically being bottom feeders, Clarias gariepinus are adaptable omnivores, adjusting their feeding behaviors to different water depths, which increases their susceptibility to aquatic pollutants (6). Given that aquatic contaminants can directly contact fish gills and enter their bloodstream (16), hematological and cytotoxic biological indicators in fish erythrocytes serve as an effective biomonitoring tool of environmental pollutants and stressors (17). DCF exposure in catfish was incriminated in inducing adverse impacts on erythrocytic indices, leucocytic profile, and redox status (18–20).
C. vulgaris, a unicellular freshwater green alga, has long been utilized as a nutritional supplement because it is rich in phytochemically active ingredients, including essential amino acids, proteins, dietary fiber, polyunsaturated fatty acids, polysaccharides, micronutrients, redox stabilizers, and photosynthetic pigments (21–23). C. vulgaris has been shown to enhance erythrocytic and leucocytic indices, and boost innate and acquired immunity against deltamethrin toxicity by up-regulating immune-related gene expression in the spleens and liver of Nile tilapia (Oreochromis niloticus) (24). Additionally, C. vulgaris mitigated inflammation caused by diazinon through the suppression of tumor necrosis factor-α gene expression and up-regulating interleukin (IL)-10 gene expression in Oreochromis niloticus (25). It also improved the percentage of poikilocytosis and nuclear deformities of RBCs in microplastics-intoxicated Clarias gariepinus (26). Therefore, this study aims to investigate the potential protective effect of C. vulgaris against DCF exposure in Clarias gariepinus, using hematological parameters, erythron profiles, and spleen histological alterations as endpoint markers.
Diclofenac (Voltaren) with the chemical formula C14H11Cl2NO2 was sourced from a local drug store with purity ≥ 98%. A stock solution was freshly formulated by dissolving 0.1 grams of Voltaren in one liter of distilled water.
C. vulgaris was purchased from the Institute of National Research Center (Cairo, Egypt) as a dried powder and incorporated into the diet at a concentration of 25 g/kg. Fish were fed twice as 5% body weight.
Adult Clarias gariepinus were sourced from Aquaponic unit at Assiut, Egypt, and brought to the Fish Biology and Environmental Pollution Laboratory at the Faculty of Science, Assiut University. The test water had the following physicochemical properties: temperature of 28.5°C, pH of 7.4, dissolved oxygen concentration of 6.9 mg/L, 12:12 hour light-dark cycle, and conductivity of 260.8 mM/cm.
During the two-week experimental period, five groups, with 36 fish in each group, were allocated to three replicates per treatment group (12 fish per glass tank). Fish were kept in aquariums (30 cm length, 60 cm wide, 100 cm high) filled with 60 L of naturally dechlorinated water and aerated continuously with air compressors.
Control group received a standard diet (The fish were fed a commercial basal diet containing 30% crude protein purchased from Elshrok Campany, 10th Ramdan city, Cairo). Group 2 received a standard diet and was exposed to 20 µg/L of DCF (20) Group 3 received a standard diet and was exposed to 10 mg/L of DCF. Group 4 received a diet containing 25% chlorella and was exposed to 20 µg/L of DCF. Group 5 received a diet containing 25% chlorella and was exposed to 10 mg/L of DCF. The fish were given a pellet diet equivalent to 5% of their average body weight daily, and 50% of the water was replaced each day to remove wastes, with redosing to maintain the experimental conditions.
At the conclusion of the exposure period, six fish from each treatment group were randomly selected and anesthetized using ice to reduce handling stress (27). These fish were then used for hematological and biochemical measurements, erythron profile analysis on glass slides, and histopathological analysis of the spleen.
After the two-week exposure, blood samples were collected via caudal incision and placed on clean glass slides. Red blood cell (RBC) and total white blood cell (WBC) counts were assessed using the hemocytometer method under a light microscope (28). The packed cell volume (PCV) was determined using the micro-hematocrit method outlined by Hesser (29). Hemoglobin levels (Hb, mg/L) were measured colorimetrically as described by Lee et al. (30) by assessing the formation of cyanomethemoglobin. The red cell indicators, such as mean corpuscular hemoglobin (MCH: µg/cell), mean corpuscular volume (MCV: µm³/cell), and mean corpuscular hemoglobin concentration (MCHC: g/dl), were determined using RBC count, hemoglobin levels, and hematocrit, based on the formulas provided by Lee et al. (30).
Lysozyme activity was assessed using a turbidity assay method (31). The phagocytic activity of leukocytes was determined following the procedure outlined by Siwicki et al. (32). Interleukin-6 (IL-6) levels were quantified using a standard method (33).
Blood smears (six slides per fish) were prepared, air-dried, fixed in absolute methanol for one minute, and stained with May-Grünwald solution (Wako, Japan). Slides were chosen based on staining quality and then randomized for blind scoring. A total of 10,000 cells per group (with a minimum of 1000 cells per slide) were examined under a 40× objective lens to detect micronucleated cells and other morphological and nuclear abnormalities in RBCs, following the procedure outlined by Al-Sabti and Metcalfe (34). Micronuclei were recognized according to the criteria determined by Schmidt (35) to guarantee precise scoring.
After air-drying at room temperature, blood smears were fixed in absolute methanol for 10 seconds and subsequently stained with Acridine Orange (Life Technologies, Carlsbad, USA) to identify erythrocyte apoptosis. The apoptosis detection in RBCs followed a modified protocol by (36), detailed by (37). Cells were observed using a Zeiss Axioplan 2 fluorescence microscope (200× magnification) fitted with a Sony AVT-Horn digital 3 CCD color video camera.
Fish spleen samples (four per group) were harvested and preserved in 10% neutral buffered formalin for 24 hours. The samples were then subjected to dehydration using an ascending series of alcohols, followed by clearing in methyl benzoate through three changes. The tissues were subsequently embedded in hard wax. Sections were cut at 5 µm, dried, and stained using Harris’s hematoxylin and eosin (H&E) to visualize tissue structure (38, 39). Masson’s trichrome staining (40) was employed to identify tissue fibrosis. Selected high-quality slides were examined and photographed utilizing a BX50F4 microscope from Olympus Optical Co., Ltd. (Tokyo, Japan).
In comparison with the control group, exposure to both doses of DCF significantly decreased RBC count and Hb content equipotently, with the low dose causing a more pronounced reduction in PCV% than the high dose. Dietary intervention with Chlorella significantly normalized RBC count and increased PCV% equally, without altering Hb content. No significant changes were observed in MCV, MCH, and MCHC across all groups. The DCF1 group showed a significant reduction in platelet count compared to the control group, while the DCF2 group did not show significant changes. Chlorella supplementation did not significantly improve platelet count.
WBC count in Clarias gariepinus significantly reduced following exposure to DCF, with no significant difference between the DCF1 and DCF2 groups. Chlorella did not improve WBC count at the studied doses. The low dose of DCF induced a greater reduction in large lymphocytes% than the high dose, but Chlorella administration in the Chlorella+DCF1 group successfully restored large lymphocytes% to normal levels. DCF intoxication caused a dose-dependent reduction in small lymphocytes%, which increased equally in the Chlorella+DS groups but remained below control levels. Neutrophil% similarly increased in DCF groups, with Chlorella failing to correct this abnormality. Only the high dose of DCF reduced monocyte%. Clarias gariepinus exposed to DCF exhibited eosinophilia, which was normalized by Chlorella supplementation in both groups equally.
Phagocytic index and lysozyme activity decreased equally in DCF groups and further decreased in supplemented groups. IL-6 levels increased dose-dependently following DCF exposure, with a significant increase occurring after dietary inclusion of Chlorella, and the Chlorella+DCF2 group showing higher IL-6 levels than the Chlorella+DCF1 group (Table 1).
Table 1. Effects of C. vulgaris on the hemato-immunological parameters in diclofenac sodium intoxicated-Clarias gariepinus.
A dosage-related rise in the percentage of erythrocyte morphological alterations was observed in the DCF groups compared to the control. However, a significant reduction in poikilocytosis was noted in the microalgae-supplemented groups compared to their respective DCF groups. Chlorella intake was equally effective in both groups in reducing erythrocyte abnormalities (Figure 1).
Figure 1. Represented blood smears from of C. gariepinus showing (a) the normal erythrocytes, (b) the deformed ones after exposure to DCF1, (c) the deformed ones after exposure to DCF2, (d) the deformed ones after exposure to Chorella+ DCF1, (e) the deformed ones after exposure to Chorella+ DCF2, and (f) The percentage of cell alteration and nuclear abnormalities of RBCs in C. gariepinus exposed to the effects of different doses of DCF for 14 days; Er, normal erythrocytes; Sch, schistocytic; Cr, crenated cell; Ac, acanthocyte; Va, Vacuolated cells; Hyc, hymolyzed cells (H & E stain, scale bar: 100 μm). Bars represent means ± SE of 6 fish/group. Different letters indicate significant differences among treatments (p < 0.05).
Compared to the control group, the DCF2 group showed a higher increase in apoptotic RBCs than the DCF1 group. However, the percentage of apoptotic cells decreased with Chlorella administration, with the Chlorella+DCF1 group exhibiting fewer apoptotic cells than the Chlorella+DCF2 group (Figure 2).
Figure 2. Apoptosis detection in red blood cells of C. gariepinus showing (a) control, (b) the apoptosis detection after exposure to DCF1, (c) apoptosis detection after exposure to DCF2, (d) apoptosis detection after exposure to Chorella+ DCF1, (e) apoptosis detection after exposure to Chorella+ DCF2, and (f) The percentage of apoptosis of RBCs in C. gariepinus exposed to the effects of different doses of DCF for 14 days; Bars represent means ± SE of 6 fish/group. Different letters indicate significant differences among treatments (p < 0.05).
Histopathological alterations observed were as follows: control groups exhibited well-defined splenic structures with distinct white pulp (WP) and red pulp (RP). The WP contained lymphocytes, few red blood cells, and dispersed macrophages, while the RP was composed mainly of hematopoietic tissues with predominantly red blood cells and a few mononuclear cells (Figure 3A). In fish exposed to DCF1, the WP appeared shrunken with sparse lymphocytes, and the RP showed enlargement with various hematopoietic cells and mixed coloration from yellow to black (Figure 3B). Amelioration was noted in fish exposed to Chlorella+DCF1, with the WP largely restored to control-like conditions, accompanied by edema (E) and an RP with hematopoietic cells, dilated blood vessels, and small macrophage centers (MMC) (Figure 3C). Fish exposed to DCF2 showed WP with lymphocyte foci and MMC in yellow coloration, a reduced red pulp boundary with few hematopoietic tissues, and the presence of ellipsoid bodies and dilated blood vessels (DBV) (Figure 3D). In contrast, fish exposed to Chlorella+DCF2 displayed clear separation between WP and RP, widened RP, restored WP size with numerous lymphocytes, some edema (E), and many small macrophage cells (MC) (Figure 3E).
Figure 3. Transverse section of fish spleen satin’s with (H&E) in all experimental groups. (A) Control, (B) fish exposed to DS1, (C) Fish exposed to DS1 + Chlorella, (D) Fish exposed to DS2, and (E) Fish exposed to DS2 + Chlorella. Notice: white pulp (WP), red pulp (RP) melanomacrophage cell (MC), melanomacrophage centers (MMC), ellipsoid bodies (EB), Edema (E), dilated blood vessel (DBV). X-400, Scale Bar= 50µm.
Masson trichrome staining revealed the following: Control groups exhibited a normal distribution of collagen fibers around the sheaths of ellipsoid bodies (EB) and fine fibers in the ground substance (Black arrow) (Figure 4A). In fish exposed to DCF1, there was an increased intensity of collagen fibers compared to the control, with a greater number of ellipsoid bodies and more background collagen than in the DCF2 group (Figures 4B, D). In the bioremediated groups, specifically those exposed to Chlorella+DCF2, a decrease in collagen fiber intensity was observed compared to DCF1 and DCF2, with the most significant reduction in Chlorella+DCF2 compared to Chlorella+DCF1 (Figures 4C, E).
Figure 4. Transverse section of spleen of all experimental groups stained by Massion trichome stain for collagen fibers. (A) Control, (B) fish exposed to DS1, (C) Fish exposed to DS1 + Chlorella, (D) Fish exposed to DS2, and (E) Fish exposed to DS2 + Chlorella. Noticed: elebsoide bodes (EB), Ground substance (black arrow). X-400, Scale Bar= 50µm.
The reduction in erythrocyte number, Hb content and PCV percent in DCF exposed Clarias gariepinus is corresponding to that observed by (18) resulting from restriction in erythrocyte production by inducing DNA damage in head kidney (41), as well as dysfunctional osmoregulatory processes in the gill epithelium linked to toxicant deposition (42, 43). The lipophilic properties of negatively charged DCF, along with its molecular composition featuring benzene and chloride residues, enhance its accumulation in non-intended organism. These properties of DCF increase its interaction with fatty acids and various polar groups in the membrane bilayer, leading to its absorption on the membrane surface and making it more likely to cause hemolysis (11). Other contradictory data suggested a marked raise in RBC count in South American catfish (Rhamdia quelen) (19). However, the experimental setup differed from ours, as fish were exposed to the tested compound dissolved in water for a short duration of 96 hours. We believe that with long-term exposure in Clarias gariepinus, the adaptation mechanisms are insufficient to cope with erythrocytic alterations. The decrease in hemoglobin levels could be attributed to hemodilution, alongside the fish’s efforts to lower the concentration of circulating toxic substances in the bloodstream (44). The reduction in RBC count and hemoglobin content diminishes the blood’s ability to deliver oxygen, leading to tissue hypoxia (45). The proportional decrease in both RBC count and hemoglobin concentration results in no significant change in MCH. The lack of significant difference regarding MCV, MCHC, and MCHC is in line with (46) and (47). The addition of Chlorella to ration formulation of DCF-exposed fish improved RBCs, Hb, and PCV in harmony with the findings in polyethylene microplastic-fed Clarias gariepinus (26). Chlorella is a rich source of omega-3-polyunsaturated fatty acids which maintains the integrity of the membrane in RBCs when exposed to oxidative damage, and reduces lipid peroxidation (48). This microalga is abundant in iron (49) and folic acid (50), both of which play a significant role in erythropoiesis. Additionally, it contains antioxidants, carotenoids, and phycocyanin, all of which contribute to enhanced RBC production (23).Consistent with our results, Ribas et al. (51) observed a decrease in leukocyte counts in wolf fish (Hoplias malabaricus) exposed to DCF, attributing this reduction to the drug’s immunosuppressive effect on WBCs. Suppression of cell growth and multiplication (52) and acceleration of cell suicidal decisions by oxidative stress-induced genotoxic damage (53) could underlie the drop in WBC populations. Chlorella administration effectively restored WBC count in the same line as that observed following fishmeal replacement with Chlorella vulgaris in Clarias gariepinus (54), owing to presence of β-1,3-glucan (55) and carotenoid (56).
Despite the overall reduction of total WBC count as reported in the present study, granulocytes populations showed significant elevation following DCF exposure. This could be attributed to the anti-inflammatory reaction exerted by NSAIDs as previously described (57). Meloxicam, a cyclooxygenase-2 inhibitor NSAID, was reported to stimulates the endogenous production of granulocyte colony-stimulating factor (58) and eliminates the negative feedback control exerted by prostaglandins on the production of hematopoietic progenitor cells (59).
Obviously, the overall reduction of total leucocytic count in DCF exposed fish was due to the marked lymphopenia and monocytopenia reported in the present study. Similar findings were reported after long-term dietary inclusion of DCF in common carp (Cyprinus carpio) (60). This outcome was attributed to the stressful condition eliciting secretion of cortisol that shortens the life of lymphocytes, promoting the apoptosis and reducing their proliferation (61). Moreover, cortisol was reported to block the release of lymphocytes from cortical sinus of lymph nodes to peripheral circulation (62).
The reduction in monocyte percent following exposure with high dose of DCF is in consistent with that noticed in Rhamdia quelen exposed to ibuprofen, another NSAID (63). DNA damage was observed in the monocytic cell lineage of wolf fish (Hoplias malabaricus) following exposure to DCF (41). Monocytes play a crucial role in phagocytosing pathogens, which is vital for innate immunity (64), while lymphocytes and thrombocytes are key components of the innate immune response (64, 65). Thrombocytes also exhibit phagocytic activity (66). A reduction in the number of immune cells leaves fish more susceptible to diseases by impairing their ability to fight off infections (63).
The improvement in hematological parameters following Chlorella administration is corresponding to what happen in Oreochromis niloticus subjected to non-lethal doses concentrations of penoxsulam herbicide (67).
Lysozymes are key defense mediators in the fish’s natural immune defense against contaminants, and they act as shield against oxidative burden (68). A decrease in phagocytic activity typically leads to a significant reduction in lysozymes levels (69). Depressed lysozymes level is similar to brown trout (Salmo trutta f. fario) when subjected to water-borne DCF (57). In the green mussel (Perna viridis), another aquatic model species, exposure to carbamazepine suppressed lysozyme activity (70). This outcome could be due to downregulation in lysozymes as that occurred in after exposure of gilthead sea bream (Sparus aurata L.) to a combination of contaminants, namely carbamazepine, cadmium chloride and polybrominated diphenyl ether (71). Ibuprofen at environmentally realistic concentration decreased serum lysozyme activity and mRNA expression of IL-6 in juvenile grass carp (Ctenopharyngodon Idella) (72). Actually, increased IL-6 in intoxicated groups points to compensatory mechanism in attempt to overcome the toxic effects of DFC as it increased the expression of immuno-related genes and improved the survival rate of the spleen tissue (73) driving lymphocyte differentiation (74) and induction of antimicrobial peptides, promotes macrophage growth and proliferation (75). The improvement in lysozyme activity in Chlorella+DCF groups is noted previously in Oreochromis niloticus fed Chlorella-enriched diet (24). Numerous components of Chlorella meal have been shown to boost immune responses, including polysaccharides (76), d-lactic acid (77), and water-soluble α-glucans (78).Mutated erythrocyte membranes due to environmental contamination can have harmful consequences on the permeability of membrane, by hindering the free flow of erythrocytes through the blood capillaries, and impairing the blood oxygen-delivering potential (39). Increased proteolytic activity in the erythrocytic membrane (79), deformation in the sub-membrane cytoskeleton, an elevated membrane cholesterol/phospholipid ratio (80), and insufficient repair of oxidant-injured erythrocyte phospholipids (81) are key factors contributing to the formation of acanthocytes. Due to their abnormal morphology, acanthocytes are prone to splenic capturing and eradication, resulting in erythrocytopenia (82). The presence of vacuolation in RBCs points to damage of their internal components (83). This histological event occurs as a prevalent trait that coincides with cell death caused by ischemic and osmolar stress, or toxicity with heavy metals or pharmaceutical agents (39, 84, 85). The existence of schistocytes presumably originates from the inherent mechanical fragility of red blood cells instead of vascular alterations (86). The crenated RBCs seem to be an outcome of mitochondrial DNA binding (87). Crenation may hasten RBC elimination and recycling, likely resulting in erythrocytopenia. Crenated RBCs may also be involved in inflammation, organ dysfunction, tissue ischemia, and vascular obstruction (88). This type of cellular distortion was reported in cases of intoxication with specific heavy metals and pesticides (39). Chlorella markedly alleviate the poikilocytosis, as seen in Clarias gariepinus intoxicated with polyethylene microplastics (26), thanks to its abundance of cytoprotective factors (89, 90).
The genotoxicity in RBCs triggered by DCF is in parallel with chromosomal injury in the hepatocytes of Oreochromis niloticus (46). Deposition of drug metabolites in the nucleus of the cells led to DNA damage and chromosomal aberrations (91). Liu et al. (92) noted that DCF caused replication blockage leading to chromosomal aberrations as well as translesion DNA synthesis in fishes (41). DCF has been shown to induce oxidative stress by increasing the production of reactive oxidants like hydroxyl radicals, leading to the formation of 8-hydroxydeoxyguanosine, which alters nucleoside and DNA structure (93). Reactive oxidants can electrophilically attack the nucleophilic groups of deoxyribose and nitrogenous bases in DNA molecules, resulting in structural damage to the DNA (46).Chlorella opposed the apoptotic signals in myocytes and hepatocytes by increasing gene expression of anti-apoptotic genes, such as b cell lymphoma-2 and myeloid cell leukemia-1, and decreasing gene expression of pro-apoptotic genes, including bcl2-associated x and caspase 3 (94, 95).
Fish spleen encompasses groups of immune cell population such as lymphocytes, macrophages and dendritic cells promoting potent immune responses near the location of antigen invasion (96). It operates as a main secondary immune organ, and has a central role in the antigen presentation and commencement of the adaptive immune response (97). Although the spleen’s role in immune system control is well recognized, its histoarchitecture have been relatively understudied. Therefore, our study aimed to investigate the histological abnormalities in the spleen of Clarias gariepinus following DCF exposure and to assess the potential improvements with Chlorella supplementation.
MMCs have the ability to clear foreign materials from the bloodstream (98) and can serve as a potential biomarker for pollutant load (99). The observed enlargement of MMCs in the spleen of the DCF groups suggests ecotoxicological effects and exposure to xenobiotics (100, 101). This enlargement may be attributed to the recruitment of additional macrophages in response to reactive oxygen species generated at sites of tissue damage (102). Additionally, it may be explained by an accelerated degradation rate of mutagenic erythrocytes (101).
The increased collagen fiber density observed in the splenic tissues of the DCF groups resembles hepatic fibrosis seen in Gilthead seabream (Sparus aurata) following exposure to erythromycin and oxytetracycline (103), and may be linked to the upregulation of pro-fibrotic mediators (104). Oxidative stress from contaminants can activate fibroblasts to produce extracellular matrix proteins, leading to fibrotic tissue formation (105). Additionally, DCF enhances hepatic lactate production (106), which stimulates pro-fibrogenic cytokines, resulting in fibroblast differentiation (107).
Chlorella mitigated the histological lesions in the spleen, similar to the effects observed in Oreochromis niloticus experimentally infected with Aeromonas hydrophila (108), likely due to its immuno-competent properties (67). It hindered tissue fibrosis by improving redox homeostasis and down-regulating the transforming growth factor-beta signaling pathway (109).
C. vulgaris supplementation in Clarias gariepinus exposed to DCF counteracted poikilocytosis and reduced the percentage of apoptotic RBCs, together with improving immuno-hematological parameters. Moreover, it mitigated the histological lesions induced by DCF in spleen and exhibited an antifibrotic effect. Incorporating Chlorella into fish feed could be beneficial for mitigating the toxicological impacts of pharmaceutical compounds discharged into aquatic medium. For future research, it would be advantageous to examine the effects of extended exposures to various NSAIDs and their mixtures, to better mimic actual conditions in aquatic habitats. Additionally, a comprehensive study of the effects of drug metabolites is warranted, as some toxicological studies have shown that the metabolites may exhibit greater toxicity than the original compounds. Extending the exposure duration would allow for better comparisons of toxicity at various developmental phases, especially if sampling is done consistently throughout the study. Additionally, comparing the effects of exposure through feed versus water would provide valuable insights. Further studies on other microalgae species following NSAID exposure also deserves special attention.
The raw data supporting the conclusions of this article will be made available by the authors, without undue reservation.
The animal studies were approved by Research Ethics Committee of the Molecular Biology Research and Studies Institute. The studies were conducted in accordance with the local legislation and institutional requirements. Written informed consent was obtained from the owners for the participation of their animals in this study.
AG: Conceptualization, Investigation, Methodology, Resources, Writing – original draft, Writing – review & editing. AM: Conceptualization, Supervision, Writing – original draft, Writing – review & editing. NA: Methodology, Writing – original draft, Writing – review & editing. AS: Conceptualization, Investigation, Methodology, Resources, Software, Supervision, Writing – original draft, Writing – review & editing.
The author(s) declare that no financial support was received for the research and/or publication of this article.
The authors declare that the research was conducted in the absence of any commercial or financial relationships that could be construed as a potential conflict of interest.
The author(s) declare that no Generative AI was used in the creation of this manuscript.
All claims expressed in this article are solely those of the authors and do not necessarily represent those of their affiliated organizations, or those of the publisher, the editors and the reviewers. Any product that may be evaluated in this article, or claim that may be made by its manufacturer, is not guaranteed or endorsed by the publisher.
1. Corcoran J, Winter JM, Tyler RC. Pharmaceuticals in the aquatic environment: a critical review of the evidence for health effects in fish. Crit Rev Toxicol. (2010) 40:287–304. doi: 10.3109/10408440903373590
2. Williams RT, Cook JC. Exposure to pharmaceuticals present in the environment. Ther Innov Regul Sci. (2007) 41:133–41. doi: 10.1177/009286150704100202
3. Bound JP, Voulvoulis N. Household disposal of pharmaceuticals as a pathway for aquatic contamination in the United Kingdom. Environ Health Perspect. (2005) 113:1705–11. doi: 10.1289/ehp.8315
4. Fent K, Weston A, Caminada D. Ecotoxicology of human pharmaceuticals. Aquat Toxicol. (2006) 76:122–59. doi: 10.1016/j.aquatox.2005.09.009
5. Loos R, Gawlik BM, Locoro G, Rimaviciute E, Contini S, Bidoglio G. EU-wide survey of polar organic persistent pollutants in European river waters. Environ pollut. (2009) 157:561–8. doi: 10.1016/j.envpol.2008.09.020
6. Ogunwole GA, Saliu JK, Osuala FI, Odunjo FO. Chronic levels of ibuprofen induces haematoxic and histopathology damage in the gills, liver, and kidney of the African sharptooth catfish (Clarias gariepinus). Environ Sci pollut Res. (2021) 28:25603–13. doi: 10.1007/s11356-020-12286-7
7. SRU (Sachverständigenrat für Umweltfragen). “Arzneimittel in der umwelt,” in Aktuelle stellungnahme. (2007).
8. Sari S, Ozdemir G, Yangin-Gomec C, Zengin GE, Topuz E, Aydin E, et al. Seasonal variation of diclofenac concentration and its relation with wastewater characteristics at two municipal wastewater treatment plants in Turkey. J Hazard Mater. (2014) 272:155–64. doi: 10.1016/j.jhazmat.2014.03.015
9. Oosterhuis M, Sacher F, Ter Laak TL. Prediction of concentration levels of metformin and other high consumption pharmaceuticals in wastewater and regional surface water based on sales data. Sci Total Environ. (2013) 442:380–8. doi: 10.1016/j.scitotenv.2012.10.046
10. Awofisayo O, Awofisayo O, Iferi I, Akpan O. The pattern of sale and use of non-steroidal anti-inflammatory drugs in rural and urban centres in Nigeria. Trop J Pharm Res. (2008) 7:1013–8. doi: 10.4314/tjpr.v7i3.14685
11. Toufexi E, Dailianis S, Vlastos D, Manariotis ID. Mediated effect of ultrasound treated Diclofenac on mussel hemocytes: First evidence for the involvement of respiratory burst enzymes in the induction of DCF-mediated unspecific mode of action. Aquat Toxicol. (2016) 175:144–53. doi: 10.1016/j.aquatox.2016.03.017
12. Vieno N, Sillanpää M. Fate of diclofenac in municipal wastewater treatment plant—A review. Environ Int. (2014) 69:28–39. doi: 10.1016/j.envint.2014.03.021
13. Zhang YongJun ZY, Geissen S, Gal C. Carbamazepine and diclofenac: removal in wastewater treatment plants and occurrence in water bodies. Chemosphere. (2008) 73:1151–61. doi: 10.1016/j.chemosphere.2008.07.086
14. Adewumi A, Olaleye V. Catfish culture in Nigeria: progress, prospects and problems. Afr J Agric Res. (2011) 6:1281–5. doi: 10.5897/AJAR09.361
15. Nwani C, Ibiam U, Ibiam O, Nworie O, Onyishi G, Atama C. Investigation on acute toxicity and behavioral changes in Tilapia zillii due to glyphosate-based herbicide, Forceup. J Anim Plant Sci. (2013) 23:888–92.
16. Barboza LGA, Lopes C, Oliveira P, Bessa F, Otero V, Henriques B, et al. Microplastics in wild fish from North East Atlantic Ocean and its potential for causing neurotoxic effects, lipid oxidative damage, and human health risks associated with ingestion exposure. Sci Total Environ. (2020) 717:134625. doi: 10.1016/j.scitotenv.2019.134625
17. Sayed AEDH, Khalil NSA, Alghriany AA, Abdel-Ghaffar SK, Hussein AA. Prefeeding of Clarias gariepinus with Spirulina platensis counteracts petroleum hydrocarbons-induced hepato-and nephrotoxicity. Sci Rep. (2024) 14:7219. doi: 10.1038/s41598-024-57420-4
18. Ajima M, Ogo O, Audu B, Ugwoegbu K. Chronic diclofenac (DCF) exposure alters both enzymatic and haematological profile of African catfish, Clarias gariepinus. Drug Chem Toxicol. (2015) 38:383–90. doi: 10.3109/01480545.2014.974108
19. Ghelfi A, Ribas JLC, Guiloski IC, Bettim FL, Piancini LDS, Cestari MM, et al. Evaluation of biochemical, genetic and hematological biomarkers in a commercial catfish Rhamdia quelen exposed to diclofenac. Bull Environ Contam Toxicol. (2016) 96:49–54. doi: 10.1007/s00128-015-1693-3
20. Guiloski IC, Piancini LDS, Dagostim AC, de Morais Calado SL, Fávaro LF, Boschen SL, et al. Effects of environmentally relevant concentrations of the anti-inflammatory drug diclofenac in freshwater fish Rhamdia quelen. Ecotoxicol Environ Saf. (2017) 139:291–300. doi: 10.1016/j.ecoenv.2017.01.053
21. McRae NK, Glover CN, Burket SR, Brooks BW, Gaw S. Acute exposure to an environmentally relevant concentration of diclofenac elicits oxidative stress in the culturally important galaxiid fish Galaxias maculatus. Environ Toxicol Chem. (2018) 37:224–35. doi: 10.1002/etc.3948
22. Morais M, Vaz B, Morais E, Costa JA. Biologically active metabolites synthesized by microalgae. BioMed Res Int. (2015) 2015:835761. doi: 10.1155/2015/835761
23. Rahimnejad S, Lee SM, Park HG, Choi J. Effects of dietary inclusion of Chlorella vulgaris on growth, blood biochemical parameters, and antioxidant enzyme activity in olive flounder, Paralichthys olivaceus. J World Aquac Soc. (2017) 48:103–12. doi: 10.1111/jwas.12320
24. Mahmoud EA, El-Sayed BM, Mahsoub YH, Neamat-Allah AN. Effect of Chlorella vulgaris enriched diet on growth performance, hemato-immunological responses, antioxidant and transcriptomics profile disorders caused by deltamethrin toxicity in Nile tilapia (Oreochromis niloticus). Fish Shellfish Immunol. (2020) 102:422–9. doi: 10.1016/j.fsi.2020.04.061
25. Abdelhamid FM, Elshopakey GE, Aziza AE. Ameliorative effects of dietary Chlorella vulgaris and β-glucan against diazinon-induced toxicity in Nile tilapia (Oreochromis niloticus). Fish Shellfish Immunol. (2020) 96:213–22. doi: 10.1016/j.fsi.2019.12.009
26. Sayed AEDH, Hamed M, Badrey AE, Soliman HA. Bioremediation of hemotoxic and oxidative stress induced by polyethylene microplastic in Clarias gariepinus using lycopene, citric acid, and chlorella. Comp Biochem Physiol C Toxicol Pharmacol. (2021) 250:109189. doi: 10.1016/j.cbpc.2021.109189
27. Wilson B, Gracey F, Evan J, Bateman A. Neuropsychological Rehabilitation: Theory, Models, Therapy and Outcome. UK: Cambridge University Press (2009).
29. Hesser EF. Methods for routine fish hematology. Prog Fish-Cult (1960) 22, 164–171. doi: 10.1577/1548-8659(1960)22[164:MFRFH]2.0.CO;2
30. Lee GR, Foerster J, Lukens J, Paraskevas F, Greer JP, Rodgers GM. Wintrobe's clinical hematology. Bethseda: Lippincort Williams and Wilkins (1999).
31. Parry RM Jr., Chandan RC, Shahani KM. A rapid and sensitive assay of muramidase. Proc Soc Exp Biol Med. (1965) 119:384–6. doi: 10.3181/00379727-119-30188
32. Siwicki AK, Anderson DP, Rumsey GL. Dietary intake of immunostimulants by rainbow trout affects non-specific immunity and protection against furunculosis. Vet Immunol Immunopathol. (1994) 41:125–39. doi: 10.1016/0165-2427(94)90062-0
33. Hanington PC, Belosevic M. Interleukin-6 family cytokine M17 induces differentiation and nitric oxide response of goldfish (Carassius auratus L.) macrophages. Dev Comp Immunol. (2007) 31:817–29. doi: 10.1016/j.dci.2006.12.001
34. Al-Sabti K, Metcalfe CD. Fish micronuclei for assessing genotoxicity in water. Mutat Res Genet Toxicol. (1995) 343:121–35. doi: 10.1016/0165-1218(95)90078-0
36. Darzynkiewicz Z. Differential staining of dna and rna in intact cells and isolated cell nuclei with acridine orange. Methods Cell Biol. (1990) 33:285–98. doi: 10.1016/s0091-679x(08)60532-4
37. Sayed AEDH, Mahmoud UM, Mekkawy IA. Erythrocytes alterations of monosex tilapia (Oreochromis niloticus, Linnaeus 1758) produced using methyltestosterone. Egypt J Aquat Res. (2016) 42:83–90. doi: 10.1016/j.ejar.2015.10.004
38. Bancroft JD, Gamble M. Theory and Practice of Histological Techniques. China: Elsevier health sciences (2008).
39. Sawhney A, Johal M. Erythrocyte alterations induced by malathion in Channa punctatus (Bloch). Bull Environ Contam Toxicol. (2000) 64:398–405. doi: 10.1007/s001280000014
40. Bancroft J J, Stevens A. Theory and practice of histological techniques. 2nd (London: Churchill-Livingston) (1982), 131–135.
41. Ribas JLC, da Silva CA, de Andrade L, Galvan GL, Cestari MM, Trindade ES, et al. Effects of anti-inflammatory drugs in primary kidney cell culture of a freshwater fish. Fish Shellfish Immunol. (2014) 40:296–303. doi: 10.1016/j.fsi.2014.07.009
42. Pereira H, Ferrier S, Walters M, Geller G, Scholes R, Bruford M, et al. Essential biodiversity variables. Science. (2013) 339:277–8. doi: 10.1126/science.122993
43. Saravanan S, Geurden I, Figueiredo-Silva AC, Kaushik SJ, Haidar MN, Verreth JAJ, et al. Control of voluntary feed intake in fish: a role for dietary oxygen demand in Nile tilapia (Oreochromis niloticus) fed diets with different macronutrient profiles. . Br J Nutr. (2012) 108:1519–29. doi: 10.1017/S0007114511006842
44. Takasusuki J, Araujo M, Fernandes M. Effect of water pH on copper toxicity in the neotropical fish, Prochilodus scrofa (Prochilodondidae). Bull Environ Contam Toxicol. (2004) 72:1075–82. doi: 10.1007/s00128-004-0353-9
45. Hamed M, Martyniuk CJ, Said RE, Soliman HA, Badrey AE, Hassan EA, et al. Exposure to pyrogallol impacts the hemato-biochemical endpoints in catfish (Clarias gariepinus). Environ pollut. (2023) 333:122074. doi: 10.1016/j.envpol.2023.122074
46. Pandey PK, Ajima MN, Kumar K, Poojary N, Kumar S. Evaluation of DNA damage and physiological responses in Nile tilapia, Oreochromis niloticus (Linnaeus 1758) exposed to sub-lethal diclofenac (DCF). Aquat Toxicol. (2017) 186:205–14. doi: 10.1016/j.aquatox.2017.03.007
47. Ogueji E, Nwani C, Iheanacho S, Mbah C, Okeke C, Yaji A. Acute toxicity effects of ibuprofen on behaviour and haematological parameters of African catfish Clarias gariepinus (Burchell 1822). Afr J Aquat Sci. (2018) 43:293–303. doi: 10.2989/16085914.2018.1465393
48. Pal M, Ghosh M. Prophylactic effect of α-linolenic acid and α-eleostearic acid against MeHg induced oxidative stress, DNA damage and structural changes in RBC membrane. Food Chem Toxicol. (2012) 50:2811–8. doi: 10.1016/j.fct.2012.05.038
49. Yeganeh S, Teimouri M, Amirkolaie AK. Dietary effects of Spirulina platensis on hematological and serum biochemical parameters of rainbow trout (Oncorhynchus mykiss). Res J Vet Sci. (2015) 101:84–8. doi: 10.1016/j.rvsc.2015.06.002
50. Khani B, Mardanian F, Fesharaki SJ. Omega-3 supplementation effects on polycystic ovary syndrome symptoms and metabolic syndrome. J Res Med Sci. (2017) 22, 64. doi: 10.4103/jrms.JRMS_644_16
51. Ribas JLC, Zampronio AR, Silva De Assis HC. Effects of trophic exposure to diclofenac and dexamethasone on hematological parameters and immune response in freshwater fish. Environ Toxicol Chem. (2016) 35:975–82. doi: 10.1002/etc.3240
52. Nemcova M, Pikula J, Zukal J, Seidlova V. Diclofenac-induced cytotoxicity in cultured carp leukocytes. Physiol Res. (2020) 69:S607. doi: 10.33549/physiolres.934609
53. Krüger K, Mooren FC. Exercise-induced leukocyte apoptosis. Exerc. Immunol Rev. (2014) 20:117–34.
54. Raji AA, Alaba PA, Yusuf H, Bakar NHA, Taufek NM, Muin H, et al. Fishmeal replacement with Spirulina Platensis and Chlorella vulgaris in African catfish (Clarias gariepinus) diet: Effect on antioxidant enzyme activities and haematological parameters. Res J Vet Sci. (2018) 119:67–75. doi: 10.1016/j.rvsc.2018.05.013
55. Koch JFA, de Oliveira CAF, Zanuzzo FS. Dietary β-glucan (MacroGard®) improves innate immune responses and disease resistance in Nile tilapia regardless of the administration period. Fish Shellfish Immunol. (2021) 112:56–63. doi: 10.1016/j.fsi.2021.02.014
56. Liu F, Qu YK, Wang AM, Yu YB, Yang WP, Lv F, et al. Effects of carotenoids on the growth performance, biochemical parameters, immune responses and disease resistance of yellow catfish (Pelteobagrus fulvidraco) under high-temperature stress. Aquaculture. (2019) 503:293–303. doi: 10.1016/j.aquaculture.2019.01.008
57. Hoeger B, Köllner B, Dietrich DR, Hitzfeld B. Water-borne diclofenac affects kidney and gill integrity and selected immune parameters in brown trout (Salmo trutta f. fario). Aquat Toxicol. (2005) 75:53–64. doi: 10.1016/j.aquatox.2005.07.006
58. Hofer M, Pospíšil M, Dušek L, Hoferová Z, Weiterová L. A single dose of an inhibitor of cyclooxygenase 2, meloxicam, administered shortly after irradiation increases survival of lethally irradiated mice. Radiat Res. (2011) 176:269–72. doi: 10.1667/rr2614.1
59. Hofer M, Hoferová Z, Falk M. Brief story on prostaglandins, inhibitors of their synthesis, hematopoiesis, and acute radiation syndrome. Molecules. (2019) 24, 4019. doi: 10.3390/molecules24224019
60. Mikula P, Hollerova A, Hodkovicova N, Doubkova V, Marsalek P, Franc A, et al. Long-term dietary exposure to the non-steroidal anti-inflammatory drugs diclofenac and ibuprofen can affect the physiology of common carp (Cyprinus carpio) on multiple levels, even at “environmentally relevant” concentrations. Sci Total Environ. (2024) 917:170296. doi: 10.1016/j.scitotenv.2024.170296
61. Weyts FAA, Cohen N, Flik G, Verburg-van-Kemenade BML. Interactions between the immune system and the hypothalamo-pituitary-interrenal axis in fish. Fish Shellfish Immunol. (1999) 9:1–20. doi: 10.1006/fsim.1998.0170
62. Coutinho AE, Chapman KE. The anti-inflammatory and immunosuppressive effects of glucocorticoids, recent developments and mechanistic insights. Mol Cell Endocrinol. (2011) 335:2–13. doi: 10.1016/j.mce.2010.04.005
63. Mathias FT, Fockink DH, Disner GR, Prodocimo V, Ribas JLC, Ramos LP, et al. Effects of low concentrations of ibuprofen on freshwater fish Rhamdia quelen. Environ Toxicol Pharmacol. (2018) 59:105–13. doi: 10.1016/j.etap.2018.03.008
64. Ferdous F, Scott T. A comparative examination of thrombocyte/platelet immunity. Immunol Lett. (2015) 163:32–9. doi: 10.1016/j.imlet.2014.11.010
65. Fischer K, Voelkl S, Berger J, Andreesen R, Pomorski T, Mackensen A. Antigen recognition induces phosphatidylserine exposure on the cell surface of human CD8+ T cells. Blood. (2006) 108:4094–101. doi: 10.1182/blood-2006-03-011742
66. Nagasawa T, Somamoto T, Nakao M. Carp thrombocyte phagocytosis requires activation factors secreted from other leukocytes. Dev Comp Immunol. (2015) 52:107–11. doi: 10.1016/j.dci.2015.05.002
67. Galal AA, Reda RM, Mohamed AAR. Influences of Chlorella vulgaris dietary supplementation on growth performance, hematology, immune response and disease resistance in Oreochromis niloticus exposed to sub-lethal concentrations of penoxsulam herbicide. Fish Shellfish Immunol. (2018) 77:445–56. doi: 10.1016/j.fsi.2018.04.011
68. Saurabh S, Sahoo P. Lysozyme: an important defence molecule of fish innate immune system. Aquac Res. (2008) 39:223–39. doi: 10.1111/j.1365-2109.2007.01883.x
69. Khalil SR, Abd Elhakim Y, Abd-El-fattah AH, Farag MR, Abd-El-Hameed NE, Abd Elhakeem EM. Dual immunological and oxidative responses in Oreochromis niloticus fish exposed to lambda cyhalothrin and concurrently fed with Thyme powder (Thymus vulgaris L.): stress and immune encoding gene expression. Fish Shellfish Immunol. (2020) 100:208–18. doi: 10.1016/j.fsi.2020.03.009
70. Juhel G, Bayen S, Goh C, Lee WK, Kelly BC. Use of a suite of biomarkers to assess the effects of carbamazepine, bisphenol A, atrazine, and their mixtures on green mussels, Perna viridis. Environ Toxicol Chem. (2017) 36:429–41. doi: 10.1002/etc.3556
71. Espinosa-Ruiz C, Manuguerra S, Morghese M, García-Beltrán JM, Esteban MÁ, Giuga M, et al. Immunity and inflammatory responses in gilthead sea bream (Sparus aurata L.) exposed to sub-lethal mixture of carbamazepine, cadmium chloride and polybrominated diphenyl ether. Fish Shellfish Immuno. (2021) 111:25–35. doi: 10.1016/j.fsi.2020.12.013
72. Zhang N, Liu X, Pan L, Zhou X, Zhao L, Mou X, et al. Evaluation of ibuprofen contamination in local urban rivers and its effects on immune parameters of juvenile grass carp. Fish Physiol Biochem. (2021) 47:1405–13. doi: 10.1007/s10695-021-00987-w
73. Wang X, Chen J, Zhang R, Liu L, Ma G, Zhu H. Interleukin-6 in Siberian sturgeon (Acipenser baeri): Molecular characterization and immune functional activity. Fish Shellfish Immunol. (2020) 102:296–306. doi: 10.1016/j.fsi.2020.03.023
74. Chen HH, Lin HT, Foung YF, Lin JHY. The bioactivity of teleost IL-6: IL-6 protein in orange-spotted grouper (Epinephelus coioides) induces Th2 cell differentiation pathway and antibody production. Dev Comp Immunol. (2012) 38:285–94. doi: 10.1016/j.dci.2012.06.013
75. Costa MM, Maehr T, Diaz-Rosales P, Secombes CJ, Wang T. Bioactivity studies of rainbow trout (Oncorhynchus mykiss) interleukin-6: effects on macrophage growth and antimicrobial peptide gene expression. Mol Immunol. (2011) 48:1903–16. doi: 10.1016/j.molimm.2011.05.027
76. Hsu HY, Jeyashoke N, Yeh CH, Song YJ, Hua KF, Chao LK. Immunostimulatory bioactivity of algal polysaccharides from Chlorella pyrenoidosa activates macrophages via Toll-like receptor 4. J Agric Food Chem. (2010) 58:927–36. doi: 10.1021/jf902952z
77. Lee SM, Kim SK, Lee N, Ahn CY, Ryu CM. d-Lactic acid secreted by Chlorella fusca primes pattern-triggered immunity against Pseudomonas syringae in Arabidopsis. Plant J. (2020) 102:761–78. doi: 10.1111/tpj.14661
78. Tabarsa M, Shin I-S, Lee JH, Surayot U, Park W, You S. An immune-enhancing water-soluble α-glucan from Chlorella vulgaris and structural characteristics. Food Sci Biotechnol. (2015) 24:1933–41. doi: 10.1007/s10068-015-0255-0
79. Bardhan A, Abraham TJ, Das R, Patil PK. Visualization of poikilocytosis as an emerging erythrocytic biomarker for fish health assessment. Anim Res One Health. (2024) 2:136–57. doi: 10.1002/aro2.47
80. Thrall MA, Weiser G, Allison RW, Campbell TW. Veterinary Hematology and Clinical Chemistry. New Jersey: John Wiley & Sons (2012).
81. Barger AM. Erythrocyte morphology. In: Marjory B, Brooks DVM, Kendal E, Harr DVM, Davis M, Seelig DVM, Jane Wardrop K, Douglas J, Weiss DVM, editors. Schalm's Veterinary Hematology. John Wiley & Sons, Inc, New Jersey (2022). p. 188–97. doi: 10.1002/9781119500537.ch24
83. Witeska M. Erythrocytes in teleost fishes: a review. Zool Ecol. (2013) 23:275–81. doi: 10.1080/21658005.2013.846963
84. Aliko V, Morina V, Sula E. Cellular and nuclear alterations of carp fish (Cyprinus carpio) erythrocytes as sensitive biomarkers of citotoxicity and genotoxicity of sitnica river waters (Kosovo). Aktet. (2015) 8, 163–167.
85. Araki T, Hayashi M, Saruta T. Anion-exchange blocker enhances cytoplasmic vacuole formation and cell death in serum-deprived mouse kidney epithelial cells in mice. Cell Biol Int. (2006) 30:93–100. doi: 10.1016/j.cellbi.2005.10.020
86. Mohamed MG, el-Mahdy AF, Kotp MG, Kuo SW. Advances in porous organic polymers: syntheses, structures, and diverse applications. Mater Adv. (2022) 3:707–33. doi: 10.1039/D1MA00771H
87. Hotz MJ, Qing D, Shashaty MG, Zhang P, Faust H, Sondheimer N, et al. Red blood cells homeostatically bind mitochondrial DNA through TLR9 to maintain quiescence and to prevent lung injury. Am J Respir Crit Care Med. (2018) 197:470–80. doi: 10.1164/rccm.201706-1161OC
88. Anderson HL, Brodsky IE, Mangalmurti NS. The evolving erythrocyte: red blood cells as modulators of innate immunity. J Immun. (2018) 201:1343–51. doi: 10.4049/jimmunol.1800565
89. Ko SC, Kim D, Jeon YJ. Protective effect of a novel antioxidative peptide purified from a marine Chlorella ellipsoidea protein against free radical-induced oxidative stress. Food Chem Toxicol. (2012) 50:2294–302. doi: 10.1016/j.fct.2012.04.022
90. Vahdati SN, Lashkari A, Navasatli SA, Ardestani SK, Safavi M. Butylated hydroxyl-toluene, 2, 4-Di-tert-butylphenol, and phytol of Chlorella sp. protect the PC12 cell line against H2O2-induced neurotoxicity. Biomed Pharmacother. (2022) 145:112415. doi: 10.1016/j.biopha.2021.112415
91. Asharani PV, Wu YL, Gong Z, Valiyaveettil S. Toxicity of silver nanoparticles in zebrafish models. Nanotechnology. (2008) 19, 255102. doi: 10.1088/0957-4484/19/25/255102
92. Ribas JLC, da Silva CA, de Andrade L, Galvan GL, Cestari MM, Trindade ES, et al. Effects of anti-inflammatory drugs in primary kidney cell culture of a freshwater fish. Fish Shellfish Immunol. (2014) 40:296–303. doi: 10.1016/j.fsi.2014.07.009
93. Kasai H. What causes human cancer? Approaches from the chemistry of DNA damage. Genes Environ. (2016) 38:1–13. doi: 10.1186/s41021-016-0046-8
94. Xi L, Lu Q, Liu Y, Su J, Chen W, Gong Y, et al. Effects of fish meal replacement with Chlorella meal on growth performance, pigmentation, and liver health of largemouth bass (Micropterus salmoides). Anim Nutr. (2022) 10:26–40. doi: 10.1016/j.aninu.2022.03.003
95. Yu H, Liang H, Ge X, Zhu J, Wang Y, Ren M, et al. Dietary chlorella (Chlorella vulgaris) supplementation effectively improves body color, alleviates muscle inflammation and inhibits apoptosis in largemouth bass (Micropterus salmoides). Fish Shellfish Immunol. (2022) 127:140–7. doi: 10.1016/j.fsi.2022.06.017
96. Press CM, Evensen Ø. The morphology of the immune system in teleost fishes. Fish Shellfish Immunol. (1999) 9:309–18. doi: 10.1006/fsim.1998.0181
97. Whyte SK. The innate immune response of finfish–a review of current knowledge. Fish Shellfish Immunol. (2007) 23:1127–51. doi: 10.1016/j.fsi.2007.06.005
98. Steinel NC, Bolnick DI. Melanomacrophage centers as a histological indicator of immune function in fish and other poikilotherms. Front Immunol. (2017) 8:827. doi: 10.3389/fimmu.2017.00827
99. Dang M, Nørregaard R, Sonne C, Bach L, Stride M, Jantawongsri K, et al. Splenic and renal melanomacrophage centers in shorthorn sculpins (Myoxocephalus scorpius) in Nuuk harbor, West Greenland. Polar Biol. (2021) 44:2011–21. doi: 10.1007/s00300-021-02934-0
100. Hamed M, Said RE, Soliman HA, Osman AG, Martyniuk CJ. Immunotoxicological, histopathological, and ultrastructural effects of waterborne pyrogallol exposure on African catfish (Clarias gariepinus). Chemosphere. (2024) 349:140792. doi: 10.1016/j.chemosphere.2023.140792
101. Qualhato G, de-Sabóia-Morais SMT, Silva LD, Rocha TL. Melanomacrophage response and hepatic histopathologic biomarkers in the guppy Poecilia reticulata exposed to iron oxide (maghemite) nanoparticles. Aquat Toxicol. (2018) 198:63–72. doi: 10.1016/j.aquatox.2018.02.014
102. Flora Filho R, Zilberstein B. [amp]]Oacute;xido nítrico: o simples mensageiro percorrendo a complexidade. Metabolismo, síntese e funções. Rev Assoc Med Bras. (2000) 46:265–71. doi: 10.1590/S0104-42302000000300012
103. Rodrigues S, Antunes SC, Nunes B, Correia AT. Histopathological effects in gills and liver of Sparus aurata following acute and chronic exposures to erythromycin and oxytetracycline. Environ Sci pollut Res. (2019) 26:15481–95. doi: 10.1007/s11356-019-04954-0
104. Störmer J, Gwinner W, Derlin K, Immenschuh S, Rong S, Jang MS, et al. A single oral dose of diclofenac causes transition of experimental subclinical acute kidney injury to chronic kidney disease. Biomedicines. (2022) 10:1198. doi: 10.3390/biomedicines10051198
105. Ramos-Tovar E, Muriel P. Molecular mechanisms that link oxidative stress, inflammation, and fibrosis in the liver. Antioxidants. (2020) 9:1279. doi: 10.3390/antiox9121279
106. Mueller D, Müller-Vieira U, Biemel KM, Tascher G, Nüssler AK, Noor F. Biotransformation of diclofenac and effects on the metabolome of primary human hepatocytes upon repeated dose exposure. Eur J Pharm Sci. (2012) 45:716–24. doi: 10.1016/j.ejps.2012.01.014
107. Kottmann RM, Kulkarni AA, Smolnycki KA, Lyda E, Dahanayake T, Salibi R, et al. Lactic acid is elevated in idiopathic pulmonary fibrosis and induces myofibroblast differentiation via pH-dependent activation of transforming growth factor-β. Am J Respir Crit Care Med. (2012) 186:740–51. doi: 10.1164/rccm.201201-0084OC
108. El-Habashi N, Fadl SE, Farag HF, Gad DM, Elsadany AY, El Gohary MS. Effect of using Spirulina and Chlorella as feed additives for elevating immunity status of Nile tilapia experimentally infected with Aeromonas hydrophila. Aquac Res. (2019) 50:2769–81. doi: 10.1111/are.14229
109. Mohseni R, Arab Sadeghabadi Z, Karimi J, Gholami H, Ghasemi H, Ghadimipour HR, et al. Chlorella vulgaris supplementation attenuates the progression of liver fibrosis through targeting TGF-β-signaling pathway in the CCl4-induced liver fibrosis in rats. Toxin Rev. (2021) 40:1347–55. doi: 10.1080/15569543.2019.1700525
Keywords: non-steroidal anti-inflammatory drug, microalgae, african catfish, physiology, haemato-immunology, spleen
Citation: Gabr A, Mohamed AM, Abou Khalil NS and Sayed AE-DH (2025) The protective effect of Chlorella vulgaris against diclofenac toxicity in Clarias gariepinus: haemato-immunological parameters and spleen histological features as outcome markers. Front. Immunol. 16:1566496. doi: 10.3389/fimmu.2025.1566496
Received: 24 January 2025; Accepted: 10 March 2025;
Published: 31 March 2025.
Edited by:
Marco Albano, University of Messina, ItalyReviewed by:
Cristobal Espinosa Ruiz, Instituto Murciano de Investigación y Desarrollo Agrario y Alimentario (IMIDA), SpainCopyright © 2025 Gabr, Mohamed, Abou Khalil and Sayed. This is an open-access article distributed under the terms of the Creative Commons Attribution License (CC BY). The use, distribution or reproduction in other forums is permitted, provided the original author(s) and the copyright owner(s) are credited and that the original publication in this journal is cited, in accordance with accepted academic practice. No use, distribution or reproduction is permitted which does not comply with these terms.
*Correspondence: Alaa El-Din H. Sayed, YWxhYXNheWVkQGF1bi5lZHUuZWc=
†ORCID: Alaa El-Din H. Sayed, orcid.org/0000-0001-7487-4729
Disclaimer: All claims expressed in this article are solely those of the authors and do not necessarily represent those of their affiliated organizations, or those of the publisher, the editors and the reviewers. Any product that may be evaluated in this article or claim that may be made by its manufacturer is not guaranteed or endorsed by the publisher.
Research integrity at Frontiers
Learn more about the work of our research integrity team to safeguard the quality of each article we publish.